Abstract
Glarea lozoyensis is an anamorphic ascomycete that produces pneumocandin B0, the starting molecule for the synthesis of the antifungal drug caspofungin (CANCIDAS™). Glarea lozoyensis was first isolated in 1985 from a water sample from Madrid, Spain. Until now, only the original strain was known, but we have discovered new strains from Argentina and the USA. Molecular phylogenetic reference to a 28S rDNA database of antibiotic-producing fungi quickly identified these strains as being conspecific with G. lozoyensis. Bayesian inference phylogeny of ITS, 28S rDNA and α-actin gene fragments revealed that G. lozoyensis is related to species of the genus Cyathicula (Helotiales). Glarea lozoyensis was not conspecific with any of the Cyathicula species sequenced, although it appears to share a common ancestor. Glarea lozoyensis and Cyathicula strains were fermented on nutritional microarrays in 96-well plates. Cyathicula extracts did not show antifungal activity and did not produce pneumocandins, whereas potent antifungal activity and pneumocandin A0 production were confirmed for the four G. lozoyensis isolates. Also, culture morphology differed, with G. lozoyensis strains producing a dark brown, profusely sporulating mycelium with pigmented multicellular conidia accumulating in conidial masses, while all Cyathicula species tested in culture formed hyaline to light brown mycelia and lacked conidia. The chemistry and taxonomic distribution of the echinocandin class of antifungals is comprehensively reviewed.
Introduction
Glarea lozoyensis Bills and F. Peláez is a medically and biosynthetically significant anamorphic ascomycete that produces naturally antifungal lipopeptides, named pneumocandins, which act by inhibiting fungal β-(1,3)-glucan synthesis. Caspofungin acetate, a semisynthetic derivative of pneumocandin B0, marketed as CANCIDAS™ since 2001, has made an invaluable contribution in the field of antifungal therapy and treatment of serious life threatening fungal infections (McCormack and Perry Citation2005). Although the echinocandin class of antifungal lipopeptides was first discovered in the early 1970s and consisted of a large number of structural analogs of echinocandin B (Benz et al. Citation1974), it was not until 2001, when the use of caspofungin acetate was pioneered in the clinic, that the global impact of the class was recognized. Since the introduction of caspofungin, two other echinocandins, micafungin (MYCAMINE™) and anidulafungin (ERAXIS™) have been introduced into the clinic. These antibiotics all function by the same mechanism, inhibiting formation of fungal cell wall, which presumably accounts for their minimal side-effects in humans (Morrison Citation2006).
Glarea lozoyensis was first isolated from a water sample from Madrid, Spain in 1985, and until now the fungus was thought to be rare and possibly endemic to Spain because only the original strain was known. Initially identified as Zalerion arboricola Buczacki by the Centraalbureau voor Schimmelcultures (CBS) (Schwartz et al. Citation1989, 1992), the pneumocandin-producing fungus was later shown to be very different to authentic Z. arboricola strains by analyzing a combination of morphological and molecular data, and the fungus was described as a new anamorphic genus and species (Bills et al. Citation1999). Analysis of the limited number of publically available ITS sequences at that time suggested the phylogenetic relationships of this fungus with some fungi within the order Helotiales, particularly with several Hymenoscyphus species, and that the fungus was unrelated to the type species of genus Zalerion, Z. maritimum, the anamorph of Lulwoana uniseptata (Nakagiri) Kohlm (Nakagiri Citation1984; Campbell et al. Citation2005). As yet, no further species have been described in Glarea.
We report that we have found more strains, which can be unambiguously assigned to G. lozoyensis based on a combination of morphological, metabolic and molecular data. Extensive sequencing of the ITS region from a broad array of species in the Helotiales allowed us to narrow the closest relatives of G. lozoyensis to some species of Cyathicula De Not. (Helotiaceae). Although current usage considers Cyathicula a synonym of Crocicreas, we have chosen to use the generic name Cyathicula and maintain the genera separate for the reasons previously discussed (Baral Citation1994; Triebel and Baral Citation1996; Iturriaga et al. Citation1999). The close kinship between G. lozoyensis and Cyathicula species inferred from similarity of the ITS region was confirmed by sequencing of the D1-D2 region of the 28S rRNA gene and the α-actin gene, and our analyses strongly suggest that G. lozoyensis shares a common ancestor with Cyathicula species. However, no anamorph states have been reported to date from Cyathicula species. Therefore, how and why a predominantly anamorph species would be evolve among predominantly teleomorph fungi poses several interesting evolutionary questions.
Materials and methods
Collection and isolation of strains
Origins of the strains used in this study are listed in . The type strain of the pneumocandin-producing fungus, G. lozoyensis (ATCC 20868 = MF5171 = CBS 492.88), was isolated by plating filtrates of pond water (Bills et al. Citation1999). New G. lozoyensis strains (F-226836, F-226838, F-239379) were recovered from soil and plant debris using an extinction culturing method developed in our laboratory for the high throughput isolation of fungi from organic particles (Collado et al. Citation2007). Fungi were isolated in 48-well tissue culture plates containing 1 ml per well of YM medium (yeast extract 1 g, malt extract 10 g, dichloran 1 mg, agar 15 g, distilled water 1 l) emended with streptomycin 50 mg, oxytetracycline 50 mg and cyclosporin A 4 mg, added after autoclaving.
Table 1. Strains and sequences. GenBank entries with FJ accession codes are new sequences obtained during this work; those with DQ codes are from Baral et al. (2006).
Cyathicula species were cultivated from field-collected apothecia growing on decayed plant material. Monosporic cultures were obtained from ascospores separated from the apothecia with a micromanipulator on CMA plates (Difco cornmeal agar with 50 mg l–1 streptomycin and oxytetracycline). It must be stressed that some collections were difficult to unambiguously ascribe to a taxon, because some of them were studied only in the dry state obscuring vital characters (Baral Citation1992), or were maintained at cold temperatures for several days before microscopic study and were, therefore, in unfavorable condition (i.e. overmature spores). Apart from such suboptimal conditions, variation and lack of clear diagnostic characters in the Cy. cyathoidea aggregate prevented unambiguous classification of the strains.
Axenic cultures of all strains were obtained after 3 weeks incubation at 22 °C and 80% relative humidity in YM medium with no antibiotics. Strains were preserved as frozen mycelial discs in 10% glycerol at –80 °C. Strains are maintained in the collection of Fundación MEDINA, Granada, Spain, unless designated otherwise.
Other strains studied
The grass endophyte (IZ 0792) was provided by the Instituto de Recursos Naturales y Agrobiología de Salamanca, Consejo Superior de Investigaciones Científicas, Spain (Sánchez Márquez et al. Citation2010). The strain of Cy. coronata was obtained from CBS (CBS 19762). Pezizella amenti (HB 6884) and Hymenoscyphus epiphyllus (HB 7054) were from H.O. Baral's private collection in Germany.
Strain characterization
The isolates were grown for 5 weeks at 22 °C and 80% relative humidity on 90-mm Petri dishes containing YM, OTM (oatmeal agar, Difco) and CMA. To induce the production of conidia, Cyathicula isolates were cultured for an additional 4 weeks with continuous exposure to a UV light source. Micromorphology was examined with a Leitz Diaplan or a Zeiss Axioskop microscope equipped with differential interference contrast optics.
DNA extraction, PCR amplification and sequencing
Genomic DNA was extracted from mycelia of agar cultures (Peláez et al. Citation1996). The ITS1-5.8S-ITS2 region was amplified using universal primers ITS5 (White et al. Citation1990) and ITS4a (Larena et al. Citation1999). The partial 28S rDNA sequence was amplified with universal primers NL1 and NL4 (O'Donnell Citation1993). The α-actin gene fragment was amplified using primers ACT-512F/ACT-783R (Carbone and Kohn, Citation1999). PCR reactions were carried out following standard procedures (40 cycles of 30 s at 93 °C, 30 s at 53 °C and 2 min at 72 °C). About 0.1 μg ml–1 of the double-stranded amplification products were sequenced using the ABI PRISM Dye Terminator Cycle Sequencing Ready Reaction Kit (Perkin Elmer, Norwalk CT) following the manufacturer's recommended procedures. Purified PCR products were directly sequenced using the same primer pairs as in the PCR reactions. Partial sequences obtained in sequencing reactions were assembled with Genestudio 2.1.1.5. (Genestudio, Inc., Suwanee, GA, USA). All sequences were deposited in GenBank ().
Phylogenetic reconstructions
Recognition of new strains of G. lozoyensis was guided by searches and alignments of a database of 28S rRNA sequences of nearly 3000 strains of antibiotic-producing fungi compiled in our laboratory (Bills et al. Citation2009). For more precise phylogenetic analyses, two separate alignments were made, one with the sequences of the ITS1-5.8S-ITS2 region combined with the D1–D2 domain of the 28S rRNA gene, and another one with the sequences of the α-actin fragment. DNA sequences were aligned with Genestudio 2.1.1.5. Due to some ambiguously aligned regions in the α-actin gene fragment, the application GapCoder (Hennequin et al. Citation2003; Young and Healy Citation2003) was used to recode the ambiguous regions without violating positional homology, to improve the quality of the alignment. The alignments were deposited in TreeBASE with numbers SN3989–19084 (ITS-28S combined dataset) and SN3989–19083 (α-actin dataset).
Bayesian analysis based on the Markov chain Monte Carlo (MCMC) approach was run in the program MrBayes 3.01 (Ronquist and Huelsenbeck Citation2003). To improve mixing of the chain, four incrementally heated simultaneous Monte Carlo Markov chains were run over 2,000,000 generations. MrModeltest 2.2 (Nylander Citation2004) was used to perform hierarchical likelihood ratio tests to calculate the Akaike Information Criterion (AIC) and hierarchical likelihood ratio tests (hLRTs) values of the nucleotide substitution models. The model selected by hLRT and AIC for both the alignment of the ITS-28S gene fragment and the α-actin gene fragment was the GTR+I+G model. This model allowed for six classes of substitution types, a portion of invariant alignment positions, and mean substitution rates varying across the remaining positions according to a gamma distribution. Priors used for the MCMC process were a Dirichlet distribution for substitution rates and nucleotide frequencies and a uniform prior for the rate parameter of the gamma distribution (Ronquist et al. Citation2009). The MCMC analysis for both trees used the following parameters: sampling frequency = 100; 1000 trees were discarded for the consensus tree; the resulting consensus tree was a majority rule consensus tree including compatible groups of lower frequencies.
Production cultures and extract preparation
Mycelia discs from YM agar cultures were used to inoculate tubes (25×150 mm) containing 8 ml of SMYA medium (Difco neopeptone 10 g, maltose 40 g, Difco yeast extract 10 g, agar 4 g, distilled H2O 1 l) and two cover glasses (22 mm2). Tubes were incubated in an orbital shaker at 200 rpm for 4–6 days at 22 °C. Inoculum cultures were arrayed in a 96-deepwell plate which was replicated across eight 96-deepwell plates containing each a different production medium (Bills et al. Citation2008). The production media formulations have been described previously (Vicente et al. Citation2009). Each production plate was replicated three times to assess the consistency of the results.
A total of 900 μl of the acetone medium supernatant from each well was transferred to 2-ml wells of a recipient plate. To minimize metabolite precipitation during solvent evaporation, 100 μl of DMSO was added to the 900-μl of the culture extract. The culture–solvent–DMSO mixture was reduced to 50% of its original volume by vacuum evaporation. After solvent removal, approximately 500 μl of aqueous extract with 20% DMSO remained per well, and extracts were stored at –20 °C until tested. Extract plates were thawed and briefly shaken on a plate mixer prior to assay. Fermentations yielding a positive antifungal phenotypes were repeated one or more times to confirm bioactivity and analytical chemistry.
Evaluation of antimicrobial activity
Antimicrobial activities of fungal extracts were determined using two species of human pathogenic bacteria, one yeast and one filamentous fungal strain from the Merck Culture Collection ( and 3). Antifungal susceptibility was tested with the strains Candida albicans MY1055 and Aspergillus fumigatus MF5668. For Ca. albicans, thawed stock inoculum suspensions from cryovials were streaked on Sabouraud dextrose agar plates (SDA, 65 g l–1) to grow new colonies. A few of the colonies were inoculated into 10 ml of Sabouraud dextrose broth (30 g l–1 H2O) that was incubated overnight at 37 °C. The Ca. albicans suspension was adjusted to an optical density of 0.4 at 660 nm. This suspension was added to yeast nitrogen base-dextrose agar (yeast nitrogen base 6.75 g l–1, dextrose 1 g l–1, agar 17.5 g l–1 H2O) in the proportion of 30 ml l–1. Twenty-milliliter aliquots of the seeded agar media were poured into Nunc OmniTray™ plates. Wells (4 mm diameter) were formed in the agar with a removable pin lid. Aqueous acetone-derived extracts (10 μl) were applied into the wells of the seeded assay plates, which were incubated at 30 °C for approximately 20 h. Amphotericin B (0.25 μg ml–1) and kanamycin (5 μg ml–1) were used as positive and negative internal plate controls, respectively.
Table 2. Antimicrobial activity of four Glarea lozoyensis strains. The production media where some activity was detected is indicated in parentheses in each case. Codes for agar diffusion assays: A (clear inhibition zone >7 mm); B (clear inhibition zone < 7 mm or hazy inhibition zone >10 mm). See Vicente et al. (Citation2009) for media formulations.
For A. fumigatus, lyophilized stock culture were resuspended into 1–2 ml of sterile H2O, rehydrated for about 1 h and 0.4 ml were spread onto several potato dextrose agar (PDA) plates. Plates were incubated for 4 days at 28 °C, and a heavy inoculum of mycelium and spores was collected and transferred to three or four fresh PDA plates. The new plates were incubated for another 5 days, and the fungus was transferred as above onto fresh PDA plates prior to the preparation of the assay plates. The fungal spore suspension was diluted to 65% transmittance at 660 nm to prepare the assay plates, which were prepared with medium yeast nitrogen base-dextrose agar (as described for Ca. albicans) and incubated at 28 °C. Assay plates were prepared in Nunc OmniTray™ plates as described above for Ca. albicans, 10 μl samples were applied into the wells and the plates were incubated for approximately 20 h. Positive and negative controls were the same as for Ca. albicans.
Antibacterial susceptibility was tested with the strains Haemophilus influenzae MB5842 and Staphylococcus aureus EP167. For H. influenzae, Chocolate Agar plates were inoculated from frozen stocks for isolated colonies. The plates were incubated for 20–24 h at 37 oC in 5% CO2 and 90% humidity, and 250-ml Erlenmeyer flasks containing 25 ml of Haemophilus Test Medium (HTM) broth plus HTM supplement were inoculated with isolated colonies. Cultures were grown statically for 20–22 h at 37 oC in 5% CO2 and 90% humidity. The culture was diluted in HTM broth and adjusted to an optical density of 0.4 at 660 nm, and 3.3 ml of the diluted culture was mixed with 100 ml HTM agar plus 400 μl HTM supplement, molten at 48 °C. Assay plates were prepared as described above for Ca. albicans; 10 μl samples were applied in each well and plates were incubated for 17–18 hours at 37 oC in 5% CO2 and 90% humidity. Antibiotics with either positive or negative responses were used as internal plate controls (ampicillin 1 mg ml–1, ciprofloxacin 0.5 μg ml–1 and clarithromycin 750 μg ml–1).
For S. aureus, thawed stock inoculum suspensions from cryovials were streaked onto Luria Broth (LB) agar plates containing 0.2% glucose and 15 μg ml–1 chloramphenicol, which were incubated at 37 °C overnight to obtain isolated colonies. Single colonies were selected to inoculate an overnight culture to be used as assay plate inoculum. The single colonies were inoculated into 10 ml LB medium containing 34 μg ml–1 chloramphenicol in 250-ml Erlenmeyer flasks. The flasks were incubated and agitated at 220 rpm overnight at 37 °C. To prepare the assay plates, the LB agar was supplemented with glucose (0.2% final), xylose (50 mM) and chloramphenicol (15 μg ml–1). The medium was inoculated to 0.1% final with the overnight cultures (1:1000 dilution in the agar). Assay plates were prepared as described above for Ca. albicans and 10 μl samples were applied in each well and plates were incubated for 16–18 hours at 37 °C. Penicillin G (5 μg ml–1) and amphotericin B (1 μg ml–1) were used as positive and negative controls, respectively. Zones of inhibition (ZOIs) were recorded by automated image analysis. The tests were duplicated with the two acetone extracts prepared for each sample. Measurements were usually very similar (differences <3 mm of ZOI), and the two values were averaged. Any extract producing a discernable ZOI ≥ 7 mm, regardless of zone quality, was considered to be positive.
LC–MS analysis of extracts
In a first line identification, 2 μl of the active extracts selected in the antibiotic assays were examined by liquid chromatography–mass spectrometry (LC–MS) and compared to a proprietary database, which in many cases yielded a positive match to a known antibiotic or metabolite. Analysis was performed on an Agilent (Santa Clara, CA, USA) 1100 single quadrupole LC–MS, using a Zorbax SB-C8 column (2.1×30 mm), maintained at 40 °C and with a flow rate of 300 μl min–1. Solvent A consisted of 10% acetronitrile and 90% water with 1.3 mM trifluoroacetic acid and ammonium formate, and solvent B was 90% acetronitrile and 10% water with 1.3 mM trifluoroacetic acid and ammonium formate. The gradient started at 10% B and went to 100% B in 6 min, kept at 100% B for 2 min and returned to 10% B for 2 min to initialize the system. Full diode array UV scans from 100 to 900 nm were collected in 4-nm steps at 0.25 s per scan. The eluting solvent was ionized using the standard Agilent 1100 electrospray ionization source adjusted to a drying gas flow of 11 l min–1 at 325 °C and a nebulizer pressure of 40 psig. The capillary voltage was set to 3500 V. Mass spectra were collected as full scans from m/z 150 to m/z1500, with one scan every 0.77 s, in both positive and negative modes. Database matching was performed using an in-house developed application where the DAD, retention time, positive and negative mass spectra of the active samples were compared to the UV–LC–MS data of known metabolites stored in a proprietary database (Vicente et al. Citation2009; Bills et al. Citation2009) where metabolite standard data were obtained using the exact same LC–MS conditions as the samples under analysis. Presence and relative abundance of different penumocandins was verified in pneumocandin-positive fermentation extracts by HPLC–high resolution electrospray ionization–mass spectrometry recorded on a Bruker maXisTM QTOF-MS instrument (Bruker Daltronics GmbH, Bremen, Germany) coupled to the same HPLC system as described above.
Results
Discovery of new strains of Glarea lozoyensis
By screening crude fungal fermentation extracts, we found three strains producing a strong antifungal activity against Ca. albicans when grown in multiple production media (). Two of the strains also produced some activity against A. fumigatus. For each fungus exhibiting an antifungal phenotype during screening of crude fungal fermentation extracts for antifungal activity, a 28S rRNA barcode was generated. The 28s rDNA barcodes were automatically aligned to database of 28S rRNA of nearly 3000 strains of antibiotic-producing fungi compiled in our laboratory. The sequences from the three new strains aligned to the type strain of G. lozoyensis (Bills et al. Citation2009). Microscopic examination of these strains revealed the characteristic multicellular conidia suggesting conspecificity with the original strain of G. lozoyensis ATCC 20868 (). As cultures mature, the conidia continue to divide, multiplying meristematically and accumulating as conspicuous conidial masses on the aerial hyphae (). These three strains were isolated from soil or leaf litter from locations distant (Santa Cruz province, Argentina and New Hampshire, USA) from that of the type strain of G. lozoyensis, isolated from a water sample collected in Madrid, Spain ().
Figure 1. Developing conidiophores and conidia from four strains of Glarea lozoyensis. A. ATCC 20868=F-160870. B. F-226836. C. F-226838. D. F-239379. Scale bar = 20 μm.
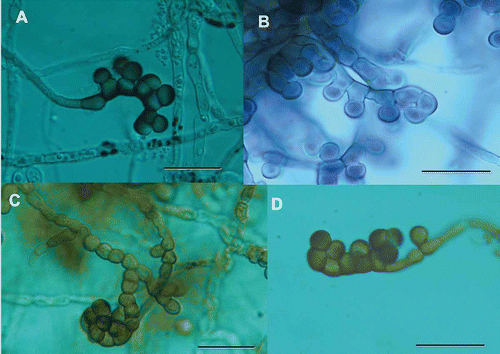
Figure 2. Accumulation of conidial masses on aerial hyphae of Glarea lozoyensis. Strains were grown on YM medium one month at room temperature. A. ATCC 20868=F-160870. B. F-226836. C. F-226838. D. F-239379. Scale bar = 2 mm.
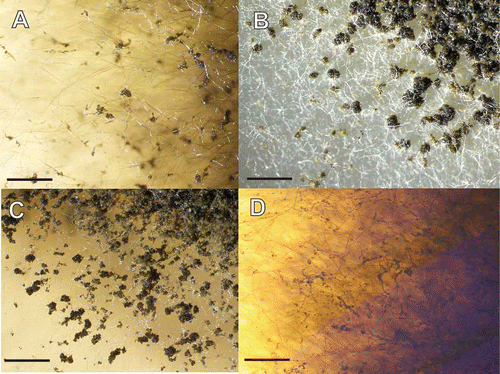
LC–MS analysis detected pneumocandin A0 in principal pneumocandin in these antifungal extracts (). Titres of pneumocandin A0 varied among the four strains, with highest production observed in the Argentinian strains. Production by the New Hampshire strain was difficult to discern by UV spectra due to low titre, but positive ion signals and antifungal activity indicated its presence (). The detection of pneumocandin A0 in all strains by low resolution measurements was verified in high-resolution electrospray mass spectra. Furthermore, high resolution spectra verified the presence of pneumocandin A1 in all strains, and pneumocandins B0 in F–160,870, F-226,836, and F-226,936 (data not shown).
Figure 3. Identification of pneumocandin A0 in fermentation extracts of strains of Glarea lozoyensis. A1. UV spectrum of pneumocandin A0 with retention time of elution (4.78 min). A2. Positive ion mass spectrum of pneumocandin A0 (MW=1078 Da) at the same retention time. The combination of A1and A2 produce the fingerprint of pneumocandin A0. See methods for LC–MS protocols. B1, C1, D1 and E1 are the UV spectra of extracts of the four strains at the corresponding retention time, and B2, C2, D2 and E2 are the positive ion mass spectra of the respective sample at the same retention time as for the UV spectrum. All UV spectra were similar to authentic pneumocandin A0, except for E1 where the signal was near baseline. Similarly, all the positive ion mass spectra indicated pneumocandin A0 was in all samples.
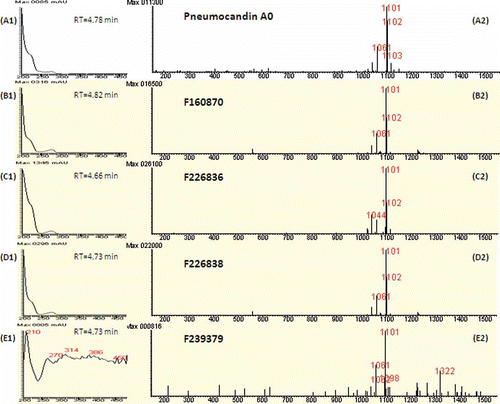
Phylogenetic analyses
BLAST searching of the ITS1-5.8S-ITS2 sequences of these four strains against a large number of species within the Helotiales from our own sequence database identified several Cyathicula species as their closest relatives. To more accurately delineate the boundaries between G. lozoyensis and species of Cyathicula, additional Cyathicula strains were isolated from field-collected specimens and their ITS region, the D1–D2 region of the 28S rRNA, and the α-actin gene were sequenced. A total of 25 strains corresponding to seven species referable to Cyathicula, together with an unidentified endophytic fungus from the grass Holcus lanatus, and five Hymenoscyphus species, were included in the analysis. A strain of Ciboria americana was designated the outgroup. The size of the amplified fragments were 464–481 bp for the ITS1-5.8-ITS2 region, 570–571 bp for the D1-D2 region of the 28S rRNA gene and 273–301 bp for the α-actin fragment.
The first phylogenetic analysis was based on the ITS-28S rRNA gene combined dataset (). The alignment consisted of 1040 nucleotides, 749 of these characters being constant. The three new pneumocandin-producing isolates appeared as a monophyletic group together with the type strain of G. lozoyensis in a clade with full statistical support, confirming the conclusion based on morphological observation. The four sequences were almost identical (<0.3% of nucleotide divergence). This clade was embedded within a larger statistically supported monophyletic clade containing all the Cyathicula species sequenced.
Figure 4. Phylogenetic tree of Glarea lozoyensis and related species generated by Bayesian analysis of combined ITS and 28S rRNA partial sequences. Ciboria americana was designated the outgroup. Clade credibility values are indicated at the branches. Colony morphology of G. lozoyensis and Cyathicula strains are mapped onto their corresponding branches.
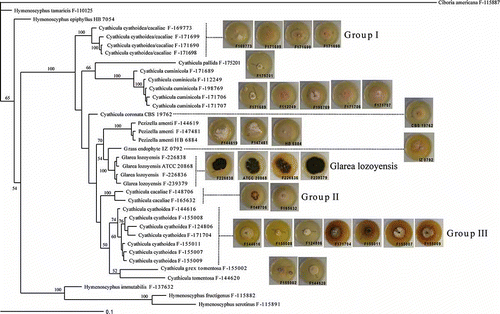
The closest sequence to G. lozoyensis was that of an unidentified grass endophyte (IZ 0792), although the node was weakly supported (54%). This nucleotide sequence diverged 1.5–1.7% with respect to the G. lozoyensis sequences. The next related species was Pezizella amenti (2.2–2.5% nucleotide divergence). All these strains aggregated in a clade with relatively modest support (70%). No evidence of any geographic pattern of diversity in G. lozoyensis was suggested in the tree. Infraspecific groupings were not evident; the two strains from Argentina were equidistant to the strains from Spain and New Hampshire.
Strains of P. amenti and Cy. culmicola appeared as monophyletic groups with strong statistical support. However, additional clades could not be unequivocally correlated with known species based on their morphological features. Particularly, the application of the names Cy. cyathoidea and Cy. cacaliae to our collections proved to be difficult. Those collections with a primarily brownish excipulum (i.e. Cy. cacaliae) clustered in two different clades (Groups I and II; ), while those with a hyaline excipulum (but often turning brownish by oxidation of the cell contents), which would be ascribed to Cy. cyathoidea, were also found in two different clades (Groups I and III; ). We, therefore, have exercised caution in naming the strains within these clades, referring to them only tentatively as Cy. cyathoidea/cacaliae (Group I), Cy. cacaliae (Group II), and Cy. cyathoidea (Group III). The seven Cy. cyathoidea strains in Group III, being similar to Group I and morphologically consistent with the current concept of Cy. cyathoidea, appeared grouped in a single monophyletic clade with moderate statistical support. The nucleotide divergence was also very low in this group (<1%). However, cultural patterns found across this group of strains differed significantly, particularly in the growth rates and the production of diffusible pigments.
Support for grouping the two strains of Cy. tomentosa was very weak. However, one of the strains (labeled as Cy. grex tomentosa) was a morphologically atypical specimen because it deviated by pale coloured apothecia, which may have placed it closer to typical Cy. cyathoidea. Nucleotide divergence at the infraspecific level was very low (<1.2%) within all these monophyletic clades, except for the two sequences of Cy. tomentosa, which differed by 4.6%. Clades encompassing potentially related species were poorly supported, therefore the tree failed to yield much useful information on the relationships among Cyathicula species.
A second phylogenetic analysis was based on α-actin partial sequences (). One of the Cy. culmicola isolates (F-171689) failed to yield an amplification product and was excluded from the analysis. The alignment consisted of 317 characters, 133 of which were constant. Again, the four strains of G. lozoyensis appeared together in a monophyletic group with strong statistical support, the nearest sequence being that of the unidentified grass endophyte. Statistical support for this node was higher than in the ITS-28S tree (77%). Also, P. amenti appeared as a sister group with moderate statistical support (68%). Actin sequences were more heterogeneous than those from the ITS-28S combined dataset, with nucleotide divergence ranging 0.4–7.3% for the G. lozoyensis strains, spanning up to 15.0–15.4% when compared to the grass endophyte and to more than 22% compared with P. amenti. Within the G. lozoyensis clade, two infraspecific clades were strongly supported, one containing the two strains from Argentina, and the second one the strains from Spain and New Hampshire. Correspondingly, nucleotide divergence within each of these two groups was significantly lower than between the groups (0.4–2.2% versus 6.2–7.3%).
Figure 5. Phylogenetic tree of Glarea lozoyensis and related species generated by Bayesian analysis of α-actin gene partial sequences. Clade credibility values are indicated at the branches.
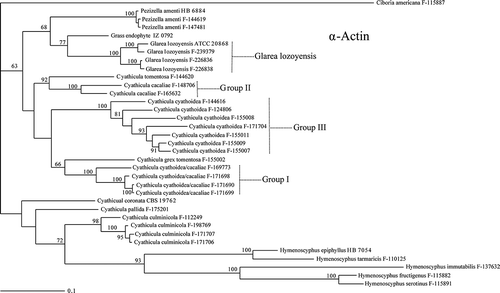
In the α-actin-based phylogenetic tree, the species of Cyathicula did not constitute a monophyletic group; several Hymenoscyphus species appeared in an internal branch related to some Cyathicula species. Consistent with the ITS-28S based phylogenetic tree, all the morphological Cyathicula species (including Groups I, II and III) appeared as monophyletic groups with strong statistical support, with the only exception of the two strains of Cy. tomentosa. Infraspecific nucleotide divergence was <10% for all the species analyzed, except for the two Cy. tomentosa strains (17.2%) and for the Cy. cyathoidea (Group III) strains (2.9–14.8%). Again, there was little or no statistical support for any clades containing related species, the only exception being a branch containing Cy. cacaliae and one of the strains of Cy. tomentosa. All the Cyathicula strains were examined morphologically, and the gross morphology of their colonies differed significantly from those of G. lozoyensis strains (). The lightly coloured, pale buff to yellowish buff pigmentation in the Cyathicula isolates contrasted sharply with the strongly pigmented brown to blackish brown colonies exhibited by all the G. lozoyensis strains. Microscopic analysis of the Cyathicula isolates failed to identify any anamorphic state with conditions used in this study.
Antimicrobial activity of Cyathicula species and Glarea lozoyensis
The Cyathicula strains were analyzed for their ability to cause antimicrobial inhibition and specifically for detection of pneumocandins by mass spectroscopy. To facilitate direct comparison, the strains were grown into deepwell plates, using culture media and conditions that supported successfully the production of pneumocandin by G. lozoyensis. Some of the Cyathicula species showed modest antibacterial activity, mostly against Haemophilus influenza (). One of the Cyathicula strains (Cy. cacaliae F-165632) produced some activity against Ca. albicans in one of the media used, and another strain (Cy. culmicola F-171706) produced a very weak activity against A. fumigatus, but none of them produced the strong antifungal signal in multiple media against Ca. albicans and A. fumigatus that was typically associated with the production of pneumocandins (). Conspecific strains did not show identical patterns of biological activity, although differences might be explained in some cases by the different production media used to culture these isolates. The only strain that produced a potent antifungal activity, comparable to that seen in the G. lozoyensis strains, was the grass endophyte IZ 0792. However, LC–MS analysis failed to recognize neither pneumocandins nor any other antifungal compounds included in our databases in the extracts from this fungus or any of the others (data not shown).
Table 3. Antimicrobial activity of Cyathicula and Pezizella strains against a panel of bacteria and fungi. The media where activity was detected are indicated in parentheses in each case. Media formulations have been previously reported (Vicente et al. Citation2009). Codes for agar diffusion assays: A (clear inhibition zone >7 mm); B (clear inhibition zone < 7 mm or hazy inhibition zone >10 mm); C (hazy inhibition zone < 10 mm).
Discussion
Echinocandins are important molecules from the medical perspective owing to their antifungal activity, which is mediated by inhibition of the glucan synthesis in susceptible yeasts and filamentous fungi. Semisynthetic members of the class currently marketed include CANCIDAS™ (caspofungin acetate); MYCAMINE™ (micafungin,) and ERAXIS™ (anidulafungin) (McCormack and Perry Citation2005; Wiederhold and Lewis Citation2007; Joseph et al. Citation2008). The discovery of pneumocandin B0 from G. lozoyensis and other echinocandins illustrates yet another example that potentially high value microbial biosynthetic pathways are usually widespread. Other valuable fungal metabolites that have been developed into drugs, e.g. cyclosporine A and lovastatin, have cosmopolitan distributions. In all these cases, the recognition of market needs and subsequent discovery of the potential application of a fungal metabolite to interfere with a drug target were critical to attaining commercial success, rather than access to rare and geographically restricted genetic resources.
Why were the pneumocandins and the sulfated echinocandins not discovered earlier? Antibiotic discovery programs have intensively screened fungi for antifungal agents since at least the early 1950s. Fungi producing pneumocandins and echinocandins produce easily detectable and very potent antifungal activity against yeasts and filamentous ascomycetes, often under a variety of fermentation conditions (Dreyfuss Citation1986; Bills et al. Citation2008). However, the first echinocandin class to be discovered was the echinocandins from the soil fungus Emericella rugulosa (Benz et al. Citation1974) and were soon followed by the aculeacins from another soil fungus, Aspergillus aculeatus (Mizuno et al. Citation1977; Saito et al. 1977). This was partly due to the fact that most fungi producing these molecules were probably not easily recovered from soil by typical dilution plating techniques. Their discovery coincided with deeper probing of fungal biodiversity during the 1980s and 1990s, especially fungi associated with plants, and use of fungal isolation methods suited for slower growing fungi that may not necessarily producing easily germinating spores (Dreyfuss Citation1986: Bills et al. Citation2009). Other contributing factors may have been the introduction of improved extraction and assay methods for water-soluble metabolites, in the case of the sulfated echinocandins (Hino et al. Citation2001), and assay methods specific for detecting antibiosis mediated by fungal cell wall perturbations. The specificity of the pneumocandins and echinocandins for fungal 1,3-β-d-glucan synthase, along with their widespread distribution among many types of filamentous ascomycetes, points to their natural role in antifungal-mediated antagonism of other fungi and yeasts. However, experimental tests of the hypothesis in natural ecosystems remain to be carried out.
Although echinocandins are produced by different ascomycetes, they share basic structural features – the presence of a hexapeptide core N-acylated with a fatty acid side chain (). The biosynthesis of echinocandins is non-randomly distributed and primarily concentrated among, but not restricted to, species of Eurotiales and the Helotiales ( and 5). Until the biosynthethic pathways responsible for different echinocandins are fully understood, correlations between the different structures and the evolutionary relationships of the fungi producing them remain unclear. The most evident patterns perhaps are that echinocandins produced by members of the Eurotiales seem to be united by the absence of glutamine or hydroxyglutamine in position R8 and that the pneumocandin family is restricted to the Helotiales ( and ).
Figure 6. Structure of the echinocandins showing the common features and the radicals varying across the different molecular subtypes. Refer to for R-group substitutions.
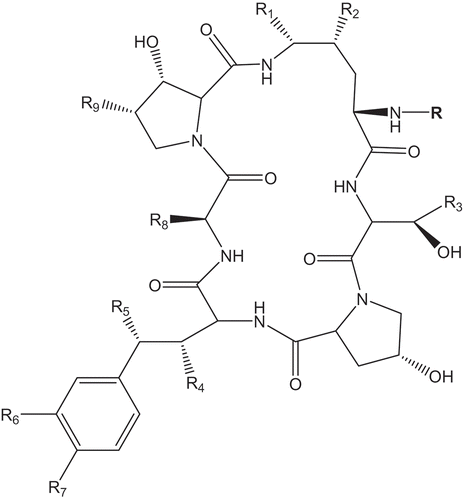
Table 4. Naturally occurring echinocandins and producing organisms.
Table 5. Naturally occurring echincocandin subtypes and variations in lipophilic sidechains and substitutions. See for positions of R groups.
Glarea lozoyensis is not the only member of the Helotiales reported to produce pneumocandins or other echinocandins (). Within the order Helotiales, Cryptosporiopsis species (and its teleomorph Pezicula, Dermateaceae) seem to be particularly apt at producing echinocandins. Cryptosporiopsis or Pezicula strains have been reported in the literature producing pneumocandin A0 (Noble et al. Citation1991) and the serine analog of pneumocandin A0, named sporiofungin (Dreyfuss Citation1986), as well as cryptocandin (Strobel et al. Citation1999). Moreover, in our own screening, we have identified other Cryptosporiopsis and Pezicula species that produce pneumocandin A0 (Bills et al. Citation2009). Interestingly, the production of another echinocandin containing a sulphate group in the homotyrosine residue (compound FR227673 or WF22210) has been reported from a strain assigned to the anamorphic genus Chalara (Kanasaki et al. 2006; Hino et al. Citation2001). Chalara species have been described as anamorphs of species within several ascomycete orders, especially the Helotiales (Kowalski and Holdenrieder Citation2009; Cai et al. Citation2009). Gams and Philippi (Citation1992) reported a Chalara anamorph in a species sometimes considered close to Cyathicula, Antinoa strobilina (Fr.) Velen. (≡ Cy. strobilina (Fr.) Korf. and Dixon (Dixon, Citation1974)), although this species appears to be more related to Calycina Nees ex Gray and Calycellina Höhn. (Hyaloscyphaceae), in which Chalara anamorphs occur (Baral Citation1989, Citation2002). In any case, to our knowledge, the Chalara strain reported to produce a sulphated echinocandin has not undergone DNA-based phylogenetic analysis; therefore, its phylogenetic affinities remain unknown.
We have revealed that G. lozoyensis is more broadly geographically distributed than initially thought, as demonstrated by the isolation of three additional strains from cool temperate locations in Patagonian Argentina and New Hampshire, and very distant from Madrid. Furthermore, the actin-based phylogeny suggested some degree of geographic divergence among the limited set of isolates, with the four strains separated in two well supported monophyletic groups, one containing the two strains from Argentina and the other the two isolates from the Northern Hemisphere. Molecular phylogenetic analysis indicated that G. lozoyensis shares a common ancestor with species of genus Cyathicula, although it is unique among the set of Cyathicula species both in morphology (dark pigmentation and production of multicellular conidia) and antifungal secondary metabolite production. Although some of the Cyathicula strains tested produced some antibacterial activity, they did not produce pneumocandins in growth conditions that supported the production of pneumocandins by G. lozoyensis. The grass endophyte IZ 0792 was most likely another Cyathicula species. The cultural morphology of endophyte IZ 0792 resembled that of other Cyathicula strains (), and it produced potent antifungal and antibacterial activity that has not been fully characterized; however, the activities are not caused by pneumocandins. It appeared to be closest relative of Glarea found to date, although clearly it was not conspecific with G. lozoyensis, based on morphological, metabolic and molecular data.
We hypothesize that conidia dispersal and antifungal antibiosis may have evolved as adaptations in G. lozoyensis during a loss of sexual reproduction and a shift from specialized colonization of specific plant hosts to non-specialized colonization of plant litter and soil. Relatively simple modifications in septation patterns of aerial hyphae could give rise to the holoblastic meristematic conidia ( and ). Melanisation of these modified hyphal-derived propagules might have improved survivability. Holoblastic anamorphs with complex multicellular conidia; integrated conidiogenous cells, and poorly differentiated morphology occur sporadically among the Helotiales (Huhtinen Citation1989; Hosoya and Huhtinen Citation2002). Such morphological features are sometimes associated with aero-aquatic hyphomycetes where complex multicellular conidia aid in floatability and water dispersal. The teleomorphs of Cyathicula, Hymenoscyphyus and other helotialean discomycetes are often found in wet environments, and the natural selection for complex holoblastic conidia might be regarded as a convergent adaptation to the habitat rather than a phylogenetic coincidence.
Cyathicula appeared as a natural monophyletic group based on ITS-28S sequences, but the actin-based phylogeny failed to confirm this conclusion. This incongruence might be an artifact caused by the small size and heterogeneity of the sequence alignment. We have refrained here from using the generic name Crocicreas Fr. in favour of Cyathicula, in contrast to the current usage (Triebel and Baral Citation1996; Lumbsch and Huhndorf, Citation2007), because the phylogenetic placement of the morphologically deviating type species of Crocicreas is still unclear (Baral Citation1994; Iturriaga et al. Citation1999). The taxonomic position of P. amenti (Batsch) Dennis (Basionym: Peziza amenti Batsch) has been controversial. The morphology of P. amenti concurs in the ascus apical apparatus and paraphysis shape with the type species of Cyathicula, Cy. coronata. Carpenter (Citation1981) made the combination Cr. amenti (Batsch) S.E. Carp., an opinion supported by the present study, and is valid if the type species of Crocicreas is included in the concept of Cyathicula, over which Crocicreas has priority. The synonymy of Crocicreas with Cyathicula was questioned by Baral (Citation1994), because it was mainly based on a single character, the gelatinized excipulum, while other characters (shape of ascus apical rings and paraphyses) differed. Whether a new combination in Cyathicula is warranted must await a comprehensive phylogenetic reevaluation of the genera Cyathicula and Crocicreas, including their respective type species, Cy. coronata and Cr. gramineum, to determine whether Crocicreas is inclusive of Cyathicula or not.
Conclusions
Fungal isolation by extinction culturing has extended the known distribution of the pneumocandin-producing G. lozoyensis from the type location in Europe to North and South America. A DNA barcode database rapidly correlated rDNA sequence data with bioactivity and LC–MS database matching to identify the new strains. Further collection and phylogenetic analysis of fungi in the Helotiales indicated that G. lozoyensis shares a common ancestor with Cyathicula species. Possibly, the shift from a plant host-specific life-cycle strategy to that of a saprobic life-cycle in competitive soil and plant litter environments deemphasized the sexual cycle, while selecting for development of holoblastic asexual propagules on differentiated aerial hyphae and acquisition of genes for biosynthesis of potent fungal cell wall inhibiting secondary metabolites.
Acknowledgments
The authors express their appreciation to the editors for the invitation to submit this article for this special edition of Mycology. We are indebted to the staff of the former Centro de Investigación Básica of Merck, Sharp and Dohme of Spain for their expert technical support. Jon Polishook provided materials from the USDA White Mountain National Forest, New Hampshire. Consulting and Professional Diving, Madrid, Spain provided materials from Argentina.
References
- Baral , HO . 1989 . Beiträge zur Taxonomie der Discomyceten II. Die Calycellina-Arten mit 4sporigen Asci . Beiträge zur Kenntnis der Pilze Mitteleuropas , 5 : 209 – 236 .
- Baral , HO . 1992 . Vital versus herbarium taxonomy: morphological differences between living and dead cells of Ascomycetes, and their taxonomic implications . Mycotaxon , 44 : 333 – 390 .
- Baral , HO . 1994 . Comments on “Outline of the ascomycetes - 1993” . Systema Ascomycetum , 13 : 113 – 128 .
- Baral , HO . 2002 . Tapesina griseovitellina, a rarely reported discomycete, and its anamorph Chalara rubi . Z Mykol. , 68 : 117 – 134 .
- Benz , F , Knüsel , F , Nüesc , J , Treichler , H , Voser , W , Nyfeler , R and Keller-Schierlein , W . 1974 . Stoffwechselprodukte von Mikroorganismen. Echinocandin B, ein neuartiges Polypetid-Antibioticum aus Aspergillus nidulans var. echinulatus: Isolierung und Bausteine . Helv Chim Acta , 57 : 2459 – 2477 .
- Bills , GF , Platas , G , Peláez , F and Masurekar , P . 1999 . Reclassification of a pneumocandin-producing anamorph, Glarea lozoyensis gen. et sp. nov., previously identified as Zalerion arboricola . Mycol Res. , 103 : 179 – 192 .
- Bills , GF , Platas , G , Fillola , A , Jiménez , R , Collado , J , Vicente , MF , Martín , J , del , González , Val , A , Bur-Zimmerman , J , Tormo , JR and Peláez , F . 2008 . Enhancement of antibiotic and secondary metabolite detection from filamentous fungi by growth on nutritional arrays . J Appl Microbiol. , 104 : 1644 – 1658 .
- Bills , GF , Martín , J , Collado , J , Platas , G , Overy , D , Tormo , JR , Vicente , F , Verkley , G and Crous , PW . 2009 . Measuring the distribution and diversity of antibiosis and secondary metabolites in the filamentous fungi . Soc Ind Microbiol News , 59 : 133 – 146 .
- Cai , L , Wu , W-P and Hyde , KD . 2009 . Phylogenetic relationships of Chalara and allied species inferred from ribosomal DNA sequences . Mycol Prog. , 8 : 133 – 143 .
- Campbell , J , Volkmann-Kohlmeyer , B , Grafenhan , T , Spatafora , JW and Kohlmeyer , J . 2005 . A re-evaluation of Lulworthiales: relationships based on 18S and 28S rDNA . Mycol Res. , 109 : 556 – 568 .
- Carbone , I and Kohn , LM . 1999 . A method for designing primer sets for speciation studies in filamentous Ascomycetes . Mycologia , 91 : 553 – 556 .
- Carpenter , SE . 1981 . Monograph of Crocicreas (Ascomycetes, Helotiales, Leotiaceae) . Mem New York Bot Garden , 33 : 1 – 290 .
- Collado , J , Platas , G , Paulus , B and Bills , GF . 2007 . High-throughput culturing of fungi from plant litter by a dilution-to-extinction technique . FEMS Microbiol Ecol. , 60 : 521 – 533 .
- Dixon , JR . 1974 . Chlorosplenium and its segregates. I. Introduction and the genus Chlorosplenium . Mycotaxon , 1 : 65 – 104 .
- Dreyfuss , MM . 1986 . Neue Erkenntnisse aus einem pharmakologischen Pilz-Screening . Sydowia , 39 : 22 – 36 .
- Gams , W and Philippi , S . 1992 . A study of Cyathicula strobilina and its Chalara anamorph in vitro . Persoonia , 14 : 547 – 552 .
- Hennequin , S , Ebihara , A , Ito , M , Iwatsuki , K and Dubuisson , JY . 2003 . Molecular systematics of the fern genus Hymenophyllum s.l. (Hymenophyllaceae) based on chloroplastic coding and noncoding regions . Mol Phylogen Evol. , 27 : 283 – 301 .
- Hino , M , Fujie , A , Iwamoto , T , Hori , Y , Hashimoto , M , Tsurumi , Y , Sakamoto , K , Takase , S and Hashimoto , S . 2001 . Chemical diversity in lipopeptide antifungal antibiotics . J Ind Microbiol Biotechnol. , 27 : 157 – 162 .
- Hosoya , T and Huhtinen , S . 2002 . Hyaloscyphaceae in Japan (7): Hyaloscypha albohyalina var. monodictys var. nov . Mycoscience , 43 : 405 – 409 .
- Huhtinen , S . 1989 . A monograph of Hyaloscypha and allied genera . Karstenia , 29 : 45 – 252 .
- Iturriaga , T , Korf , RP and Babcock , JF . 1999 . Fungi on Epifagus Crocicreas epifagicola sp. nov., with comments on the generic names Crocicreas and Cyathicula . Mycol Res. , 103 : 28 – 30 .
- Iwamoto , T , Fujie , A , Sakamoto , K , Tsurumi , T , Shigematsu , N , Yamashita , M , Hashimoto , S , Okuhara , M and Kohsaka , M . 1994 . WF11899A, WF11899B and WF11899C, novel antifungal lipopeptides. 1. Taxonomy, fermentation, isolation and physicochemical properties . J Antibiot. , 47 : 1084 – 1091 .
- Joseph , JM , Kim , R and Reboli , AC . 2008 . Anidulafungin: a drug evaluation of a new echinocandin . Expert Opin Pharmacother. , 9 : 2339 – 2348 .
- Kanasaki , R , Sakamoto , K , Hashimoto , M , Takase , S , Tsurumi , Y , Fujie , A , Hino , M , Hashimoto , S and Hori , Y . 2006a . FR209602 and related compounds, novel antifungal lipopeptides from Coleophoma crateriformis no.738 - I. Taxonomy, fermentation, isolation and physico-chemical properties . J Antibiot. , 59 : 137 – 144 .
- Kanasaki , R , Abe , F , Kobayashi , M , Katsuoka , M , Hashimoto , M , Takase , S , Tsurumi , Y , Fujie , A , Hino , M , Hashimoto , S and Hori , Y . 2006b . FR220897 and FR22089, novel antifungal lipopeptides from Coelophoma empetri No. 14573 . J Antibiot. , 59 : 149 – 157 .
- Kanasaki , R , Kobayashi , M , Fujine , K , Sato , I , Hashimoto , M , Takase , S , Tsurumi , Y , Fujie , A , Hino , M , Hashimoto , S and Hori , Y . 2006c . FR227673 and FR190293, novel antifungal lipopeptides from Chalara sp. No. 22210 and Tolypocladium parasiticum No. 16616 . J Antibiot. , 59 : 158 – 167 .
- Keller-Juslén , C , Kuhn , M , Loosli , HR , Petcher , TJ , Weber , HP and von Wartburg , A . 1976 . Struktur des cyclopeptide –antibiotikums SL 7810 (=Echinochandin B) . Tetrahedron Lett. , 46 : 4147 – 4150 .
- Kowalski , S and Holdenrieder , O . 2009 . The teleomorph of Chalara fraxinea, the causal agent of ash dieback . For Pathol. , 39 : 1 – 7 .
- Larena , I , Salazar , O , González , V , Julián , MC and Rubio , V . 1999 . Design of a primer for ribosomal DNA internal transcribed spacer with enhanced specificity for ascomycetes . J Biotechnol. , 75 : 187 – 194 .
- Lumbsch TH, Huhndorf SM. 2007. Outline of Ascomycota. Myconet 13: 1–58. Available from http://www.fieldmuseum.org/myconet/outline.asp (http://www.fieldmuseum.org/myconet/outline.asp)
- McCormack , PL and Perry , CM . 2005 . Caspofungin. A review of its use in the treatment of fungal infections . Drugs , 65 : 2049 – 2068 .
- Mizuno , K , Yagi , A , Satoi , S , Takada , M , Hayashi , M , Asano , K and Matsuda , T . 1977 . Studies on aculeacin. I. Isolation and characterization of aculeacin A . J Antibiot. , 30 : 297 – 302 .
- Morrison , V . 2006 . Echinocandin antifungals: review and update . Expert Rev Anti-infect Ther. , 4 : 325 – 342 .
- Mukhopadhyay , T , Roy , K , Bhat , RG , Sawant , SN , Blumbach , J and Ganguli , BN . 1992 . Deoxymulundocandin–a new echinocandin type antifungal antibiotic . J Antibiot. , 45 : 618 – 623 .
- Nakagiri , A . 1984 . Two new species of Lulworthia and evaluation of genera-delimiting characters between Lulworthia and Lindra (Halosphaeriaceae) . Trans Mycol Soc Jpn. , 25 : 377 – 388 .
- Noble , HM , Langley , D , Sidebottom , PJ , Lane , SJ and Fisher , PJ . 1991 . An echinocandin from an endophytic Cryptosporiopsis and Pezicula sp. in Pinus sylvestris and Fagus sylvatica . Mycol Res. , 95 : 1439 – 1440 .
- Nylander , JAA . 2004 . MrModeltest v2. Program distributed by the author , Uppsala University, , Sweden : Evolutionary Biology Centre .
- O'Donnell , K . 1993 . “ Fusarium and its near relatives ” . In The fungal holomorph: mitotic, meiotic and pleomorphic speciation in fungal systematic , Edited by: Reynolds , DR and Taylor , JW . 225 – 233 . Wallingford, UK : CAB International. p .
- Peláez , F , Platas , G , Collado , J and Díez , MT . 1996 . Infraspecific variation in two species of aquatic hyphomycetes, assessed by RAPD analysis . Mycol Res. , 100 : 831 – 837 .
- Petersen , LA , Hughes , DL , Hughes , R , Di Michele , L , Salmon , P and Connors , N . 2001 . Effects of amino acid and trace element supplementation on pneumocandin production by Glarea lozoyensis: impact on titer, analogue levels, and the identification of new analogues of pneumocandin B0 . J Ind Microbiol Biotechnol. , 26 : 216 – 221 .
- Ronquist , F and Huelsenbeck , JP . 2003 . MrBayes 3: Bayesian phylogenetic inference under mixed models . Bioinformatics , 19 : 1572 – 1574 .
- Ronquist , F , van der Mark , P and Huelsenbeck , JP . 2009 . “ Chapter 7. Bayesian phylogenetic analysis using MrBayes ” . In editors. The phylogenetic handbook: A practical approach to phylogenetic analysis and hypothesis testing, 2nd , Edited by: Lemey , P , Salemi , M and Vandamme , AM . 210 – 266 . Cambridge, UK. p : Cambridge University Press .
- Roy , K , Mukhopadhyay , T , Reddy , GCS , Desikan , KR and Ganguli , BN . 1987 . Mulundocandin, a new lipopeptide antibiotic. I. Taxonomy, fermentation, isolation and characterization . J Antibiot. , 40 : 275 – 280 .
- Sánchez Márquez , S , Bills , GF , Domínguez , A and Zabalgogeazcoa , I . 2010 . Endophytic mycobiota of leaves and roots of the grass Holcus lanatus . Fung Divers. , 41 : 115 – 123 .
- Satoi , S , Yagi , A , Asano , K , Mizuno , K and Watanabe , T . 1977 . Studies on aculeacin. II. Isolation and characterization of aculeacins B, C, D, E, F and G . J Antibiot. , 30 : 303 – 307 .
- Schwartz , RE , Giacobbe , RA , Bland , JA and Monaghan , RL . 1989 . L-671,329, a new antifungal agent. I. Fermentation and isolation . J Antibiot. , 42 : 163 – 167 .
- Schwartz , RE , Sesin , DF , Joshua , H , Wilson , KE , Kempf , AJ , Goklen , KA , Kuehner , D , Gailliot , P , Gleason , C , White , R , Inamine , E , Bills , G , Samon , P and Zitano , L . 1992 . Pneumocandins from Zalerion arboricola. I. Discovery and isolation . J Antibiot. , 45 : 1853 – 1866 .
- Strobel , GA , Miller , RV , Martinez-Miller , C , Condron , MM , Teplow , DB and Hess , WM . 1999 . Cryptocandin, a potent antimycotic from the endophytic fungus Cryptosporiopsis cf. quercina . Microbiology , 145 : 1919 – 1926 .
- Traber , R , Keller-Juslén , C , Loosli , H-R , Kuhn , M and von Wartburg , A . 1979 . Cyclopeptid-Antibiotika aus Aspergillus-Arten . Struktur der Echinocandin C und D. Helv Chim Acta , 62 : 1252 – 1267 .
- Triebel , D and Baral , HO . 1996 . Notes on the ascus types in Crocicreas (Leotiales) with a characterization of selected taxa . Sendtnera , 3 : 199 – 218 .
- Vicente , F , Basilio , A , Platas , G , Collado , J , Bills , GF , del , González , Val , A , Martín , J , Tormo , JR , Harris , GH , Zink , DL , Justice , M , Nielsen Kahn , J and Peláez , F . 2009 . Distribution of the antifungal agents sordarins across filamentous fungi . Mycol Res. , 113 : 754 – 770 .
- White , TJ , Bruns , T , Lee , S and J- , Taylor . 1990 . “ Amplification and direct sequencing of fungal ribosomal RNA genes for phylogenetics ” . In PCR Protocols: A Guide to Methods and Amplifications , Edited by: Innis , MA , Gelfand , DH , Sninsky , JJ and White , TJ . 315 – 322 . New York : Academic Press .
- Wiederhold , NP and Lewis , JS . 2007 . The echinocandin micafungin: a review of the pharmacology, spectrum of activity, clinical efficacy and safety . Expert Opin Pharmacother. , 8 : 1155 – 1166 .
- Young , ND and Healy , J . 2003 . GapCode automates the use of indel characters in phylogenetic analysis . BMC Bioinformatics , 4 : 6