Abstract
Random insertional mutagenesis of Colletotrichum higginsianum using Agrobacterium tumefaciens-mediated transformation was utilised to tag genes required for fungal pathogenicity. Two of the mutants, path-19 and path-35, were arginine auxotrophs and produced no symptoms on susceptible Arabidopsis thaliana plants. The T-DNA tagged genes showed high homology to the polyprotein arg-6 precursor and carbamoyl-phosphate synthetase, respectively, both of which are involved in arginine biosynthesis. In both mutants, conidial germination and appressorium formation were similar to the wild-type strain, but appressorial penetration and subsequent invasive growth in planta were severely reduced. Complementation of the T-DNA tagged mutants with cosmid genomic clones containing wild-type copies of the respective genes fully restored pathogenicity and the ability to grow on minimal media. These results suggest that arginine biosynthesis is critical for the early stages of plant infection by C. higginsianum.
Introduction
For pathogens, the ability to sense nutrition and adjust metabolism accordingly is crucial to successfully infect hosts. In many plant pathogenic fungi, tight regulation of the pre- and post-penetration process at the right time and in the right place is critical for successful completion of their life cycle. Particularly, fungal pathogens including obligate biotrophs and hemibiotrophs, which have an initial biotrophic stage of infection, undergo a series of morphological changes to develop feeding structures inside the host. This precise morphogenetic programme is accomplished through changes in fungal gene expression that are regulated by the perception of environmental conditions (Pellier et al. Citation2003). Patterns of fungal gene expression upon host infection have been analysed in several obligate biotrophs and hemibiotrophs (Hahn and Mendgen Citation1997; Both et al. Citation2005; Jakupovic et al. 2005; Tang et al. Citation2006), leading to the identification of many genes expressed in planta and induced by different environmental conditions, such as several nutrient starvations (Divon and Fluhr Citation2007; Mathioni et al. Citation2011).
Mutants deficient in amino acid biosynthesis, so called auxotrophs, have been reported in several plant pathogenic fungi. The pth3 and met1 mutants showing histidine and methionine auxotrophy, respectively, were isolated from Magnaporthe grisea following random insertional mutagenesis (Sweigard et al. Citation1998). Other amino acid auxotrophic mutants were identified in mutant screens of Ustilago maydis (Holliday Citation1961), Fusarium oxysporum (Namiki et al. Citation2001), Stagonospora nodorum (Bailey et al. Citation2000) and Cladosporium fulvum (Solomon et al. Citation2000). A link between auxotrophy and a loss, or reduction, in pathogenicity was found in most cases. This indicates that the ability to synthesise certain amino acids is important for development in planta, when such nutrients may be present at limiting concentrations.
The ascomycete, Colletotrichum higginsianum Sacc. (1917), belongs to one of the most economically important groups of pathogens, causing anthracnose disease on a wide range of cruciferous plants, such as Brassica, Raphanus, and the model plant Arabidopsis thaliana (Narusaka et al. Citation2004; O'Connell et al. Citation2004). To invade host tissue, this fungus sequentially develops a series of specialized infection structures. The infection begins with the adhesion of conidia to the plant surface; the conidia then germinate to form darkly pigmented, dome-shaped appressoria that pierce the plant cuticle and cell wall directly and mediate entry into host epidermal cells. After successful penetration, bulbous primary hyphae grow biotrophically inside living host epidermal cells and invaginate the host plasma membrane. This haustorium-like structure is entirely restricted to the first infected epidermal cell, and seems to obtain nutrients and water from the plant apoplast. Subsequently, abundant nutrients are probably released during the destructive necrotrophic stage of host infection through the activity of secreted degradative enzymes. At this stage, the pathogen also undergoes a morphological switch, producing narrow secondary hyphae, which rapidly colonise the entire host tissue. Based on this two-stage infection strategy, C. higginsianum is classified as a hemibiotroph. Molecular analysis of the mechanism of compatibility between plants and biotrophs is difficult because the genetic manipulation of biotrophs is generally impossible (Voegele et al. Citation2006; Djulic et al. Citation2011). However, the study of hemibiotrophic pathogens like C. higginsianum, which can be readily cultured in vitro and stably transformed, can facilitate the molecular analysis of biotrophy (O'Connell et al. Citation2004; O'Connell and Panstruga Citation2006).
The C. higginsianum-A thaliana pathosystem provides an experimental model in which both partners can be genetically manipulated and genome sequences are available for both (Narusaka et al. Citation2004; O'Connell et al. Citation2004). Analysis of this pathosytem has facilitated identification of two loci conferring anthracnose resistance in A. thaliana (Narusaka et al. 2009; Birker et al. Citation2009). On the fungal side, important genomic resources include several cDNA libraries generated from specialized infection structures (Kleemann et al. Citation2008; Takahara et al. Citation2009). These analyses have enabled the identification of several candidate pathogenicity genes for future functional analysis, and deep sequencing of the fungal transcriptome in planta using next-generation sequencing technologies is on-going (O'Connell, unpublished data). In addition, a forward genetic screen was performed to discover pathogenicity genes in C. higginsianum (Huser et al. Citation2009). A total of 8850 transformants were generated by Agrobacterium tumefaciens-mediated transformation (AtMT), among which 40 independent mutants were significantly reduced in their pathogenicity or non-pathogenic. Those pathogenicity mutants were classified according to their morphological or infection phenotypes as melanin-deficient, penetration-deficient, inducers of plant defence responses or impaired in the switch from biotrophy to necrotrophy. In this article, we report on the characterization of two completely non-pathogenic mutants, designated as path-19 and path-35, which were shown to be arginine auxotrophs. The T-DNA tagged genes in these mutants encoded enzymes involved in the arginine biosynthesis pathway, namely carbamoyl-phosphate synthetase and a polyprotein precursor of N-acetylglutamate kinase and N-acetylglutamyl-phosphate reductase. Complementation of the mutants with cosmid genomic clones harbouring wild-type copies of these genes fully restored pathogenicity on Arabidopsis and the ability to grow on minimal media. These results indicate that arginine biosynthesis is critical for fungal pathogenicity in C. higginsianum on the host plant A. thaliana.
Materials and Methods
Fungal and plant material
Colletotrichum higginsianum strain IMI349061 was maintained on Mathur's agar medium at 25 °C (O'Connell et al. Citation2004). T-DNA insertional mutants previously generated by Agrobacterium tumefaciens-mediated transformation (AtMT; Huser et al. Citation2009) were maintained on potato dextrose agar (PDA; Difco, Becton, Dickinson and Company, Heidelberg, Germany) supplemented with 50 μg ml−1 hygromycin B (Sigma-Aldrich, St. Louis, MO, USA). Conidia were harvested from 10–12-day-old cultures and stored as glycerol stocks at −80 °C. Plants of Arabidopsis thaliana Landsberg (Ler-0) were grown in peat-based compost in a controlled environment (10-h photoperiod, 180 μmol m−2 s−1, 22 °C, 65% humidity). For infection assays, conidial suspension was spray-inoculated onto either 3–4-week-old plants (5 × 105 conidia per ml) or 10-day-old seedlings (5 × 106 conidia per ml) using an atomiser. Inoculated plants were then placed in sealed propagator boxes to maintain 100% humidity and incubated in a controlled environment (16 h photoperiod, 80 μmol m−2 s−1, 25 °C). For light microscopy, infected leaf tissues were stained with lactophenol-Trypan blue and cleared in chloral hydrate solution (Takahara et al. Citation2009).
Phenotypic and molecular characterization of mutants
Radial growth rate was measured on PDA and Czapek-dox agar (CDA; Difco) as described previously (Huser et al. Citation2009), or on CDA supplemented with 100 μg ml−1 l-glutamate, l-ornithine, l-citrulline or l-arginine (Sigma-Aldrich). To assess the development of infection structures in vitro, droplets of conidial suspension (5 × 105 conidia per ml) were placed onto polystyrene dishes or pieces of sterile cellophane membrane (Visking dialysis membrane, Roth) with or without l-arginine supplementation (100 μg ml−1), and maintained at 25 °C. Most molecular manipulations were as described by Huser et al. (Citation2009). Fungal genomic DNA was extracted from mycelia grown on PDA for 4 days at 25 °C and Southern blot hybridization was performed to determine T-DNA copy number using part of the T-DNA sequence as a probe. Genomic sequences flanking the T-DNA insertion were obtained by inverse PCR. Those sequences were used to screen a cosmid genomic library prepared from C. higginsianum strain IMI349061 to isolate clones containing a wild-type copy of the T-DNA-tagged locus (Huser et al. Citation2009). Further sequence was obtained from the cosmid clones by primer walking using gene-specific primers. Identified genomic regions were verified using the C. higginsianum genome database available from the Max Planck Institute for Plant Breeding Research (http://www.mpiz-koeln.mpg.de/english/research/pmi-dpt/Fungal_genomes/index.html). The open reading frames (ORFs) were predicted by the Conrad gene predictor program based on semi-Markov conditional random fields (SMCRFs; DeCaprio et al. 2007) and the FGENESH prediction tool using Fusarium and Magnaporthe matrices (Softberry Inc., Mount Kisco, NY, USA). Sequence homology searches were performed against the NCBI nr protein data base using appropriate BLAST algorithms. Functional motifs were predicted by querying protein sequences against NCBI conserved domain data base using RPS BLAST and InterProScan. Putative subcellular localization, mitochondrial target sequences and propeptide cleavage sites were predicted by TargetP1.1 MitoProtII, and ProP 1.0 server prediction tools on the respective web sites.
Functional complementation of mutants
To verify that the T-DNA integration events detected in mutants path-19 and path-35 were responsible for the observed auxotrophy and pathogenicity phenotypes, Agrobacterium tumefaciens-mediated transformation (AtMT) was used to complement the mutants with cosmid clones harbouring wild-type copies of the corresponding genes. The binary vector used for constructing the cosmid genomic library, pBIG5CosB1, carries the bialaphos resistance gene cassette between the T-DNA left and right borders. The cosmid clones designated pPath-19cos1 and pPath-35cos1 (containing the respective wild-type genomic regions) were first transformed into A. tumefaciens C58C1 and then integrated into corresponding mutants background, independently. The protocol used for AtMT was slightly modified from that of Tsuji et al. (Citation2003), Takahara et al. (Citation2004) and Huser et al. (Citation2009). Transformants were selected on CDA supplemented with 50 μg ml−1 cefotaxim (Sigma-Aldrich), 50 μg ml−1 spectinomycin (Sigma-Aldrich) and 5 μg ml−1 bialaphos (Wako Pure Chemical, Tokyo, Japan). The complemented mutants were then tested for growth on CDA minimal medium and pathogenicity on 4-week-old Arabidopsis plants as described above.
Reverse transcription-polymerase chain reaction (RT-PCR)
RT-PCR was performed using total RNA extracted from (a) saprophytic mycelia grown for 4 days on PDA or CDA or (b) infected Arabidopsis seedlings at different times after inoculation (24, 48 and 72 h), corresponding to the appressorial penetration, biotrophic and necrotrophic stages of infection, respectively. RNA was extracted using TRIzol reagent (Invitrogen, Mannheim, Germany), purified with the RNeasy plant mini kit (Qiagen, Mannheim, Germany) and treated with RNase-free DNase I (Qiagen). Integrity of those RNAs was assessed by agarose gel electrophoresis, and 1 μg of total RNA was reverse-transcribed to cDNA using oligo-dT(18) primer and Super script II reverse transcriptase (Invitrogen). The PCR reaction mixture and conditions were as described previously (Kleemann et al. Citation2008) using the following gene-specific primer pairs: arg6 precursor-Forward 5′-CGT CGG CGT TGA CGT TG-3′; arg6 precursor-Reverse 5′-CTC GAG GGC CTT GGC G-3′; carbamoyl-phosphate synthetase-Forward 5′-TCA AGC CTT CTG CCA GCG-3′; carbamoyl-phosphate synthetase-Reverse 5′- CTC GAG AGT CTT GAC GCT AG-3′. The C. higginsianum beta tubulin gene was used as internal control gene (Takahara et al. Citation2009). Thermal cycling conditions were 95 °C for 2 min, and cycles of 94 °C for 30 s, 55 °C for 30 s, 72 °C for 30 s and final extension at 72 °C for 5 min. The cycle number was adjusted with reference to the beta-tubulin gene: 24 cycles for saprophytic mycelia, 34, 31 and 27 cycles for 24, 48 and 72 h after inoculation, respectively.
Results
AtMT of C. higginsianum and pathogenicity mutants
To discover pathogenicity genes in the crucifer anthracnose fungus, Colletotrichum higginsianum, a total of 8850 transformants were generated by Agrobacterium tumefaciens-mediated transformation and 40 mutants (path-1 to path-40) were found to be non-pathogenic or significantly reduced in their pathogenicity on Arabidopsis plants (Huser et al. Citation2009). Here, we report the characterization of two of those mutants, designated as path-19 and path-35.
Two mutants path-19 and path-35 showed a similar pathogenicity phenotype, producing no visible symptoms on A. thaliana plants at 6 days post inoculation (dpi) (), in contrast to the wild type strain which caused complete maceration of the host tissue. Microscopic inspection of the inoculated leaf tissue showed that pre-penetration development of mutants path-19 and path-35 resembled that of the wild-type, producing normal melanised appressoria containing basal penetration pores (). However, while appressoria of the wild-type had already penetrated into host epidermal cells to form biotrophic primary hyphae at 2 dpi, those of the mutants had not formed visible primary hyphae even at 6 dpi. Induction of host defence responses was analysed and it was shown previously that path-35 induced deposition of callose papillae by host cells at sites of attempted penetration more frequently than the wild-type (Huser et al. Citation2009), but papilla formation was not observed beneath appressoria of path-19. In addition, Trypan blue staining showed that some of appressoria of path-35 induced the death of some (∼15%) underlying epidermal cells, resembling a single-cell hypersensitive response.
Figure 1. (A) Pathogenicity assays of Colletotrichum higginsianum mutants. Three-week-old plants of susceptible Arabidopsis thaliana accession Ler-0 were spray-inoculated with spore suspension of C. higginsianum wild-type strain IMI349061 and two pathogenicity mutants (path-19 and path-35). Symptoms were assessed at 6 days post-inoculation (dpi) (top row) and stained with lactophenol-Trypan blue for light microscopy (bottom row). The wild-type strain caused massive necrosis, but the two pathogenicity mutants produced almost no symptoms. In the wild-type, biotrophic primary hypha (PH) developed beneath appresoria (AP) at 2 dpi. PH were strongly stained by Trypan blue. However, two mutants failed to penetrate even after 6 dpi. Death of single epidermal cells, resembling a hypersensitive response, was observed beneath appressoria of path-35. Scale bar corresponds to 10 μm. (B) Verification of the T-DNA tagged locus by complementation with a wild-type gene copy. Three-week-old plants of A. thaliana accession Ler-0 were spray-inoculated with spore suspensions of C. higginsianum wild-type strain and two complemented mutants derived from the path-19 and path-35 mutant backgrounds, respectively. Symptoms were assessed 6 dpi (top row). Vegetative growth in vitro was analysed on minimal medium (Czapek-dox agar) at 25 °C after 4 days (bottom row).
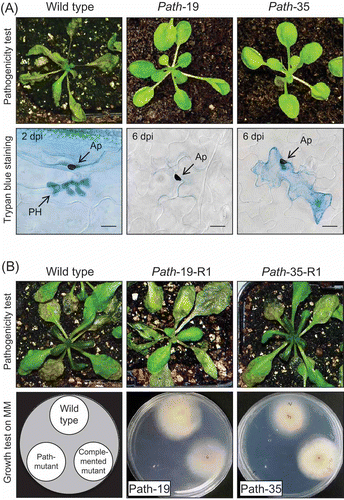
T-DNA tagged locus of mutants
Southern blot hybridization analysis was performed to identify the number of T-DNA insertion sites in each mutant genome. Genomic DNAs were digested with BglII (no cut site within the vector), XbaI (which gives 3.8-kb fragments after digestion), or XhoI (to determine the T-DNA orientation for tandem repeat integrations) and hybridized with a probe prepared from a T-DNA-specific sequence ( and (B)). The mutant path-19 harboured a tandem T-DNA integration with head-to-tail orientation located at a single site in the genome, while the mutant path-35 contained perhaps three T-DNA copies inserted at a single site in the genome ( and (B)).
Figure 2. (A) Southern blot hybridization of the pathogenicity mutants. Genomic DNAs were digested with three different restriction enzymes (BglII: Bg, XbaI: Xb, XhoI: Xh) and hybridized with DIG-labelled T-DNA specific probe (Huser et al. Citation2009). (B) Physical maps of the T-DNA integration events in the mutants path-19 and path-35. Positions of XbaI (Xb) and XhoI (Xh) restriction sites and a probe (box with stripe) are indicated. (C) The cosmid clones carrying genes corresponding to both tagged genes, respectively. T-DNA integration site is shown by an arrow head. Genomic regions adjoining the two genes were obtained from sequence flanking the T-DNA integration site. (D) The linear arginine biosynthesis pathway of microorganisms; modified from Davis (Citation1986) and Caldovic and Tuchman (Citation2003).
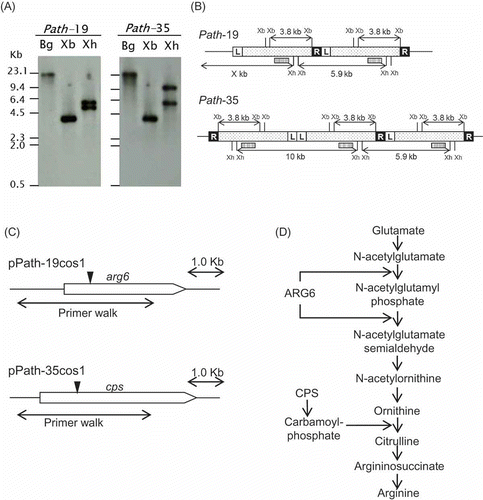
Sequence flanking the T-DNA-tagged locus of both mutants was obtained by inverse PCR. A BLAST database search detected homologous sequences encoding an Arg-6 precursor and a carbamoyl-phosphate synthetase. The T-DNAs were integrated near the N-termini of the respective ORFs (). A cosmid genomic library of C. higginsianum was screened with those flanking sequences, and cosmid clones containing the respective genes, designated as pPath-19cos1 and pPath-35cos1, were obtained independently (). Further genomic regions were directly sequenced from the respective cosmid clones by primer walking using gene-specific primers. Identified sequences were submitted to the EMBL database (ID FM201305 and FM201306; Huser et al. Citation2009). It was possible to identify and confirm additional flanking sequence information for both genes using the draft C. higginsianum genome sequence project currently available online from the Max Planck Institute for Plant Breeding Research. The predicted ORF of the tagged gene in mutant path-19 showed high homology to the Arg-6 precursor of Neurospora crassa (EMBL accession EAA35492, E = 0.0), while the tagged gene in mutant path-35 was highly homologous to the large subunit of arginine-specific carbamoyl-phosphate synthetase (CPS) of N. crassa (EAA36214, E = 0.0). Thus, remarkably, both tagged genes in two independent mutants were found to be involved in the biosynthesis of arginine ().
Gene structure of the mutated genes
The putative C. higginsianum Arg-6 precursor (ChArg6) encodes a proprotein of 891 amino acids with a single translation product containing two enzyme domains – ArgB, N-acetylglutamate kinase (NAGK) and ArgC, N-acetylglutamyl phosphate reductase (NAGPR), respectively (Boonchird et al. Citation1991; Parra-Gessert et al. Citation1998; Figure S1). This bifunctional proprotein may act in the sequential conversion of N-acetylglutamate to N-acetylglutamate semialdehyde, corresponding to the second and third steps of the linear arginine biosynthesis pathway (). The putative localization of ChARG6 was predicted to be mitochondria (probability 0.952 by TargetP1.1) and a mitochondrial targeting sequence located at the N-terminal 47 amino acids of the protein was predicted by MitoProtII (probability 0.9999). Although the probability was relatively low (0.616 by ProP), the propeptide cleavage site was predicted at amino acid 506 within the spacer sequence between domain NAGK and NAGPR, which contained the predicted consensus sequence “R-X-[R/K]-R” cleaved by furin-type (Arginine/Lysine residues) propeptide proteinases (Nakayama Citation1997; Duckert et al. Citation2004).
The putative carbamoyl-phosphate synthetase (ChCps) encodes a protein of 1161 amino acids including several conserved domains, including the N-terminal domain, two ATP-binding domains, oligomerisation domain and methylglyoxal synthase-like domain at the C-terminus (Figure S2). The CPS enzyme catalyzes the formation of carbamoyl phosphate which is, in turn, required for the conversion of l-ornithine to l-citrulline by ornithine carbamoyltransferase in the arginine biosynthesis pathway (Price at al. Citation1978; ). Mitochondrial localization was predicted (probability 0.905 by TargetP1.1), and a mitochondrial targeting sequence was identified at the N-terminal 65 amino acids (provability 0.9996 by MitoProtII).
Verification of the functional roles of tagged genes
To verify that the T-DNA integration events detected in mutant path-19 and path-35 were responsible for the observed arginine auxotrophy phenotype, AtMT was used to complement the mutants with cosmid clones harbouring wild-type copies of the corresponding genes. We obtained five and eight complemented transformants from path-19 and path-35 mutants, respectively. All tested transformants (five from each mutant) were fully restored in their pathogenicity on Arabidopsis plants, producing symptoms of similar severity as the wild-type at 6 dpi. Representative pictures were shown in . In addition, complementation restored the ability of the mutants to grow on synthetic minimal medium (Czapek-dox agar; CDA) to wild-type levels (). These results demonstrate that ChArg6 and ChCps are essential not only for arginine biosynthesis but also for pathogenicity on A. thaliana.
In vitro characterisation of arginine auxotroph mutants
To confirm that the mutated genes function for arginine biosynthesis in C. higginsianum, the wild-type strain and the two auxotrophic mutants were cultured on CDA supplemented with different intermediates of the arginine biosynthesis pathway (). The wild-type strain grew on CDA supplemented with l-glutamate, l-ornithine and l-citrulline. On the other hand, path-19 only grew on ornithine- and citrulline-supplemented medium, while path-35 only grew on citrulline-supplemented medium. Both mutants grew on CDA supplemented with l-arginine as well (data not shown). Therefore we concluded that path-19 and path-35 are both arginine auxotrophs.
Figure 3. (A) Vegetative growth assay of auxotrophic mutants. Requirement for amino acids was analysed after 4 days growth at 25 °C on minimal medium (Czapek-dox agar) supplemented with 100 μg per ml of l- glutamate, l-citrulline or l- ornithine, respectively. Wild-type strain (W), path-19 (19), path-35 (35). (B) Appressorial penetration and invasive growth on cellophane membrane. The spores of C. higginsianum wild-type and two auxotrophic mutants were placed onto cellophane dialysis membrane supplemented with 100 μg per ml of l-arginine, or without supplementation and incubated for 2 days. Arrow indicates invasive hyphae that have penetrated into the cellophane membrane. Scale bar corresponds to 10 μm.
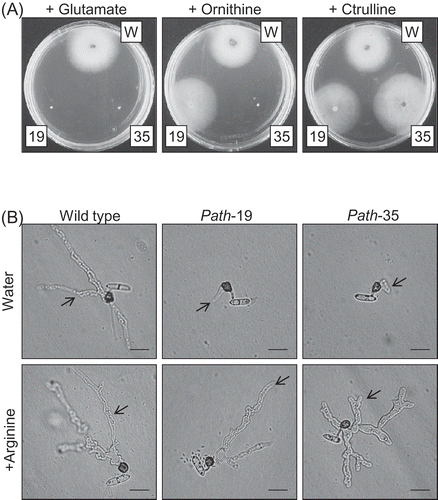
To assess whether spore germination or the morphogenesis of appressoria were affected in these mutants, conidial suspensions of path-19, path-35 and the wild-type were placed onto polystyrene dishes and incubated 24 h in a humid box. The two mutants germinated and formed melanised appressoria with similar efficiency as the wild-type: thus, appressorium formation was 91% in wild-type, 89% in path-19, and 91% in path-35, respectively. These frequencies were not changed when l-arginine was supplemented in the conidial suspensions (data not shown). On cellophane membranes, wild-type appressoria penetrated and produced extensive mycelia inside the membrane after 2 days. However, invasive hyphae developing from appressoria of the mutants were very small (, top panel). Invasive growth of the mutants on cellophane was also fully restored by supplementing conidial suspensions with l-arginine (). The mutants were indistinguishable from the wild-type in their level of sporulation, conidial size, and mycelial growth rate on rich nutrient media (data not shown).
Detection of transcripts in vitro and in planta
We used RT-PCR to profile the expression of the C. higginsianum Arg6 and Cps orthologues in the wild-type strain during mycelial growth in vitro and at different stages of host infection (). Transcripts of both genes were more abundant in mycelia grown on minimal medium (CDA) than on nitrogen-rich medium (PDA), suggesting that expression of ChArg6 and ChCps is subject to nitrogen catabolite repression. On Arabidopsis seedlings, ChArg6 appeared to be constitutively expressed at 24, 48 and 72 h after inoculation, which correspond to the appressorial penetration, biotrophic and necrotrophic stages of infection, respectively. In contrast, transcripts of ChCps were not detectable at the appressorial penetration stage, but expression was strongly up-regulated during the biotrophic stage and then down-regulated in the necrotrophic phase. Thus, although ChArg6 and ChCps are involved in the same biosynthetic pathway, they are differentially regulated during the fungal infection process in planta.
Figure 4. RT-PCR analysis of ChArg6 and ChCps transcripts. Total RNAs were extracted from C. higginsianum wild-type mycelia grown on rich medium (RM: Potato dextrose agar), minimal medium (MM: Czapek-dox agar), and infected Arabidopsis seedlings at 24, 48 and 72 h after inoculation, corresponding to the appressorial penetration, biotrophic and necrotrophic stages of infection, respectively. Five or two micrograms total RNA from mycelia and inoculated plant tissue were electrophoresed and stained with ethidium bromide to indicate RNA quality. C. higginsianum beta tubulin was used as a constitutively expressed control gene.
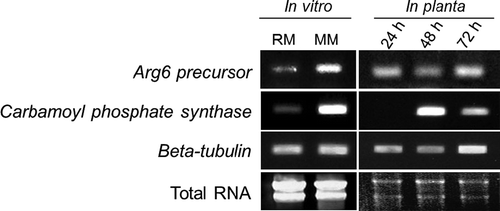
Discussion
In this study, we characterized in detail two C. higginsianum pathogenicity mutants that were previously generated by Agrobacterium-mediated random insertional mutagenesis (Huser et al. Citation2009). Interestingly, these mutants had T-DNA insertions within the predicted open reading frames of two genes encoding different enzymes in the same amino acid biosynthesis pathway. The tagged gene of mutant path-19 was highly homologous to Arg6 precursor and the tagged gene of path-35 showed high homology to the large subunit of arginine-specific carbamoyl-phosphate synthetase (CPS). Complementation with wild-type copies of the two tagged genes showed that all complementation mutants tested were fully restored in their pathogenicity on Arabidopsis plants. It is remarkable that out of only 12 C. higginsianum pathogenicity mutants previously characterized (Huser et al. Citation2009), two were affected in the same biochemical pathway, suggesting that arginine biosynthesis is critical for host infection by C. higginsianum. In addition, two other pathogenicity mutants showing auxotroph phenotypes were obtained by Huser and co-workers (2009) but not further characterised. This suggests that other aspects of nutrition may be required for full virulence of C. higginsianum.
Several studies provide evidence that nutritional requirements, such as amino acid biosynthesis or nutrient availabilities, are important for fungal infection cycles and pathogenicity. In Magnaporthe grisea, for example, pth3 and met1 mutants generated by insertional mutagenesis and showed reduced pathogenicity and requirement for histidine and methionine, respectively (Sweigard et al, Citation1998; Balhadere et al. Citation1999). In Cladosporium fulvum, mutation of the methionine biosynthesis gene (methionine synthase) also affected pathogenicity (Solomon et al. Citation2000). Namiki and associates (2001) reported that mutation of Arg1, which encodes argininosuccinate lyase catalysing the last step of the arginine biosynthesis pathway, reduced pathogenicity in Fusarium oxysporum f.sp. melonis, a causal agent of vascular wilt of melon. Although not many genes related to amino acid biosynthesis have been genetically analysed in plant pathogenic fungi so far (Divion and Fluhr 2007), it is notable that those amino acids were present at limiting concentrations in planta (Solomon and Oliver 2000), indicating that the ability to synthesise minor amino acids that are not abundant in planta may be essential for infection by several pathogenic fungi (Horst et al. Citation2010). In this study, we found that arginine biosynthesis was dispensable for conidial germination and appressorial morphogenesis of C. higginsianum, suggesting that arginine reserves in conidia are sufficient for the completion of pre-penetration development. However, arginine biosynthesis was critical for initial host penetration by appressoria and early biotrophic growth inside living host cells. In both mutants, penetration and invasive growth ability on cellophane membranes were restored by supplementation with arginine, indicating that C. higginsianum has an uptake mechanism to utilise amino acids from the environment. Although the auxotrophic mutants possess this uptake mechanism, they appear unable to obtain sufficient amino acids during the early stages of host infection, suggesting that the concentration of minor amino acids, such as arginine, available in the apoplast of A. thaliana could be limiting.
Genes related to nutrient uptake were identified from many plant pathogenic fungi, including amino acid transporters in Uromyces fabae (Hahn et al. Citation1997; Struck et al. Citation2002, Citation2004), peptide transporters in F. oxysporum (Divon et al. Citation2005) and Stagonospora nodorum (Solomon et al. Citation2004), and several sugar transporters in Blumeria graminis (Zhang et al. Citation2005), U. fabae (Voegle et al. 2001) and F. graminearum (Kruger et al. Citation2002). Divion and Fluhr (2007) have reviewed the role of many nutrition-related genes during the fungal infection cycle. However, the requirement of these genes for pathogenicity was not verified in most cases, partly due to the difficulty of genetic transformation in many biotrophic fungi. The C. higginsianum–A. thaliana pathosystem provides a useful model to analyse fungal–plant interactions because both partners are genetically tractable (O'Connell et al. Citation2004). The differential regulation of many nutrition-related genes, including amino acid biosynthesis genes, nutrient transporters and enzymes required for nitrogen and carbohydrate metabolism, was previously observed early during C. higginsianum pathogenesis (Takahara et al. Citation2009 and unpublished data). Further analysis of auxotrophic mutants will help elucidate the role of nutrients in (hemi-) biotrophic growth in planta.
The biosynthetic pathway of arginine is very well-studied, and was first discovered in the urea cycle in mammals (Krebs and Henseleit Citation1932). More recently, it has been intensively studied in bacteria (Cunin et al. Citation1986) and fungi (Davis Citation1986). Genetic analysis of the arginine biosynthesis pathway is also provided as the earliest example of the one-gene, one-enzyme hypothesis in Neurospora crassa (Srb and Horowitz Citation1944). However, recent analysis revealed that the Arg6 precursor encoded two enzyme activities in a single mRNA derived from one gene in N. crassa (Wandinger-Ness and Weiss Citation1987), and a similar situation was proposed for the Arg5/Arg6 precursor of Saccharomyces cerevisiae (Boonchird et al. Citation1991). Computational analysis revealed that ChArg6 contains a putative proprotein cleavage consensus sequence “R-X-[R/K]-R” between the NAGK and NAGPR domains, which might be cleaved by furin-type (Arginine/Lysine residues) propeptide proteinases (Nakayama Citation1997; Duckert et al. Citation2004). This suggests that the ChArg6 precursor is transcribed as a single mRNA, translated as one protein, imported into mitochondria via the N-terminal targeting sequence, and then post-translationally processed into active mitochondrial enzymes. In S. cerevisiae, the functional enzymatic domains of NAGK and NAGPR were determined with expressed Arg5/Arg6 proteins using domain deletion experiments, and a putative junction region between the two enzyme activities was predicted (Boonchird et al. Citation1991). However, this junction site did not contain a typical consensus sequence for proprotein cleavage by this type of proteinases, indicating that a different enzyme may be required for this processing in S. cerevisiae.
Both arginine C. higginsianum auxotroph mutants showed reduced penetration and invasive growth ability on cellophane membranes, which was restored when l-arginine was supplied. This result indicates that these mutants possess an arginine uptake mechanism. In preliminary experiments, we attempted to restore pathogenicity of these mutants by supplementing the conidial suspensions used for host inoculation with exogenous l-arginine. However, supplementation did not produce clear infection phenotypes (i.e. symptoms and appressorial penetration efficiency), because the fungus grew saprophytically on the leaf surface as mycelium, without penetrating. Similar findings were reported in F. oxysporum f.sp. melonis, namely that supplementation of l-arginine did not restore the full pathogenicity of Arg1 arginine auxotrophic mutant, although their pathogenicity was partially recovered when spores were pre-incubated in arginine solution (Namiki et al. Citation2001). These results suggest that supplying excess nutrients reprograms fungal gene expression during infection. In fact, gene expression of Arg1 was repressed under nitrogen rich condition in F. oxysporum f.sp. melonis (Namiki et al. Citation2001). Many genes required for arginine biosynthesis undergo negative feedback regulation by arginine (Davis Citation1986). In this study, transcripts of both ChArg6 and ChCps were detected in planta, whereas expression was down-regulated in nutrient-rich medium compared to synthetic minimal medium. Many fungal genes that are induced in planta, including pathogenicity genes, are also induced under nutrient starvation conditions (Snoeijers et al. Citation2000). Regulatory genes upstream of amino acid biosynthesis or nitrogen metabolism, such as the CPC cross pathway control gene in N. crassa and CLNR1, the AreA-like global nitrogen regulator of C. lindemuthianum, are known to be upregulated under nitrogen starvation conditions (Hinebusch and Natarajan Citation2002; Pellier et al. Citation2003). In addition, Avr9 and CgDN3, encoding fungal secreted effector proteins in Cladosporium fulvum and Colletotrichum gloeosporioides, respectively, were also expressed under nitrogen starvation (Thomma et al. Citation2006; Stephenson et al. Citation2000). The relationship between fungal pathogenesis and changes in gene expression affecting nitrogen metabolism/catabolism may be much more complex. In the future, genome-wide transcriptome profiling of fungal pathogens at precise stages of plant infection will help elucidate the role of fungal nutrition in pathogenicity.
Acknowledgements
This work was supported by funding from the Max Planck Gesellschaft. HT was supported by a Max Planck Post-doctoral Fellowship.
References
- Bailey , A , Mueller , E and Bowyer , P. 2000 . Ornithine decarboxylase of Stagonospora (Septoria) nodorum is required for virulence toward wheat . J Biol Chem. , 275 : 14242 – 14247 .
- Balhadere , PV , Foster , AJ and Talbot , NJ. 1999 . Identification of pathogenicity mutants in the rice blast fungus Magnaporthe grisea by insertional mutagenesis . Mol Plant–Microbe Interact. , 12 : 129 – 142 .
- Birker , D , Heidrich , K , Takahara , H , Narusaka , M , Deslandes , L , Narusaka , Y , Reymond , M , Parker , JE and O'Connell , RJ. 2009 . A locus conferring resistance to Colletotrichum higginsianum is shared by four geographically distinct Arabidopsis accessions . Plant J. , 60 : 602 – 613 .
- Boonchird , C , Messenguy , F and Dubois , E. 1991 . Determination of amino acid sequences involved in the processing of the ARG5/ARG6 precursor in Saccharomyces cerevisiae . Eur J Biochem. , 199 : 325 – 335 .
- Both , M , Csukai , M , Stumpf , MPH and Spanu , PD. 2005 . Gene expression profiles of Blumeria graminis indicate dynamic changes to primary metabolism during development of an obligate biotrophic pathogen . Plant Cell , 17 : 2107 – 2122 .
- Caldovic , L and Tuchman , M. 2003 . N-acetylglutamate and its changing role through evolution . Biochem J. , 372 : 279 – 290 .
- Cunin , R , Glansdorff , N , Pierard , A and Stalon , V. 1986 . Biosynthesis and metabolism of arginine in bacteria . Microbiol Rev. , 50 : 193 – 225 .
- Davis , RH. 1986 . Compartmental and regulatory mechanisms in the arginine pathways of Neurospora crassa and Saccharomyces cerevisiae . Microbiol Rev. , 50 : 280 – 313 .
- Divon , HH and Fluhr , R. 2007 . Nutrition acquisition strategies during fungal infection of plants . FEMS Microbiol Lett. , 266 : 65 – 74 .
- Divon , HH , Rothan-Denoyes , B , Davydov , O , Di Pietro , A and Fluhr , R. 2005 . Nitrogen responsive genes are differentially regulated in planta during Fusarium oxysporum f.sp. lycopersici infection . Mol Plant Pathol. , 6 : 459 – 470 .
- Djulic , A , Schmid , A , Lenz , H , Sharma , P , Koch , C , Wirsel , SG and Voegele , RT. 2011 . Transient transformation of the obligate biotrophic rust fungus Uromyces fabae using biolistics . Fungal Biol. , 115 : 633 – 642 .
- Duckert , P , Brunak , S and Blom , N. 2004 . Prediction of proprotein convertase cleavage site . Protein Eng Des Sel. , 17 : 107 – 112 .
- Hahn , M and Mendgen , K. 1997 . Characterization of in planta induced rust genes isolated from a haustorium-specific cDNA library . Mol Plant–Microbe Interact. , 10 : 427 – 437 .
- Hahn , M , Neef , U , Struck , C , Gottfert , M and Mendgen , K. 1997 . A putative amino acid transporter is specifically expressed in haustoria of the rust fungus Uromyces fabae . Mol Plant–Microbe Interact. , 10 : 438 – 445 .
- Hinebusch , AG and Natarajan , K. 2002 . Gcn4p, a master regulator of gene expression, is controlled at multiple levels by diverse signals of starvation and stress . Eukaryot Cell. , 1 : 22 – 32 .
- Holliday , R. 1961 . Genetics of Ustilago maydis . Genet Res. , 2 : 204 – 230 .
- Horst , RJ , Doehlemann , G , Wahl , R , Hofmann , J , Schmiedl , A , Kahmann , R , Kämper , J , Sonnewald , U and Voll , LM. 2010 . Ustilago maydis infection strongly alters organic nitrogen allocation in maize and stimulates productivity of systemic source leaves . Plant Physiol. , 152 : 293 – 308 .
- Huser , A , Takahara , H , Schmalenbach , W and O'Connell , RJ. 2009 . Discovery of pathogenicity genes in the crucifer anthracnose fungus Colletotrichum higginsianum, using random insertional mutagenesis . Mol Plant–Microbe Interact. , 22 : 143 – 156 .
- Jakupovic , M , Heintz , M , Reichmann , P , Mendgen , K and Hahn , M. 2006 . Microarray analysis of expressed sequence tags from haustoria of the rust fungus Uromyces fabae . Fungal Genet Biol. , 43 : 8 – 19 .
- Kleemann , J , Takahara , H , Stuber , K and O'Connell , RJ. 2008 . Identification of soluble secreted proteins from appressoria of Colletotrichum higginsianum by analysis of expressed sequence tags . Microbiology , 154 : 1204 – 1217 .
- Krebs , HA and Henseleit , K. 1932 . Untersuchungen uber die Harnstotlhildung im TierkLrper . Hoppe-Seyler's Z. Physiol. Chem. , 210 : 33 – 66 .
- Kruger , WM , Pritsch , C , Chao , S and Muehlbauer , GJ. 2002 . Functional and comparative bioinformatic analysis of expressed genes from wheat spikes infected with Fusarium graminearum . Mol Plant–Microbe Interact. , 15 : 445 – 455 .
- Mathioni , SM , Beló , A , Rizzo , CJ , Dean , RA and Donofrio , NM. 2011 . Transcriptome profiling of the rice blast fungus during invasive plant infection and in vitro stresses . BMC Genomics , 19 : 12 – 49 .
- Nakayama , K. 1997 . Furin: a mammalian subtilisin/Kex2p-like endoprotease involved in processing of a wide variety of precursor proteins . Biochem J. , : 625 – 635 .
- Namiki , F , Matsunaga , M , Okuda , M , Inoue , I , Nishi , K , Fujita , Y and Tsuge , T. 2001 . Mutation of an arginine biosynthesis gene causes reduced pathogenicity in Fusarium oxysporum f.sp. melonis . Mol Plant–Microbe Interact. , 14 : 580 – 584 .
- Narusaka , Y , Narusaka , M , Park , P , Kubo , Y , Hirayama , T , Seki , M , Shiraishi , T , Ishida , J , Nakashima , M , Enju , A , Sakurai , T , Satou , M , Kobayashi , M and Shinozaki , K. 2004 . RCH1, a locus in Arabidopsis that confers resistance to the hemibiotrophic fungal pathogen Colletotrichum higginsianum . Mol Plant–Microbe Interact. , 17 : 749 – 762 .
- O'Connell , RJ and Panstruga , R. 2006 . Tête ê tête inside a plant cell: establishing compatibility between plants and biotrophic fungi and oomycetes . New Phytol. , 171 : 699 – 718 .
- O'Connell , RJ , Herbert , C , Sreenivasaprasad , S , Khatib , M , Esquerre-Tugaye , MT and Dumas , B. 2004 . A novel Arabidopsis – Colletotrichum pathosystem for the molecular dissection of plant-fungal interactions . Mol Plant–Microbe Interact. , 17 : 272 – 282 .
- Parra-Gessert , L , Koo , K , Fajardo , J and Weiss , RL. 1998 . Processing and function of a polyprotein precursor of two mitochondrial proteins in Neurospora crassa . J Biol Chem. , 273 : 7972 – 7980 .
- Pellier , AL , Lauge , R , Veneault-Fourrey , C and Langin , T. 2003 . CLNR1, the AREA/NIT2-like global nitrogen regulator of the plant fungal pathogen Colletotrichum lindemuthianum is required for the infection cycle . Mol Microbiol. , 48 : 635 – 655 .
- Rockwell , NA , Kyrsan , DJ , Komiyama , T and Fuller , RS. 2002 . Precursor processing by kex2/furin proteases . Chem Rev. , 102 : 4525 – 4548 .
- Price , CW , Holell , JH and Abdelal , ATH. 1978 . Purification and properties of the arginine-specific carbamoyl-phosphate synthase from Saccharomyces cerevisiae . J Gen Microbiol. , 106 : 145 – 151 .
- Snoeijers , SS , Perez-Garcia , A , Joosten , MHAJ and De Wit , PJGM. 2000 . The effect of nitrogen on disease development and gene expression in bacterial and fungal plant pathogens . Eur J Plant Pathol. , 106 : 493 – 506 .
- Solomon , PS and Oliver , RP. 2001 . The nitrogen content of the tomato leaf apoplast increases during infection by Cladosporium fulvum . Planta , 213 : 241 – 249 .
- Solomon , PS , Nielsen , PS , Clark , AJ and Oliver , RP. 2000 . Methionine synthase, a gene required for methionine synthesis, is expressed in planta by Cladosporium fulvum . Mol Plant Pathol , 1 : 315 – 323 .
- Solomon , PS , Thomas , SW , Spanu , P and Oliver , RP . 2004 . The utilisation of di/tripeptides by Stagonospora nodorum is dispensable for wheat infection . Physiol Mol Plant Pathol , 63 : 191 – 199 .
- Srb , AM and Horowitz , NH. 1944 . The ornithine cycle in Neurospora and its genetic control . J Biol Chem. , 154 : 129 – 139 .
- Stephenson , SA , Hatfield , J , Rusu , AG , Maclean , DJ and Manners , JM. 2000 . CgDN3: An essential pathogenicity gene of Colletotrichum gloeosporioides necessary to avert a hypersensitive-like response in the host Stylosanthes guianensis . Mol Plant–Microbe Interact. , 13 : 929 – 941 .
- Struck , C , Ernst , M and Hahn , M. 2002 . Characterization of a developmentally regulated amino acid transporter (AAT1p) of the rust fungus Uromyces fabae . Mol Plant Pathol. , 3 : 23 – 30 .
- Struck , C , Mueller , E , Martin , H and Lohaus , G. 2004 . The Uromyces fabae UfAAT3 gene encodes a general amino acid permease that prefers uptake of in planta scarce amino acids . Mol Plant Pathol. , 5 : 183 – 190 .
- Sweigard , JA , Carroll , AM , Farrall , L , Chumley , FG and Valent , B. 1998 . Magnaporthe grisea pathogenicity mutants obtained through insertional mutagenesis . Mol Plant–Microbe Interact. , 11 : 404 – 412 .
- Takahara , H , Tsuji , G , Kubo , Y , Yamamoto , M , Toyoda , K , Inagaki , Y , Ichinose , Y and Shiraishi , T. 2004 . Agrobacterium tumefaciens mediated transformation as a tool for random mutagenesis of Colletotrichum trifolii . J Gen Plant Pathol. , 70 : 93 – 96 .
- Takahara , H , Dolf , A , Endl , E and O'Connell , R. 2009 . Flow cytometric purification of Colletotrichum higginsianum biotrophic hyphae from Arabidopsis leaves for stage-specific transcriptome analysis . Plant J. , 59 : 672 – 683 .
- Tang , W , Coughlan , S , Crane , E , Beatty , M and Duvick , J. 2006 . The application of laser microdissection to in planta gene expression profiling of the maize anthracnose stalk rot fungus Colletotrichum graminicola . Mol Plant–Microbe Interact. , 19 : 1240 – 1250 .
- Thomma , BPHJ , Bolton , MD , Clergeot , PH and De Wit , PJ. 2006 . Nitrogen controls in planta expression of Cladosporium fulvum Avr9 but no other effector genes . Mol Plant Pathol. , 7 : 125 – 130 .
- Tsuji , G , Fujii , S , Fujihara , N , Hirose , C , Tsuge , S , Shiraishi , T and Kubo , Y. 2003 . Agrobacterium tumefaciens-mediated transformation for random insertional mutagenesis in Colletotrichum lagenarium . J Gen Plant Pathol. , 69 : 230 – 239 .
- Voegele , RT , Struck , C , Hahn , M and Mendgen , K. 2001 . The role of haustoria in sugar supply during infection of broad bean by the rust fungus Uromyces fabae . Proc Natl Acad Sci USA , 98 : 8133 – 8138 .
- Voegele , RT , Wirsel , S , Möll , U , Lechner , M and Mendgen , K. 2006 . Cloning and characterization of a novel invertase from the obligate biotroph Uromyces fabae and analysis of expression patterns of host and pathogen invertases in the course of infection . Mol Plant–Microbe Interact. , 19 : 625 – 634 .
- Wandinger-Ness , AU and Weiss , RL. 1987 . A single precursor protein for two separable mitochondrial enzymes in Neurospora crassa . J Biol Chem. , 262 : 5823 – 5830 .
- Zhang , Z , Henderson , C , Perfect , E , Carver , TLW , Skamnioti , P and Gurr , SJ. 2005 . Of genes and genomes, needles and haystacks: Blumeria graminis and functionality . Mol Plant Pathol. , 6 : 561 – 575 .
Supplementary figure S1. Amino acid sequence of putative Arg6 precursor protein. The open reading frame was predicted by FGENESH and Conrad prediction programs. Single underlining indicates a putative mitochondrial target signal. White and blue boxes indicate the ArgB and ArgC domains, corresponding to the N-acetylglutamate kinase (NAGK) and N-acetylglutamyl phosphate reductase (NAGPR), respectively. Putative propeptide cleavage site and the consensus sequence “R-X-[R/K]-R” were predicted between NAGK and NAGPR, as shown by asterisk and double underline.
![Supplementary figure S1. Amino acid sequence of putative Arg6 precursor protein. The open reading frame was predicted by FGENESH and Conrad prediction programs. Single underlining indicates a putative mitochondrial target signal. White and blue boxes indicate the ArgB and ArgC domains, corresponding to the N-acetylglutamate kinase (NAGK) and N-acetylglutamyl phosphate reductase (NAGPR), respectively. Putative propeptide cleavage site and the consensus sequence “R-X-[R/K]-R” were predicted between NAGK and NAGPR, as shown by asterisk and double underline.](/cms/asset/6a5f66d7-9224-4da4-aa32-be7b3e8374c0/tmyc_a_654353_o_f0005g.jpg)
Supplementary figure S2. Amino acid sequence of putative carbamoyl-phosphate synthetase large subunit. The open reading frame was predicted by FGENESH and Conrad prediction programs. Single underlining indicates a putative mitochondrial target signal. Conserved domains are indicated with boxes. N-terminal domain; white box, ATP-binding domains: blue boxes, oligomerisation domain; green box, methylglyoxal synthase-like domain; red box, respectively.
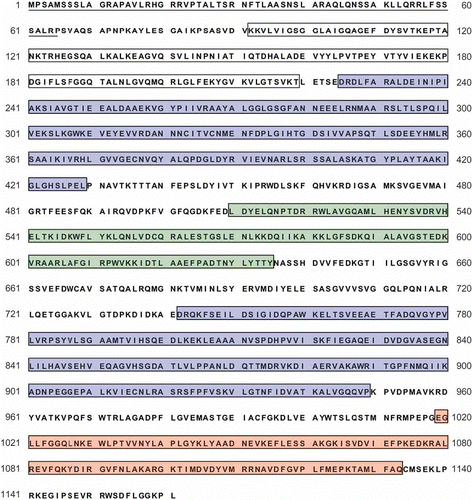