Abstract
Assemblage of aquatic hyphomycetes and decomposition of banyan leaf litter (Ficus benghalensis) were assessed in riparian tree holes and in a tropical coastal stream during rainy season up to 8 weeks in south-west coast of India. Although fungal assemblage was similar, leaf decomposition and leaf chemical changes differed between tree holes and the stream. Out of the 18 aquatic hyphomycetes, 17 were common on leaf litter in tree holes and the stream. Anguillospora longissima, Flagellospora curvula, Lunulospora curvula, Triscelophorus acuminatus and T. konajensis were the top five species in tree holes and the stream. The species richness was the highest during the second week in tree holes (11 species) and the sixth week in the stream (14 species), while the conidial output was the highest in tree holes and the stream during sixth week. The daily decay coefficient (k) was significantly faster in streams than in tree holes. Mass loss of banyan leaves between tree holes and the stream was positively correlated with conidial output by the aquatic hyphomycetes. The estimated half-life (t50) of leaf decomposition ranged between 70 days (stream) and 128 days (tree holes). Organic carbon in leaf litter gradually decreased, while nitrogen content decreased up to 1–2 weeks and then gradually increased over the incubation period. Ergosterol showed a gradual increase in tree hole litter, while it attained its peak in 4 weeks and declined thereafter in the stream litter. Loss of phosphorus concentration was rapid in the stream than in tree holes. Total phenolics concentration in leaves decreased rapidly in the stream than in tree holes. However, low dissolved oxygen in water and the slow release of phenolics from the leaf litter showed negative impact on the fungal activity and the rate of leaf decomposition in tree holes.
1. Introduction
Filamentous fungi play an important role in the decomposition of organic matter and energy flow in food webs in terrestrial and aquatic ecosystems. Aquatic hyphomycetes, a phylogenetically heterogeneous group, dominant on dead leaf litter and constitute an important trophic link between leaves and the stream invertebrates (Bärlocher Citation1992a, 1992b). In comparison with mycological studies in aquatic habitats, the canopies of riparian trees attracted less attention. Tree canopies represent a structurally complex and ecologically important subsystem support the evolution of flora, fauna and micro-organisms, which are rare or not commonly encountered on the forest floor (Nadkarni et al. Citation2001; Sridhar Citation2009). Fungi involving in breakdown and improvement of nutritional quality of accumulated leaf litter for the inhabiting fauna (e.g. invertebrates and tadpoles) in tree holes (vertical or lateral cavities formed in the trunk or branches of the trees either naturally due to stress or damage by the attack of microbes or fauna) assumes importance in trophic structure and energy flow in tree canopies. Additionally, tree holes might serve as source of inoculum of aquatic fungi that colonize organic matter in streams. A few studies have been carried out to evaluate the water-borne fungi in tree canopies, which are mainly confined to temperate regions (Magyar et al. Citation2005; Gönczöl & Révay Citation2006; Sridhar Citation2009).
Western Ghats and south-west coast of India receives substantial rains during south-west monsoon (June–September) and the tree canopies are wet throughout monsoon and part of post-monsoon season (October–January). Thus, occurrence of aquatic or water-borne fungi in tree canopies (e.g. leaf surface, through fall and stem flow) is not surprising (Sridhar Citation2009). The question remains to understand the pattern of fungal assemblage and leaf litter decomposition in tree canopies in comparison to the stream ecosystem. There are a few detailed studies on leaf litter decomposition in the Indian streams (Raviraja et al. Citation1996, 1998; Sridhar et al. Citation2011; Sudheep & Sridhar Citation2013). The primary objective of this study was to investigate the structure and function of aquatic hyphomycete assemblages in riparian tree holes and to compare it with that of a second-order stream in the south-west coast of India during monsoon season. The secondary objective was to assess the relationship between descriptions of fungal colonization, decomposition and chemical changes of banyan leaves.
2. Materials and methods
2.1. Leaf litter
As banyan leaves showed higher richness of aquatic hyphomycetes in Konje stream (Sridhar et al. Citation1992), senescent and freshly fallen leaves of banyan (Ficus benghalensis L.) were collected from a single tree in Mangalore University Campus. The lamina portions of the leaves were punched into 1.5 cm discs using a cork borer. They were air-dried up to 3–4 weeks (30°C), 75 leaf discs were placed in each big nylon litter bags (15 × 15 cm, mesh size 1 mm), and 25 pre-weighed leaf discs were placed in small nylon litter bags (5 × 5 cm, mesh size 1 mm) and placed in each big litter bag for mass-loss determination. The mass of 25 leaf discs enclosed in mesh bags ranged between 590 and 670 mg.
2.2. Field experiments
The field experiment was conducted during monsoon season (July–August 2006). The second-order stream, Konaje (12° 48′ N, 74° 55′ E) flowing through forest and plantation crops adjacent to the Mangalore University Campus was selected. On 6 July 2006, the litter bags were introduced into tree holes and to the stream. The litter bags were tied to tree roots along the margin in five different locations at a distance of approximately 50–100 m, where there was water flow throughout the experimental period. As banyan trees were not close to the stream, five riparian trees were chosen (three of Holigarna ferruginea Marchand and two of Syzygium caryophyllatum (Linn.) Alston at a distance of approximately 50–100 m from each other. Litter bags were tied to the branches or the trunk and incubated in a single hole in each tree. During the experimental period, litter bags were fully or partially submerged in tree holes. One litter bag from each tree hole and stream location was sampled in five occasions (1, 2, 4, 6 and 8 weeks) and brought to the laboratory for fungal analysis, leaf mass-loss determination and leaf chemical analysis.
Water temperature, pH and conductivity of each tree hole and stream location were measured at the time of sampling between 9 am and 12 noon (Water Analyzer 371, Systronics, Gujarat, India). Water samples collected were fixed during 9 am – 12 noon and the dissolved oxygen was estimated by Winkler’s method (APHA Citation1995).
2.3. Examination for fungi
On each sampling, five leaf discs per replicate were incubated in 150 ml distilled water in 250 ml Erlenmeyer flasks, and aerated with Pasteur pipettes connected to aquarium pumps, for 48 h (23 ± 2°C) to induce the production of conidia by aquatic hyphomycetes. The conidial suspension was filtered through Millipore filter (5 μm; diameter 47 mm) and the filter was stained with 0.1% cotton blue in lactophenol. The filter was cut into half, mounted on a microscope slide with lactic acid and the conidia were identified (magnification, 200–1000 ×) and counted (1/8–1/4 of the filter area was scanned if the conidial density in the filter was high, the whole filter was scanned if conidia were sparse). The leaf discs were dried at 100°C up to 24 h and the dry mass was determined on attaining constant weight. The conidial output was expressed per gram dry mass of leaf discs. Fungal conidia were identified using monographs and taxonomic keys (see Sudheep & Sridhar Citation2011).
2.4. Ergosterol estimation
To estimate ergosterol in leaf discs, modified microwave-assisted extraction was followed (Young Citation1995). Lyophilized leaf discs (50–100 mg) were churned in liquid nitrogen, suspended in a solution of 2 ml methanol and 0.5 ml 2 M NaOH and sealed in 15 ml screw-cap glass tubes. Six extraction tubes with samples were placed in a 250 ml capped plastic bottle and microwaved (50% power for 95 s in a microwave oven) and then removed from the plastic bottle after cooling to room temperature. The solution was neutralized with 1 ml of 1 M HCl, and ergosterol concentration was extracted with three consecutive hexane washes. The combined hexane fractions were evaporated to dryness, and the residue was re-extracted in 1 ml of methanol. The solution was injected into a HPLC-C18 column (Sigma-Aldrich, St. Louis, MO, USA) and eluted with methanol at 1.5 ml min−1 at 20°C (elution time, 5.3 min). The ergosterol content was estimated by comparison of peak areas with those of external standards.
2.5. Leaf mass loss
The leaf discs in small litter bags placed within big litter bags were sampled for mass-loss assessment. This was determined by comparing original and the remaining leaf mass after air-drying and corrected by exposing subsamples to 100°C for 24 h in five replicates. Exponential decay coefficient per day (k/d) was estimated by linear regression on ln-transformed data (MATLAB 6.5).
2.6. Leaf chemical analysis
Dried leaf discs were powdered and the organic carbon was measured as outlined by Kalembasa and Jenkinson (Citation1973). Nitrogen estimation of dried leaf powder was carried out according to Chale (Citation1993). Ascorbic acid method was followed to determine the total phosphorus in leaf samples (APHA Citation1995). Total phenolics of the leaf disc powder was determined by Rosset et al. (Citation1982).
2.7. Data analysis
Water parameters, ergosterol content and leaf chemistry between tree holes and the stream were compared by t-test (StatSoft Inc. Citation2008). Sporulation by aquatic hyphomycetes (log transformed) against incubation period were compared among habitats using two-way ANOVA (habitats and time as categorical variables) followed by Tukey’s test (Zar Citation1996). Cumulative conidial output among the habitats was compared by ANCOVA followed by Tukey’s test.
Exponential decay coefficients per day (k/d) were estimated for leaf litter in tree holes and in the Konaje stream, based on the negative exponential decay model (Petersen & Cummins Citation1974) using MATLAB 6.5. Comparison of slopes of habitats (tree holes and stream) was carried out by ANCOVA (habitats and time as categorical and continuous variables, respectively) followed by Tukey’s test. Relationship between the rate of decomposition and conidial output in tree holes and the stream was assessed by linear regression (MATLAB 6.5). The time (days) required for the decomposition of half of the initial leaf mass (t50) was determined by the following relation:
3. Results
3.1. Water quality
gives water quality in tree holes and Konaje stream during leaf immersion and sampling period. The mean temperature in tree holes during study period ranged from 23.2 to 26.3°C, pH 6.5 to 6.9, conductivity 37.6 to 51.2 μS cm−1 and dissolved oxygen 3.2 to 3.9 mg l−1, while in the stream 23.2 to 26.3°C, 6.7 to 8.1, 117 to 1330 μS cm−1 and 1.1 to 1.7 mg l−1, respectively. Water temperature (t-test, p < 0.001), pH (t-test, p < 0.01), conductivity (t-test, p < 0.001) and dissolved oxygen (t-test, p < 0.01) were significantly differed between tree holes and the stream.
Table 1. Water parameters in tree holes and Konaje stream during sampling period (n = 18, mean ± SD) (range in parenthesis)
3.2. Fungal colonization and ergosterol
reveals colonization of aquatic hyphomycetes on leaf litter submerged in tree holes and the Konaje stream during 8 weeks of study. Altogether, 18 fungi colonized the leaf litter in tree holes and the stream. Seventeen fungi were found in tree holes as well as in the stream with exception of Helicomyces roseus, which was confined to tree holes. Anguillospora longissima, Flagellospora curvula, Lunulospora curvula, Triscelophorus acuminatus and T. konajensis were the top five species in tree holes and the stream. Maximum species richness was attained after 2 weeks in tree holes (11 species) and 6 weeks in the stream ((a)). Except for eighth week, conidial output was higher in the stream than in tree holes ((b)). The mean conidial output was the highest in the stream compared to the tree holes (2437 vs. 1848 g−1 leaf litter) (). Considering all sampling dates, the conidial output was one-third lower in tree holes than in the stream (two-way ANOVA, p < 0.001) (). The cumulative conidial output was higher in the stream than in tree holes (ANCOVA, p = 0.004).
Table 2. Water-borne fungi (conidia g−1 dry mass) on banyan leaf litter exposed to tree holes and Konaje stream during sampling period
Figure 1. Fluctuation in (a) species richness, (b) conidial output and (c) ergosterol during the decomposition of banyan leaf litter immersed in tree holes and the Konaje stream (n = 5, mean ± SD).
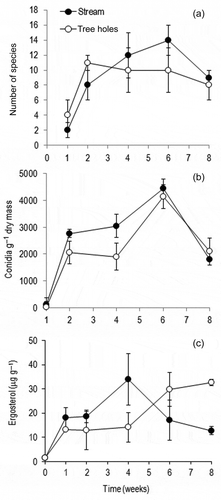
The ergosterol concentration in leaf discs was 1.4 μg g−1 before immersion. In tree holes, ergosterol increased gradually over the study period, while it attained a peak at 4 weeks (33.9 μg g−1) in the stream ((c)). The ergosterol content in leaf litter between tree holes and the stream was significantly differed (t-test, p < 0.05).
3.3. Leaf mass loss
After 8 weeks immersion, litter had lost 11% initial mass in tree holes and 22% initial mass in the stream ((a)) indicating significantly higher mass loss in the stream (ANCOVA, p = 0.020). This translated into a significantly lower decomposition rate in tree holes (k = 0.0024 d−1) than in the stream (k = 0.0043 d−1) (). Mass loss of banyan leaves between the habitats was positively correlated with the conidial output by aquatic hyphomycetes (linear regression, p = 0.003, r2 = 0.25).
Figure 2. Leaf mass remaining (a), organic carbon (b), nitrogen (c), C:N ratio (d), phosphorus (e) and total phenolics (f) of banyan leaf litter during decomposition in tree holes and the Konaje stream (n = 5, mean ± SD).
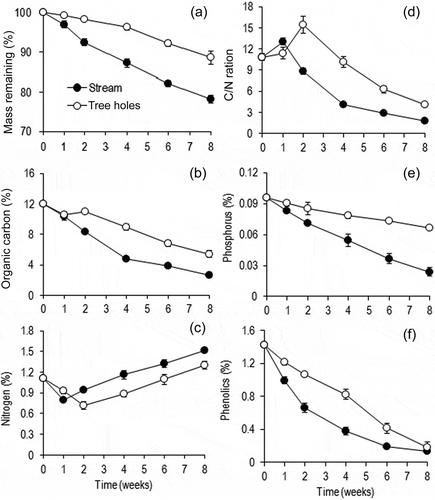
The estimated time for 50% litter mass loss was higher in tree holes (128 days) than in the stream (70 days) (). Comparison of decay dynamics between environments was performed by considering all replicates and sampling dates. The daily decay coefficient (k) varied between 0.0024 (tree hole) and 0.0043 (stream). Estimated time for 50% mass loss (t50), was ranged between 70 (stream) and 128 (tree holes) days.
3.4. Leaf chemical changes
Organic carbon concentration in leaf discs decreased gradually in the stream and tree holes, however, stream leaf discs lost organic carbon faster compared to leaf discs in tree holes ((b)). Nitrogen concentration in leaf discs decreased initially (1–2 weeks) and then increased gradually until the end of the experiment in both habitats ((c)). The C:N ratio of leaf discs peaked after 1 (stream)–2 weeks (tree holes) and then decreased until the end of the incubation period ((d)). Phosphorus concentration decreased faster in the stream than in tree hole leaf discs ((e)). A steep decrease in total phenolics concentrations in leaf discs was found in the stream than in tree holes; however, after 8 weeks the phenolics concentration was similar in both habitats ((f)). Organic carbon, nitrogen, phosphorus and phenolics in leaf discs were significantly differed between tree holes and the stream (t-test, p < 0.05).
4. Discussion
Aquatic hyphomycetes have evolved different strategies to survive under unusual conditions (e.g. soil, tree roots and tree canopies) (Sridhar & Kaveriappa Citation1987; Bärlocher Citation1992b; Sridhar & Bärlocher Citation1993; Gönczöl & Révay Citation2003, Citation2004). Their ability to survive outside the water (e.g. leaf/wood litter in the stream margins) and tree canopies (e.g. tree holes and epiphytes) make these environments potential sources of aquatic hyphomycetes that can colonize the streams. In this study, we found 18 species of aquatic hyphomycetes colonizing leaf litter in tree holes, which is similar to the species richness associated with decomposing litter in the Konaje stream (18–20 species; this study; Sridhar & Kaveriappa Citation1984; Sridhar et al. Citation1992).
Table 3. Summary of regression analysis of mass losses of banyan leaf discs in tree holes and stream (k = decay coefficient; r2 = coefficient of determination; t50 = half-life)
Based on daily decay coefficients (k), Petersen and Cummins (Citation1974) classified the decomposition rates of leaf litter roughly into three groups: ‘slow’ (k < 0.005), ‘medium’ (k = 0.005–0.01) and ‘fast’ (k > 0.01). The previous studies on decay of acacia, banyan, cashew and eucalypt leaves in Konaje streams revealed medium decay coefficient compared to the present study (0.0063–0.0090 vs. 0.0043 d−1, respectively) possibly due to environmental differences between the stream reaches considered in this study and in the previous ones (Raviraja et al. Citation1996). The decay coefficient was also higher in mid-altitude of the Western Ghat stream (Sampaje) than the present study (0.0063–0.009 vs. 0.0043), so also the polluted stretches of River Nethravathi (0.0066–0.0075 vs. 0.0043) (Raviraja et al. Citation1996, 1998). No studies are available to compare the decay coefficient of leaf litter in tree holes. The current study revealed that the decay rate is 1–3 folds slower in tree holes compared to the Konaje or Sampaje streams and the River Nethravathi (0.0024 vs. 0.0043–0.009).
The nitrogen concentration on decomposing leaf litter decreased initially, which can be attributed to initial leaching (Davis et al. Citation2003). Subsequently, nitrogen concentration increased most likely as a result from microbial colonization and the formation of complexes between phenolics/lignin and proteins or other nitrogenous compounds (Graça & Bärlocher Citation1998; Canhoto & Graça Citation1999; Bärlocher Citation2005). The slower and more prolonged decrease in nitrogen concentration of leaf litter in tree holes than in the stream suggests a less intense leaching as well as a delayed fungal colonization of leaf litter in tree holes. Initial increase and subsequent decrease in C:N ratio of leaf litter in the streams and tree holes in the present study also reflected the elevation of nitrogen concentration and loss of carbon.
Changes in phosphorus in decomposing leaf litter are dependent on the type of leaf litter and nature of the stream. Phosphorus seems to reflect a balance between leaching and immobilization by fungal activity. Alder, poplar and willow leaf litter in temperate regions showed an initial increase followed by a gradual decrease (Chauvet Citation1987). Eucalypt in Spanish streams lost phosphorus in headwaters, while accumulated in lower reaches (Pozo Citation1993). In fresh and dried leaves of Rhizophora mucronata in a south-west mangrove, an initial loss of phosphorus was seen up to 1 week followed by its elevation (Ananda et al. Citation2008). In the present study, loss of phosphorus was rapid in the stream than in tree holes and coincided with more conidial output. Similar observation was made in the streams of Konaje and Sampaje, where banyan and cashew leaves lost phosphorus more rapidly (than acacia and eucalypt) and coincided with elevated conidial output (Raviraja et al. Citation1996). Sharp decline of phosphorus in banyan leaf in River Nethravathi also showed more conidial output of aquatic hyphomycetes (Raviraja et al. Citation1998).
Greater conidial output of aquatic hyphomycetes was associated with faster leaf colonization and rates of leaf decomposition (Gulis & Subekropp Citation2003). Most likely, the very high or too low nutrients (especially nitrogen and phosphorus contents) in the Konaje stream during the study period might be responsible for low rates of leaf decomposition. On the contrary, although tree holes possess more nitrogen and phosphorus concentration, they reflect low dissolved oxygen (0–4 weeks, 1.1–1.7 mg l−1) or increased phenolic contents in water might have a negative effect on the colonization and functions of aquatic hyphomycetes. The low dissolved oxygen concentration (Medeiros et al. Citation2009) and the higher phenolics concentration in water resulting from leaf leaching in a contained environment (Canhoto & Graça Citation1999), might negatively affect fungal colonization of and activity on litter in tree holes than in the stream. Loss of phenolics in banyan litter in the present study is comparable to banyan and cashew litter in the Konaje and Sampaje streams, while it was slow in acacia and eucalypt (Raviraja et al. Citation1996, 1998). Besides phenolics, perhaps higher bacterial activity depending on the nutrient levels in tree holes might reflect in low dissolved oxygen in our study.
Aquatic hyphomycete assemblages seem to have adapted to harsh conditions, which might partially be due to the presence of species-specific requirements (for instance, L. curvula is known to be a warm water preferring species and its dominance in tree holes and streams in the present study is not surprising). Similar adaptation or tolerance for low dissolved oxygen might exist in tree hole inhabitants. The species richness in tree holes is similar to that in the stream and thus tree holes serve as a potential source of inoculum to the streams. Nevertheless, an increased fungal biomass and nitrogen concentration in leaf litter in tree holes by aquatic hyphomycetes meet the nutritional requirement of invertebrates inhabiting in tree holes.
5. Conclusions
Compared to temperate region, studies on leaf litter decomposition in aquatic habitats of tropical region are scarce. The present study constitutes the first study on the leaf litter decomposition in tree holes of tropical habitats. Future studies need to evaluate the pattern and process of plant litter decomposition in different tropical aquatic habitats, so also the canopies of riparian tree species. Such studies will give insight into the basic process of energy flow and food web in tropical ecosystem. Besides, these studies serve as baseline data to forecast the status of the habitat (healthy or impoverished or regenerated) to impart remedial measures to control human interference on the ecosystem.
Acknowledgements
The authors are grateful to Mangalore University for granting permission to carry out this study at the Department of Biosciences. One of us (KSK) acknowledges the research fellowship granted under SC/ST Cell, Mangalore University and Rajiv Gandhi Fellowship, University Grants Commission, New Delhi, India. Authors are thankful to Prof. F. Bärlocher for helping in the estimation of ergosterol and Madhu S. Kandikere for his statistical analysis. We are indebted to the referees for critical comments to improve this paper.
References
- Ananda K, Sridhar KR, Bärlocher F. 2008. Breakdown of fresh and dried Rhizophora mucronata leaves in a mangrove of southwest India. Wetlands Ecol Manage. 16:1–9.
- APHA. 1995. Standard methods for examination of water and waste water. 19th ed. Washington (DC): American Public Health Association.
- Bärlocher F. 1992a. The ecology of aquatic hyphomycetes. Berlin: Springer-Verlag.
- Bärlocher F. 1992b. Recent developments in stream ecology and their relevance to aquatic mycology. In: Bärlocher F, editor. The ecology of aquatic hyphomycetes. Berlin: Springer-Verlag; p. 16–37.
- Bärlocher F. 2005. Freshwater fungal communities. In: Dighton J, Oudemans P, White J, editors. The fungal community. 3rd ed. Boca Raton (FL): CRC Press; p. 39–59.
- Canhoto C, Graça MAS. 1999. Leaf barriers to fungal colonization and shredders (Tipula lateralis) consumption of decomposing Eucalyptus globulus. Microbial Ecol. 37:163–172.
- Chale FMM. 1993. Degradation of mangrove leaf litter under aerobic conditions. Hydrobiologia. 257:177–183.
- Chauvet E. 1987. Changes in the chemical composition of alder, poplar and willow leaves during decomposition in a river. Hydrobiologia. 148:35–44.
- Davis SE III, Corronado-Molina C, Childers DL, Day JW Jr. 2003. Temporally dependent C, N, and P dynamics associated with the decay of Rhizophora mangle L. leaf litter in oligotrophic mangrove wetlands of the Southern Everglades. Aquat Bot. 75:199–215.
- Gönczöl J, Révay Á. 2003. Treehole fungal communities: aquatic, aero-aquatic and dematiaceous hyphomycetes. Fungal Divers. 12:19–24.
- Gönczöl J, Révay Á. 2004. Fungal spores in rainwater: stemflow, throughfall and gutter conidial assemblages. Fungal Divers. 16:67–86.
- Gönczöl J, Révay Á. 2006. Species diversity if rainborne hyphomycete conidia from living trees. Fungal Divers. 22:37–54.
- Graça MAS, Bärlocher F. 1998. Proteolytic gut enzymes in Tipula caloptera – interaction with phenolics. Aquat Insects. 21:11–18.
- Gulis V, Suberkropp K. 2003. Leaf decomposition and microbial activity in nutrient enriched and unaltered reaches of a headwater strand. Freshwat Biol. 48:123–134.
- Kalembasa SJ, Jenkinson DS. 1973. A comparative study of titrimetric and gravimetric methods for the determination of organic carbon in soil. J Sci Food Agric. 24:1985–1090.
- Magyar D, Gönczöl J, Révay Á, Grillenzoni F, Seijo-Coello MDC. 2005. Stauro- and scolecoconidia in floral and honeydew honeys. Fungal Divers. 20:103–120.
- Medeiros AO, Pascoal C, Graça MAS. 2009. Diversity and activity of aquatic fungi under low oxygen conditions. Freshwat Biol. 54:142–149.
- Nadkarni NM, Mewin MC, Niedert J. 2001. Forest canopies, plant diversity. In: Levin S, editor. Encyclopedia of biodiversity. Vol. 3. New York (NY): Academic Press; p. 27–40.
- Petersen RC, Cummins KW. 1974. Leaf processing in woodland stream. Freshwat Biol. 4:343–368.
- Pozo J. 1993. Leaf litter processing of alder and eucalypts in the Agüera stream system (North Spain) I. Chemical changes. Arch Hydrobiol. 127:299–317.
- Raviraja NS, Sridhar KR, Bärlocher F. 1996. Breakdown of introduced and native leaves in two Indian streams. Int Rev Ges Hydrobiol. 81:529–539.
- Raviraja NS, Sridhar KR, Bärlocher F. 1998. Breakdown of Ficus and Eucalyptus leaves in an organically polluted river in India: fungal diversity and ecological functions. Freshwat Biol. 39:537–545.
- Rosset J, Bärlocher F, Oertli JJ. 1982. Decomposition of conifer needles and deciduous leaves in two Black Forest and two Swiss Jura streams. Int Rev Ges Hydrobiol. 67:695–711.
- Sridhar KR. 2009. Fungi in the tree canopy – an appraisal. In: Rai M, Bridge P, editors. Applied mycology. Wallingford: CAB International; p. 73–91.
- Sridhar KR, Arun AB, Madhyastha MN. 2011. Aquatic hyphomycetes and leaf litter decomposition in River Kali (Western Ghats), India. Kavaka. 39:15–22.
- Sridhar KR, Bärlocher F. 1993. Aquatic hyphomycetes on leaf litter in and near a stream in Nova Scotia, Canada. Mycol Res. 97:1530–1535.
- Sridhar KR, Chandrashekar KR, Kaveriappa KM. 1992. Research on the Indian subcontinent. In: Bärlocher F, editor. The ecology of aquatic hyphomycetes. Heidelberg: Springer-Verlag; p. 182–211.
- Sridhar KR, Kaveriappa KM. 1984. Seasonal occurrence fungi in Konaje stream (Mangalore), India. Hydrobiologia. 119:101–105.
- Sridhar KR, Kaveriappa KM. 1987. Occurrence and survival of aquatic hyphomycetes in terrestrial conditions. Trans Brit Mycol Soc. 89:606–609.
- Sudheep NM, Sridhar KR. 2011. Diversity of lignicolous and Ingoldian fungi on woody litter in River Kali (Western Ghats, India). Mycology. 2:98–108.
- Sudheep NM, Sridhar KR. 2013. Colonization and diversity of aquatic hyphomycetes in relation to decomposition of submerged leaf litter in River Kali (Western Ghats, India). Mycosphere. 4:456–476.
- StatSoft Inc. 2008. Statistica, Version # 8. Tulsa (OK): StatSoft Inc.
- Young CJ. 1995. Microwave-assisted extraction of the fungal metabolite ergosterol and total fatty acids. J Agric Food Chem. 43:2904–2910.
- Zar JH. 1996. Biostatistical analysis. 3rd ed. Englewood Cliffs (NJ): Prentice Hall International Inc.