ABSTRACT
A concerted evolution model has been proposed to explain the observed lack of sequence variation among the multiple ribosomal RNA (rRNA) gene copies in many different eukaryotic species. Recent studies on the level of intragenomic variations in the rRNA gene repeats of fungi resulted in controversial conclusions. In this study, we clearly showed that significant polymorphisms of the internal transcribed spacers (ITS1 and ITS2) of ribosomal DNA (rDNA) exist within the genome of a strain of the yeast species Pichia membranifaciens. More interestingly, we showed that the intragenomic ITS sequence polymorphisms were formed by intergenomic rDNA recombination among different P. membranifaciens strains with significantly different ITS sequences. Intergenomic rDNA recombination was also responsible for the diversification of rDNA sequences in different strains of the species. After the events bring together different rDNA types in individual genomes of the P. membranifaciens strains compared, rDNA sequence heterogeneity has remained in the genome of one but eliminated by homogenisation in the genomes of other strains. Our findings show new clue for further investigation on the mechanism of concerted evolution of rRNA genes in eukaryotes.
Introduction
Ribosomal RNAs (rRNAs) are ubiquitous to all living organisms. Four rRNA genes, 26/28S, 18S, 5.8S and 5S, are present in eukaryotes. The 18S, 5.8S and 26/28S rRNA genes, separated by the two internal transcribed spacers (ITS1 and ITS2), are arranged in a transcription unit (Venema and Tollervey Citation1999). The rRNA unit is arrayed in tandemly repeated clusters with several hundred to a few thousand copies on one or separate chromosomes in the genome. It is generally believed that all the copies of the rRNA unit are identical or nearly identical in nucleotide sequence within the genome of a given organism because of the highly stringent functional constraints on rRNA molecules (Long and Dawid Citation1980; Hillis and Dixon Citation1991). A “concerted evolution” model has been proposed to explain the observed lack of sequence variation among rRNA gene copies in many different species (Zimmer et al. Citation1980; Coen et al. Citation1982; Liao Citation1999, Citation2000; Eickbush and Eickbush Citation2007).
However, exceptional cases have been reported in different organisms, including archaea, bacteria and eucaryotes (Mylvaganam and Dennis Citation1992; Wang et al. Citation1997; Yap et al. Citation1999). In eukaryotes, two types of 18S rRNA genes differing by 3.5% nucleotides were found within the individual genome of the parasite Plasmodium berghei (Gunderson et al. Citation1987). Two types of 18S rRNA genes differing by 8% nucleotides coexisted in the individual genome of the metazoan Dugesia mediterranea (Carranza et al. Citation1996). Intragenomic ribosomal DNA (rDNA) sequence polymorphisms have also been reported in fungi. Divergent ITS types have been found in single genomes of Fusarium and Ganoderma species (O’Donnell and Cigelnik Citation1997; Wang and Yao Citation2005). In a yeast species, Clavispora lusitaniae, intragenomic variations in the 26S rDNA D1/D2 domain have been reported (Lachance et al. Citation2003).
More recently, the level of intragenomic variations in the rRNA gene repeats of fungi were examined in two separate studies using whole-genome shotgun sequencing (WGSS) data, but the conclusions are inconsistent. Ganley and Kobayashi (Citation2007) analysed the rDNA reads from WGSS projects of five fungi, including four ascomycetous species Ashbya gossypii, Saccharomyces cerevisiae, Saccharomyces paradoxus and Aspergillus nudulans, and one basidiomycetous species Cryptococcus neoformans. They found that the level of sequence variation within the rDNA arrays of these eukaryotic microorganisms was remarkably low, supporting the concerted evolution model. James et al. (Citation2009) analysed the rDNA shotgun reads from the WGSS data sets for 34 strains of S. cerevisiae. Contrary to the result of Ganley and Kobayashi (Citation2007), James et al. (Citation2009) found that significant rDNA sequence variation exists within individual genomes and that the intragenomic variation can reach an order of magnitude between individual strains. Unexpectedly high intragenomic polymorphisms in nuclear ribosomal genes were also observed in four plant pathogenic ascomycetes species by Simon and Weiß (Citation2008), who consequently assumed that nuclear ribosomal genes might not always evolve in a strictly concerted manner.
The origins of intragenomic rRNA gene polymorphisms in prokaryotes were explained by horizontal gene transfer (Yap et al. Citation1999). Gene conversion was shown to be the mechanism for homogenisation of rRNA genes in prokaryotes (Liao Citation2000). Compared to the tandem-arrayed organisation of eukaryotic rRNA genes in high copy number (generally over 100; in some cases >1000), prokaryotic rRNA genes are generally dispersed throughout a genome in limited copy numbers (generally <10) (Liao Citation2000). Since the concerted evolution theory has been proposed mainly based on sequence homogeneity in tandemly repeated multigene families (Eickbush and Eickbush Citation2007), the findings of intragenomic rRNA gene polymorphisms in eukaryotes are more challenging.
In an investigation on genetic diversity of strains belonging to a yeast species Pichia membranifaciens, remarkable intragenomic polymorphisms of the rDNA ITS regions were discovered. More interestingly, the intragenomic ITS sequence polymorphisms were clearly shown to be formed by intergenomic rDNA recombination among different P. membranifaciens strains. Intragenomic recombinations between the polymorphic ITS repeats were also observed. Furthermore, we found that intergenomic rDNA recombination is also responsible for the diversification of rDNA sequences in different strains of the species.
Materials and methods
Organisms, single-cell colony isolation and continuous cultivation
The yeast strains employed are listed in . They were obtained from the Centraalbureau voor Schimmelculture (CBS), The Netherlands. About 100 μl of adequately diluted actively growing cell suspension of a yeast strain was spread onto yeast extract-malt extract-peptone-glucose (Yarrow Citation1998) plate. The well isolated single cells were marked under a microscope. After incubation at 25°C for 48 h, the clearly isolated colonies developed from the marked single cells were picked for further study.
Table 1. Yeast strains studied.
P. membranifaciens strain CBS 215 which exhibits remarkable intragenomic polymorphisms in the rDNA ITS regions was subjected for continuous cultivation. The strain was grown in 200 ml of YPD (1% yeast extract, 2% peptone and 2% dextrose) medium and incubated at 25°C in a shaker (160 rpm). At the end of the exponential phase, 1 ml of the cell suspension was transferred to 200 ml new medium for continuing cell duplication. This procedure went on for 4 weeks until completion of around 400 generations. About 20 μl of cells taken at the end of approximately 100, 200, 300 and 400 generations were diluted and plated on YPD plates. Three to five well-isolated colonies were randomly picked for DNA sequence analysis.
Genomic DNA extraction, PCR and direct sequencing
Genomic DNA of yeast strains were extracted by the method of Makimura et al. (Citation1994). The DNA fragment covering the ITS1-5.8S-ITS2 region was amplified and sequenced with the primer pair ITS1 (5ʹ–GTC GTA ACA AGG TTT CCG TAG GTG–3ʹ) and ITS4 (5ʹ–TCC TCC GCT TAT TGA TAT GC–3ʹ).
Cloning sequencing
Purified polymerase chain reaction (PCR) products of the ITS1-5.8S-ITS2 region from strain CBS 215 were cloned using the pUCm-T Vector system (BBI) and transformed into E. coli DH5α competent cells. Positive clones containing the target fragment were randomly picked for sequence analysis. The target fragments inserted in the plasmids were directly sequenced.
Sequence alignment and phylogenetic analysis
Alignment of sequences was carried out with the Clustal X program (Thompson et al. Citation1997). The phylogenetic trees were constructed from the evolutionary distance data calculated from Kimura’s two-parameter model (Kimura Citation1980) by using the neighbour-joining method (Saitou and Nei Citation1987). Reference sequences were retrieved from GenBank under the accession numbers indicated in the phylogenetic tree.
Pulsed-field gel electrophoresis
Intact yeast chromosomal DNA was prepared for pulsed-field gel electrophoresis (PFGE) by the method of Bai et al. (Citation2000). Chromosomal DNA bands were separated in 0.8% (w/v) agarose gel in 0.5 × TBE (45 mM Tris/borate, 1 mM EDTA, pH 8.0) buffer in a contour-clamped homogeneous electric field (CHEF) electrophoresis apparatus (CHEF-Mapper XA, Bio-Rad, Hercules, CA, USA). Electrophoresis was performed for 51 h at 3 V/cm with pulse time ramping from 300 s to 600 s. The temperature of the running buffer was maintained at 12–14°C. After electrophoresis, the PFGE results were imaged with the AlphaImager 2200 gel documentation system (Alpha Innotech, San Leandro, CA, USA).
Flow cytometry
Yeast cells for flow cytometric analysis were prepared using the method described in Burke et al. (Citation2000) with minor modifications. Cells were grown in 3 ml of YPD broth at 28°C for 12 h and harvested from 300 μl of the culture for further processing. DNA was stained with propidium iodide and DNA content of yeast cells was determined using a BD FACSAria™ Flow Cytometer (Becton Dickinson, NJ, USA). S. cerevisiae strains FY1679-01B (haploid), FY1679-01D (haploid) and FY1679 (diploid) were used as reference strains.
Results
Intragenomic rDNA sequence polymorphisms in P. membranifaciens strain CBS 215
The fragment covering the rDNA ITS1-5.8S-ITS2 regions of strain CBS 215 was amplified by PCR using nuclear DNA as the template. When we tried to determine the sequence of the purified PCR product by direct sequencing method, we found that overlapped peaks appeared after certain positions in the sequencing chromatograms using ITS1 or ITS4 as the sequencing primer (). The phenomena indicated that non-homogenous sequences were included in the PCR products. Repeated purification of strain CBS 215 using the streak plate technique failed to eliminate the phenomena. In order to exclude the possibility of impurity, colonies developed from single cells were isolated sterilely by using single-cell colony isolation technique. Dozens of single-cell colonies were randomly selected for direct ITS region sequencing. The overlapped peaks appeared in each of the sequencing chromatograms of every colony at the same locations as in those of the original culture of strain CBS 215. The result indicated that the sequences of the rDNA ITS repeats within the genome of strain CBS 215 were polymorphic.
Sequence variations among different ITS types within the genome of strain CBS 215
The PCR product covering the ITS region (including the 5.8S gene) amplified from the DNA template from a single-cell colony of strain CBS 215 was cloned. A total of 107 clones containing target fragments were randomly selected and sequenced. Clear sequence chromatograms without overlapped peaks were obtained for every clone. Two types of ITS1 and two types of ITS2 were recognised from the clones (). ITS1-A (89 bp in length, 76 clones) differed from ITS1-B (88 bp in length, 31 clones) by 14 mismatches (11 substitutions and 3 indels). ITS2-A (148 bp in length, 75 clones) differed from ITS2-B (150 bp in length, 32 clones) by 32 mismatches (18 substitutions and 14 indels) ().
Figure 2. Sequence alignments of the different ITS types within the genome of Pichia membranifaciens strain CBS 215. At a given position, a nucleotide identical to that in the upper line is indicated by a dot, a gap is indicated by a hyphen.
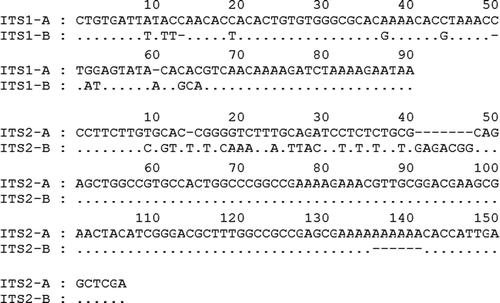
When the whole sequences of the ITS (ITS1-5.8S-ITS2) clones were compared, four types of ITS repeats formed by different combinations of the two types of ITS1 with the two types of ITS2 were recognised. Their sequences were type I (68 clones), ITS1-A + 5.8S + ITS2-A; type II (24 clones), ITS1-B + 5.8S + ITS2-B; type III (7 clones), ITS1-B + 5.8S + ITS2-A; and type IV (8 clones), ITS1-A + 5.8S + ITS2-B.
Direct amplification of different ITS types from the genome of strain CBS 215
PCR amplification is know to be susceptible to the formation of chimeric products if the template DNA contains multiple divergent copies of the target fragment (Meyerhans et al. Citation1990; Wang and Wang Citation1996, Citation1997; Von Wintzingerode et al. Citation1997; Qiu et al. Citation2001; Boucher et al. Citation2004). Since the genome DNA from strain CBS 215 contained multiple divergent ITS repeats dominated by types I and II, the possibility that the clones of types III and IV were the chimera formed during PCR process could not be excluded, though the amplified fragment was less than 500 bp and chimera formation was less likely for such short products (Boucher et al. Citation2004).
In order to exclude this possibility, PCR primers were designed based on the variable sequences of different ITS1 and ITS2 types for specific amplification of the four types of ITS repeats from the genome DNA of strain CBS 215. Two forward primers ITS1-AF (5ʹ–CCG GCC ATC ATT ACT GTG ATT ATA CC–3ʹ) and ITS1-BF (5ʹ–GCG CGG ACT ATA GTA TAA CAG CA–3ʹ) and two reverse primers ITS2-AR (5ʹ–ATC TGC AAA GAC CCC GGT G–3ʹ) and ITS2-BR (5ʹ–AGC AGC TCT GCC GTC TCC AC–3ʹ) were designed. The primer pair ITS1-AF and ITS2-AR was used for specific amplification of type I; ITS1-BF and ITS2-BR for type II; ITS1-BF and ITS2-AR for type III; and ITS1-AF and ITS2-BR for type IV. PCR was performed at a very stringent condition to avoid non-specific amplification (36 cycles with denaturation at 94°C for 30 s, annealing at 69°C for 30 s and extension at 72°C for 20 s). The theoretic lengths of the fragments flanked by the primer pairs for ITS types I–IV are 287, 261, 233 and 315 bp, respectively.
Clear amplicons with the sizes corresponding to the theoretic values were obtained from PCRs using each of the primer pairs and the DNA template of CBS 215 genome (). Sequence analysis of each of the amplicons confirmed that the four types of ITS repeats were directly and specifically amplified. The result confirmed that in addition to the dominant ITS types I and II, types III and IV coexist in the genome of strain CBS 215.
Sequence comparison of ITS repeats among different P. membranifaciens strains
In order to investigate the origin of the polymorphic ITS sequences in the genome of strain CBS 215, representatives of other strains of P. membranifaciens with different ITS sequences were selected for comparison. Intragenomic ITS polymorphisms were not found in these strains by direct sequencing (Wu et al. Citation2006). Evolutionary relationships of different ITS types of strain CBS 215 with those of other separate strains were depicted by phylogenetic trees drawn from the sequence alignments of the whole ITS fragment, ITS1 only and ITS2 only, respectively (). Two strains of a closely related species P. mandshurica (Wu et al. Citation2006) were used as the outgroup. In the tree drawn from the whole ITS sequences, five clusters I–V were recognised. ITS types I–IV of CBS 215 were, respectively, distributed in the first four clusters. Cluster V was formed by strains CBS 189 and CBS 1329 ()). In the ITS1 tree, two clusters corresponding to ITS1-A and ITS1-B sequences were recognised ()). In the ITS2 tree, three clusters were formed ()). The first two possessed ITS2-A and ITS2-B sequences, respectively. The third cluster consisted of strains CBS 189 and CBS 1329, indicating that they possess a unique ITS2 sequence, which is apparently responsible for their separation from the other strains in the tree drawn from the whole ITS sequences ()). The base mismatches of different ITS1 and ITS2 types of strain CBS 215 with the corresponding ITS regions of related strains compared are summarised in .
Table 2. Sequence mismatches of different ITS1 and 2 types of strain CBS 215 with the corresponding ITS regions of closely related strains of Pichia membranifaciens.
Figure 4. Neighbour-joining trees drawn from the sequence alignments of (a) the whole ITS repeat, (b) ITS1 only and (c) ITS2 only, of Pichia membranifaciens strains. Pichia manchurica strains CBS 209 and CBS 241 are used as the outgroup. Bootstrap percentages obtained from 1000 bootstrap replicates are shown.
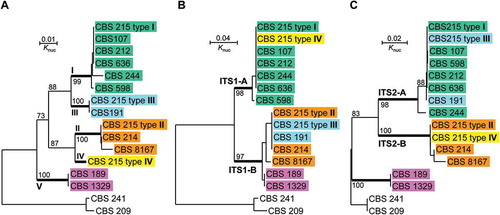
The phylogenetic analysis and sequence comparison showed that each of the ITS1 and ITS2 types in strain CBS 215 exist separately in other P. membranifaciens strains () and ); ). In regard to the whole ITS repeat, types I, II and III in strain CBS 215 were also separately found from other independent strains ()). Strains CBS 107, CBS 212 and CBS 244 possess ITS type I; strains CBS 214 and CBS 8167 possesses ITS type II and strain CBS 191 possesses ITS type III.
Electrophoretic karyotyping and flow cytometry
Chromosomal DNA banding patterns of nine P. membranifaciens strains studied were compared (). Two to four bands were resolved with molecular sizes ranging from 2.06 to 3.15 Mb. Strain CBS 244 appeared to have two additional small bands with molecular sizes of approximately 900 and 980 Kb, respectively. One additional small band (~780 Kb in length) was observed in strain CBS 636. Although the chromosome profiles of some of the strains compared were similar, none of them were identical. The result indicates that all of the strains compared represent differentiated strains, other than clones of single isolates.
Figure 5. Electrophoretic karyotypes of Pichia membranifaciens strains. Hw, Hansenula wingei YB-4662-VIA.
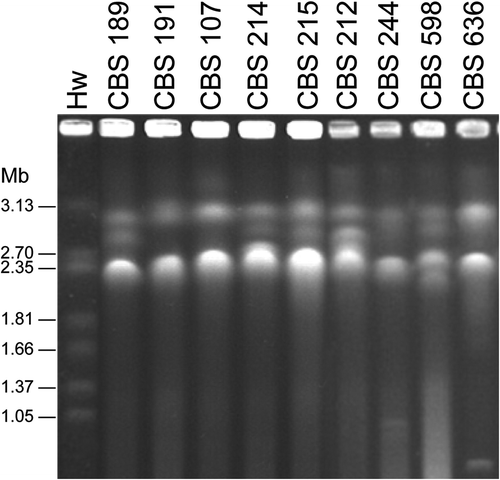
As shown earlier, the polymorphic ITS types of strain CBS 215 come from different strains with different ITS sequences. It is possible that this strain is a diploid or polyploid organism simply formed by cell fusion of two or more strains with different ITS sequences. Flow cytometry analysis denied this assumption. Strains CBS 107 representing cluster I and CBS 214 representing cluster II were selected together with strain CBS 215 for comparative flow cytometry analysis. The level of fluorescence per cell as shown in indicates that the DNA content per cell of the latter is even slightly lower than those of the former two strains.
Discussion
In this study, we clearly showed that significant polymorphisms of the rDNA ITS region exist within the genome of a strain (CBS 215) belonging to the yeast species Pichia membranifaciens. Four types of ITS repeat (ITS types I–IV) formed by different combinations of two types of ITS1 (ITS1-A and B) with two types of ITS2 (ITS2-A and B) were recognised in the genome of strain CBS 215. ITS1-A differs from ITS1-B and ITS2-A from ITS2-B by up to 15.6% and 20.5% base mismatches, respectively ( and ). Sequence comparison of different types of the whole ITS repeat, ITS1 and ITS2 of strain CBS 215 with the corresponding rDNA regions of other strains of the same species showed that (i) the polymorphic ITS sequences in the genome of strain CBS 215 originate from separate genomes of different strains and (ii) intergenomic recombination between different types of ITS repeats results in further diversification of the ITS sequences among different strains of P. membranifaciens.
The rDNA ITS region is one of the most frequently used molecular markers in phylogeny and identification of yeasts at the species level (James et al. Citation1996; Montrocher et al. Citation1998; Sugita et al. Citation1999a, Citationb; Fell et al. Citation2000; Scorzetti et al. Citation2002; Kurtzman and Robnett Citation2003) and has been selected as a universal DNA barcode marker for Fungi (Schoch et al. Citation2012). Previous studies have claimed that conspecific yeast strains usually have fewer than 1% nucleotide differences in the ITS 1 and 2 regions overall (Nagahama et al. Citation1999; Sugita et al. Citation1999a, Citationb; Scorzetti et al. Citation2002). However, the present study showed that divergent ITS1 and ITS2 types which differed by up to 15.6% and 20.5% base mismatches, respectively, coexist in the single genome of strain CBS 215. Each of the different ITS types distribute separately in other P. membranifaciens strains. Molecular data obtained in previous studies suggest that strains in the five clusters with remarkable divergent ITS sequences recognised in ) are apparently conspecific. The 26S rDNA D1/D2 sequences of the strains in these groups were identical or differed by only three or fewer substitutions (Wu et al. Citation2006). The DNA similarity values obtained from DNA/DNA hybridisation between strains CBS 107 (= IFO 10215) in cluster I, CBS 8167 (= IFO 10731) in cluster II and CBS 1329 (= IFO 10725) in cluster V, were 71–87% (Ueda-Nishimura and Mikata Citation2001). It is reasonable to expect similar or higher DNA similarity values between other strains from clusters I to V. Comparison of genetic cross and DNA reassociation has indicated that yeast strains showing DNA similarity at 70% or higher are usually conspecific (Kurtzman Citation1998). Our data suggest that conspesific yeast strains may occasionally have significantly divergent ITS sequences.
Sequence comparison of multiple independent strains clearly indicates that the polymorphic ITS sequences of strain CBS 215 arise from different strains with different ITS types. Since the majority of the strains compared possess ITS type I only (cluster I in )) and the dominant ITS types in strain CBS 215 are types I and II, the most possibility is that ITS type I of strain CBS 215 is from a strain in cluster I and type II from a strain in cluster II. The possibility that the other two minor ITS types III and IV in the genome of strain CBS 215 also come from different stains (e.g. type III from a strain in cluster III and type IV from a strain in Cluster IV) cannot be excluded. However, this possibility may be very low, because the process needs multiple hybridisation or similar events and strains with ITS type III or IV only may be rarely distributed in nature (one strain with ITS type III only but none with ITS type IV only has been recognised). Therefore, we think that the minor ITS types III and IV in the genome of strain CBS 215 are most possibly formed by intragenomic recombination between the two dominant types I and II.
The independent distribution of each of ITS types I to III in separate strains is clearly shown in this study ()). It is reasonable to infer that ITS type IV also distributes independently in other strains in nature. Since the four ITS types (I–IV) are apparently formed by different combinations of two types of ITS1 with two types of ITS2 (), the reasonable explanation to the independent occurrence of each of the four ITS types in separate strains is intergenomic recombination. For instance, the ITS repeat of strain CBS 191 which possesses type III only may be formed by intergenomic recombination between two strains with ITS types I and II, respectively. The sequence similarity of ITS1 of strains CBS 189 and CBS 1329 to ITS1-B suggests that the ITS repeat of these two strains may be formed by intergenomic recombination between the ITS repeats from a strain with ITS1-B and a strain with another unique ITS2 type ().
Intragenomic polymorphisms of the ITS region have also been reported in other eukaryotes, including plants (Baldwin et al. Citation1995; Alvarez and Wendel Citation2003), beetles (Vogler and DeSalle Citation1994), nematodes (Zijlstra et al. Citation1995), sponges (Wörheide et al. Citation2004) and filamentous fungi (O’Donnell and Cigelnik Citation1997; Wang and Yao Citation2005). The divergent ITS types in the same individual genomes of these organisms are usually described as nonorthologous and attributed to the origin of ancient interspecific hybridisation (xenologous origin) or gene duplication (paralogous origin), but direct evidences have not been presented. Recombination or gene conversion in the ITS region has been observed in a hybrid of a mushroom (Hughes and Petersen Citation2001). The hybrid was recognised as being formed by a natural hybridisation event between two separate species of Flammulina (F. velutipes and F. rossica) which resulted in a homogenised ribosomal repeat containing elements of both parents (Hughes and Petersen Citation2001). The present study clearly showed that the ITS heterogeneity of strain CBS 215 arises from separate strains with different ITS sequences in the same species. An event of mating, fusion or hybridisation between strains with different ITS types may account for the phenomenon. Flow cytometry analysis showed that strain CBS 215 is not simply a diploid or polyploid yeast formed by fusion of two or more cells of two or more different strains (). Therefore, the exact way that brings together different ITS types into a single genome is uncertain.
As discussed earlier, the ITS repeats of strains CBS 198, CBS 191 and CBS 1329 are apparently formed by intergenomic recombination of different ITS types from different strains. However, intragenomic ITS sequence polymorphisms have not been detected in any of these strains. It is interesting to note that, after the events bringing together different rRNA gene repeats into single genomes, concerted evolution has occurred in strains CBS 189, CBS 191 and CBS 1329, but not in strain CBS 215. The genome of strain CBS 215 seems stable, since ITS sequence polymorphisms maintained in repeated subcultures and in all randomly selected colonies of the strain after repeated purification using the plate streak method. The escape of rRNA gene repeats of strain CBS 215 from homogenisation by concerted evolution is challenging. One possible explanation is the defect in gene conversion mechanisms required for concerted evolution of rRNA genes in strain CBS 215. The hypotheses proposed to explain long-term persistence of rDNA polymorphsims following hybridisation or polyplodisation have been summarised by O’Donnell and Cigelnik (Citation1997) as (1) their chromosomal location may not be telomeric, (2) the sequences may have diverged beyond the point that interchromosomal conversion can occur and (3) concerted evolution may be more efficient within a chromosomal locus than between repeats dispersed on non-homologous chromosomes. Rooney (Citation2004) has shown that the intragenomic heterogeneous 18S rRNA genes in apicomplexan protists undergo birth-and-death rather than concerted evolution. In these protists, rRNA genes are dispersed throughout the genome and are not organised in a tandem array. More recently, Rooney and Ward (Citation2005) revealed that the dispersed 5S rRNA genes of filamentous fungi also evolve under a birth-and-death model with strong purifying selection and rapid gene turnover. Strain CBS 215 with intragenomic heterogeneous ITS repeats and strains CBS 189, CBS 191 and CBS 1329 with homogenous ITS repeats are closely related within a single yeast species. It will be interesting to compare rRNA gene organisation and structure of these strains using high-quality genome sequencing. The results will certainly be helpful to elucidate the mechanism allowing the maintenance of the rDNA polymorphsims in strain CBS 215, and in return, to have a better understanding of the concerted evolution model of rRNA genes.
Acknowledgements
We thank Dr Vincent Robert from CBS, The Netherlands, for kindly supplying the yeast strains. We also thank Dr Alejandro P. Rooney, Agricultural Research Service, U.S. Department of Agriculture, USA, and Dr Jianping Xu, Department of Biology, McMaster University, Canada, for their invaluable suggestions and comments on this study and on the drafts of this manuscript. This study was supported by the National Natural Science Foundation of China (NSFC) under grant numbers 30470005 and 30825002.
Disclosure statement
No potential conflict of interest was reported by the authors.
Additional information
Funding
References
- Alvarez I, Wendel JF. 2003. Ribosomal ITS sequences and plant phylogenetic inference. Mol Phyl Evol. 29:417–434.
- Bai FY, Liang HY, Jia JH. 2000. Taxonomic relationships among the taxa in the Candida guilliermondii complex, as revealed by comparative electrophoretic karyotyping. Int J Syst Evol Microbiol. 50:417–422.
- Baldwin BG, Sanderson MJ, Porter JM, Wojciechowski MF, Campbell CS, Donghue MJ. 1995. The ITS region of nuclear ribosomal DNA: a valuable source of evidence on angiosperm phylogeny. Ann Mo Bot Gard. 82:247–277.
- Boucher Y, Douady CJ, Sharma AK, Kamekura M, Doolittle WF. 2004. Intragenomic heterogeneity and intergenomic recombination among haloarchaeal rRNA genes. J Bacteriol. 186:3980–3990.
- Burke D, Dawson D, Stearns T. 2000. Methods in yeast genetics: a cold spring harbor laboratory course manual, 2000 edition. Plainview, NY: Cold Spring Harbor Laboratory Press.
- Carranza S, Giribet G, Riberat C, Baguna J, Riutort M. 1996. Evidence that two types of 18S rDNA coexist in the genome of Dugesia (Schmidtea) mediterranea (Platyhelminthes, Turbellaria, Tricladida). Mol Biol Evol. 13:824–832.
- Coen E, Strachan T, Dover G. 1982. Dynamics of concerted evolution of ribosomal DNA and histone gene families in the melanogaster species subgroup of Drosophila. J Mol Biol. 158:17–35.
- Eickbush TH, Eickbush DG. 2007. Finely orchestrated movements: evolution of the ribosomal RNA genes. Genetics. 175:477–485.
- Fell JW, Boekhout T, Fonseca A, Scorzetti G, Statzell-Tallman A. 2000. Biodiversity and systematics of basidiomycetous yeasts as determined by large subunit rDNA D1/D2/domain sequence analysis. Int J Syst Bacteriol. 50:1351–1371.
- Ganley AR, Kobayashi T. 2007. Highly efficient concerted evolution in the ribosomal DNA repeats: total rDNA repeat variation revealed by whole-genome shotgun sequence data. Genome Res. 17:184–191.
- Gunderson JH, Sogin ML, Wollet G, Hollingdale M, de la Cruz VF, Waters AP, McCutchan TF. 1987. Structurally distinct, stage-specific ribosomes occur in Plasmodium. Science. 238:933–937.
- Hillis DM, Dixon MT. 1991. Ribosomal DNA: molecular evolution and phylogenetic inference. Q Rev Biol. 66:411–453.
- Hughes KW, Petersen RH. 2001. Apparent recombination or gene conversion in the ribosomal ITS region of a Flammulina (Fungi, Agaricales) hybrid. Mol Biol Evol. 18:94–96.
- James SA, Collins MD, Roberts IN. 1996. Use of an rRNA internal transcribed spacer region to distinguish phylogenetically closely related species of the genera Zygosaccharomyces and Torulaspora. Int J Syst Bacteriol. 46:189–194.
- James SA, O’Kelly MJ, Carter DM, Davey RP, van Oudenaarden A, Roberts IN. 2009. Repetitive sequence variation and dynamics in the ribosomal DNA array of Saccharomyces cerevisiae as revealed by whole-genome resequencing. Genome Res. 19:626–635.
- Kimura M. 1980. A simple method for estimating evolutionary rate of base substitutions through comparative studies of nucleotide sequences. J Mol Evol. 16:111–120.
- Kurtzman CP. 1998. Nuclear DNA hybridization: quantitation of close genetic relationships. In: Kurtzman CP, Fell JW, editors. The yeasts, A taxonomic study. 4th ed. Amsterdam: Elsevier; p. 63–68.
- Kurtzman CP, Robnett CJ. 2003. Phylogenetic relationships among yeasts of the ‘Saccharomyces complex’ determined from multigene sequence analyses. FEMS Yeast Res. 3:417–432.
- Lachance MA, Daniel HM, Meyer W, Prasad GS, Gautam SP, Boundy-Mills K. 2003. The D1/D2 domain of the large-subunit rDNA of the yeast species Clavispora lusitaniae is unusually polymorphic. FEMS Yeast Res. 4:253–258.
- Liao D. 1999. Concerted evolution: molecular mechanism and biological implications. Am J Hum Genet. 64:24–30.
- Liao D. 2000. Gene conversion drives within genic sequences: concerted evolution of ribosomal RNA genes in bacteria and archaea. J Mol Evol. 51:305–317.
- Long EO, Dawid IB. 1980. Repeated genes in eukaryotes. Annu Rev Biochem. 49:727–764.
- Makimura K, Murayama YS, Yamaguchi H. 1994. Detection of a wide range of medically important fungi by the polymerase chain reaction. J Med Microbiol. 40:358–364.
- Meyerhans A, Vartanian JP, Wain-Hobson S. 1990. DNA recombination during PCR. Nucleic Acids Res. 18:1687–1691.
- Montrocher R, Verner M-C, Briolay J, Gautier C, Marmeisse R. 1998. Phylogenetic analysis of the Saccharomyces cerevisiae group based on polymorphisms of rDNA spacer sequences. Int J Syst Bacteriol. 48:295–303.
- Mylvaganam S, Dennis PP. 1992. Sequence heterogeneity between the two genes encoding 16S rRNA from the halophilic archaebacterium Halobacterium marismortui. Genetics. 130:399–410.
- Nagahama T, Hamamoto M, Nakase T, Horikoshi K. 1999. Kluyveromyces nonfermentans sp. nov., a new yeast species isolated from the deep sea. Int J Syst Bacteriol. 49:1899–1905.
- O’Donnell K, Cigelnik E. 1997. Two divergent intragenomic rDNA ITS2 types within a monophyletic lineage of the fungus Fusarium are nonorthologous. Mol Phyl Evol. 7:103–116.
- Qiu X, Wu L, Huang H, McDonel PE, Palumbo AV, Tiedje JM, Zhou J. 2001. Evaluation of PCR-generated chimeras, mutations, and heteroduplexes with 16S rRNA gene-based cloning. Appl Environ Microbiol. 67:880–887.
- Rooney AP. 2004. Mechanisms underlying the evolution and maintenance of functionally heterogeneous 18S rRNA genes in apicomplexans. Mol Biol Evol. 21:1704–1711.
- Rooney AP, Ward TJ. 2005. Evolution of a large ribosomal RNA multigene family in filamentous fungi: birth and death of a concerted evolution paradigm. Proc Natl Acad Sci USA. 102:5084–5089.
- Saitou N, Nei M. 1987. The neighbor-joining method: a new method for reconstructing phylogenetic trees. Mol Biol Evol. 4:406–425.
- Schoch CL, Seifert KA, Huhndorf S, Robert V, Spouge JL, Levesque CA, Chen W; Fungal Barcoding Consortium. 2012. Nuclear ribosomal internal transcribed spacer (ITS) region as a universal DNA barcode marker for Fungi. Proc Natl Acad Sci USA 109:6241–6246.
- Scorzetti G, Fell JW, Fonseca A, Statzell-Tallman A. 2002. Systematics of basidiomycetous yeasts: a comparison of large subunit D1/D2 and internal transcribed spacer rDNA regions. FEMS Yeast Res. 2:495–517.
- Simon UK, Weiß M. 2008. Intragenomic variation of fungal ribosomal genes is higher than previously thought. Mol Biol Evol. 25:2251–2254.
- Sugita T, Cañete-Gibas CF, Takashima M, Nakase T. 1999a. Three new species of Bullera isolated from leaves in the Ogasawara Islands. Mycoscience. 40:491–501.
- Sugita T, Nishikawa A, Ikeda R, Shinoda T. 1999b. Identification of medically relevant Trichosporon species based on sequences of internal transcribed spacer regions and construction of a database for Trichosporon identification. J Clin Microbiol. 37:1985–1993.
- Thompson JD, Gibson TJ, Plewniak F, Jeanmougin F, Higgins DG. 1997. The Clustal X windows interface: flexible strategies for multiple sequence alignment aided by quality analysis tools. Nucleic Acids Res. 24:4876–4882.
- Ueda-Nishimura K, Mikata K. 2001. Reclassification of Pichia scaptomyzae and Pichia galeiformis. Antonie van Leeuwenhoek. 79:371–375.
- Venema J, Tollervey D. 1999. Ribosome synthesis in Saccharomyces cerevisiae. Annu Rev Genet. 33:261–311.
- Vogler AP, DeSalle R. 1994. Evolution and phylogenetic information content of the ITS-1 region in the tiger beetle Cicindela dorsalis. Mol Biol Evol. 11:393–405.
- von Wintzingerode F, Gobel UB, Stackebrandt E. 1997. Determination of microbial diversity in environmental samples: pitfalls of PCR-based rRNA analysis. FEMS Microbiol Rev. 21:213–229.
- Wang DM, Yao YJ. 2005. Intra-strain ITS heterogeneity in Ganoderma species. Can J Microbiol. 51:113–121.
- Wang GCY, Wang Y. 1996. The frequency of chimeric molecules as a consequence of PCR co-amplification of 16S rRNA genes from different bacterial species. Microbiology. 142:1107–1114.
- Wang GCY, Wang Y. 1997. Frequency of formation of chimeric molecules as a consequence of PCR coamplification of 16S rRNA genes from mixed bacterial genomes. Appl Environ Microbiol. 63:4645–4650.
- Wang Y, Zhang ZS, Ramanan N. 1997. The actinomycete Thermobispora bispora contains two distinct types of transcriptionally active 16S rRNA genes. J Bacteriol. 179:3270–3276.
- Wörheide G, Nicholsb SA, Goldberg J. 2004. Intragenomic variation of the rDNA internal transcribed spacers in sponges (Phylum Porifera): implications for phylogenetic studies. Mol Phyl Evol. 33:816–830.
- Wu ZW, Robert V, Bai FY. 2006. Genetic diversity of the Pichia membranifaciens strains revealed from rRNA gene sequencing and electrophoretic karyotyping, and the proposal of Candida californica comb. Nov FEMS Yeast Res. 6:305–311.
- Yap WH, Zhang Z, Wang Y. 1999. Distinct types of rRNA operons exist in the genome of the actinomycete Thermomonospora chromogena and evidence for horizontal transfer of an entire rRNA operon. J Bacteriol. 181:5201–5209.
- Yarrow D. 1998. Methods for the isolation, maintenance and identification of yeasts. In: Kurtzman CP, Fell JW, editors. The yeasts, A taxonomic study. 4th ed. Amsterdam: Elsevier; p. 77–100.
- Zijlstra C, Lever AEM, Uenk BJ, van Silfhout CH. 1995. Differences between ITS regions of isolates of root-knot nematodes Meloidogyne hapla and M. chitwoodi. Phytopathology. 85:1231–1237.
- Zimmer EA, Martin SL, Beverley SM, Kan YW, Wilson AC. 1980. Rapid duplication and loss of genes coding for the α chains of hemoglobin. Proc Natl Acad Sci USA. 77:2158–2162.