ABSTRACT
Microsclerotia (MS) formation was successfully induced in Metarhizium rileyi (Ascomycetes: Hypocreales) in liquid culture. To optimise the process variables of liquid fermentation, we first used a two-level fraction design to confirm the variables, including inoculum density, initial pH, shaker speed, and temperature, affecting M. rileyi MS production. Three variables were found to be important. Subsequently, a 23 full factorial central composite design (CCD) and response surface methodology were applied to ascertain the optimal level of each variable. A second-order polynomial was determined and shaker speed and inoculum density were found to be the primary variables affecting MS yields. Finally, we realised and optimised M. rileyi MS submerged fermentation based on previous findings. A maximum MS yields (3.84 × 104 MS/mL) were recorded in submerged fermentation at an initial pH of 5.5, growth temperature of 26°C, inoculum density of 10%, higher aeration rate (150 rpm in the initial 3 days and 200 rpm in the subsequent 3 days), and higher agitation rate of 800 L/h sterile air.
Introduction
Microsclerotia (MS), with a diameter of 50–600 μm, are pseudoparenchymatous aggregations of hyphae, comprising only a few cells (Yin et al. Citation2012). They are produced as overwintering structures by many phytopathogenic fungi (Colotelo Citation1974; López-Escudero et al. Citation2006). Fungi can survive for long periods in adverse environments by producing MS. For biological applications, MS has been successfully induced in entomopathogenic fungi such as Beauveria bassiana (Ascomycetes: Hypocreales), Beauveria brongniartii, Metarhizium anisopliae (Ascomycetes: Hypocreales), Metarhizium rileyi, and Purpureocillium lilacinum in liquid culture (Jackson & Jaronski Citation2009; Wang et al. Citation2011; Yin et al. Citation2012; Yang et al. Citation2014; Song et al. Citation2016b). The stability of dried MS preparations and their potential to produce infective conidia when conditions are suitable for growth make MS a promising fungal propagule for use as a mycoinsecticide to manage insect pests (Jackson et al. Citation2010; Behle et al. Citation2013; Song et al. Citation2014a), as antagonists of plant pathogenic fungi (Kobori et al. Citation2015), as bioherbicides for the management macrophytes (Shearer Citation2007), or bionematicides against root knot nematodes (Song et al. Citation2016b). Therefore, for the commercial production of fungi that are not cost-effective by solid substrate fermentation, the conidia, the effective propagule against pests, can be replaced with the MS.
The Nomuraea rileyi as a well-known entomopathogenic fungus, re-classified as M. rileyi according to multigene phylogenetics (Kepler et al. Citation2014), thrives in obligate hosts, particularly, lepidopterous pests (Chen et al. Citation2012). The current large-scale solid fermentation methods for the production of M. rileyi conidia, the present effective component, are not cost-effective and require fastidious sporulation conditions along with stimulatory light, which limit large-scale fermentation and commercialisation of M. rileyi (Faria & Wraight Citation2007).
Our laboratory had successfully accomplished M. rileyi MS production in liquid amended medium (AM) culture (Yin et al. Citation2012; Song et al. Citation2013), and further research aimed at the scale-up fermentation for M. rileyi MS production is underway. The quality as well as quantity of MS propagules in liquid culture should be examined with equal attention, because both the parameters can be affected by medium composition and culture conditions. Recently, our group optimised the medium composition to improve MS yields (Song et al. Citation2014a). As the sources of medium are widespread, large-scale fermentation for M. rileyi MS production could be easily achieved. Our primary experiments suggested that shaker speed, inoculum density, pH, and temperature are the major culture variables that affect M. rileyi MS production. For the development of efficient fermentation, the culture conditions and liquid culture fermentation process should be optimised owing to their impact on the M. rileyi MS yields. Optimisation studies could also help in understanding the interactions among the parameters as well as the mechanism of MS development (Song et al. Citation2013, Citation2016a; Jiang et al. Citation2014; Liu et al. Citation2014; Zhou et al. Citation2015).
The application of statistical experimental design techniques in fermentation process development can result in improved MS yields, reduced process variability, and reduced development time and overall costs. Response surface methodology (RSM), a collection of mathematical and statistical techniques for building empirical models, can employ quantitative data obtained from properly designed experiments to solve multivariate equations simultaneously and carry out only a limited and fixed number of experiments. More importantly, this method can be used in the presence of complex interactions. RSM has been successfully employed for optimizing the biomass of many microorganisms, such as Bacillus thuringiensis, M. anisopliae, and Pichia anomala (Liu & Tzeng Citation1998; Vohra & Satyanarayana Citation2002; Prakash et al. Citation2008; Li et al. Citation2009; Shi et al. Citation2009).
The present study was aimed at optimisation of the variables of culture conditions and development of an optimal process of submerged fermentation for M. rileyi MS production. We first used a two-level fractional factorial design (FFD) to confirm the important variables that significantly affect M. rileyi MS yields. Then, central composite design (CCD) and RSM methods were applied to optimise the major variables, including shaker speed, inoculum density, and temperature. The results showed that shaker speed and inoculum density were the primary variables that significantly affected M. rileyi MS yields. Finally, we developed and optimised the process of submerged fermentation for M. rileyi MS production in a 30-L bioreactor based on the confirmed primary variables.
Materials and methods
Strain and inoculum preparation
The M. rileyi strain, CQNr01, was obtained from the Chongqing Engineering Research Center for Fungal Insecticides, Chongqing, China. The strain had been used to study the optimisation of culture medium for MS production (Yin et al. Citation2012; Song et al. Citation2014a) and study the molecular mechanism of MS development in our earlier research (Song et al. Citation2013, Citation2016a; Jiang et al. Citation2014; Liu et al. Citation2014; Zhou et al. Citation2015). The strain was cultured on Sabouraud’s maltose agar fortified with 1% yeast extract (SMAY) under continuous light at 25°C. After 14 days, the conidia were harvested and suspended in sterile water with 0.1% Tween 80 at a concentration of 1 × 108 conidia/mL. For subsequent optimisation of the culture conditions, the conidial suspension was inoculated into 250-mL sterile Erlenmeyer flasks containing 100 mL of liquid AM. Prior to autoclaving, the pH of the liquid AM was adjusted to 6.0 with HCl or NaOH. After 6 days following inoculation, the MS matured and samples were collected to measure the MS yields.
For the preparation of blastospore inoculum for 30-L bioreactor studies, the conidial suspension was inoculated into five 1-L baffled Erlenmeyer flasks containing 400 mL of liquid SDA medium (glucose, 4%; peptone, 0.25%; and yeast extract, 0.5%) and incubated at 25°C and 250 rpm on a rotary shaker incubator for 48 h.
Two-level FFD
A total of four variables were studied for MS production, namely, inoculum density, initial pH, shaker speed, and temperature. To determine the accuracy of the results of statistical experiments, we used 24−1 FFD leading to eight sets of experiments, performed in duplicate, to identify the important variables affecting MS yields. Each variable was represented at two levels, high and low, denoted by (1) and (−1) ().
Table 1. Different variables screened and their levels in the two-level FFD.
CCD
After identifying the important variables, 23 factorial CCD was adopted to optimise the levels of the variables. The full CCD with six star points (α = 1.682) and three replications of the centre point, leading to 20 sets of experiments, was used to optimise the production of M. rileyi MS. The MS yields were considered as the response, and the levels of each variable are given in . The results of CCD are usually used to fit a second-order polynomial equation because they represent the behaviour of such systems more appropriately than the first-order designs (Liu & Tzeng Citation1998; Souza et al. Citation1999; Prakash et al. Citation2008; Shi et al. Citation2009).
Table 2. Range, code values, and levels of independent variables in CCD.
For a three-variable system, the model equation is as follows:
where Y is the predicted response (MS yields); β0 is the intercept; β1, β2, and β3 are the linear effects; β11, β22, and β33 are the squared coefficients; and β12, β23, and β13 are the interaction coefficients.
Liquid submerged fermentation
For M. rileyi MS production in 30-L bioreactor, the total volume of the fermentation medium was maintained at 20 L and AM components at 20-fold concentrations were added. The pH of the medium was adjusted to 6.0 before autoclaving and was not controlled during fermentation. A total of 2 L of the blastospore inoculum was inoculated into the 30-L bioreactor. The fermentation temperature was 26°C and six bilayer Rushton impellors with a diameter of 1.5 cm were used for agitation.
To optimise the process of M. rileyi MS fermentation, we investigated the concentration of dissolved oxygen, which was determined periodically every 8 h. The aeration and agitation rates were found to affect the concentration of dissolved oxygen during submerged fermentation. Therefore, the agitation and aeration rates were varied, as shown in . The effects of these variations on MS production were evaluated by analysing the MS yields and biomass.
Figure 1. Trends in the hourly mean records of the dissolved oxygen level in the 30-L bioreactor in various trials. Trials 1–6 comprised treatments with various aeration and agitation rates (Trial 1: 100 rpm and 600 L/h; Trial 2: 100 rpm for the first 3 days and 150 rpm for the subsequent 3 days and 600 L/h; Trial 3: 150 rpm and 600 L/h; Trial 4: 150 rpm and 800 L/h; Trial 5:150 rpm for the first 3 days and 200 rpm for the subsequent 3 days and 800 L/h; and Trial 6: 150 rpm for the first 3 days and 200 rpm for the subsequent 3 days and 1200 L/h). Standard error bars indicate variation in measurements.
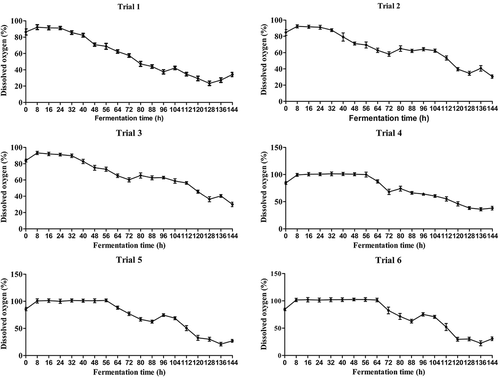
Assessment of MS production and statistical analysis
All the experiments were repeated three times. To analyse the biomass, 100 mL of fresh culture broth was weighed and the suspensions were subsequently centrifuged (5000 rpm). The resulting precipitate was weighed and dried to constant weight in an oven at 95°C. The biomass was estimated by determining the dry weight. The MS yields were measured as described by Jackson and Jaronski (Citation2009). One-way ANOVA was employed to analyse the variance, and the proportion of variance, explained by the polynomial models obtained, was given by the multiple coefficient of determination, R2. The statistical software SPSS 16.0 (SPSS, Chicago, IL, USA) and GraphPad Prism 5 were used for statistical analysis.
Results
Identification of important variables by using FFD
The corresponding FFD matrix was used for the screening of the four variables. presents the experimental design and results of 24−1 FFD experiments. The resulting effects (Exi) of the variables on the response, associated F values, and significant levels are shown in . A p value <0.1 for the shaker speed indicated that this variable was significant for the MS yields, while the other variables were less significant. An increase in the levels of shaker speed, inoculum density, and temperature had positive effects on the MS yields. However, an increase in the level of initial pH had a negative effect on the MS yields. The shaker speed, inoculum density, and temperature were selected based on the p values, and CCD was used for further optimisation.
Table 3. Two-level FFD matrix for the screening of important variables for MS production.
Table 4. Analysis of the two-level FFD for the screening of important variables.
Optimisation of the MS yields by using RSM
The CCD and corresponding experimental data are presented in . By applying ANOVA to the experimental data, the results of CCD were fitted by a polynomial quadratic equation. Although temperature was excluded, the linear, quadratic, and interaction coefficients of the variables examined were found to be significant at F2, 19 = 5.611, p = 0.013 < 0.05 and were retained in the reduced models. The equation that emerged was as follows:
Table 5. CCD plan matrix in coded value and the observed response.
where Y is the MS yields and X1 and X2 are the coded levels of shaker speed and inoculum density, respectively.
The fit of the model was expressed by the R2 value, which provided a measure of the extent to which the variability in the observed response values could be explained by the experimental variables and their interactions (Liu & Tzeng Citation1998; Vohra & Satyanarayana Citation2002). The corresponding ANOVA results are given in . However, the R2 value was 0.398 for MS production, indicating that the model was inadequate.
Table 6. Analysis of variance and model summary.
It had been pointed out that when a large amount of data are included in the analysis, a model with a significant lack of fit could still be used (Taragano & Pilosof Citation1999). Therefore, in the present study, we performed many experiments to predict data quality by evaluating the difference between the predicted data and measured results. Based on the variables shaker speed and inoculum density (), the results showed that there was a significant difference between the predicted data (4.55 × 104 MS/mL) and measured results (3.41 ± 0.7 × 104 MS/mL) for the treatment group subjected to minimal culture conditions (shaker speed: 200 rpm, inoculum density: 8%) (F1, 4 = 785.68, p < 0.001). Similarly, there was also a significant difference between the predicted data (5.56 × 104 MS/mL) and measured results (5.80 ± 0.03 × 104 MS/mL) for the treatment group subjected to moderate culture conditions (shaker speed: 250 rpm, inoculum density: 13%) (F1, 4 = 192.00, p < 0.001). However, the predicted data (6.07 × 104 MS/mL) and measured results (6.06 ± 0.12 × 104 MS/mL) for the treatment group subjected to maximal culture conditions (shaker speed: 300 rpm, inoculum density: 18%) showed no difference (F1, 4 = 0.009, p > 0.05). Furthermore, no difference (F1, 4 = 1.36, p > 0.05) between the obtained MS yields (5.98 ± 0.06 × 104 and 6.08 ± 0.11 × 104 MS/mL, respectively) following treatment with different inoculum densities (13% and 18%) under a shaker speed of 300 rpm was noted.
MS fermentation in 30-L bioreactor
To optimise the fermentation process for M. rileyi MS production, six trials were independently carried out (). Under the controlled conditions, the whole fermentation process was completed within 6 days. Overall, varied concentrations of dissolved oxygen were observed among the six trials during fermentation. After 24 h of fermentation with low aeration rate and low agitation rate, the concentration of dissolved oxygen decreased slowly at different rates (Trials 1–3) (). However, after 56 h of fermentation with higher aeration rate and higher agitation rate, the decrease in the concentration of dissolved oxygen was delayed and slow (Trials 4–6) (). Subsequently, the concentration of dissolved oxygen rapidly declined at different rates. Considering the development of MS, these results indicated that the growth of polar hyphae composed of the MS and the forming MS consume abundant dissolved oxygen (Song et al. Citation2013, Citation2016a; Jiang et al. Citation2014; Liu et al. Citation2014; Zhou et al. Citation2015).
The indices for MS production in the 30-L bioreactor are shown in and . The MS harvested from 20-L culture medium of Trials 1–6 were, respectively, weighed and the MS yields were calculated (). The M. rileyi MS yields varied with the aeration and agitation rates tested (F5, 12 = 46.15, p < 0.001): Trial 1, 1.52 ± 0.31 × 104 MS/mL; Trial 2, 2.15 ± 0.22 × 104 MS/mL; Trial 3, 2.21 ± 0.13 × 104 MS/mL; Trial 4, 3.14 ± 0.17 × 104 MS/mL; Trial 5, 3.65 ± 0.19 × 104 MS/mL; and Trial 6, 3.52 ± 0.25 × 104 MS/mL ()). Similarly, the biomass varied with the aeration and agitation rates examined (F5, 12 = 203.08, p < 0.001): Trial 1, 13.41 ± 0.61 g/L; Trial 2, 15.62 ± 0.41 g/L; Trial 3, 17.33 ± 0.64 g/L; Trial 4, 21.25 ± 0.81 g/L; Trial 5, 24.36 ± 0.42 g/L; and Trial 6, 23.35 ± 0.22 g/L ()). Furthermore, in Trials 4–6, especially in Trial 6, among the harvested MS, a larger number of matured MS with secondary mycelia growth were observed.
Figure 2. Production of M. rileyi MS and biomass in various trials. (a) The MS yields obtained in various trials. (b) The biomass obtained in various trials. Trials 1–6 comprised treatments with various aeration and agitation rates (Trial 1: 100 rpm and 600 L/h; Trial 2: 100 rpm for the first 3 days and 150 rpm for the subsequent 3 days and 600 L/h; Trial 3: 150 rpm and 600 L/h; Trial 4: 150 rpm and 800 L/h; Trial 5:150 rpm for the first 3 days and 200 rpm for the subsequent 3 days and 800 L/h; and Trial 6: 150 rpm for the first 3 days and 200 rpm for the subsequent 3 days and 1200 L/h). Standard error bars indicate variation in measurements. Means followed by different letters are significantly different (Duncan’s multiple range tests).
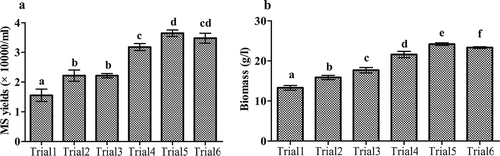
Discussion
In our preliminary experiments, we found that the biomass accumulation and MS yields were not positively correlated. High biomass accumulation with mass hyphal growth could be achieved with low MS yields (Yin et al. Citation2012). Hence, in the present study, we used the MS yields as an indicator of culture productivity in optimizing the process variables. The effect of initial pH of the liquid AM on M. rileyi MS production was studied with the two-level FFD ( and ). The results showed that an increase in the levels of initial pH had negative effects on MS production (). Likewise, in Sclerotinia sclerotiorum, neutral or alkaline pH suppressed MS development, while acidic pH conditions favoured MS development (Rollins & Dickman Citation2001; Chen et al. Citation2004). In the present study, further investigation of the effect of increasing levels of initial pH showed that an increase in the pH was not favourable for M. rileyi MS development (data not shown). Therefore, the pH of the medium was adjusted to 6.0 and was not altered in the following experiments. Our fermentation trials revealed that the pH of the culture medium regularly changed during M. rileyi MS formation. Further studies on the effects of changing pH on M. rileyi MS differentiation are currently being carried out in our laboratory.
The three-dimensional response surfaces were plotted by using a statistically significant model to understand the interaction of the three important variables and determine the optimum level of each variable required for effective MS production. Although temperature had been shown to significantly influence MS production in other plant pathogenic fungi (Jackson et al. Citation2011), it was an excluded variable in the RSM design to achieve optimum M. rileyi MS production because the optimised temperature range may not be suitable for M. rileyi growth. At 24–28°C, significantly higher biomass accumulation and MS production were observed, whereas at temperatures above/below this range, the growth of M. rileyi was greatly reduced (data not shown). Therefore, 26°C was set as the growth temperature for fermentation in the bioreactor.
Although the model was inadequate, it also required a significant lack of fit when a large amount of data were included in the analysis (Levin et al. Citation2008). Nevertheless, the p value for the regression was <0.05 (), suggesting that at least one of the terms in the regression equation was significantly correlated to the response variable (Tarocco et al. Citation2005). The predicted conditions for MS yields against those measured exhibited imperfect matching with a confidence interval of 95%. The difference in the predicted data and measured experimental results confirmed that the model may not have included all the appropriate functions of independent variables or the experimental range may be too large for a quadratic model (Tarocco et al. Citation2005). We individually verified the two variables shaker speed and inoculum density in the equation and found that they independently had a significant effect on MS production (p = 0.026 and 0.036, respectively). However, the independent variable (temperature) had no significant effect on MS production (p = 0.49). The interactive terms (AB, AC, BC) had no significant effect on MS production (p = 0.50). Although the inoculum density could affect MS production, the MS yields were not significantly different when the inoculum densities exceeded a concentration of 13%. Nevertheless, the upper limit of inoculum concentration was lower during bioreactor fermentation (data not shown). Therefore, the inoculum density was set at 10% in the following fermentation experiments. Furthermore, we found that the shaker speed played a major role in M. rileyi MS production by influencing the dissolved oxygen levels during fermentation. The reactive oxygen species, the by-products of oxygen metabolism, mediate MS formation and the results are verified in our research of the molecular mechanism of MS development (Song et al. Citation2013, Citation2016a; Jiang et al. Citation2014; Liu et al. Citation2014; Zhou et al. Citation2015). Lower shaker speed affected MS formation resulting in lower quantity and larger diameter of the MS produced.
In our preliminary experiments, we found no significant difference in the MS yields when using conidia and blastospore inoculum, respectively (Song et al. Citation2014b). This finding was important for accomplishing large-scale fermentative production of M. rileyi MS because blastospore inoculum could be easily realised on a large scale. Furthermore, we also determined the optimal culture period for hyphal inoculum, which was 2 days (Song et al. Citation2014b). Therefore, we carried out fermentation in a 30-L bioreactor by using hyphal inoculum for a period of 2 days. During the first 3 days of submerged fermentation, rapid reproduction and growth of hyphae for aggregation were observed, and subsequently, the aggregating hyphal initiated melanisation, MS formation, and MS maturation. These findings showed that large-scale fermentation for M. rileyi MS production was achieved and that the dissolved oxygen levels of the culture medium regularly changed during fermentation. Oxygen is the second most abundant element in the cells and the oxygen levels could affect the growth of M. rileyi. The abundant hyphal growth is accompanied by material and energy metabolism and oxygen consumption. Furthermore, the reactive oxygen species, the by-products of oxygen metabolism, mediate MS formation (Georgiou et al. Citation2006; Song et al. Citation2013; Jiang et al. Citation2014; Liu et al. Citation2014; Zhou et al. Citation2015). Therefore, for the optimisation of the submerged fermentation for M. rileyi MS production, dissolved oxygen level is an important monitor variable. However, the present study revealed that aeration and agitation rates were the major variables that affected the dissolved oxygen levels during fermentation, which, in turn, affected the MS yields ( and ). The low aeration and agitation rates resulted in low biomass accumulation and MS concentration. Therefore, for the optimum fermentation process for M. rileyi MS production, higher aeration and agitation rates should be employed. Based on the optimised process of fermentation of MS, we realise the stable submerged fermentation for M. rileyi MS production in 300-L bioreactor (data not shown).
The RSM was performed to optimise the process variables for M. rileyi MS production. One of the process variables, namely, shaker speed, obtained by the CCD was determined to significantly affect the MS yields. The optimal conditions for MS production were an initial pH of 5.5, growth temperature of 26°C, inoculum density of 10%, higher aeration rate (150 rpm in the initial 3 days and 200 rpm in the subsequent 3 days), and higher agitation rate of 800 L/h sterile air. To our knowledge, these findings on M. rileyi MS fermentation are novel, and the short duration of production cycle and lower cost of M. rileyi MS fermentation could promote its commercialisation.
Acknowledgements
This work was supported financially by the Agricultural Achievements Transformation Found under Grant [No.CSTC2014JCSF-NYCGZHB80011]; National Science Foundation of the People’s Republic of China under Grant [No. 31570073]; The Fundamental Research Funds for the Central Universities under Grant [No. 106112015CDJRC291209]; and China Postdoctoral Science Foundation under Grant [No. 2015M582514].
Disclosure statement
No potential conflict of interest was reported by the author(s).
Additional information
Funding
References
- Behle RW, Jackson MA, Flor-Weiler LB. 2013. Efficacy of a granular formulation containing Metarhizium brunneum F52 (Hypocreales: Clavicipitaceae) microsclerotia against nymphs of Ixodes scapularis (Acari: Ixoididae). J Econ Entomol. 106:57–63.
- Chen CB, Harel A, Gorovoits R, Yarden O, Dickman MB. 2004. MAPK regulation of sclerotial development in Sclerotinia sclerotiorum is linked with pH and cAMP sensing. MPMI. 17:404–413.
- Chen H, Yin YP, Li Y, Mahmud MS, Wang ZK. 2012. Identification and analysis of gene differentially expressed in the Spodoptera litura fat body in response to the biocontrol fungus, Nomuraea rileyi. Comp Biochem Physiol B Biochem Mol Biol. 163:203–210.
- Colotelo N. 1974. A scanning electron microscope study of developing sclerotia of Sclerotinia sclerotiorum. Can J Bot. 52:1127–1130.
- Faria MR, Wraight SP. 2007. Mycoinsecticides and Mycoacaricides: a comprehensive list with worldwide coverage and international classification of formulation types. BioControl. 43:237–256.
- Georgiou CD, Patsoukis N, Papapostolou I, Zervoudakis G. 2006. Sclerotial metamorphosis in filamentous fungi is induced by oxidative stress. Integr Comp Biol. 46:691–712.
- Jackson MA, Dunlap CA, Jaronski ST. 2010. Ecological considerations in producing and formulating fungal entomopathogens for use in insect biocontrol. BioControl. 55:129–145.
- Jackson MA, Dunlap CA, Shearer JF, Heilman MA, Palmquist DE. 2011. The impact of temperature on the production and fitness of microsclertia of the fungal bioherbicide Mycoleptodiscus terrestris. Biocontrol Sci Technol. 21:547–562.
- Jackson MA, Jaronski ST. 2009. Production of microsclerotia of the fungal entomopathogen Metarhizium anisopliae and their potential for use as a biocontrol agent for soil-inhabiting insects. Mycol Res. 113:842–850.
- Jiang S-S, Yin Y-P, Song Z-Y, Zhou G-L, Wang Z-K. 2014. RacA and Cdc42 regulate polarized growth and microsclerotium formation in the dimorphic fungus Nomuraea rileyi. Res Microbiol. 165:233–242.
- Kepler RM, Humber RA, Bischoff JF, Rehner SA. 2014. Clarification of generic and species boundaries for Metarhizium and related fungi through multigene phylogenetics. Mycologia. 106:811–829.
- Kobori NN, Mascarin GM, Jackson MA, Schisler DA. 2015. Liquid culture production of microsclerotia and submerged conidia by Trichoderma harzianum active against damping-off disease caused by Rhizoctonia solani. Fungal Biol. 119:179–190.
- Levin L, Herrmann C, Papinutti VL. 2008. Optimization of lignocellulolytic enzyme production by the white-rot fungus Trametes trogii in solid-state fermentation using response surface methodology. Biochem Eng J. 39:207–214.
- Li X, Ouyang J, Xu Y, Chen M, Song XY, Yong Q, Yu S. 2009. Optimization of culture conditions for production of yeast biomass using bamboo wastewater by response surface methodology. Bioresour Technol. 100:3613–3617.
- Liu BL, Tzeng YM. 1998. Optimization of growth medium for the production of spores from Bacillus thuringiensis using response surface methodology. Bioproc E. 18:413–418.
- Liu JJ, Yin YP, Song ZY, Li Y, Jiang SS, Shao CW, Wang ZK. 2014. NADH:flavin oxidoreductase/NADH oxidase and ROS regulate microsclerotium development in Nomuraea rileyi. World J Microb Biot. 30:1927–1935.
- López-Escudero FJ, Mwanza C, Blanco-López MA. 2006. Production of homogeneous and viable Verticillium dahliae microsclerotia effective for Verticillium wilt studies. Biotechnology. 5:421–428.
- Prakash GVSB, Padmaja V, Kiran RRS. 2008. Statistical optimization of process variables for the large-scale production of Metarhizium anisopliae conidiospores in solid-state fermentation. Bioresource Technol. 99:1530–1537.
- Rollins JA, Dickman MB. 2001. pH signaling in Sclerotinia sclerotiorum: identification of a pacC/RIMI homolog. Appl Environ Microb. 67:75–81.
- Shearer JF. 2007. Some observations concerning microsclerotia and spore production of Mycoleptodiscus terrestris in culture. Mycologia. 99:88–90.
- Shi YJ, Xu XQ, Zhu Y. 2009. Optimization of Verticillium lecanii spore production in solid-state fermentation on sugarcane bagasse. Appl Microbiol Biot. 82:921–927.
- Song ZY, Jiang W, Yin YP, Wang ZK. 2016a. Polarity proteins Mrcdc24 and Mrbem1 required for hypha growth and microsclerotia formation in Metarhizium rileyi. Biocontrol Sci Technol. 26:733–745.
- Song ZY, Shen L, Zhong Q, Yin YP, Wang ZK. 2016b. Liquid culture production of microsclerotia of Purpureocillium lilacinum for use as bionematicide. Nematology. 18:719–726.
- Song ZY, Yin YP, Jiang SS, Liu JJ, Chen H, Wang ZK. 2013. Comparative transcriptome analysis of microsclerotia development in Nomuraea rileyi. BMC Genom. 14:411.
- Song ZY, Yin YP, Jiang SS, Liu JJ, Wang ZK. 2014a. Optimization of culture medium for microsclerotia production by Nomuraea rileyi and analysis of their viability for use as a mycoinsecticide. BioControl. 59:597–605.
- Song ZY, Yin YP, Jiang W, Zhou GL, Tan WY, Wang ZK. 2014b. One step production of Nomuraea rileyi (Ascomycetes: Hypocreales) microsclerotia in submerged fermentation. Chin J Biol Control. 30:660–664. Chinese.
- Souza M-D-O, Roberto I, Milagres A. 1999. Solid-state fermentation for xylanase production by Thermoascus aurantiacus using response surface methodolody. Appl Microbiol Biot. 52:768–772.
- Taragano VM, Pilosof AMR. 1999. Application of Doehlert designs for water activity, pH, and fermentation time optimization for Aspergillus niger pectinolytic activities production in solid-state and submerged fermentation. Enzyme Microb Technol. 25:411–419.
- Tarocco F, Lecuona RE, Couto AS, Arcas JA. 2005. Optimization of erythritol and glycerol accumulation in conidia of Beauveria bassiana by solid-state fermentation, using response surface methodology. Appl Microbiol Biot. 68:481–488.
- Vohra A, Satyanarayana T. 2002. Statistical optimization of the medium components by response surface methodology to enhance phytase production by Pichia anomala. Process Biochem. 37:999–1004.
- Wang HH, Wang JL, Li YP, Liu X, Wen JZ, Lei ZR. 2011. Liquid culturing of microsclerotia of Beauveria bassiana, an entomopathgeonic fungus to control western flower thrip, Frankliniella occidentalis. Chin J Appl Entomol. 48:588–595. Chinese.
- Yang X, Zhang DM, Li ZZ. 2014. Induction and culture condition optimization of Beauveria brongniartii for microsclerotia production. Mycosystema. 33:827–837. Chinese.
- Yin YP, Huang S, Song ZY, Wang ZK. 2012. Microsclerotia artificial inductions of Nomuraea rileyi CQNr01. Sci Agric Sin. 45:4801–4807. Chinese.
- Zhou GL, Song ZY, Yin YP, Jiang W, Wang ZK. 2015. Involvement of an alternative oxidase in the regulation of hyphal growth and microsclerotial formation in Nomuraea rileyi CQNr01. World J Microb Biot. 31:1343–1352.