ABSTRACT
Filamentous fungi grow by adding cell wall and membrane exclusively at the apex of tubular structures called hyphae. Growth was previously believed to occur only through exocytosis at the Spitzenkörper, an organised body of secretory macro- and microvesicles found only in growing hyphae. More recent work has indicated that an area deemed the sub-apical collar is enriched for endocytosis and is also required for hyphal growth. It is now generally believed that polarity of filamentous fungi is achieved through the balancing of the processes of endocytosis and exocytosis at these two areas. This review is an update on the current progress and understanding surrounding the occurrence of endocytosis and its spatial regulation as they pertain to growth and pathogenicity in filamentous fungi.
1. Overview
Filamentous fungi (FF) uniquely produce tubular cells called hyphae in a polarised manner. This occurs through the synthesis and addition of new cell wall and membrane exclusively at the apex (Steinberg et al. Citation2017; Riquelme et al. Citation2018). The paradigm of hyphal growth has previously been to focus on exocytosis through the Spitzenkörper (SPK), an organised body of secretory macro- and microvesicles which is found only in growing fungal hyphae (Bartnicki-Garcia et al. Citation1989, Citation1995; Reynaga-Peña et al. Citation1997; Virag and Harris Citation2006). An early hypothesis proposed that the establishment of polarity is a stochastic process that autonomously transpires without the need for membrane markers (Harris Citation2006). However, the evidence provided since then indicates that the series of events is much less random. More recent work has forced reconsideration due to the discovery of an area that is enriched for endocytosis. This work has indicated that the area distal to the SPK, deemed the sub-apical collar, is also required for hyphal growth (Taheri-Talesh et al. Citation2008; Upadhyay and Shaw Citation2008). It is now generally understood that polarity of FF is achieved through the balancing of the processes of endocytosis and exocytosis.
Endocytosis in yeast has been thoroughly characterised (Goode et al. Citation2015); however, comparatively little is known about endocytosis in FF (). In particular, little attention has been paid to proteins whose localisations and functions border between endocytosis and exocytosis in the growing hyphal apex. While a variety of process are conserved from yeasts to FF, not all components are identical, and some must be compensated for in order to afford the multiple axes of polarity and increased extension rates observed in FF. The most well-studied single-cell yeasts, Saccharomyces cerevisiae and Schizosaccharomyces pombe, also display polarised growth. However, this budding or fission growth is limited by the cell cycle, while hyphae of FF are able to continuously extend in an unrestricted unidirectional manner (Takeshita Citation2016). Evidence that endocytosis itself varies between yeasts and FF has also been provided through studies on clathrin localisation. While clathrin is observed both internally as well as at the cortex of S. cerevisiae cells, a corresponding localisation to the endocytic collar is not observed in A. nidulans (Newpher et al. Citation2005; Schultzhaus et al. Citation2017).
Figure 1. The endocytic process at the sub-apical collar of FF
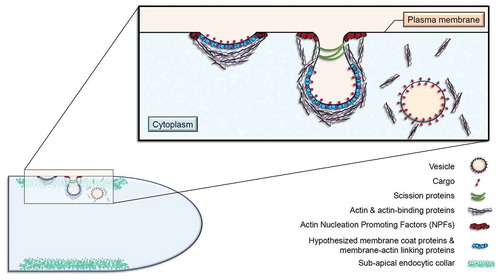
Several reviews have summarised the various aspects of polarised growth and hyphal morphogenesis in FF over the past decade (Peñalva Citation2010; Harris Citation2011; Shaw et al. Citation2011; Steinberg Citation2014; Takeshita Citation2016; Steinberg et al. Citation2017; Riquelme et al. Citation2018; Verdín et al. Citation2019). For a more comprehensive review on the process of endocytosis itself, see (Steinberg Citation2014; Steinberg et al. Citation2017). The priority of this review is to update the current progress and understanding surrounding the occurrence of endocytosis as it pertains to growth in FF. Evidence to support the necessity of endocytosis for the maintenance of normal hyphal extension and shape in FF is provided here, as well as various theories behind its spatial regulation. Our understanding of this process as well as hyphal growth is crucial, as each one is required for the disease progression of plants, animals, and humans (Köhler et al. Citation2014; Zeilinger et al. Citation2016; Riquelme et al. Citation2018).
2. Does endocytosis occur in filamentous fungi?
It has been demonstrated that many proteins associated with membrane turnover (such as adapter proteins, cargoes, endocytic machinery, etc.) localise to three different apical regions of growing hyphae: the SPK, the apical crescent, and the sub-apical collar () (Upadhyay and Shaw Citation2008; Araujo-Bazan et al. Citation2008; Sudbery Citation2011). Since endocytosis is a vital life process in FF, it is imperative that it is evaluated based on studies which implement live cell imaging. Fluorescently tagging proteins to evaluate localisation patterns was a rather arduous process at the turn of the century, and it still can be in many organisms, particularly those without sequenced or publicly available genomes. Alternatively, select vital stains or dyes that had been created for use in live cell imaging were instrumental in allowing more comprehensive studies at the time. It was through the use of these dyes that endocytosis was first discovered in FF (Hoffmann and Mendgen Citation1998). The former belief behind the mechanisms of hyphal growth was based solely on the notion of exocytosis, which predominantly occurs through the SPK. However, FM4-64 staining, combined with the discovery of the sub-apical collar and genomic data, forced re-evaluation of the commonly accepted notion of the vesicle supply centre.
Figure 2. Three apical areas to which proteins associated with membrane turnover localise
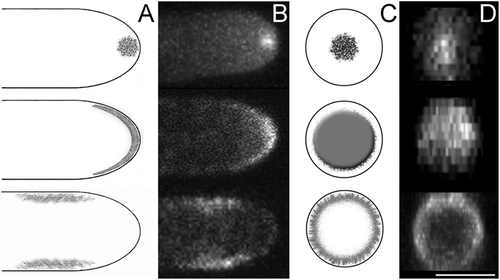
2.1. History of markers for endocytosis
Several membrane-selective dyes and markers have previously been utilised in order to investigate the countless processes and pathways occurring within living fungi. For example, Lucifer Yellow, TMA-DPH, FITC-dextran, FM1-43, and FM4-64 were all utilised in an attempt to visualise internalisation and subsequent trafficking (Fischer-Parton et al. Citation2000; Peñalva Citation2005). Of the various dyes used, the lipophilic marker dye FM4-64 was particularly instrumental in revolutionising the current understanding of endocytosis in FF.
FM4-64 was initially shown to be endocytosed at the plasma membrane in S. cerevisiae (Vida and Emr Citation1995), and it subsequently became a vital stain which was, and still is, considered a marker for endocytosis in the yeasts. This discovery was soon-after followed by the application of FM4-64 to FF. In Uromyces fabae, FM4-64 uptake was observed within seconds of its application, and the most intense localisation was to the membrane at the hyphal apex, making it the first evidence for endocytosis in a fungal germ tube (Hoffmann and Mendgen Citation1998). This sparked an interest in using it to study FF, and shortly thereafter, endocytosis was also demonstrated in ungerminated and germinated spores of the FF M. grisea (Atkinson et al. Citation2002). In addition to labelling the apical hyphal membrane, FM4-64 uptake was also observed in the SPK (Fischer-Parton et al. Citation2000). This evidence was rather controversial, particularly because the SPK was believed to be involved only with apical secretion through Golgi-derived exocytic vesicles (Reynaga-Peña et al. Citation1997). These new data, however, indicated that the SPK functions additionally in recycling endocytic vesicles produced at the hyphal tip (Upadhyay and Shaw Citation2008). Furthermore, it suggested that endocytosis is also vital for hyphal growth, which has since been corroborated by numerous studies.
In the years following this discovery, FM4-64 was applied to a multitude of filamentous species (Fischer-Parton et al. Citation2000; Atkinson et al. Citation2002). While the use of FM4-64 in FF was still relatively limited in the early 2000s (Peñalva Citation2005), its use is now considered standard practice for experiments involving endocytic or apically localised proteins in FF.
2.2. The discovery of the sub-apical collar
The evidence provided from various experiments involving FM4-64 made it clear that the internalisation of membranes also contributes to apical growth. This was subsequently reinforced by the discovery of the sub-apical collar in A. nidulans (Taheri-Talesh et al. Citation2008; Upadhyay and Shaw Citation2008; Araujo-Bazan et al. Citation2008), which is an area enriched for endocytosis that lies 1–5 µm distal to the SPK. The location of the collar in the sub-apex is maintained over time during hyphal growth, and dissipates with the cessation of growth, similar to the maintenance of the SPK. Prior to this discovery, it was primarily believed that fungal growth occurred due to secretion through the SPK.
2.3. Theories regarding the need for endocytosis in the collar
It was previously shown that endocytosis in S. pombe is spatially associated with the actin cytoskeleton during polarised growth (Gachet and Hyams Citation2005). Therefore, it does not come as a surprise that the “zone of endocytosis” in FF also happens to be where actin is predominantly and intensely localised (Upadhyay and Shaw Citation2008; Delgado-Alvarez et al. Citation2010; Schultzhaus et al. Citation2016). However, it is still unclear why endocytosis is enriched in the sub-apical collar. At this time, several theories have been proposed to elucidate the reasoning behind the presence and spatial regulation of the collar in FF. The original hypothesis for tip growth proposed that the SPK was comprised of Golgi-derived exocytic vesicles which were destined to fuse with the apex. However, this vesicle supply centre concept needed to be expanded to account for the function of endocytosis at the apex and sub-apical collar once it was identified in FF (Gierz and Bartnicki-Garcia Citation2001).
One explanation is provided by the apical recycling model, which indicates endocytosis at the sub-apical collar maintains the polarisation of apically localised membrane proteins (Shaw et al. Citation2011; Hernandez-Gonzalez et al. Citation2018). These membrane proteins would in turn be marking areas of polarisation at the apices of hyphae, and they would be displaced along the membrane during growth. These proteins would therefore need to then be removed by the sub-apical collar in order for the hyphoid shape to be maintained.
Recently, another hypothesis suggested that endocytosis in the collar acts as a means for removing excess secreted plasma membrane, which has recently been quantified in Neurospora crassa (Riquelme et al. Citation2018; Bartnicki-Garcia et al. Citation2018). Based on the data provided by Bartnicki-Garcia et al. in 2018, an estimated 9800 vesicles per minute are needed in order to maintain hyphal growth and cell wall expansion. This is likely on the higher end compared to many other FF due to the large size and fast growth rate of N. crassa hyphae. Nonetheless, the number of vesicles discharged is estimated, on the lower end, to be around 59,000 vesicles per minute (Bartnicki-Garcia et al. Citation2018). Some of these proteins must therefore be recycled at the sub-apical collar in turn. In this model, endocytosis acts primarily to remove the excess membrane provided through secretion, and does so efficiently due to its close proximity to the apex. While the idea of removing excess plasma membrane was proposed as an explanation for reducing the sheer quantity of secreted membrane, it also parallels the apical recycling model.
Another comparable hypothesis is that the apex is lined with proteins which localise to the plasma membrane and are deemed “cell-end markers” or “polarity markers.” Cell-end markers, such as A. nidulans KipA, TeaA, and TeaR, have been shown to be involved in localising growth machinery to hyphal tips (Konzack et al. Citation2005; Takeshita et al. Citation2013). These polarised proteins are cytoskeleton-dependent, and subsequently direct cell polarity. Secretion at the hyphal apex dilutes the accumulation of polarity markers, creating a cyclical process of assembling and disseminating cell-end markers in a pulse-like pattern (Takeshita Citation2016). This proposed circulation of proteins also bares striking similarities to the apical recycling model. These are not mutually exclusive theories, as each one was proposed through the perspective of a different area at the hyphal apex.
3. Experimental evidence
Regardless of the reasoning for the location and maintenance of the sub-apical collar in FF, there is now little doubt about its existence. Countless experiments have shown that a sub-apical area enriched for endocytosis arises during growth. Further studies have provided evidence for the recycling of various polarised proteins. For example, the essential A. nidulans chitin-synthase ChsB is polarised through indirect endocytic recycling, which involves both exocytosis at the apex and endocytosis at the sub-apical collar. ChsB is subsequently trafficked to the TGN cisternae and then ultimately re-delivered to the apex (Hernandez-Gonzalez et al. Citation2018). Additional evidence is discussed in more detail below.
3.1. Fimbrin mutant in Aspergillus
The processes of endocytosis and exocytosis are synchronised and regularly maintain a spatial and temporal relationship during growth. Evidence for this relationship has been provided in the form of multiple mutational studies. When a gene involved in one of the two processes is disrupted, so too is normal hyphal elongation and the uniquely notable hyphoid shape. For example, when the A. nidulans F-actin cross-linking gene and endocytic marker fimbrin (fimA) was disrupted, cells displayed abnormal isotropic swelling and an inability to otherwise maintain polarity. Furthermore, FimA and the secretory vesicle marker GsaA were fused with mCherry and localised using the conditional niiA promoter in A. nidulans. Aberrant growth was evident when endocytosis was disrupted, and neither the SPK nor the standard zone of endocytosis at the collar could be seen (Upadhyay and Shaw Citation2008). This evidence highlighted the partnership of these two associated processes, and the aforementioned apical recycling model was proposed as a result of these findings.
3.2. NPFxD motif
One of only two endocytic signal sequences discovered in yeast to date is the NPFX(1,2)D motif (and the similar but less effective variations, DPFxD, NPF, or DPF), which was shown to mark proteins for endocytosis and is required for polarity in yeast (Tan et al. Citation1996; Howard et al. Citation2002; Piao et al. Citation2007). This motif is found in multiple membrane proteins, and is recognised by the adapter protein Sla1p through an interaction with the SHD1 (Sla1p Homology Domain) region (Costa et al. Citation2005). Mutating one or more of the initial three residues in this motif to Alanine (A) has been shown to halt endocytic uptake of the protein (Tan et al. Citation1996). Studies have shown that the contribution of the motif to the function and localisation of specific endocytic cargo proteins (Liu et al. Citation2007; Schultzhaus et al. Citation2015). For example, yeast proteins Drs2p and Dnf1p each contain at least one NPFxD motif. In wild-type (WT) cells, Drs2p localises to the trans-Golgi network (TGN) and Dnf1p localises both to the plasma membrane and to endo-membranes. When the NPFxD-dependent endocytic mechanisms were inactivated, the localisation of each protein was altered (Liu et al. Citation2007). To further test the motif, as well as the apical recycling model, the orthologous NPFxD-containing protein DnfA in A. nidulans was also mutated. DnfA::GFP displayed polarised localisation to the apical plasma membrane and the SPK; however, this polarised localisation was also lost upon mutating the motif to AAFxD (Schultzhaus et al. Citation2015). The re-localisation of DnfA::GFP upon disruption of the motif corroborates the notion that apical recycling maintains the polarisation of the protein. These studies also reinforce the proposition that the peptide motif is essential for proper endocytic uptake in a variety of cargo proteins.
3.3. The requirement of endocytosis for pathogenicity
Recent studies have also provided evidence that the endocytic pathway is important for pathogenicity in many plant pathogens. For example, a multitude of proteins in Magnaporthe oryzae have been shown to be involved in both endocytosis and pathogenicity. It was recently found that M. oryzae protein MoEnd3 is critical for development and virulence due to its role in mediating receptor endocytosis (Li et al. Citation2017b). Additionally, MoRab5A and MoRab5B, both of which are Rab5 homologs in M. oryzae, are critical for endocytosis and plant pathogenesis, among other things (Yang et al. Citation2017). Other studies have linked MoArk1, MoAbp1, MoAct1, and MoCAP proteins (among others) to endocytosis, growth, the actin cytoskeleton, and therefore, pathogenicity (Li et al. Citation2017a, Citation2019). Investigation of Ustilago maydis protein Yup1 similarly demonstrated that endocytosis is essential for the beginning stages of pathogenesis (Fuchs et al. Citation2006). This requirement has been further demonstrated in multiple species of Fusarium as well. For example, Fusarium graminearum proteins FgMon1 and FgRab7 have been shown to play critical roles in modulating vesicle trafficking, endocytosis, and plant infection (Li et al. Citation2015). Deletions of FgSnc1, FgSnx41, or FgSnx4 also affect endosomal sorting, polarised growth, and ultimately pathogenicity (Zheng et al. Citation2018).
3.4. Genome-wide studies & bioinformatic analysis
An invaluable tool made widely available to fungal biologists in recent years is bioinformatic analysis. Combined with genome-wide studies, these analyses allow for evolutionary relationships and dynamics to be revealed and reconsidered. Examples of this include the recently elucidated origin of fungal hyphae and adaptation mechanisms in dimorphic fungi (Muñoz et al. Citation2018; Kiss et al. Citation2019). Trends within and between organisms have been made visible with the availability of databases of information, and the field has been able to utilise this information to expand the current understanding of signalling networks and orthologous relationships. Bioinformatic analysis of fungal genomes has also provided overwhelming evidence that endocytosis and endocytic recycling are important life processes for FF (Baker Citation2006; Jorgensen et al. Citation2009, Citation2010; De Souza et al. Citation2013; Ramsubramaniam et al. Citation2014). For example, sequencing and annotating the A. nidulans genome revealed that there were many more genes implicated in vegetative growth and morphogenesis than in yeast (Harris et al. Citation2009). In addition, genomic studies using expressed sequence tag (EST) analyses and whole-genome sequencing in A. oryzae have elucidated genes involved in growth and secretion (Abe et al. Citation2006). In A. niger, a transcriptomic fingerprint was also developed for apical branching and hyphal elongation (Meyer et al. Citation2009).
4. Conclusions & outstanding questions
Both endocytosis and the spatial coupling of endocytosis with secretion have now been shown to be of great importance for hyphal extension in several FF. In the last two decades, our understanding of how FF grow has been entirely revolutionised by the discovery of endocytosis and the areas in which it occurs. Since then, many questions have been answered, and even more have been posed.
Single particle tracking, fluorescence recovery after photobleaching (FRAP), and various other advanced microscopy techniques have provided a means for scientists to answer pressing questions about endocytosis. For example, data on the quantification of endocytosis was previously very limited (Thilo Citation1985); however, Bartnicki et. al were able to implement a comprehensive FRAP protocol in 2018 and better estimate the number of vesicles that are endocytosed in the sub-apical collars of N. crassa hyphae. This impressive milestone serves as a baseline on which others in the field can build. This also answered multiple questions posed by Peñalva et. al in 2010 regarding whether or not the apex contains an excess of membrane for which endocytosis compensates. It was found that, while endocytosis does partially compensate for the excess membrane, there is still far more membrane produced at the apex than endocytosis can account for. This result was somewhat unexpected and suggests that perhaps these secreted proteins function in additional ways that we have yet to identify. It is possible that there are additional routes of endocytosis that are not adequately measured by this method, or that the overwhelming amounts of molecules which are recycled cannot be captured in their entirety by FRAP data alone.
The understanding of the secretory network at the hyphal tip has progressed significantly in recent years. However, comparably little has focused on the process of internalisation, which has been shown to be equally necessary for hyphal growth and polarity, and is the hallmark characteristic of FF. Now that it is generally accepted that endocytosis does occur in FF, priority should be given to identifying the proteins that act as cargo for endocytosis, or that participate in apical recycling. A reverse genetics approach to identify genes which encode the endocytosis peptide motif NPFxD is currently underway. In addition, whole genome sequencing provides an opportunity to identify genes from mutant collections created in the classical genetics era that are involved in endocytosis.
It was also recently suggested that “endocytic components are underexplored targets for engineering fungal cell factories” (Cairns et al. Citation2019). Many species of fungi are industrially utilised and harvested in mass for useful molecules such as enzymes, proteins, secondary metabolites, and organic acids (Cairns et al. Citation2018, Citation2019). Since many enzymes and molecules are secreted at the apex, the amount produced tends to initially correspond to the number of hyphal tips. However, an increased number of hyphal tips does not always correlate to an elevation in protein concentration. A recent explanation for this is that unconventional protein secretion pathways are activated during the fermentation process, meaning secretion is no longer predominantly occurring at the apex, but instead through the cell membrane or septa (Veiter et al. Citation2018). Little work has been done to investigate the role of endocytosis in the fermentation process, even though we know that the processes of exocytosis and endocytosis are tightly coupled. It is entirely possible that the decrease in overall secretion could instead be due to an increase in endocytosis, or an alteration of the specific cargo which is endocytosed. The preference for recycled products could fluctuate with various environmental conditions, such as diauxic shifts, for example. If this is elucidated, the fermentation environment could potentially be manipulated or optimised based on an ideal organismal ratio, or by first increasing endocytosis.
Furthermore, the dynamic localisation of GFP-fusion proteins must continue to be tested. Researchers should take advantage of organisms that cooperate in large-scale studies so that curated databases such as FungiDB can consolidate and distribute these data (Stajich et al. Citation2012). Not only do we need to characterise and localise multiple proteins, but we also need to investigate the relationships among them and focus on those whose roles vary between yeast-like and hyphal growth. Since many pathogenic fungi are dimorphic, priority should also be given to signalling networks and proteins that do not follow the typical yeast model of life and growth, or that are specific to FF altogether.
As the availability and affordability of advanced technology increases, so too will the discoveries in this field. The development of advanced microscopic tools and methods for bioinformatic analysis will continue to significantly contribute to our current knowledge on filamentous fungal growth, as well as its interconnectedness with the environment.
Acknowledgements
BC received an Excellence Fellowship from the College of Agriculture and Life Sciences at Texas A&M University and a Graduate Student Fellowship from the Mycological Society of America. The Shaw lab was also supported in part by USDA NIFA Project #TEX0-1-9018.
Disclosure statement
No potential conflict of interest was reported by the authors.
Additional information
Funding
References
- Abe K, Gomi K, Hasegawa F, Machida M. Impact of Aspergillus oryzae genomics on industrial production of metabolites. Mycopathologia. 2006;162(3):143–153. doi:10.1007/s11046-006-0049-2.
- Araujo-Bazan L, Peñalva MA, Espeso EA. Preferential localization of the endocytic internalization machinery to hyphal tips underlies polarization of the actin cytoskeleton in Aspergillus nidulans. Mol Microbiol. 2008;67(4):891–905. doi:10.1111/mmi.2008.67.issue-4.
- Atkinson HA, Daniels A, Read ND. Live-cell imaging of endocytosis during conidial germination in the rice blast fungus Magnaporthe grisea. Fungal Genet Biol. 2002;37(3):233–244. doi:10.1016/S1087-1845(02)00535-2.
- Baker SE. Aspergillus niger genomics: past, present and into the future. Medical Mycology. 2006;44:S17–S21. doi:10.1080/13693780600921037.
- Bartnicki-Garcia S, Bartnicki DD, Gierz G, Lopez-Franco R, Bracker CE. Evidence that Spitzenkörper behavior determines the shape of a fungal hypha: a test of the hyphoid model. Exp Mycol. 1995;19(2):153–159. doi:10.1006/emyc.1995.1017.
- Bartnicki-Garcia S, Garduno-Rosales M, Delgado-Alvarez DL, Mourino-Perez RR. Experimental measurement of endocytosis in fungal hyphae. Fungal Genet Biol. 2018;118:32–36. doi:10.1016/j.fgb.2018.07.001.
- Bartnicki-Garcia S, Hergert F, Gierz G. Computer simulation of fungal morphogenesis and the mathematical basis for hyphal (tip) growth. Protoplasma. 1989;153:46–57. doi:10.1007/BF01322464.
- Cairns TC, Nai C, Meyer V. How a fungus shapes biotechnology: 100 years of Aspergillus niger research. Fungal Biol Biotechnol. 2018;5(13):14. doi:10.1186/s40694-018-0054-5.
- Cairns TC, Zheng X, Zheng P, Sun J, Meyer V. Moulding the mould: understanding and reprogramming filamentous fungal growth and morphogenesis for next generation cell factories. Biotechnol Biofuels. 2019;12:77. doi:10.1186/s13068-019-1400-4.
- Costa R, Warren DT, Ayscough KR. Lsb5p interacts with actin regulators Sla1p and Las17p, ubiquitin and Arf3p to couple actin dynamics to membrane trafficking processes. Biochem J. 2005;387:649–658. doi:10.1042/BJ20041729.
- De Souza CP, Hashmi SB, Osmani AH, Andrews P, Ringelberg CS, Dunlap JC, Osmani SA. Functional analysis of the Aspergillus nidulans kinome. PLoS One. 2013;8(3):e58008–e58008. doi:10.1371/journal.pone.0058008.
- Delgado-Alvarez DL, Callejas-Negrete OA, Gomez N, Freitag M, Roberson RW, Smith LG, Mourino-Perez RR. Visualization of F-actin localization and dynamics with live cell markers in Neurospora crassa. Fungal Genet Biol. 2010;47(7):573–586. doi:10.1016/j.fgb.2010.03.004.
- Fischer-Parton S, Parton RM, Hickey PC, Dijksterhuis J, Atkinson HA, Read ND. Confocal microscopy of FM4-64 as a tool for analysing endocytosis and vesicle trafficking in living fungal hyphae. J Microsc. 2000;198(3):246–259. doi:10.1046/j.1365-2818.2000.00708.x.
- Fuchs U, Hause G, Schuchardt I, Steinberg G. Endocytosis is essential for pathogenic development in the corn smut fungus Ustilago maydis. Plant Cell. 2006;18(8):2066–2081. doi:10.1105/tpc.105.039388.
- Gachet Y, Hyams JS. Endocytosis in fission yeast is spatially associated with the actin cytoskeleton during polarised cell growth and cytokinesis. J Cell Sci. 2005;118(18):4231–4242. doi:10.1242/jcs.02530.
- Gierz G, Bartnicki-Garcia S. A three-dimensional model of fungal morphogenesis based on the vesicle supply center concept. J Theor Biol. 2001;208(2):151–164. doi:10.1006/jtbi.2000.2209.
- Goode BL, Eskin JA, Wendland B. Actin and endocytosis in budding yeast. Genetics. 2015;199(2):315–358. doi:10.1534/genetics.112.145540.
- Harris SD. Cell polarity in filamentous fungi: shaping the mold. Int Rev Cytol Elsevier Inc. 2006;251:41–77.
- Harris SD. Hyphal morphogenesis: an evolutionary perspective. Fungal Biol. 2011;115(6):475–484. doi:10.1016/j.funbio.2011.02.002.
- Harris SD, Turner G, Meyer V, Espeso EA, Specht T, Takeshita N, Helmstedt K. Morphology and development in Aspergillus nidulans: A complex puzzle. Fungal Genet Biol. 2009;46(1):S82–S92. doi:10.1016/j.fgb.2008.07.023.
- Hernandez-Gonzalez M, Bravo-Plaza I, Pinar M, de Los Rios V, Arst HN Jr., Peñalva MA. Endocytic recycling via the TGN underlies the polarized hyphal mode of life. PLoS Genet. 2018;14(4):e1007291. doi:10.1371/journal.pgen.1007291.
- Hoffmann J, Mendgen K. Endocytosis and membrane turnover in the germ tube of Uromyces fabae. Fungal Genet Biol. 1998;24(1–2):77–85. doi:10.1006/fgbi.1998.1059.
- Howard JP, Hutton JL, Olson JM, Payne GS. Sla1p serves as the targeting signal recognition factor for NPFX(1,2)D-mediated endocytosis. J Cell Biol. 2002;157(2):315–326. doi:10.1083/jcb.200110027.
- Jorgensen TR, Goosen T, Hondel CA, Ram AF, Iversen JJ. Transcriptomic comparison of Aspergillus niger growing on two different sugars reveals coordinated regulation of the secretory pathway. BMC Genomics. 2009;10:44. doi:10.1186/1471-2164-10-44.
- Jorgensen TR, Nitsche BM, Lamers GE, Arentshorst M, van den Hondel CA, Ram AF. Transcriptomic insights into the physiology of Aspergillus niger approaching a specific growth rate of zero. Appl Environ Microbiol. 2010;76(16):5344–5355. doi:10.1128/AEM.00450-10.
- Kiss E, Hegedüs B, Virágh M, Varga T, Merényi Z, Kószó T, Bálint B, Prasanna AN, Krizsán K, Kocsubé S, et al. Comparative genomics reveals the origin of fungal hyphae and multicellularity. Nat Commun. 2019;10(1):4080. doi:10.1038/s41467-019-12085-w.
- Köhler JR, Casadevall A, Perfect J. The spectrum of fungi that infects humans. Cold Spring Harb Perspect Med. 2014;5(1):a019273. doi:10.1101/cshperspect.a019273.
- Konzack S, Rischitor PE, Enke C, Fischer R. The role of the kinesin motor KipA in microtubule organization and polarized growth of Aspergillus nidulans. Mol Biol Cell. 2005;16(2):497–506. doi:10.1091/mbc.e04-02-0083.
- Li L, Chen X, Zhang S, Yang J, Chen D, Liu M, Zhang H, Zheng X, Wang P, Peng Y, et al. MoCAP proteins regulated by MoArk1-mediated phosphorylation coordinate endocytosis and actin dynamics to govern development and virulence of Magnaporthe oryzae. PLoS Genet. 2017a;13(5):e1006814. doi:10.1371/journal.pgen.1006814.
- Li L, Zhang S, Liu X, Yu R, Li X, Liu M, Zhang H, Zheng X, Wang P, Zhang Z. Magnaporthe oryzae Abp1, a MoArk1 kinase-interacting actin binding protein, links actin cytoskeleton regulation to growth, endocytosis, and pathogenesis. Molecular Plant-Microbe Interactions. 2019;32(4):437–451. doi:10.1094/MPMI-10-18-0281-R.
- Li X, Gao C, Li L, Liu M, Yin Z, Zhang H, Zheng X, Wang P, Zhang Z. MoEnd3 regulates appressorium formation and virulence through mediating endocytosis in rice blast fungus Magnaporthe oryzae. PLoS Pathog. 2017b;13(6):e1006449. doi:10.1371/journal.ppat.1006449.
- Li Y, Li B, Liu L, Chen H, Zhang H, Zheng X, Zhang Z. FgMon1, a guanine nucleotide exchange factor of FgRab7, is important for vacuole fusion, autophagy and plant infection in Fusarium graminearum. Sci Rep. 2015;5:18101. doi:10.1038/srep18101.
- Liu K, Hua Z, Nepute JA, Graham TR. Yeast P4-ATPases Drs2p and Dnf1p are essential cargos of the NPFXD/Sla1p endocytic pathway. Mol Biol Cell. 2007;18(2):487–500. doi:10.1091/mbc.e06-07-0592.
- Lu R, Drubin DG, Sun Y. Clathrin-mediated endocytosis in budding yeast at a glance. J Cell Sci. 2016;129(8):1531–1536. doi:10.1242/jcs.182303.
- Meyer V, Arentshorst M, Flitter SJ, Nitsche BM, Kwon MJ, Reynaga-Pena CG, Bartnicki-Garcia S, van den Hondel CA, Ram AF. Reconstruction of signaling networks regulating fungal morphogenesis by transcriptomics. Eukaryot Cell. 2009;8(11):1677–1691. doi:10.1128/EC.00050-09.
- Muñoz JF, McEwen JG, Clay OK, Cuomo CA. Genome analysis reveals evolutionary mechanisms of adaptation in systemic dimorphic fungi. Sci Rep. 2018;8(1):4473. doi:10.1038/s41598-018-22816-6.
- Newpher TM, Smith RP, Lemmon V, Lemmon SK. In vivo dynamics of clathrin and Its adaptor-dependent recruitment to the actin-based endocytic machinery in yeast. Dev Cell. 2005;9(1):87–98. doi:10.1016/j.devcel.2005.04.014.
- Peñalva MA. Tracing the endocytic pathway of Aspergillus nidulans with FM4-64. Fungal Genet Biol. 2005;42(12):963–975. doi:10.1016/j.fgb.2005.09.004.
- Peñalva MA. Endocytosis in filamentous fungi: cinderella gets her reward. Curr Opin Microbiol. 2010;13(6):684–692. doi:10.1016/j.mib.2010.09.005.
- Piao HL, Machado IMP, Payne GS. NPFXD-mediated endocytosis is required for polarity and function of a yeast cell wall stress sensor. Mol Biol Cell. 2007;18(1):57–65. doi:10.1091/mbc.e06-08-0721.
- Ramsubramaniam N, Harris SD, Marten MR. The phosphoproteome of Aspergillus nidulans reveals functional association with cellular processes involved in morphology and secretion. Proteomics. 2014;14(21–22):2454–2459. doi:10.1002/pmic.v14.21-22.
- Reynaga-Peña CG, Gierz G, Bartnicki-Garcia S. Analysis of the role of the Spitzenkörper in fungal morphogenesis by computer simulation of apical branching in Aspergillus niger. Proc Natl Acad Sci USA. 1997;94(17):9096–9101. doi:10.1073/pnas.94.17.9096.
- Riquelme M, Aguirre J, Bartnicki-Garcia S, Braus GH, Feldbrugge M, Fleig U, Hansberg W, Herrera-Estrella A, Kamper J, Kuck U, et al. Fungal morphogenesis, from the polarized growth of hyphae to complex reproduction and infection structures. Microbiol Mol Biol Rev. 2018;82:2.
- Schultzhaus Z, Johnson TB, Shaw BD. Clathrin localization and dynamics in Aspergillus nidulans. Mol Microbiol. 2017;103(2):299–318. doi:10.1111/mmi.2017.103.issue-2.
- Schultzhaus Z, Quintanilla L, Hilton A, Shaw BD. Live cell imaging of actin dynamics in the filamentous fungus Aspergillus nidulans. Microsc Microanal. 2016;22(2):264–274. doi:10.1017/S1431927616000131.
- Schultzhaus Z, Yan H, Shaw BD. Aspergillus nidulans flippase DnfA is cargo of the endocytic collar and plays complementary roles in growth and phosphatidylserine asymmetry with another flippase, DnfB. Mol Microbiol. 2015;97(1):18–32. doi:10.1111/mmi.13019.
- Shaw BD, Chung DW, Wang CL, Quintanilla LA, Upadhyay S. A role for endocytic recycling in hyphal growth. Fungal Biol. 2011;115(6):541–546. doi:10.1016/j.funbio.2011.02.010.
- Stajich JE, Harris T, Brunk BP, Brestelli J, Fischer S, Harb OS, Kissinger JC, Li W, Nayak V, Pinney DF, et al. FungiDB: an integrated functional genomics database for fungi. Nucleic Acids Res. 2012;40(Database issue):D675–D681. doi:10.1093/nar/gkr918.
- Steinberg G. Endocytosis and early endosome motility in filamentous fungi. Curr Opin Microbiol. 2014;20:10–18. doi:10.1016/j.mib.2014.04.001.
- Steinberg G, Peñalva MA, Riquelme M, Wosten HA, Harris SD. Cell biology of hyphal growth. Microbiol Spectr. 2017;5(2):34.
- Sudbery P. Fluorescent proteins illuminate the structure and function of the hyphal tip apparatus. Fungal Genet Biol. 2011;48(9):849–857. doi:10.1016/j.fgb.2011.02.004.
- Taheri-Talesh N, Horio T, Araujo-Bazan L, Dou X, Espeso EA, Peñalva MA, Osmani SA, Oakley BR. The tip growth apparatus of Aspergillus nidulans. Mol Biol Cell. 2008;19(4):1439–1449. doi:10.1091/mbc.e07-05-0464.
- Takeshita N. Coordinated process of polarized growth in filamentous fungi. Biosci Biotechnol Biochem. 2016;80(9):1693–1699. doi:10.1080/09168451.2016.1179092.
- Takeshita N, Mania D, Herrero S, Ishitsuka Y, Nienhaus GU, Podolski M, Howard J, Fischer R. The cell-end marker TeaA and the microtubule polymerase AlpA contribute to microtubule guidance at the hyphal tip cortex of Aspergillus nidulans to provide polarity maintenance. J Cell Sci. 2013;126:5400–5411. doi:10.1242/jcs.129841.
- Tan PK, Howard JP, Payne GS. The sequence NPFXD defines a new class of endocytosis signal in Saccharomyces cerevisiae. J Cell Biol. 1996;135(6):1789–1800. doi:10.1083/jcb.135.6.1789.
- Thilo L. Quantification of endocytosis-derived membrane traffic. BBA-Biomembranes. 1985;822(2):243–266. doi:10.1016/0304-4157(85)90010-3.
- Upadhyay S, Shaw BD. The role of actin, fimbrin and endocytosis in growth of hyphae in Aspergillus nidulans. Mol Microbiol. 2008;68(3):690–705. doi:10.1111/j.1365-2958.2008.06178.x.
- Veiter L, Rajamanickam V, Herwig C. The filamentous fungal pellet-relationship between morphology and productivity. Appl Microbiol Biotechnol. 2018;102(7):2997–3006. doi:10.1007/s00253-018-8818-7.
- Verdín J, Sánchez-León E, Rico-Ramírez AM, Martínez-Núñez L, Fajardo-Somera RA, Riquelme M. Off the wall: the rhyme and reason of Neurospora crassa hyphal morphogenesis. Cell Surf. 2019;5(1):100020. doi:10.1016/j.tcsw.2019.100020.
- Vida TA, Emr SD. A new vital stain for visualizing vacuolar membrane dynamics and endocytosis in yeast. J Cell Biol. 1995;128(5):779–792. doi:10.1083/jcb.128.5.779.
- Virag A, Harris SD. The Spitzenkörper: a molecular perspective. Mycol Res. 2006;110:4–13. doi:10.1016/j.mycres.2005.09.005.
- Yang CD, Dang X, Zheng HW, Chen XF, Lin XL, Zhang DM, Abubakar YS, Chen X, Lu G, Wang Z, et al. Two Rab5 homologs are essential for the development and pathogenicity of the rice blast fungus Magnaporthe oryzae. Front Plant Sci. 2017;8:620. doi:10.3389/fpls.2017.00620.
- Zeilinger S, Gupta VK, Dahms TES, Silva RN, Singh HB, Upadhyay RS, Gomes EV, Tsui CK-M, Nayak SC. Friends or foes? Emerging insights from fungal interactions with plants. FEMS Microbiol Rev. 2016;40(2):182–207. doi:10.1093/femsre/fuv045.
- Zheng W, Lin Y, Fang W, Zhao X, Lou Y, Wang G, Zheng H, Liang Q, Abubakar YS, Olsson S, et al. The endosomal recycling of FgSnc1 by FgSnx41-FgSnx4 heterodimer is essential for polarized growth and pathogenicity in Fusarium graminearum. New Phytologist. 2018;219(2):654–671. doi:10.1111/nph.2018.219.issue-2.