ABSTRACT
Rock-inhabiting fungi (RIF) constitute an ecological group associated with terrestrial rocks. This association is generally restricted to the persistent colonisation of rocks and peculiar morphological features based on melanisation and slow growth, which endow RIF with significance in eukaryotic biology, special status in ecology, and exotic potential in biotechnology. There is a need to achieve a better understanding of the hidden biodiversity, antistress biology, origin and convergent evolution of RIF, which will facilitate cultural relic preservation, exploitation of the biogeochemical cycle of rock elements and biotechnology applications. This review focuses on summarising the current knowledge of rock-inhabiting fungi, with particular reference to terminology, biodiversity and geographic distribution, origin and evolution, and stress adaptation mechanisms. We especially teased out the definition through summing up the terms related to rock-inhabting fungi, and also provided a checklist of rock-inhabiting fungal taxa recorded following updated classification schemes.
1. Introduction
Rock represents the most ancient and widespread terrestrial niche among various substrates or habitats for life on earth (Gorbushina et al. Citation2002; Beraldi-Campesi Citation2013). During the long-term evolutionary history of fungi, rock-inhabiting fungi (RIF) forming black microcolonies on the surface of rocks have evolved. Most of them grow slowly, produce melanin, and mainly undergo meristematic development or produce yeast-like forms (Gorbushina et al. Citation1993; Wollenzien et al. Citation1995; Chertov et al. Citation2004). Based on these characteristics, RIF are also named microcolonial fungi, meristematic fungi or black yeasts according to different perspectives of morphology or physiology (de Hoog and Hermanides-Nijhof Citation1977; Staley et al. Citation1982; Sterflinger Citation2006). Compared to lichens, which symbiotically live with photosynthetic microorganisms such as Cyanobacteria or algae to form conspicuous thalli on rock surfaces, RIF generally refer to heterotrophic free-living eukaryotic microorganisms (Palmer et al. Citation1990) and thus can hardly be observed effortlessly. Rock-inhabiting black fungi exhibit excellent performance in a broad range of extreme environments, from hot tropical deserts to semidry and humid Mediterranean coasts and McMurdo Antarctic dry valleys with multiple erratic stresses, such as solar radiation, desiccation and rehydration, and temperature fluctuations (Staley et al. Citation1982; Selbmann et al. Citation2005; Onofri et al. Citation2014).
Although the existence of heterotrophic microorganisms on rocks was previously reported (Gromov Citation1957), it is generally believed that meticulous academic studies of RIF began with Staley et al. (Citation1982), who observed dark microcolonial structures on bare rock surfaces without algae or lichen by scanning electron microscopy (SEM) as well as high rates of physiological activity by respiration detection. Further confirmation was achieved with pure isolates of black fungi that could recolonize clean sterile marble within 3–6 months in the laboratory (Gorbushina et al. Citation1993). Subsequently, microscopic observation indicated limited diagnostic features of morphology based on their meristematic development or yeast-like form and a lack of ascosporic or conidial sporification (Palmer et al. Citation1987; Gorbushina et al. Citation1993; Sterflinger Citation2006; Gorbushina Citation2007). Due to the lack of morphological characteristics, reliable species delimitation of RIF did not become practicable by molecular phylogenetic analysis until the last decade of the 20th century (Bruns et al. Citation1991). Many microcolonial black fungi have been isolated and identified as new species and even higher ranks in recent years (Egidi et al. Citation2014; Isola et al. Citation2016; Sun et al. Citation2020). The molecular phylogeny of these fungal strains indicated their unique affiliation within Dothideomycetes, Eurotiomycetes and Arthoniomycetes in Ascomycota (Gueidan et al. Citation2008; Ruibal et al. Citation2008, Citation2009; Egidi et al. Citation2014). Furthermore, the phylogenetic frame of RIF within Dothideomycetes based on multiple genes was proposed at the order or family level by Ruibal et al. (Citation2009) and Egidi et al. (Citation2014). With novel taxa discovered from rocks, physiological studies of RIF have also been conducted, mainly including utilization of carbon or nitrogen sources (Nai et al. Citation2013) and resistance to harsh stresses such as desiccation, radiation, acids, hypersaline and temperature fluctuations (Palmer et al. Citation1987; Sterflinger and Krumbein Citation1995; Sterflinger Citation1998a, Citation1998b; Onofri et al. Citation2007; Gorbushina et al. Citation2008; Zakharova et al. Citation2013). In addition, the origin of this ecological group and its evolutionary relationship with other lifestyles, such as plant pathogens, black yeasts and lichens, were also observed (Gueidan et al. Citation2008, Citation2011; Ruibal et al. Citation2009; Abdollahzadeh et al. Citation2020).
Despite the extremely slow growth and thick melanised cell wall of RIF (Isola et al. Citation2011), their adaptation mechanism to harsh niches has been investigated by morphological observation, physiological testing, and comparative genome, transcriptome and proteome analyses (Tesei et al. Citation2012; Zakharova et al. Citation2013, Citation2014; Coleine et al. Citation2017, Citation2020). Gene editing and RNA interference approaches to elucidate unique RIF genes have been successfully established (Noack-Schönmann, Bus, et al. Citation2014) and applied in the typical rock-inhabiting fungus Knufia petricola (Voigt et al. Citation2020). RIF richness in nature has been well documented, and characterization of its antistress biological characteristics, significance in exobiology, and ecological functions has made significant progress in recent decades. However, many terms related to the fungi on rocks are not well defined and correctly used. There are also no calculations how many rock-inhabiting fungi (RIF) have been reported worldwide. This paper reviews advances in describing RIF in the areas of morphology, physiology, taxonomy, ecology, evolutionary biology, genomics, molecular biology and biotechnological applications (Selbmann et al. Citation2015; Prenafeta-Boldú et al. Citation2019; Favero-Longo and Viles Citation2020; Vasileiou and Summerer Citation2020). In addition, terms related to RIF and a RIF checklist are especially provided.
2 Terminology
Fungi that colonise rocks suffer multiple stresses and have evolved adaptation traits to cope with the hard conditions in the niches they reside. Various terms have been applied to describe fungi on rocks in the literature with overlapping meanings, such as rock-inhabiting fungi, lithophilic fungi, microcolonial fungi, meristematic fungi, and black yeasts. Although each term emphasises different characteristics, the fungi can be classified into different groups according to their predominant morphological characteristics (Sterflinger Citation2006). To precisely use those terms and assign the fungi to relevant groups, we discussed and provided a comprehensive understanding of each term.
2.1. Rock-inhabiting fungi (RIF)
“Rock-inhabiting fungi” (RIF) is an extensively used term when exploring taxonomy, evolution, ecology, physiology and molecular mechanisms to emphasise the “inhabiting” trait on rocks (Sterflinger and Krumbein Citation1995; Wollenzien et al. Citation1995). RIF gives a broad sense of emphasising the “rock” habitats that fungi colonise. However, neither the temporary colonisation of some ubiquitous hyphomycetes nor dormant spore contamination without physiological activity are recognised as inhabitants (Wollenzien et al. Citation1995; Sterflinger et al. Citation2012). Indeed, the term “rock inhabiting microbiota”, including either fungi or bacteria as well as algae colonising rocks, has been used in earlier studies (Urzì et al. Citation1995). Another infrequent term, “rock dwelling fungi”, highlighting the “inhabiting” feature, is sometimes adopted: these fungi utilise rocks not as a source of organic or inorganic nutrients but rather as a dwelling for colonisation and propagation (Gorbushina and Krumbein Citation2000). In addition, some more popular terms, such as “stone/rock eating fungi”, are employed to visually describe their colonisation and corrosion of rock surfaces (Urzì et al. Citation2000; Kirtzel et al. Citation2017).
2.2. Lithobiontic fungi
The term “Lithobiontic fungi” is derived from “lithobiont” through the ancient Greek etyma “litho-”, meaning “rocks” and “biont”, referring to “one having a (specified) mode of life”. Lithobiontic fungi generally refers to either slow-growing black yeast or extensive mould that lives on or inside rocks (Heinen and Lauwers Citation1986; Caretta et al. Citation2006). This term is much less frequently used than RIF. Some related concepts are more accurate or convenient to convey, for example, epilithic, chasmolithic, and chasmoendolithic/endolithic fungi to describe fungi colonising the surface, gathering in fissures and cracks, and those penetrating actively into the interior of rocks forming tunnels, respectively (Bentis et al. Citation2000; Miura and Urabe Citation2017). In addition, “endolithic fungi”, along with another derived phrase, “cryptoendolithic fungi”, are commonly used terms in extreme biology, especially studies of cryptoendolithic communities in the McMurdo Dry Valleys, Antarctica, which is known as the location most closely resembling the Martian environment on Earth (Palmer and Friedmann Citation1988; Selbmann et al. Citation2005; Onofri et al. Citation2015; Coleine et al. Citation2018).
2.3. Microcolonial fungi (MCF)
“Microcolonial fungi” (MCF) were proposed by Staley et al. (Citation1982) to refer to the colony appearance of the fungal assemblage residing on mineral substrates, mostly rock surfaces but also glass or metal, based on an ultrastructural examination of the microcolonial structures of black or brown stains on desert rocks. Generally, microcolonial fungi, especially those from rocks, are morphologically identified as having meristematic or yeast-like growth, with alterations of each other to a certain extent (Gorbushina et al. Citation1993; Wollenzien et al. Citation1995; Sterflinger Citation2006).
2.4. Meristematic fungi
The term “meristematic fungi” was introduced by de Hoog and Hermanides-Nijhof (Citation1977) to refer to fungi that form aggregates of thick-walled, melanised cells enlarging and reproducing by isodiametrical division. Meristematic growth by isodiametric cellular expansion, which results in a minimal surface/volume ratio, facilitates survival under extreme temperatures and desiccation and economises energy requirements (Wollenzien et al. Citation1995).
2.5. Black yeast
“Black yeast” refers a group of fungi that are quite heterogeneous from taxonomic and phylogenetic perspectives but have common melanised cell walls and form daughter cells by yeast-like multilateral or polar budding (de Hoog and Hermanides-Nijhof Citation1977; Sterflinger Citation2006). Most black yeasts additionally exhibit mycelial growth and generate conidia from simple phialides. Some meristematic fungi can also be classified morphologically as black yeast and vice versa. Both forms have close phylogenetic relationships (Sterflinger et al. Citation1999). Either meristematic fungi or black yeasts describe partial microscopic traits of filamentous melanised fungi not only from rock surfaces but also from other ecological niches, such as soils, plants, animals (Sterflinger Citation2006) and epilithic lichens (Selbmann et al. Citation2013). In particular, some black yeasts are pathogenic to humans, causing chromoblastomycosis as well as phaeohyphomycosis (Moreno et al. Citation2018).
2.6. Melanized/black/dematiaceous fungi
Many fungi, not limited to RIF, produce black pigments, mainly melanin, in fungal cell walls to make their colonies melanised (Revankar Citation2007; Revankar and Sutton Citation2010). Therefore, “melanised fungi”, “black fungi” or “dematiaceous fungi” frequently appear in the literature on RIF. It is noteworthy that the term “melanised” is a more accurate description and is more frequently used, especially concerning the opposite word “non-melanised” of melanin-mutated cells (Dadachova et al. Citation2007), while “dematiaceous” has gradually become disused and is restricted to ubiquitous, mostly plant-associated hyphomycetous fungi (Revankar Citation2007).
2.7. Lithophilic/lithotolerant fungi
Many extremophilic fungi living in unique habitats suffering single stresses, such as high temperature and high salinity, can be separated into “-philic” and “-tolerant” fungi by some parameters, for instance, thermophilic fungi, which have a maximum temperature for growth at or above 50°C and a minimum temperature for growth at or above 20°C, while thermotolerant fungi have a minimum temperature for growth below 20°C (Cooney and Emerson Citation1964). These terms are well understood and widely accepted. Although the term “rock-inhabiting fungi” has been extensively accepted with its broad meaning, fungi on rocks often suffer multiple stresses and cannot be separated into “-phillic” and “-tolerant” by some parameters. Generally, fungal strains isolated from rocks are classified as typical RIF with a slow-growing meristematic or yeast-like microcolonial appearance or nontypical strains with a relatively fast-growing and melanised appearance (Wollenzien et al. Citation1995; Gorbushina and Krumbein Citation2000). Most typical RIF species are isolated only from rocks and are considered obligate rock dwellers, while nontypical RIF species can colonise various habitats and thus be facultative on rocks. Sometimes, certain nontypical RIF cannot survive the fluctuating stresses on rocks and might be regarded not as rock-inhabiting fungi but as contaminating fungi (Palmer et al. Citation1987; Wollenzien et al. Citation1995). The term “lithophile” refers to microorganisms that usually benefit from the rock niche either on the surface or within deep cracks by making use of light or mineral energy (Mikhailyuk Citation2008; Kuklinski Citation2009). Another term, “polyextremotolerant”, describes the tolerance and adaptation to multiple and changing stresses in rock habitats (Gostinčar et al. Citation2012, Citation2015; Grube et al. Citation2013). Therefore, the terms “lithophilic” and “lithotolerant” have been redefined and proposed to be applied to the distinction between typical and nontypical rock fungi. Lithophilic fungi grow extremely slow by less than 1 mm. per week in general, while relatively fast-growing lithotolerant fungi could reach to 1 mm. or more per week (). Some distigushing parameters are also given ().
Table 1. Characteristics comparison between typical and nontypical rock fungi
Figure 1. Growth rate comparison on culture plates between litholithic fungi and lithotolerant fungi of rock-inhabiting fungi. Values of plots represents growth rates (mm. per week) of published RIF species on culture plates. Significant difference between green and Orange plots represents the distinction between typical and nontypical RIF.
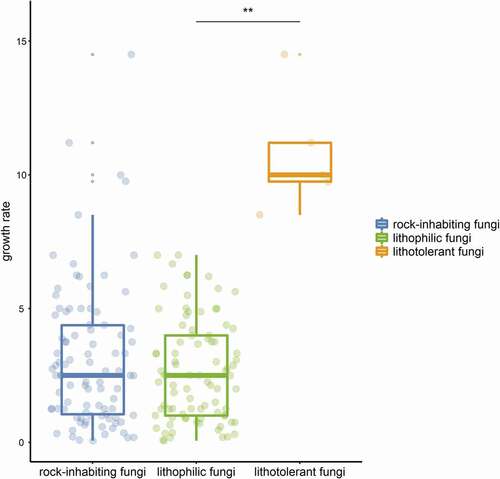
3. Isolation of rock-inhabiting fungi
The methodology to isolate slow-growing melanised rock-inhabiting fungi from stone objects has been gradually improved. RIF were originally isolated by picking up dark microcolonies from rock surfaces onto MEA (malt extract agar) plates with a sterilised needle or scalpel under a dissecting microscope (Wollenzien et al. Citation1995; Sterflinger and Gorbushina Citation1997; Ruibal et al. Citation2018) or scraping the greyish-black patina of samples (de Leo et al. Citation2019) onto agar plates. Melanised fungal strains could also be obtained via the inoculation of disinfected rock samples on MEA plates (Selbmann et al. Citation2005). Disinfection of rock surfaces is necessary to reduce contamination by airborne spores or propagative fragments of some ubiquitous hyphomycetes (Su et al. Citation2015). Rock samples are generally disinfected by several rinses for several seconds with disinfectors such as 95% ethanol, 8% H2O2 solution or physiological saline containing 0.01% – 0.001% Tween 20 (Selbmann et al. Citation2005; Su et al. Citation2015) and finally washed with sterilised distiled water several times.
Recently, the pour plate method with ground rock pieces has been applied for RIF isolation, which has proven to be effective for large-scale rock samples (Ruibal et al. Citation2005). The isolation efficiency was also improved by the adoption of some special agar plates, such as 1/10 MEA (Su et al. Citation2015), DG18 (dichloran-glycerol 18% agar) or DRBC (dichloran-rose Bengal chlortetracycline), to provide oligotrophic conditions, low water activity or inhibition of fast-growing fungi (King Jr et al. Citation1979; Ruibal et al. Citation2008). Certainly, a lower culture temperature also avoids contamination by fast-growing fungi. Supplementation with stable antibiotics such as chloramphenicol, streptomycin sulphate and terramycin in agar plates to suppress bacterial growth is beneficial for RIF purification (King Jr et al. Citation1979; Su et al. Citation2015; Sun et al. Citation2019, Citation2020). When appearing on DRBC plates, black colonies should be transferred onto MEA or PDA (potato extract agar) plates (Ruibal et al. Citation2005) for purification. Inoculation conditions can be flexible according to the circumstances of the sampled rocks to achieve a better isolation effect, for example, an adjustment of the appropriate temperature ranging from 10 to 25 degrees (Ruibal et al. Citation2005; Hubka et al. Citation2014; Su et al. Citation2015; Sun et al. Citation2019; Sun et al. Citation2020), 12 h of fluorescent light exposure, and continual removal of rapidly extending unpigmented colonies (Ruibal et al. Citation2005).
4. Research progress
4.1. Biodiversity and geographic distribution
Phylogenetic analysis has provided an essential tool for RIF identification. Worldwide, RIF investigations have been carried out in the past few decades. Rock samples were collected in environments ranging from hot deserts in subtropical and tropical areas (Staley et al. Citation1982; Sterflinger et al. Citation2012) to the cold McMurdo Dry Valleys in the Antarctic (Selbmann et al. Citation2005, Citation2008; Egidi et al. Citation2014) or from moderately humid and semidry Mediterranean coasts (Wollenzien et al. Citation1995, Citation1997; Sterflinger et al. Citation1997; Onofri et al. Citation2014) to mountain peaks in the Andes, Alps and Indian Himalayan ranges (Egidi et al. Citation2014; Hubka et al. Citation2014; Selbmann et al. Citation2014; Su et al. Citation2015; Sun et al. Citation2020). Sampling was distributed in Europe, Asia, Antarctica and America, including at least 16 countries. The rock samples included natural field rocks such as granite, marble, pegmatite, quartz, limestone and sandstone and litholic heritages such as museums, cathedrals, temples, cemeteries and ancient caves, chambers of historical sites, roof tiles and metro systems (Sert et al. Citation2007a, Citation2007b, Citation2011, Citation2012; Sert and Sterflinger Citation2010; Martin-Sanchez et al. Citation2012; Egidi et al. Citation2014; Isola et al. Citation2016; Réblová et al. Citation2016; Kiyuna et al. Citation2018; Trovão et al. Citation2019).
Many melanised microcolonial fungal strains isolated on rocks from various extreme environments have raised great interest in the hidden species diversity of RIF worldwide. It was a formidable task to identify slow-growing fungi with yeast-like morphology, meristematic development, or extremely thin hyphal structures (Gorbushina et al. Citation1993; Ascaso et al. Citation1995; Wollenzien et al. Citation1995) until the development of molecular phylogenetic analysis and its application to fungal taxonomy (Sterflinger et al. Citation1997; Taylor et al. Citation2000).
Although RIF are an ecological group of fungi, their phylogenetic positions are mainly affiliated with Dothideomycetes and Eurotiomycetes in Ascomycota (Gueidan et al. Citation2008; Ruibal et al. Citation2009; Egidi et al. Citation2014), as well as an unidentified lineage closely related to Arthoniomycetes (Ruibal et al. Citation2009). Furthermore, dothideomycetous RIF mainly cluster in the orders of Capodiales s. lat., Dothideales and Myriangiales in Dothideomycetidae, and Coniosporiales, Pleosporales and Venturiales in Pleosporomycetidae (Sterflinger et al. Citation1999; Ruibal et al. Citation2009; Egidi et al. Citation2014). Rencently, Capnodiales s. lat. was shown to be polyphyletic and separated into 7 orders, namely, Capnodiales. str., Cladosporiales, Comminutisporales, Mycosphaerellales, Neophaeothecales, Phaeothecales and Racodiales (Abdollahzadeh et al. Citation2020). Meanwhile, eurotiomycetous RIF are mostly gathered in Chaeotothyriales within Chaeotothyriomycetidae along with a few taxa in Eurotiales and Verrucariales (Sterflinger and Hain Citation1999; Gueidan et al. Citation2008). The RIF lineages closely related to Arthoniomycetes did not seem to form a monophyletic group, and their position in this class are not clear yet (Ruibal et al. Citation2009). According to the updated fungal nomenclature based on the databases of the Index Fungorum (http://www.indexfungorum.org) and MycoBank (http://www.mycobank.org) and a recent phylogenetic revision (Abdollahzadeh et al. Citation2020), more than 175 RIF species distributed in at least 16 countries worldwide are recorded in 1 phylum, 2 classes, 11 orders, 19 families and 64 genera, among which 1 new family, 27 new genera and 95 new species were established recently (; ).
Table 2. Species numbers and their affiliation of rock-inhabiting fungi
Figure 2. Distribution of rock-inhabiting fungi. Size of blue circles represents the genus number of rock-inhabiting fungi.
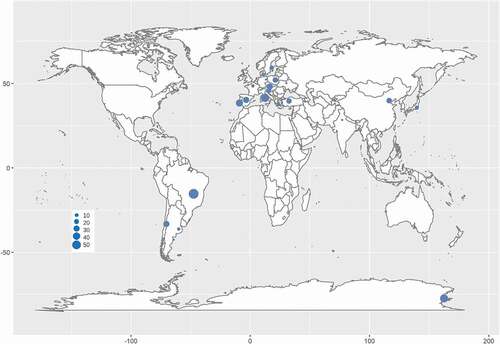
RIF have been deemed a typical ecological group rather than a phylogenetic lineage, as they were revealed to be polyphyletic based on either teleomorphic traits or molecular data (Golubic et al. Citation1981; Wollenzien et al. Citation1995; Sterflinger et al. Citation1999). These lineages possess various lifestyles, such as plant pathogens, epiphytes, saprobes and lichen-forming fungi in Dothideomycetes (Ruibal et al. Citation2009) and aquatic, ant-associated, myco-parasitic and human opportunistic lifestyles in Chaetothyriales (Teixeira et al. Citation2017). RIF often form early phylogenetic clades in Dothideomycetes and Eurotiomycetes, triggering the question of whether the rock surface was a terroir for ancient lineages or a reservoir for plant-associated fungi (Ruibal et al. Citation2009).
A phylogenomic approach was adopted to resolve relationships among fungi in Dothideomycetes, and two rock-inhabiting fungal genera, Lichenothelia and Saxomyces, have been suggested to be early diverging lineages. Ancestral character state reconstruction also suggested that the rock-inhabiting lifestyle is ancestral within the class (Ametrano et al. Citation2019). Another ancestral state reconstruction of Capnodiales s. lat., the second largest order possessing the most RIF taxa within Dothideomycetes, revealed its saprobic lifestyle, not specifically referring to its extremotolerant rock-inhabiting but rather an ancestral state relative to parasitic, epi- or ecto-phytic and lichenized lifestyles (Abdollahzadeh et al. Citation2020). Reconstructions of RIF-related orders within Eurotiomycetes also revealed that the most recent common ancestor of Verrucariales and Chaetothyriales is a nonlichenized rock inhabitant. Verrucariales is one of the independent groups where lichenization has evolved on a hostile rock surface that might have favoured the shift to a symbiotic lifestyle (Gueidan et al. Citation2008). The origin of RIF has been estimated back to the evolution of Dothideomycetes and Chaetothyriales in the Late Devonian and a much later period, the Middle Triassic, respectively, through a relaxed clock model combined with several fossil and secondary calibrations, which is confirmed by the fact that the lineages of RIF related to Chaetothyriales show a much narrower phylogenetic spectrum than Dothideomycetetes. The Devonian and Triassic epochs share characteristics of large arid landmasses, but the temperatures of the latter were much cooler than those of the former (Gueidan et al. Citation2011).
The evolutionary footprint was also traced by the shared characteristics between rock inhabiting fungi and other lifestyles within Dothideomycetes or Chaetothyriales. Melanised fungi form microcolonies not only on mineral substrates such as rocks but also on various extensive hard material surfaces, including outdoor and indoor glass, plastics, roof tiles, solar panels, moisteners, humidifiers and dishwashers (Gostinčar et al. Citation2011; Ruibal et al. Citation2018). Indeed, the bounds of this special group can be expanded to be polyextremotolerant, since stress factors on rocks are partly found in hyperhaline, acidic and radiation- or toxin-polluted soils/waters, where many rock dwellers have been observed to be holotolerants, acidotolerants and exotic carbohydrate degraders (Selbmann et al. Citation2008; Isola et al. Citation2013; Coleine et al. Citation2020; Su et al. Citation2020; Tesei et al. Citation2020). Although the CFPP-like (carbon fixation pathway of plants) pathway from fungi for carbon fixation is incomplete due to the absence of the unique enzymes Rubisco (ribulose-1,5-bisphosphate carboxylase-oxygenase) and PRK (phosphoribulokinase) (Lyu et al. Citation2015), nutritional pathways, especially in RIF, might have developed, since lithotrophs or photoheterotrophs were reported among hypersaline-tolerant and endolithic marine fungi through the oxidation of elemental sulfur, iron and manganese or light-driven rhodopsin transmembrane protons and sodium pumps for extra energy (Gleason et al. Citation2019) and radiotropism in reactor cooling water through melanin-dominant radiation adaptation, capture and energy transduction (Dadachova and Casadevall Citation2008). Equal photoreceptors (PRs) were detected in RIF genomes, which are similar to the plant pathogen Botrytis cinerea (Leotiomycetes), in which PRs function in sensing and avoiding sunlight stresses and locating susceptible hosts (Schumacher and Gorbushina Citation2020). Co-culture experiments confirmed the capacity for RIF to maintain structural and functional associations, an underlying protolichen format, with bacteria, especially cyanobacteria (Gorbushina et al. Citation2005; Gueidan et al. Citation2008; Ruibal et al. Citation2009; Gostinčar et al. Citation2012). Some studies also indicated that ant‐associated lifestyles might have driven the diversification of Chaetothyriales due to the metabolic capabilities of toxic compounds produced by ants (Teixeira et al. Citation2017; Moreno et al. Citation2018). Regarding the pathogenicity presented by rock dwellers, there was a statistically significant co-occurrence in the orders of Capnodiales, Dothideales, and Chaetothyriales in a kingdom-wide phylogenetic analysis (Gostinčar et al. Citation2018b). Although physiological virulence characteristics such as tolerance to raised temperature in warm-blooded animals or haemolysis on blood agar were rarely significantly detected in typical melanized rock fungi (Gostinčar et al. Citation2011; Gonçalves et al. Citation2017), virulence-associated genes were shown to be unnecessary in opportunistic human fungi (Gostinčar et al. Citation2018b). As melanin has exhibited great talent for oxidative stress resistance, virulence, camouflage, immune defence and copper and zinc homeostasis in mammalian tissues (Cunha et al. Citation2010; Silva-Bailao et al. Citation2018; Freitas et al. Citation2019; Smith and Casadevall Citation2019), it might be an evolutionary alternative form of stress adaptability to human opportunistic pathogenicity (Gostinčar et al. Citation2018b).
There are several hypotheses to elucidate the evolutionary dynamics of rock-inhabiting fungi. A proposal highlighting the potential oneness between rock-inhabiting fungi and their related lifestyles within both Dothideomycetes and Chaetothyriales has been mentioned. Some niches, such as rock or hard material surfaces, phyllosphere, and living mammalian tissues, which are widely divergent as described, share some main ecological similarities, such as increased temperature, osmotic stress, UV radiation and oxygenic action (Sterflinger Citation2006). Another proposal may underlie the separate evolutionary tactics of the two classes. Lineages with true extremophilic traits, such as psychrophiles, acidophiles or halophiles, tend to cluster in Dothideomycetes, while those with a larger spectrum of assimilative abilities, such as thermotolerance, toxin degradation or opportunistic pathogenicity, cluster in Chaetothyriales (Isola et al. Citation2016).
4.2. Niche adaptation mechanisms
4.2.1 Morphological and physiological adaptation traits
Adaptative evolution of RIF to a niche with multiple stresses has resulted in their idiosyncratic cellular structures. One is the strong accumulation of melanin in the cell walls, which is thickened with multilayered construction, and the other is microcolonies forming on solid substrates via meristematic or yeast-like growth.
Melanisation endows RIF with inimitable survival advantages compared to soil- or plant-related fungi (Ruibal et al. Citation2008), algae and even lichens (Perry et al. Citation2004; Scalzi et al. Citation2012; Pacelli et al. Citation2017b) among eukaryotic organism domains. Fungal melanin is regarded as a strong safeguard against a wide range of electromagnetic radiation, from nonionising UV radiation to even ionising X-rays, gamma radiation beta-radiation and deuterons (Casadevall et al. Citation2017; Vasileiou and Summerer Citation2020). In addition, melanin exhibits sufficient protection for fungal cell growth under other stresses by performing oxidative or free radical scavenging, withstanding dehydration or high temperatures, and increasing mechanical-chemical cellular strength and heavy metal ion binding (Cordero and Casadevall Citation2017). Other protective soluble compounds with multiple stress resistance to desiccation, temperature, and irradiation also tend to be accumulated in black fungal cells. Intracellular mycosporines, such as mycosporine-glutaminol, mycosporine-glutaminol-glucoside, mycosporine-glutamicol, and mycosporine-glutamicol-glucoside, act as UV filters, antioxidants and minor osmolytes (Volkmann et al. Citation2003; Gorbushina et al. Citation2008) as well as possible hyphal development regulators of nonexpansive intracolonial growth (Gorbushina Citation2003). Diverse carotenoids including carotene, didehydrolycopene, lycopene, phytoene, torulene and torularhodin contribute to cell membrane stabilisation (Gorbushina et al. Citation2008) as well as antioxidative function and cold resistance (Gorbushina Citation2003; Flieger et al. Citation2018). Accumulation of some regular modulating substances, such as trehalose, mannitol and glycerol (Sterflinger Citation1998a, Citation1998b; Coleine et al. Citation2021), assists in survival under high temperatures, desiccation and osmotic stress on rocks.
Additionally, extracellular polymeric substances (EPS) composed of polysaccharides, glycoproteins and enzymes around fungal cells form a complex network to survive against various stresses, including temperature fluctuations, low water availability, UV radiation, and nutrient deficiency (Gorbushina Citation2003; Omelon Citation2008; Noack-Schönmann et al. Citation2014b; Breitenbach et al. Citation2018). These EPSs are also involved in the interaction of RIF with algae, cyanobacteria and heterotrophic bacteria (Gorbushina and Broughton Citation2009)
Compared with the typical filamentous growth and spore spreading habits of normal fungi, RIF meristematic or yeast-like growth is the common format to survive in rock niches. Meanwhile, sexual reproduction was spontaneously abandoned, and typical conidiophores rarely develop for RIF (Sterflinger Citation2006; Gorbushina Citation2007). Along with the fluctuation of environmental conditions, drastical morphological alterations were observed from meristematic growth to yeast-like cells, even to merely thin hyphae (Wollenzien et al. Citation1995; Ruibal et al. Citation2008; de Leo et al. Citation2019). There are numerous benefits for RIF derived from this slow radial extension and extraordinary propagation:
Meristematic or yeast-like growth results in a microcolony that can be embedded in extracellular polymeric substances to have a thermodynamically optimal surface and efficient exchange process rate to protect against extensive evaporation (Gorbushina and Krumbein Citation2000; Gorbushina Citation2003);
Meristematic swelling of cells and the formation of endocondia also have advantages for water-independent propagation, such as budding or germination. (Wollenzien et al. Citation1995; Gorbushina Citation2003)
Morphological alteration from spherical yeast cells to the filamentous hyphal form responding to environmental fluctuation represents the flexible balance between adversity survival and nutritional exploration (Chertov et al. Citation2004; Tonon et al. Citation2021).
4.2.2. Genomic and proteomic features
RIF adapted to the rock niche have evolved special phenotypic traits, including morphological and physilogical characteristics and antistress biology. To understand the phenotypic traits, several typical RIF have been genome sequenced, including Cryomyces antarcticus MA 5682 (Sterflinger et al. Citation2014), Friedmanniomyces endolithicus CCFEE 5311, Friedmanniomyces simplex CCFEE 5184, Hortaea thailandica CCFEE 6315 (Coleine et al. Citation2020), Rachicladosporium antarcticum CCFEE 5527 and Rachicladosporium sp. CCFEE 5018 (Coleine et al. Citation2017) from the coldest and most hyperarid desert McMurdo Dry Valleys of the Antarctic and one strain, Coniosporium apollinis CBS 100218 (Sterflinger et al. Citation2014), from the environment incorporating high temperature, desiccation and radiation occurring in the semiarid Mediterranean. Other melanized fungal strains isolated from hypersaline or peracid substrates or as human opportunistics were also genome sequenced (). Two strains, C. antarcticus MA 5682 and F. endolithicus CCFEE 5311, possessing excellent stress resistance against solar radiation, radioactivity, desiccation and oligotrophic conditions, were analysed in detail. The preliminary genome analysis of C. antarcticus MA 5682 indicated that there were no significant differences compared with the model filamentous fungus Neurospora crassa or other RIF strains, concerning either their genomic size (24 Mb) or GC content (53.84%) and the percentage (0.33) of repetitive sequences (Sterflinger et al. Citation2014). However, genome assembly and annotation of F. endolithicus CCFEE 5311 and R. antarcticum CCFEE 5527 showed that their genome sizes were double the average in black fungi with raletively high GC content (49–56.5%) (Coleine et al. Citation2017, Citation2020). Additionally, some genomic features unique to Friedmanniomyces spp. strains were detected, such as responses to X-ray radiation, DNA damage, and salt tolerance stress. It is noteworthy that the large genome size of F. endolithicus CCFEE 5311 (Coleine et al. Citation2020), similar to the halophilic strain H. werneckii EXF-2000 from hypersaline environments, suggests a large-scale genome duplication in the Antarctic species to adapt and survive in the hostile conditions of the ice-free areas of the Antarctic, which are prohibitive for most life forms (Lenassi et al. Citation2013). Another study proposed hybridization between two haploids in the genome of H. werneckii, other than endoreduplication, as suggested previously (Gostinčar et al. Citation2018a), which might provide novel ideas regarding the genomic features of RIF.
Table 3. Genomes of rock-inhabiting fungi and related strains
The dynamic changes in whole-cell protein patterns of extremotolerant RIF under stresses also implied their peculiar potential adaptation mechanisms. The protein profiles of three niche-adapted groups of RIF were determined, e.g. F. endolithicus CCFEE 5208 (Tesei et al. Citation2012) and C. antarcticus MA 5682 (Zakharova et al. Citation2013, Citation2014), which are extremophilic cryptoendolithic fungi from cold and dry Antarctica; Knufia perforans (=Coniosporium perforans) MA 1299 (Tesei et al. Citation2012; Zakharova et al. Citation2013, Citation2014), which is a mesophilic but highly stress-tolerant microcolonial fungus from hot and dry environments in the Mediterranean; and Exophiala jeanselmei MA 2853 (Tesei et al. Citation2012; Zakharova et al. Citation2013, Citation2014), which is a rock-inhabiting black yeast closely related to opportunistic pathogens of humans. The 2-D protein spectra were not the same for the three types of RIF after desiccation stress, and both mesophilic strains E. jeanselmei and K. perforans (=C. perforan) showed clear production of small proteins (Zakharova et al. Citation2013). When RIF were stimulated with high temperature up to 40°C, the extremotolerant C. antarcticu did not show any response to desiccation but seemed to downregulate its metabolism. Nevertheless, compared with the mesophilic hyphomycete Penicillium chrysogenum, which expressed a higher number of proteins exhibiting real signs of temperature-induced reactions (Tesei et al. Citation2012), all three groups of RIF decreased their expressed protein numbers, indicating a downregulation of their metabolism under stress (Tesei et al. Citation2012). When exposed to thermophysical Mars-like conditions in terms of simulant gas composition, pressure and humidity with day-night fluctuating simulations of radiation spectra ranging from 200 nm to 2200 nm and temperatures ranging from −55°C to 15°C, those fungi showed certain stable survival traits, with upregulation of some unidentified proteins, significant decreases in protein numbers detected and no expression of any additional proteins such as heat shock proteins (HSPs) (Zakharova et al. Citation2014). Indeed, it would be a better survival strategy to express a specialized basic set of proteins in RIF compared with the production of HSPs, which is more energy-consuming (Naranjo‐Ortiz and Gabaldón Citation2019). The “Shed light in The daRk lineagES of the fungal tree of life” (STRES) project focusing on extremotolerant black fungi in different ecologies and life-styles, e.g. black yeasts, ant- and lichen-associated fungi, rock-inhabiting fungi etc., by genome sequencing and analysis coupled with transcriptomics and metabolomics experiments, may provide a comprehensive understanding of RIF (Selbmann et al. Citation2020).
4.2.3. Metabolic response to stresses
The metabolic adaptation of RIF to stresses has been investigated in recent years. C. antarcticu, a typical melanized RIF, constitutively synthesizes melanin pigments by both the 1,8-dihydroxynaphthalene (DHN) and 1,3–4 dihydroxyphenylalanine (L-DOPA) pathways (Pacelli et al. Citation2020). The resistance of melanized fungi to cosmic and terrestrial ionizing radiation suggests that melanin plays a pivotal role in radioprotection. Melanin afforded protection for both Cryptococcus neoformans, a fast-growing pathogenic basiodiomycete, and C. antarcticu against high-dose deuterons, as well as C. antarcticus against X-rays. Deuterons increased XTT activity in melanized cells of these two species, which reflected the metabolic activity of the cells (Pacelli et al. Citation2017a). A similar response was observed within another cryptoendolithic fungus, F. endolithicus, isolated from Antarctica under γ-radiation stress (Pacelli et al. Citation2018b). These results may be attributed to the interaction of radiation with melanin reflected by the XTT assay and the increase in cell metabolic rates in response to radiation insult, perhaps resulting in damage repair (Pacelli et al. Citation2017a, Citation2017c, Citation2018a).
Notably, the highly damaging deuteron dosage caused a decrease in ATP levels in both melanised cells and non-melanised cells, with a sharper gradient observed in the melanised cells of C. antarcticus (Pacelli, Bryan, et al. Citation2017). A consistent drop in the ATP pattern was observed when C. neoformans forming induced DOPA-melanin was exposed to a series of emission spectra from visible light to nonionising UV radiation and ionising gamma radiation (Bryan et al. Citation2011). Meanwhile, a slight decline was detected in non-melanised cells of C. antarcticus (Pacelli, Bryan, et al. Citation2017), while there was maintenance or even an increase in non-melanized C. neoformans cells (Bryan et al. Citation2011; Pacelli et al. Citation2017a). An interesting possibility could be that melanins have functions analogous to other energy harvesting pigments, such as chlorophylls, resulting in ATP consumption during the synthesis of simple sugars by melanised fungi (Bryan et al. Citation2011). In fact, it is common to enhance the growth of melanised fungal cells after exposure to ionising radiation, such as black yeasts Wangiella dermatitidis and pathogenic C. neoformans (Dadachova et al. Citation2007). Apart from radiant energy transduction by melanin, it was also proposed that upregulation of many key genes caused by radiation stimulation and an inducible microhomology-mediated recombination pathway could be a potential mechanism of adaptative evolution in eukaryotes (Dadachova and Casadevall Citation2008).
Extremely slow-growing RIF has resulted in difficulties in performing deep investigation of their molecular mechanisms. However, the efficient genetic manipulation of the RIF strain Knufia petricola A95, a model species with the most typical characteristics of RIF that is widespread in most rock niches (Nai Citation2014), has been successfully established with a protoplast-based DNA transfer system (Noack-Schönmann et al. Citation2014). Traditional gene knockout, editing and replacement via plasmid-based or ribonucleoprotein (RNP)-based CRISPR/Cas9 and silencing by RNA interference (RNAi) have been realized (Voigt et al. Citation2020). These results certainly provide a new approach for adaptation mechanism studies of RIF to stresses.
5. Ecological significance and biotechnological exploration
5.1. Astrobiology and extraterrestrial life applications
Bare rocks represent a reasonably complicated ecological niche that is the closest to Mars-like conditions on Earth, especially in the McMurdo Dry Valleys, which is characterised by extremely hard desiccation, high UV exposure, extremely low temperatures and wide thermal fluctuations in the Antarctic (Onofri et al. Citation2004, Citation2007). It is thus of great significance to select rock-inhabiting fungi as eukaryotic models in astrobiology to investigate the possibility of extinct or extant life on extraterrestrial planets such as Mars. Melanin, with the detection of its clear and strong Raman signal (Culka et al. Citation2017), has been designated as a potential feasible biosignature by the BIOlogy and Mars EXperiment (BIOMEX), which aims to detect signatures of extinct or extant life using sensitive and nondestructive approaches (Selbmann et al. Citation2015; Coleine et al. Citation2021).
In the framework of the Lichens and Fungi Experiments (LIFE) programme conducted by the European Space Agency (Onofri et al. Citation2008), the Antarctic cryptoendolithic black fungal strain C. antarcticu CCFEE 515 exhibited great resistance with 12.5% viability of culturable cells and more than 60% of the cells remaining intact after long-term (1.5 years) exposure to the Simulated Martian Condition imitation experiment on the International Space Station (ISS) (Onofri et al. Citation2012, Citation2015), with only slight ultrastructural and molecular damage (Pacelli et al. Citation2017a, Citation2017c; Onofri et al. Citation2018). Concerning exposure to space-relevant irradiation, UV radiation, and even sparsely and densely ionising gamma, deuteron and X-ray radiation, C. antarcticus CCFEE 515 also exhibited striking endurance (Selbmann et al. Citation2018; Pacelli et al. Citation2019).
5.2. Biodeterioration and biogeochemistry
Fungi have been considered significant “invaders” (Vázquez-Nion et al. Citation2016; Pinheiro et al. Citation2019) that cause aesthetic, chemical, physical and mechanical deterioration of stones and especially rocky cultural relics such as sculptures, monuments and reliefs. Rock-inhabiting fungi were proven to be a more difficult enemy due to their excellent stress resistance and ability to perennially infect exposed bare rocks (Gorbushina Citation2007) and tenaciously recolonise stone walls after treatment with biocides or radiation (Sterflinger and Piñar Citation2013).
It was determined that biodeterioration mechanisms for RIF should involve mechanical destruction through hyphal penetration rather than the general acid dissolution mechanism used by many other types of fungi (Gorbushina et al. Citation1993). RIF tend to search for cavities or cause micropits on rock surfaces, forming a habitat or shelter to contain fungal colonies (Gadd Citation2017). These microcolonial fungi also developed an endolithic or chasmoendolithic ability to grow in cracks and pores (Caneva et al. Citation2014) by observation of junctions between crystals or thigmotropic penetration in weak areas (Scheerer et al. Citation2009). When subjected to extremely hostile conditions, they disappear from the surface and struggle to dig into sedimentary soft rock substrates such as carbonate at depths ranging from a few hundred microns to several millimetres for new substrates (Favero-Longo et al. Citation2011). It has been confirmed that fungal growth exerts a strong mechanical pressure, up to 12.39 bar, equal to 4.5 times the pressure that a person would require to crush a glass bottle (Dornieden et al. Citation2000; Bogomolova et al. Citation2003). Additionally, melanin confers extramechanical strength to the hyphae and enhances mechanical penetration (Onofri et al. Citation2014). Indeed, the chemically corrosive capacity of RIF cannot be ignored, including the secretion of siderophore-like compounds causing increased dissolution of limestone by the model iron chelator desferrioxamine (Favero-Longo et al. Citation2011).
RIF are also able to form biofilms in cooperation with other organisms, such as bacteria or algae (Seiffert et al. Citation2014). Subaerial biofilms (SABs) are composed mainly of phototrophic algae, cyanobacteria, heterotrophic bacteria and black rock fungi (Noack-Schönmann et al. Citation2014b). It has been frequently reported that SABs growing on solar panels may lead to a severe blockage of up to 70% of light transmission (Noack-Schönmann et al. Citation2014b; Shirakawa et al. Citation2015). During biofilm development processes, RIF are considered secondary residents, in contrast to the fast colonisation of rapid-growing pioneer colonisers, mainly Chlorophyta and Cyanobacteria, at the cost of a high organismal loss rate, with slower growth but more sustainable colonisation ability to cause low-speed but persistent material losses (Bogomolova et al. Citation2003; Vázquez-Nion et al. Citation2016).
5.3. Biotechnological exploration
Research on RIF has been carried out for broad biotechnological applications in the manufacturing, electronics engineering, astronautics, cosmetic, biomaterials, pharmaceutical and environmental bioremediation industries. Melanin is one of the most applicable materials derived from RIF.
To achieve good ultraviolet absorption properties, fungal melanin can be added as a protective component in the manufacture of blinkers, windows, packaging material, umbrellas, canopies (Pombeiro-Sponchiado et al. Citation2017), and even some skin photoprotection formulations such as face and hand creams, lotions, antiaging ointments, or foundation makeup (Liberti et al. Citation2020). Additionally, the capacity of melanin to attenuate ionising radiation, such as beta-radiation in outer space, holds great significance in radiation shield design for human space flight in general and habitat structures on the Moon and Mars (Lakk et al. Citation2018; Vasileiou and Summerer Citation2020). Considered an organic semiconductor that is cheaper and easier to process than inorganic semiconductors due to its similarity to amorphous semiconductor solids in terms of electrical conductivity properties, melanin could be a promising material for sensors and photovoltaic devices (Vahidzadeh et al. Citation2018).
Melanin was also proven sufficiently biocompatible to be applied as a nanocarrier drug release device during the treatment of intestinal and colon diseases or radiation therapy to tumours (Araújo et al. Citation2014). It is pleasantly surprising that some black fungi showed strong biological activities against the pathogenic bacteria Staphylococcus aureus and Escherichia coli, pathogenic fungi Candida albicans and C. glabrata, and even breast tumour cells (Gonçalves et al. Citation2014), indicating potential sources of bioactive compounds for drug discovery.
Given the ability of melanin to bind to metals and degrade exotic carbohydrates, black rock fungi could be good candidates for environmental bioremediation in contaminated sites with heavy metals and radionuclides, for example, to absorb or remove harmful volatile chemicals in decorated rooms (Prenafeta-Boldú et al. Citation2019) or to clean up industrial effluent with radioactive contamination (Singleton and Tobin Citation1996).
6. Conclusion and future remarks
Despite their sparse colonisation on terrestrial lands and slow growth on nutrient culture dishes, melanised microcolnial fungi inhabiting bare rocks, the “dark matter” in the world of eukaryotic life, have been unveiled to show formidable vitality, amazing biodiversity, momentous evolutionary status, extraordinary adaptation mechanisms, and promising biotechnological exploration.
Approaches based on culture-dependent and multilocus sequences have revealed huge biological diversity and the great potential of novel RIF taxa and have contributed to understanding the richness and distribution of RIF on rocks, especially cryptoendolithic communities in Antarctica (Coleine et al. Citation2021), in recent decades. High-throughput amplicon sequencing, as a new culture-independent method, has significantly enhanced the fungal communities in rock. However, there are some weaknesses in RIF community studies, such as exogenous DNA contamination of low-biomass assemblages (Cuscó et al. Citation2018) and limited identification by short amplicon regions such as ITS1 or ITS2 (Luo et al. Citation2020; Nagano et al. Citation2020; Zhang et al. Citation2020). The application of full-length amplicon sequencing by single-molecule real-time (SMRT) sequencing methods for species-level analysis (Zhang et al. Citation2020) might boost progress on the species diversity of RIF. Moreover, shotgun metagenomics and a single-cell genomics could also be a powerful means to detect novel taxa (Wu et al. Citation2019).
Although the term “rock-inhabing fungi” has been well accepted by mycologists and “lithophilic fungi” and “lithotolerant fungi” are also proposed in this paper, a thorough description and comprehensive understanding of fungi on rocks remain to be provided in future studies. Primarily, what is the boundary of rock-inhabiting fungi? In the “lithophilic and lithotolerant fungi” classification system of RIF, lithophilic fungi with typical RIF traits can be regarded as “kernels”, while lithotolerant fungi can be regarded as a “border” with polyextremotolerance of rock-like niches, since they play an ambiguous role between rock inhabitants and contamitants. Furthermore, is there a succinct parameter distinguishing lithophilic fungi and lithotolerant fungi? In general, the tiny colonies on rocks exhibit two extension forms on agar culture: extremely slow-growing microcolonies and moderate fast-growing ones (; ). The ratio of the growth on rock to that on agar culture should be more accurately evaluated and calculated, and a clear parameter might be deduced, which should be considered a meaningful attempt to delimit their true characteristics.
Now that this ecological fungal group is mainly affiliated with two classes, Dothideomycetes and Eurotiomycetes, and is distributed in different families and genera, similar adaptation to rock niches by different lineages of RIF might underlie their convergent evolution. Cellular adaptation to the rock niche may occur with different patterns; for example, phenotypic variation, such as the accumulation of chemical compounds and control of membrane fluidity, is easy and nonheritable (Naranjo‐Ortiz and Gabaldón Citation2019), and the evolution of genomes and transcription regulation styles is more profound. In the case of higher eukaryotes, a general stress response was observed when compared to different environmental stresses, such as light, heat or salt, underlying commonalities among diverse biotic stimuli (Cole and Tringe Citation2021). Unlike the HSP response common in fungal cells in response to stressful stimuli, the extraordinary proteomic dynamics of RIF indicate a potential specific adaptation mechanism following exposure to multiple and fluctuating stresses.
Rock-inhabiting fungi represent a broader research field and will provide a deeper understanding of eukaryotic organisms. Taxonomic studies tremendously enrich the biological diversity of fungi, ecology and evolution studies contribute to the inferrence of species origins, and exploration of adaptation mechanisms triggers the elucidation of antistress biology and cosmobiology. RIF is also a good example of resource utilisation from natural environments with broad applications in medicine, industry, and agriculture.
7. Species Checklist
Typical rock-inhabiting fungi
Ascomycota
incertae sedis
Knufia L.J. Hutchison & Unter., Mycologia 87: 903 (1996)
Knufia calcicola L. Su, W. Sun & M.C. Xiang, Journal of Fungi 6 (4, no. 187): 18 (2020)
Obligate synonyms: Knufia calcarecola L. Su, W. Sun & M.C. Xiang (2020) Orthographic variant
Knufia karalitana Isola & Onofri, Fungal Systematics and Evolution 3: 128 (2019)
Taxon synonyms: Knufia karalitana Isola & Onofri, Fungal Diversity 76: 88 (2015) invalid Art. 40.7 (Melbourne)
Knufia marmoricola Onofri & Zucconi, Fungal Systematics and Evolution 3: 128 (2019)
Taxon synonyms: Knufia marmoricola Onofri & Zucconi, Fungal Diversity 76: 88 (2015) invalid Art. 40.7 (Melbourne)
Knufia mediterranea Selbmann & Zucconi, Fungal Systematics and Evolution 3: 128 (2019)
Knufia separata L. Su, W. Sun & M.C. Xiang, Journal of Fungi 6 (4, no. 187): 19 (2020)
Knufia vaticanii Zucconi & Onofri, Fungal Diversity 76: 88 (2015) invalid Art. 40.7 (Melbourne)
Knufia perforans (Sterfl.) Tsuneda, Hambl. & Currah, Botany 89: 887 (2011)
Obligate synonyms: Knufia perforans (Sterfl.) Tsuneda, Hambl. & Currah, Botany 89 (8): 534 (2011) Invalid Art. 41.5 (Melbourne)
Basionym: Coniosporium perforans Sterfl., Antonie van Leeuwenhoek 72 (4): 352 (1997)
Knufia petricola (Wollenz. & de Hoog) Gorbushina & Gueidan, Fungal Genetics & Biology 56: 58 (2013)
Basionym: Sarcinomyces petricola Wollenz. & de Hoog, Antonie van Leeuwenhoek 71 (3): 283 (1997)
Knufia chersonesos (Bogomolva & Minter) Tsuneda, Hambl. & Currah, Botany 89: 887 (2011)
Basionym: Phaeococcomyces chersonesos Bogom. & Minter, Mycotaxon 86: 203 (2003)
Obligate synonyms: Knufia chersonesos (Bogomolva & Minter) Tsuneda, Hambl. & Currah, Botany 89 (8): 535 (2011) Invalid
Dothideomycetes
incertae sedis
Cryomyces Selbmann, de Hoog, Mazzaglia, Friedmann & Onofri, Studies in Mycology 51: 19 (2005)
Cryomyces antarcticus Selbmann, de Hoog, Mazzaglia, Friedmann & Onofri, Studies in Mycology 51: 19 (2005)
Cryomyces funiculosus Selbmann & de Hoog, Fungal Diversity 86: 123 (2017)
Taxon synonyms: Cryomyces funiculosus Selbmann & de Hoog, Fungal Diversity 65 (1): 175 (2013) Invalid Art. 40.6
Cryomyces minteri Selbmann, de Hoog, Mazzaglia, Friedmann & Onofri, Studies in Mycology 51: 21 (2005)
Cryomyces montanus Isola & Zucconi, Fungal Diversity 86: 123 (2017)
Taxon synonyms: Cryomyces montanus Isola & Zucconi, Fungal Diversity 65 (1): 177 (2013) Invalid Art. 40.6
Phaeosclera Sigler, Tsuneda & J.W. Carmich., Mycotaxon 12 (2): 461 (1981)
Rupestriomyces L. Su, L.Y. Guo & X.Z. Liu, Mycologia 107 (4): 839 (2015)
Rupestriomyces ampulliformis L. Su, L.Y. Guo & X.Z. Liu, Mycologia 107 (4): 841 (2015)
Rupestriomyces sinensis L. Su, L.Y. Guo & X.Z. Liu, Mycologia 107 (4): 840 (2015)
Rupestriomyces torulosus L. Su, L.Y. Guo & X.Z. Liu, Mycologia 107 (4): 840 (2015)
Saxomyces Selbmann & Isola, Fungal Diversity 86: 422 (2017)
Taxon synonyms: Saxomyces Selbmann & Isola, Fungal Diversity 65 (1): 174 (2013) Invalid Art. 40.1
Saxomyces alpinus Zucconi & Selbmann, Fungal Diversity 86: 422 (2017)
Taxon synonyms: Saxomyces alpinus Zucconi & Selbmann, Fungal Diversity 65 (1): 174 (2013) Invalid Art. 40.6
Saxomyces penninicus Zucconi & Onofri, Fungal Diversity 86: 422 (2017)
Taxon synonyms: Saxomyces penninicus Zucconi & Onofri, Fungal Diversity 65 (1): 175 (2013) Invalid Art. 40.6
Spissiomyces Lei Su, Li Y. Guo & Xing Z. Liu, Mycologia 107 (4): 837 (2015)
Spissiomyces aggregatus Lei Su, Li Y. Guo & Xing Z. Liu, Mycologia 107 (4): 838 (2015)
Spissiomyces ramosus Lei Su, Li Y. Guo & Xing Z. Liu, Mycologia 107 (4): 838 (2015)
Coniosporiales
Coniosporiaceae
Coniosporium Link, Magazin der Gesellschaft Naturforschenden Freunde Berlin 3 (1): 8 (1809)
Taxon synonyms: Conisporium Link, Magazin der Gesellschaft Naturforschenden Freunde Berlin 3 (1): 8 (1809) Orthographic variant
Coniosporium apollinis Sterfl., Antonie van Leeuwenhoek 72 (4): 358 (1997)
Coniosporium sümbülii Sert & Sterflinger, Mycological Progress 9 (3): 356 (2010)
Coniosporium uncinatum De Leo, Urzì & de Hoog, Studies in Mycology 43: 75 (1999)
Dothideomycetidae
Capnodiales
incertae sedis
Arthrocatena Egidi & Selbmann, Fungal Systematics and Evolution 3: 126 (2019)
Taxon synonyms: Arthrocatena Egidi & Selbmann, Fungal Diversity 65: 159 (2014) Invalid Art. 40.7 (Shenzhen)
Arthrocatena tenebrosa Egidi & Selbmann, Fungal Systematics and Evolution 3: 126 (2019)
Taxon synonyms: Arthrocatena tenebrio Egidi & Selbmann, Fungal Diversity 65: 159 (2014) Invalid Art. 40.7 (Shenzhen)
Capnobotryella Sugiy., Pleomorphic Fungi: The Diversity and its Taxonomic Implications (Tokyo): 148 (1987)
Capnobotryella antalyensis Sert & Sterflinger, Mycological Research 111 (10): 1237 (2007)
Capnobotryella renispora Sugiy., Two metacapnodiaceous sooty moulds from Japan: their identity and behaviour in pure culture: 148 (1987)
Capnobotryella erdogani
Capnobotryella isiloglui
Capnobotryella kiziroglui
Catenulomyces Egidi & de Hoog, Fungal Systematics and Evolution 3: 126 (2019)
Taxon synonyms: Catenulomyces Egidi & de Hoog, Fungal Diversity 65: 154 (2014) Invalid Art. 40.7 (Shenzhen)
Catenulomyces convolutus Egidi & de Hoog, Fungal Systematics and Evolution 3: 126 (2019)
Taxon synonyms: Catenulomyces convolutus Egidi & de Hoog, Fungal Diversity 65: 154 (2014) Invalid Art. 40.7 (Shenzhen)
Constantinomyces Egidi & Onofri, Fungal Systematics and Evolution 3: 126 (2019)
Taxon synonyms: Constantinomyces Egidi & Onofri, Fungal Diversity 65: 155 (2014) Invalid Art. 40.7 (Shenzhen)
Constantinomyces macerans de Hoog & Onofri, Fungal Systematics and Evolution 3: 126 (2019)
Taxon synonyms: Constantinomyces macerans de Hoog & Onofri, Fungal Diversity 65: 157 (2014) Invalid Art. 40.7 (Shenzhen)
Constantinomyces minimus de Hoog & Isola, Fungal Systematics and Evolution 3: 126 (2019)
Taxon synonyms: Constantinomyces minimus de Hoog & Isola, Fungal Diversity 65: 157 (2014) Invalid Art. 40.7 (Shenzhen)
Constantinomyces nebulosus Isola & Zucconi, Fungal Systematics and Evolution 3: 126 (2019)
Taxon synonyms: Constantinomyces nebulosus Isola & Zucconi, Fungal Diversity 65: 157 (2014) Invalid Art. 40.7 (Shenzhen)
Constantinomyces oldenburgensis Gorbushina, P.M. Martin-Sanchez, Ruibal & Selbmann, Life 8 (3/30): 8 (2018) Invalid Art. 40.7 (Shenzhen)
Constantinomyces patonensis Ruibal & Selbmann, Life 8 (3/30): 11 (2018) Invalid Art. 40.7 (Shenzhen)
Constantinomyces virgultus Egidi & Onofri, Fungal Systematics and Evolution 3: 127 (2019)
Taxon synonyms: Constantinomyces virgultus Egidi & Onofri, Fungal Diversity 65: 155 (2014) Invalid Art. 40.7 (Shenzhen)
Elasticomyces Zucconi & Selbmann, Studies in Mycology 61: 11 (2008)
Elasticomyces elasticus Zucconi & Selbmann, Studies in Mycology 61: 11 (2008)
Friedmanniomyces Onofri, Nova Hedwigia 68: 176 (1999)
Friedmanniomyces endolithicus Onofri, Nova Hedwigia 68: 177 (1999)
Friedmanniomyces simplex Selbmann, de Hoog, Mazzaglia, Friedmann & Onofri, Studies in Mycology 51: 16 (2005)
Hyphoconis Egidi & Quaedvl., Fungal Systematics and Evolution 3: 127 (2019)
Taxon synonyms: Hyphoconis Egidi & Quaedvl., Fungal Diversity 65: 153 (2014) Invalid Art. 40.7 (Shenzhen)
Hyphoconis sterilis Egidi & Quaedvl., Fungal Systematics and Evolution 3: 127 (2019)
Taxon synonyms: Hyphoconis sterilis Egidi & Quaedvl., Fungal Diversity 65: 153 (2014) Invalid Art. 40.7 (Shenzhen)
Incertomyces Egidi & Zucconi, Fungal Systematics and Evolution 3: 127 (2019)
Taxon synonyms: Incertomyces Egidi & Zucconi, Fungal Diversity 65: 157 (2014) Invalid Art. 40.7 (Shenzhen)
Incertomyces perditus Egidi & Zucconi, Fungal Systematics and Evolution 3: 127 (2019)
Taxon synonyms: Incertomyces perditus Egidi & Zucconi, Fungal Diversity 65: 157 (2014) Invalid Art. 40.7 (Shenzhen)
Incertomyces vagans Egidi & Selbmann, Fungal Diversity 65: 157 (2014)
Lapidomyces de Hoog & Stielow, Fungal Systematics and Evolution 3: 128 (2019)
Taxon synonyms: Lapidomyces de Hoog & Stielow, Fungal Diversity 65: 159 (2014) Invalid Art. 40.7 (Shenzhen)
Lapidomyces hispanicus de Hoog & Stielow, Fungal Systematics and Evolution 3: 128 (2019)
Taxon synonyms: Lapidomyces hispanicus de Hoog & Stielow, Fungal Diversity 65: 159 (2014) Invalid Art. 40.7 (Shenzhen)
Meristemomyces Isola & Onofri, Fungal Systematics and Evolution 3: 128 (2019)
Taxon synonyms: Meristemomyces Isola & Onofri, Fungal Diversity 65: 158 (2014) Invalid Art. 40.1, see Arts 40.3 and Arts 6.3, 12.1 (Melbourne)
Meristemomyces frigidus Isola & Onofri, Fungal Systematics and Evolution 3: 129 (2019)
Taxon synonyms: Meristemomyces frigidus Isola & Onofri, Fungal Systematics and Evolution 3: 129 (2019) Invalid Art. 40.7 (Shenzhen)
Monticola Selbmann & Egidi, Fungal Systematics and Evolution 3: 128 (2019)
Taxon synonyms: Monticola Selbmann & Egidi, Fungal Diversity 65: 155 (2014) Invalid Art. 40.1, see Arts 40.3 and Arts 6.3, 12.1 (Melbourne)
Monticola elongata Selbmann & Egidi, Fungal Systematics and Evolution 3: 128 (2019)
Taxon synonyms: Monticola elongata Selbmann & Egidi, Fungal Diversity 65: 155 (2014) Invalid Art. 40.7 (Melbourne)
Oleoguttula Selbmann & de Hoog, Fungal Systematics and Evolution 3: 129 (2019)
Taxon synonyms: Oleoguttula Selbmann & de Hoog, Fungal Diversity 65: 152 (2014) Invalid Art. 40.1, see Arts 40.3 and Arts 6.3, 12.1 (Melbourne)
Oleoguttula mirabilis Selbmann & de Hoog, Fungal Systematics and Evolution 3: 129 (2019)
Taxon synonyms: Oleoguttula mirabilis Selbmann & de Hoog, Fungal Systematics and Evolution 3: 129 (2019) Invalid Art. 40.7 (Shenzhen)
Penidiella Crous & U. Braun, Studies in Mycology 58: 17 (2007)
Penidiella ellipsoidea Crous, Persoonia 26: 78 (2011)
Perusta Egidi & Stielow, Fungal Systematics and Evolution 3: 130 (2019)
Taxon synonyms: Perusta Egidi & Stielow, Fungal Diversity 65: 155 (2014) Invalid Art. 40.1 (Shenzhen)
Perusta inaequalis Egidi & Stielow, Fungal Systematics and Evolution 3: 130 (2019)
Taxon synonyms: Perusta inaequalis Egidi & Stielow, Fungal Diversity 65: 155 (2014) Invalid Art. 40.7 (Shenzhen)
Petrophila de Hoog & Quaedvl., Fungal Systematics and Evolution 3: 130 (2019)
Taxon synonyms: Petrophila de Hoog & Quaedvl., Fungal Diversity 65: 152 (2014) Invalid Art. 40.1, see Arts 40.3 and Arts 6.3, 12.1 (Shenzhen)
Petrophila incerta de Hoog & Quaedvl., Fungal Systematics and Evolution 3: 130 (2019)
Taxon synonyms: Petrophila incerta de Hoog & Quaedvl., Fungal Diversity 65: 152 (2014) Invalid Art. 40.7 (Shenzhen)
Pseudotaeniolina J.L. Crane & Schokn., Mycologia 78 (1): 88 (1986)
Pseudotaeniolina globosa De Leo, Urzì & de Hoog, Antonie van Leeuwenhoek 83 (4): 356 (2003)
Ramimonilia Stielow & Quaedvl., Fungal Systematics and Evolution 3: 130 (2019)
Taxon synonyms: Ramimonilia Stielow & Quaedvl., Fungal Diversity 65: 155 (2014) Invalid Art. 40.1, see Arts 40.3 and Arts 6.3, 12.1 (Shenzhen)
Ramimonilia apicalis Stielow & Quaedvl., Fungal Systematics and Evolution 3: 131 (2019)
Taxon synonyms: Ramimonilia apicalis Stielow & Quaedvl., Fungal Diversity 65: 155 (2014) Invalid Art. 40.7 (Shenzhen)
Recurvomyces Selbmann & de Hoog, Studies in Mycology 61: 10 (2008)
Recurvomyces mirabilis Selbmann & de Hoog, Studies in Mycology 61: 11 (2008)
Saxophila Selbmann & de Hoog, Fungal Systematics and Evolution 3: 131 (2019)
Taxon synonyms: Saxophila Selbmann & de Hoog, Fungal Diversity 76: 90 (2015) Invalid Art. 40.1 (Shenzhen)
Saxophila tyrrhenica Selbmann & de Hoog, Fungal Systematics and Evolution 3: 131 (2019)
Taxon synonyms: Saxophila tyrrhenica Selbmann & de Hoog, Fungal Diversity 76: 90 (2015) Invalid Art. 40.7 (Shenzhen)
Vermiconidia Egidi & Onofri, Fungal Systematics and Evolution 3: 131 (2019)
Taxon synonyms: Vermiconia Egidi & Onofri, Fungal Diversity 65: 150 (2014) Invalid Art. 40.1, see Arts 40.3 and Arts 6.3, 12.1 (Shenzhen)
Vermiconidia antarctica Egidi & Selbmann, Fungal Systematics and Evolution 3: 132 (2019)
Taxon synonyms: Vermiconia antarctica Egidi & Selbmann, Fungal Diversity 65: 152 (2014) Invalid Art. 40.7 (Shenzhen)
Vermiconidia calcicola de Hoog & Onofri, Fungal Systematics and Evolution 3: 132 (2019)
Taxon synonyms: Vermiconia calcicola de Hoog & Onofri, Fungal Diversity 76: 90 (2015) Invalid Art. 40.7 (Shenzhen)
Vermiconidia flagrans Selbmann & Isola, Fungal Systematics and Evolution 3: 132 (2019)
Taxon synonyms: Vermiconia flagrans Selbmann & Isola, Fungal Diversity 65: 152 (2014) Invalid Art. 40.7 (Shenzhen)
Vermiconidia foris Egidi & Onofri, Fungal Systematics and Evolution 3: 132 (2019)
Taxon synonyms: Vermiconia foris Egidi & Onofri, Fungal Diversity 65: 150 (2014) Invalid Art. 40.7 (Shenzhen)
Aeminiaceae
Aeminiaceae J. Trovão, I. Tiago & A. Portugal, MycoKeys 45: 62 (2019)
Aeminium J. Trovão, I. Tiago & A. Portugal, MycoKeys 45: 64 (2019)
Aeminium ludgeri J. Trovão, I. Tiago & A. Portugal, MycoKeys 45: 64 (2019)
CapnodiaceaeLeptoxyphium Speg., Physis Revista de la Sociedad Argentina de Ciencias Naturales 4 (17): 294 (1918)
Taxon synonyms: Astragoxyphium Bat., Nascim. & Cif., Quaderno del Laboratorio Crittogamico del Istituto Botanico dell’Università di Pavia 31: 45 (1963)
Taxon synonyms: Megaloxyphium Cif., Bat. & Nascim., Publicações do Instituto de Micologia da Universidade do Recife 47: 3 (1956)
ParadevriesiaceaeParadevriesia Crous, Fungal Systematics and Evolution 3: 98 (2019)
Paradevriesia compacta Crous, Fungal Systematics and Evolution 3: 129 (2019)
Taxon synonyms: Devriesia compacta de Hoog & Quaedvl., Fungal Diversity 65: 148 (2014) Invalid Art. 40.7 (Shenzhen)
TeratosphaeriaceaeAcrodontium de Hoog, Studies in Mycology 1: 23 (1972)
Acrodontium crateriforme (J.F.H. Beyma) de Hoog, Studies in Mycology 1: 26 (1972)
Basionym: Chloridium crateriforme J.F.H. Beyma, Zentralblatt für Bakteriologie und Parasitenkunde, Abteilung 2 89: 241 (1933)
Obligate synonyms: Tritirachium crateriforme (J.F.H. Beyma) Matsush., Icones Microfungorum a Matsushima lectorum: 160 (1975)
Austroafricana Quaedvl. & Crous, Persoonia 33: 25 (2014)
Austroafricana parva (R.F. Park & Keane) Quaedvl. & Crous, Persoonia 33: 25 (2014)
Basionym: Mycosphaerella parva R.F. Park & Keane, Transactions of the British Mycological Society 79 (1): 99 (1982)
Obligate synonyms: Teratosphaeria parva (R.F. Park & Keane) Crous & U. Braun, Studies in Mycology 58: 10 (2007)
Taxon synonyms: Mycosphaerella grandis Carnegie & Keane, Mycological Research 98: 414 (1994)
Catenulostroma Crous & U. Braun, Studies in Mycology 58: 13 (2007)
Catenulostroma protearum (Crous & M.E. Palm) Crous & U. Braun, Studies in Mycology 58: 17 (2007)
Basionym: Trimmatostroma protearum Crous & M.E. Palm, Mycological Research 103 (10): 1303 (1999)
Hortaea Nishim. & Miyaji, Japanese Journal of Medical Mycology 26 (2): 145 (1984)
Hortaea thailandica Crous & K.D. Hyde, Studies in Mycology 64: 39 (2009)
Hortaea werneckii (Horta) Nishim. & Miyaji, Japanese Journal of Medical Mycology 26 (2): 145 (1984)
Basionym: Cladosporium werneckii Horta, Revista Med. Cirurgía Brasil 29: 274 (1921)
Obligate synonyms: Exophiala werneckii (Horta) Arx, The genera of fungi sporulating in pure culture: 180 (1970)
Obligate synonyms: Pullularia werneckii (Horta) G.A. de Vries, Contribution to the knowledge of the genus Cladosporium: 101 (1952)
Obligate synonyms: Phaeoannellomyces werneckii (Horta) McGinnis & Schell, Sabouraudia 23: 184 (1979)
Obligate synonyms: Dematium werneckii (Horta) C.W. Dodge, Medical mycology. Fungous diseases of men and other mammals: 676 (1935)
Taxon synonyms: Cladosporium rietmanni Sartory, Rev. Pat. Malad. Pays Chauds: 9–44 (1935)
Taxon synonyms: Pullularia fermentans var. leaoi E.S. Wynne & Gott, Journal of General Microbiology 14: 517 (1956)
Taxon synonyms: Cryptococcus metaniger Castell., Archives of Dermatology and Syphilology 16 (4): 402 (1927)
Taxon synonyms: Cladosporium metaniger (Castell.) Ferrari, Atti dell’Istituto Botanico della Università e Laboratorio Crittogamico di Pavia 3: 183 (1932)
Taxon synonyms: Pullularia fermentans var. castellanii E.S. Wynne & Gott, Journal of General Microbiology 14: 518 (1956)
Taxon synonyms: Circinotrichum metaniger (Castell.) M. Ota, C.R. Soc. Biol. Paris: 1187 (1936)
Neocatenulostroma Quaedvl. & Crous, Persoonia 33: 26 (2014)
Neocatenulostroma abietis (Butin & Pehl) Quaedvl. & Crous, Persoonia 33: 27 (2014)
Basionym: Trimmatostroma abietis Butin & Pehl, Antonie van Leeuwenhoek 69 (3): 204 (1996)
Obligate synonyms: Catenulostroma abietis (Butin & Pehl) Crous & U. Braun, Studies in Mycology 58: 15 (2007)
Cladosporiales
Cladosporiaceae
Rachicladosporium Crous, U. Braun & C.F. Hill, Studies in Mycology 58: 38 (2007)
Rachicladosporium alpinum Egidi & Zucconi, Fungal Systematics and Evolution 3: 130 (2019)
Taxon synonyms: Rachicladosporium alpinum Egidi & Zucconi, Fungal Diversity 65: 159 (2014) Invalid Art. 40.7 (Shenzhen)
Rachicladosporium antarcticum Onofri & Egidi, Fungal Diversity 65: 162 (2014)
Rachicladosporium inconspicuum de Hoog & Stielow, Fungal Systematics and Evolution 3: 130 (2019)
Taxon synonyms: Rachicladosporium inconspicuum de Hoog & Stielow, Fungal Diversity 65: 162 (2014) Invalid Art. 40.7 (Shenzhen)
Rachicladosporium mcmurdoi Selbmann & Onofri, Fungal Systematics and Evolution 3: 130 (2019)
Taxon synonyms: Rachicladosporium mcmurdoi Selbmann & Onofri, Fungal Diversity 65: 159 (2014) Invalid Art. 40.7 (Shenzhen)
Rachicladosporium monterosanum Isola & Zucconi, Fungal Systematics and Evolution 3: 130 (2019)
Taxon synonyms: Rachicladosporium monterosium Isola & Zucconi, Fungal Diversity 65: 161 (2014) Invalid Art. 40.7 (Shenzhen)
Rachicladosporium paucitum Isola & Egidi, Fungal Systematics and Evolution 3: 130 (2019)
Taxon synonyms: Rachicladosporium paucitum Isola & Egidi, Fungal Diversity 65: 162 (2014) Invalid Art. 40.7 (Shenzhen)
Verrucocladosporium K. Schub., Aptroot & Crous, Studies in Mycology 58: 41 (2007)
Verrucocladosporium dirinae K. Schub., Aptroot & Crous, Studies in Mycology 58: 41 (2007)
Dothideales
Dothioraceae
Aureobasidium Viala & G. Boyer, Revue Génerale de Botanique 3: 371 (1891)
Obligate synonyms: Aureobasis Clem. & Shear, The genera of Fungi: 343, 381 (1931)
Obligate synonyms: Chrysobasidium Clem., The genera of Fungi: 107 (1909)
Taxon synonyms: Pullularia Berkhout, De schimmelgeslachten Monilia, Oidium, Oospora en Torula: 55, 64 (1923)
Taxon synonyms: Dematoidium Stautz, Phytopathologische Zeitschrift 3: 204 (1931)
Taxon synonyms: Pachybasidiella Bubák & Syd., Annales Mycologici 13 (1): 9 (1915)
Taxon synonyms: Protocoronospora G.F. Atk. & Edgerton, Journal of Mycology 13 (5): 186 (1907)
Taxon synonyms: Protocoronis Clem. & Shear, The genera of Fungi: 197, 344 (1931)
Taxon synonyms: Dematoideum Stautz (1931)
Aureobasidium pullulans (de Bary) G. Arnaud, Annales de l’École Nationale d’Agriculture de Montpellier 16 (1–4): 39 (1918)
Basionym: Dematium pullulans de Bary, Vergleichende Morphologie und Biologie der Pilze Mycetozoen und Bacterien: 182 (1884) [MB#219317]
Obligate synonyms: Anthostomella pullulans (de Bary) F.T. Benn., Annals of Applied Biology 15: 381 (1928)
Obligate synonyms: Pullularia pullulans (de Bary) Berkhout, De schimmelgeslachten Monilia, Oidium, Oospora en Torula: 55 (1923)
Obligate synonyms: Hormonema pullulans (de Bary) Lagerb. & Melin, Nytt Magazin for Naturvidenskapene 71: 256 (1932)
Obligate synonyms: Cladosporium pullulans (de Bary) Sacc. & Trotter, Sylloge Fungorum 22: 1250 (1913)
Taxon synonyms: Aureobasidium vitis Viala & G. Boyer, Revue Génerale de Botanique 3: 371 (1891)
Taxon synonyms: Exobasidium vitis (Viala & G. Boyer) Prill. & Delacr. (1894) Taxon synonyms: Taxon synonyms: Chrysobasidium vitis (Viala & G. Boyer) Clem., The genera of Fungi: 107 (1909)
Taxon synonyms: Aureobasis vitis (Viala & G. Boyer) Clem. & Shear, The genera of Fungi: 343, 381 (1931)
Taxon synonyms: Dematoidium nigrescens Stautz, Phytopathologische Zeitschrift 3: 204 (1931)
Taxon synonyms: Phymatotrichum baccarum Oudem., Verslag Verg. Afd. Natuurkunde KNAW: 392 (1900)
Taxon synonyms: Aureobasidium pullulans (De Bary) G. Arnaud ex Cif., Ribaldi & Corte, Atti dell’Istituto Botanico della Università e Laboratorio Crittogamico di Pavia 14: 85 (1957)
Endoconidioma Tsuneda, Hambl. & Currah, Mycologia 96 (5): 1129 (2004)
Taxon synonyms: Coniozyma Crous, CBS Biodiversity Series 7: 97 (2008)
Hormonema Lagerb. & Melin, Svenska Skogsvårdsföreningens Tidskrift 2 (2–4): 219 (1927)
Hormonema carpetanum Bills, Peláez & Ruibal, Studies in Mycology 50 (1): 152 (2004)
Pringsheimia Schulzer, Verhandlungen der Zoologisch-Botanischen Gesellschaft Wien 16: 57 (1866)
Pringsheimia smilacis E. Müll., Sydowia 11: 458 (1957)
Mycosphaerellales
Extremaceae
Extremus Quaedvl. & Crous, Fungal Systematics and Evolution 3: 127 (2019)
Taxon synonyms: Extremus Quaedvl. & Crous, Persoonia 33: 21 (2014) Invalid Art. 40.7 (Shenzhen)
Extremus adstrictus Quaedvl. & Crous, Fungal Systematics and Evolution 3: 127 (2019)
Taxon synonyms: Extremus adstrictus Egidi & Onofri ex Quaedvl. & Crous, Persoonia 33: 22 (2014) Invalid Art. 40.7 (Shenzhen)
Taxon synonyms: Devriesia adstricta Egidi & Onofri, Fungal Diversity 65: 150 (2014)
Extremus antarcticus Quaedvl. & Crous, Fungal Systematics and Evolution 3: 127 (2019)
Taxon synonyms: Extremus antarcticus Selbmann & de Hoog ex Quaedvl. & Crous, Persoonia 33: 22 (2014) Invalid Art. 40.7 (Shenzhen)
Taxon synonyms: Devriesia antarctica Selbmann & de Hoog, Fungal Diversity 65: 150 (2014)
Mycosphaerellaceae
Pseudocercospora Speg., Anales del Museo Nacional de Historia Natural Buenos Aires ser. 3, 13: 438 (1911)
Taxon synonyms: Stigmina Sacc., Michelia 2 (6): 22 (1880)
Taxon synonyms: Ciferriella Petr., Annales Mycologici 28 (5–6): 409 (1930)
Taxon synonyms: Ancylospora Sawada, Report of the Department of Agriculture Government Research Institute of Formosa 87: 77 (1944)
Taxon synonyms: Cercocladospora G.P. Agarwal & S.M. Singh, Proc. natn. Acad. Sci. India, Sect. B, Biol. Sci.: 439 (1974)
Taxon synonyms: Cercosporiopsis Miura, Flora of Manchuria and East Mongolia. Part III. Cryptogams, fungi 3: 527–528 (1928)
Taxon synonyms: Helicomina L.S. Olive, Mycologia 40 (1): 16 (1948)
Taxon synonyms: Pseudocercospora sect. Helicomina (L.S. Olive) U. Braun, A monograph of Cercosporella, Ramularia and allied genera (phytopathogenic Hyphomycetes) 2: 398 (1998)
Taxon synonyms: Jaczewskiella Murashk., Mater. Mikol. Fitopat. Ross.: 5 (1926)
Taxon synonyms: Marcosia Syd. & P. Syd., Annales Mycologici 14 (1–2): 96 (1916)
Taxon synonyms: Pseudopuccinia Höhn., Mitt. bot. Inst. tech. Hochsch. Wien: 41 (1925)
Taxon synonyms: Semipseudocercospora J.M. Yen, Mycotaxon 17: 361 (1983)
Taxon synonyms: Pseudocercospora sect. Cercocladospora G.P. Agarwal & S.M. Singh ex U. Braun, A monograph of Cercosporella, Ramularia and allied genera (phytopathogenic Hyphomycetes) 2: 397 (1998)
Taxon synonyms: Neopseudocercospora Crous, Persoonia 31: 219 (2013)
NeodevriesiaceaeNeodevriesia Quaedvl. & Crous, Persoonia 33: 24 (2014)
Neodevriesia bulbillosa Egidi & Zucconi, Fungal Systematics and Evolution 3: 129 (2019)
Taxon synonyms: Neodevriesia bulbillosa E. Egidi & Zucconi ex Crous, Sydowia 67: 108 (2015) Invalid Art. 40.7 (Shenzhen)
Taxon synonyms: Devriesia bulbillosa Egidi & Zucconi, Fungal Diversity 65: 148 (2014) Invalid Art. 40.7 (Shenzhen)
Neodevriesia capensis (Crous) Crous, Sydowia 67: 108 (2015)
Basionym: Teratosphaeria capensis Crous, Persoonia 27: 38 (2011)
Neodevriesia lagerstroemiae (Crous & M.J. Wingf.) Crous, Sydowia 67: 108 (2015)
Basionym: Devriesia lagerstroemiae Crous & M.J. Wingf., Studies in Mycology 64: 38 (2009)
Neodevriesia modesta Isola & Zucconi, Fungal Systematics and Evolution 3: 129 (2019)
Taxon synonyms: Neodevriesia modesta Isola & Zucconi ex Crous, Sydowia 67: 108 (2015) Invalid Art. 40.7 (Shenzhen)
Taxon synonyms: Devriesia modesta Isola & Zucconi, Fungal Diversity 65: 148 (2014) Invalid Art. 40.7 (Shenzhen)
Neodevriesia sardiniae Isola & de Hoog, Fungal Systematics and Evolution 3: 129 (2019)
Taxon synonyms: Neodevriesia sardiniae D. Isola & de Hoog ex M.M. Wang & L. Cai, Mycologia 109 (6): 972 (2017) Invalid Art. 40.7 (Shenzhen)
Taxon synonyms: Devriesia sardiniae Isola & de Hoog, Fungal Diversity 76: 85 (2015) Invalid Art. 40.7 (Shenzhen)
Neodevriesia simplex Selbmann & Zucconi, Fungal Systematics and Evolution 3: 129 (2019)
Taxon synonyms: Neodevriesia simplex Selbmann & Zucconi ex Crous, Sydowia 67: 108 (2015) Invalid Art. 40.7 (Shenzhen)
Taxon synonyms: Devriesia simplex Selbmann & Zucconi, Fungal Diversity 65: 148 (2014) Invalid Art. 40.7 (Shenzhen)
Neophaeothecales
NeophaeothecaceaeNeophaeotheca Abdollahz. & Crous, Studies in Mycology 95: 392 (2020)
Neophaeotheca triangularis (de Hoog & Beguin) Abdollahz. &Crous, Studies in Mycology 95: 392 (2020)
Basionym: Phaeotheca triangularis de Hoog & Beguin, Antonie van Leeuwenhoek 71 (3): 290 (1997)
Phaeothecales
PhaeothecaceaePhaeotheca Sigler, Tsuneda & J.W. Carmich., Mycotaxon 12 (2): 450 (1981)
Pleosporomycetidae
Venturiales
Sympoventuriaceae
Ochroconis de Hoog & Arx, Kavaka 1: 57 (1973)
Ochroconis anomala A. Nováková & P.M. Martin-Sanchez, Fungal Biology 116 (5): 584 (2012)
Obligate synonyms: Scolecobasidium anomalum (A. Nováková & P.M. Martin-Sanchez) G.Y. Sun & Lu Hao, Fungal Biology 12: 491 (2013)
Ochroconis lascauxensis A. Nováková & P.M. Martin-Sanchez, Fungal Biology 116 (5): 580 (2012)
Obligate synonyms: Scolecobasidium lascauxense (A. Nováková & P.M. Martin-Sanchez) G.Y. Sun & Lu Hao, Fungal Biology 12: 492 (2013)
Eurotiomycetes
incertae sedis
Sarcinomyces Lindner, Mikroskopische Betriebskontrolle in den Gährungsgewerben: 228 (1898)
Sarcinomyces sideticae Sert & Sterfl., Botanical Journal of the Linnean Society 154 (3): 379 (2007) Invalid
Chaetothyriales
incertae sedis
Bacillicladium Hubka, Réblová, Thureborn, PLoS One 11 (10): 14 (2016)
Bacillicladium lobatum Hubka, Réblová, Thureborn, PLoS One 11 (10): 17 (2016)
Bradymyces Hubka, Réblová, Selbmann & M. Kolařík, Antonie van Leeuwenhoek 106 (5): 983 (2014)
Bradymyces alpinus Hubka, Selbmann, Réblová & M. Kolařík, Antonie van Leeuwenhoek 106 (5): 985 (2014)
Bradymyces graniticola Hubka, Réblová & Thureborn, PLoS One 11 (10): e0163396, 19 (2016)
Bradymyces pullus L. Su, W. Sun & M.C. Xiang, Journal of Fungi 6 (4, no. 187): 15 (2020)
Bradymyces yunnanensis L. Su, W. Sun & M.C. Xiang, Journal of Fungi 6 (4, no. 187): 16 (2020)
Neophaeococcomyces Crous & M.J. Wingf., Persoonia 35: 287 (2015)
Neophaeococcomyces catenatus (de Hoog & Herm.-Nijh.) Crous & M.J. Wingf., Persoonia 35: 287 (2015)
Basionym: Phaeococcus catenatus de Hoog & Herm.-Nijh., Studies in Mycology 15: 126 (1977)
Taxon synonyms: Phaeococcomyces catenatus (de Hoog & Herm.-Nijh.) de Hoog, Taxon 28: 348 (1979)
Cyphellophoraceae
Cyphellophora G.A. de Vries, Mycopathologia et Mycologia Applicata 16: 47 (1962)
Cyphellophora botryose
Cyphellophora guizhouensis
Herpotrichiellaceae
Cladophialophora Borelli, Proceedings of the 5th International Conference on Mycoses: 355 (1980)
Cladophialophora humicola Crous & U. Braun, Studies in Mycology 58: 189 (2007)
Cladophialophora nyingchiensis W. Sun, L. Su, M.C. Xiang & Xing Z. Liu, Journal of Fungi 6 (4, no. 187): 26 (2020)
Cladophialophora tengchongensis W. Sun, L. Su, M.C. Xiang & X.Z. Liu, Journal of Fungi 6 (4, no. 187): 27 (2020)
Cladophialophora tumbae Kiyuna, K.D. An, R. Kigawa & Sugiy., Mycoscience 59 (1): 80 (2017)
Cladophialophora tumulicola Kiyuna, K.D. An, R. Kigawa & Sugiy., Mycoscience 59 (1): 81 (2017)
Exophiala J.W. Carmich., Sabouraudia 5 (1): 122 (1966)
Taxon synonyms: Wangiella McGinnis, Mycotaxon 5 (1): 354 (1977)
Taxon synonyms: Foxia Castell., Journal of Tropical Medicine and Hygiene 11: 261 (1908) Invalid nomen nudum
Exophiala angulospora Iwatsu, Udagawa & T. Takase, Mycotaxon 41 (2): 322 (1991)
Exophiala bonariae Isola & Zucconi, Fungal Systematics and Evolution 3: 127 (2019)
Taxon synonyms: Exophiala bonariae Isola & Zucconi, Fungal Diversity 76: 85 (2015) Invalid Art. 40.7 (Shenzhen)
Exophiala cinerea W. Sun, M.C. Xiang & Xing Z. Liu, Journal of Fungi 6 (4, no. 187): 28 (2020)
Exophiala clavispora W. Sun, M.C. Xiang & Xing Z. Liu, Journal of Fungi 6 (4, no. 187): 29 (2020)
Exophiala ellipsoidea W. Sun, L. Su, M.C. Xiang & X.Z. Liu, Journal of Fungi 6 (4, no. 187): 29 (2020)
Exophiala nagquensis W. Sun, L. Su, M.C. Xiang & X.Z. Liu, Journal of Fungi 6 (4, no. 187): 30 (2020)
Phaeococcomyces de Hoog, Taxon 28: 348 (1979)
Phaeococcomyces nigricans (M.A. Rich & A.M. Stern) de Hoog, Taxon 28: 348 (1979)
Basionym: Cryptococcus nigricans M.A. Rich & A.M. Stern, Mycopathologia et Mycologia Applicata 9: 191 (1958)
Obligate synonyms: Phaeococcus nigricans (M.A. Rich & A.M. Stern) de Hoog, Studies in Mycology 15: 125 (1977)
Obligate synonyms: Melanocryptococcus nigricans (M.A. Rich & A.M. Stern) Della Torre & Cif.: 9 (1964)
Obligate synonyms: Nigrococcus nigricans (M.A. Rich & A.M. Stern) Novák & Zsolt, Acta Botanica Academiae Scientiarum Hungarica 7: 142 (1961)
Phialophora Medlar, Mycologia 7 (4): 202 (1915)\
Rhinocladiella Nannf., Svenska Skogsvårdsföreningens Tidskrift 32: 461 (1934)
Taxon synonyms: Racodium Pers., Neues Magazin für die Botanik 1: 123 (1794)
Taxon synonyms: Phialoconidiophora M. Moore & F.P. Almeida, Annals of the Missouri Botanical Garden 23: 548 (1936)
Taxon synonyms: Carrionia Bric.-Irag., Rev. Clin. Luiz Razetti, (Caracas): 121 (1938)
Rhinocladiella atrovirens Nannf., Svenska Skogsvårdsföreningens Tidskrift 32: 462 (1934)
Taxon synonyms: Melanchlenus eumetabolus Calandron, Revue de Mycologie (Paris) 17: 190 (1953)
Taxon synonyms: Melanchlenus cumetabolus Calandron (1953)
Trichomeriaceae
Anthracina L. Su, W. Sun & M.C. Xiang, Journal of Fungi 6 (4, no. 187): 12 (2020)
Anthracina ramosa L. Su, W. Sun & M.C. Xiang, Journal of Fungi 6 (4, no. 187): 13 (2020)
Anthracina saxicola L. Su, W. Sun & M.C. Xiang, Journal of Fungi 6 (4, no. 187): 14 (2020)
Obligate synonyms: Anthracina saxincola L. Su, W. Sun & M.C. Xiang (2020) Orthographic variant
Lithohypha Selbmann & Isola, Fungal Diversity 86: 258 (2017)
Basionym: Lithophila Selbmann & Isola, Fungal Diversity 76: 88 (2015) Illegitimate Art. 40.1 (Melbourne); Art. 53.1, non Lithophila Sw. 1788 (Amaranthaceae)
Taxon synonyms: Lithophila Selbmann & Isola, Fungal Systematics and Evolution 3: 128 (2019) Illegitimate Art. 53.1, non Lithophila Sw. 1788 (Amaranthaceae)
Lithohypha catenulata L. Su, W. Sun & M.C. Xiang, Journal of Fungi 6 (4, no. 187): 20 (2020)
Lithohypha guttulata Selbmann & Isola, Fungal Diversity 86: 258 (2017)
Taxon synonyms: Lithophila guttulata Selbmann & Isola, Fungal Systematics and Evolution 3: 128 (2019) superfluous
Taxon synonyms: Lithophila guttulata Selbmann & Isola, Fungal Diversity 76: 90 (2015) invalid Art. 40.7 (Melbourne)
Trichomerium Speg., Physis Revista de la Sociedad Argentina de Ciencias Naturales 4 (17): 284 (1918)
Taxon synonyms: Capnobatista Cif. & F.B. Leal ex Bat. & Cif., Saccardoa 2: 75 (1963)
Taxon synonyms: Triposporiopsis W. Yamam., Pap. Dedic. Tochinai & Fukushi Commem. 60th Birthdays: 52–56 (1955)
Taxon synonyms: Paropodia Cif. & Bat., Publicações do Instituto de Micologia da Universidade do Recife 36: 5 (1956)
Trichomerium cicatricatum L. Su, W. Sun & M.C. Xiang, Journal of Fungi 6 (4, no. 187): 21 (2020)
Trichomerium flexuosum W. Sun, X.Z. Liu & M.C. Xiang, Journal of Fungi 6 (4, no. 187): 23 (2020)
Trichomerium lapideum L. Su, W. Sun & M.C. Xiang, Journal of Fungi 6 (4, no. 187): 24 (2020)
Trichomerium leigongense W. Sun, L. Su & M.C. Xiang, Journal of Fungi 6 (4, no. 187): 25 (2020)
Nontypical rock-inhabiting fungi
Ascomycota
Dothideomycetes
Cladosporiales
Cladosporiaceae
Cladosporium Link, Magazin der Gesellschaft Naturforschenden Freunde Berlin 7: 37 (1816)
Taxon synonyms: Heterosporium Klotzsch ex Cooke, Grevillea 5 (35): 122 (1877)
Taxon synonyms: Cladosporium subgen. Heterosporium (Klotzsch ex Cooke) J.C. David, Mycological Papers 172: 29 (1997)
Taxon synonyms: Beejadwaya Subram., Kavaka 5: 97 (1978)
Taxon synonyms: Acrosporella Riedl & Ershad, Sydowia 29 (1–6): 166 (1977)
Taxon synonyms: Azosma Corda, Deutschlands Flora, Abt. III. Die Pilze Deutschlands 3 (12): 35 (1831)
Taxon synonyms: Mydonosporium Corda, Deutschl. Flora, III (Pilze): 95 (1833)
Taxon synonyms: Myxocladium Corda, Icones fungorum hucusque cognitorum 1: 12 (1837)
Taxon synonyms: Polyrhizium Giard, Bulletin Scientifique de la France et de la Belgique 20: 217 (1889)
Taxon synonyms: Spadicesporium V.N. Boriss. & Dvoïnos, Novosti Sistematiki Nizshikh Rastenii 19: 35 (1982)
Taxon synonyms: Sporocladium Chevall., Flore Générale des Environs de Paris 1: 35 (1826)
Taxon synonyms: Davidiella Crous & U. Braun, Mycological Progress 2 (1): 8 (2003)
Pleosporomycetidae
Pleosporales
incertae sedis
Phoma Sacc., Michelia 2 (6): 4 (1880) [MB#9358]
Taxon synonyms
Chlamydosporium Peyronel, I germi astmosferici dei fungi con micelio: 18 (1913)
Leptophoma Höhn., Sitzungsberichte der Kaiserlichen Akademie der Wissenschaften Math.-naturw. Klasse Abt. I 124: 73 (1915)
Macroplodiella Speg., Anales del Museo Nacional de Historia Natural Buenos Aires 17: 134 (1908)
Paraphoma Morgan-Jones & J.F. White, Mycotaxon 18 (1): 58 (1983)
Phomopsina Petr., Annales Mycologici 20: 145 (1922)
Pseudosclerophoma Petr., Annales Mycologici 21 (3–4): 283 (1923)
Rhizosphaerella Höhn., Hedwigia 59: 254 (1917) [MB#9729]
Sclerophomina Höhn., Hedwigia 59: 240 (1917)
Vialina Curzi, Bolletino della Stazione di Patologia Vegetale di Roma 15: 252 (1935)
Peyronellaea Goid., Atti della Accademia Nazionale dei Lincei Sér. 8, 1: 451 (1946)
PericoniaceaePericonia Tode, Fungi Mecklenburgenses Selecti 2: 2 (1791)
Taxon synonyms
Harpocephalum G.F. Atk., Bulletin of the Cornell University (Science) 3 (1): 41 (1897)
Pachytrichum Syd., Annales Mycologici 23 (3–6): 420 (1925)
Sporodum Corda, Icones fungorum hucusque cognitorum 1: 18 (1837)
Trichocephalum Costantin, Revue agric. Sucr. Ile Maurice: 106 (1888)
PleosporaceaeAlternaria Nees, System der Pilze und Schwämme: 72 (1817)
Taxon synonyms
Embellisia E.G. Simmons, Mycologia 63: 380 (1971)
Alternaria sect. Embellisia Woudenb. & Crous, Studies in Mycology 75: 190 (2013)
Ulocladium Preuss, Linnaea 24: 111 (1851) [MB#10346]
Alternaria sect. Ulocladium Woudenb. & Crous, Studies in Mycology 75: 205 (2013)
Chmelia Svob.-Pol., Biológia Bratislava 21: 82 (1966) [MB#7626]
Macrosporium Fr., Systema Mycologicum 3: 373 (1832) [MB#8821]
Nimbya E.G. Simmons, Sydowia 41: 316 (1989) [MB#25376]
Alternaria sect. Nimbya Woudenb. & Crous, Studies in Mycology 75: 197 (2013)
Allewia E.G. Simmons, Mycotaxon 38: 260 (1990) [MB#25500]
Lewia M.E. Barr & E.G. Simmons, Mycotaxon 25 (1): 289 (1986)
Elosia Pers., Mycologia Europaea 1: 12 (1822)
Prathoda Subram., Journal of the Indian Botanical Society 35 (1): 73 (1956)
Rhopalidium Mont., Annales des Sciences Naturelles Botanique 6: 30 (1836)
Trichoconiella B.L. Jain, Kavaka 3: 39 (1976)
Crivellia Shoemaker & Inderbitzin, Canadian Journal of Botany 84 (8): 1308 (2006)
Alternaria sect. Crivellia Woudenb. & Crous, Studies in Mycology 75: 189 (2013)
Ybotromyces Rulamort, Bulletin de la Société Botanique du Centre-Ouest 17: 192 (1986)
Chalastospora E.G. Simmons, CBS Biodiversity Series 6: 668 (2007)
Alternaria sect. Chalastospora (E.G. Simmons) Woudenb. & Crous, Studies in Mycology 75: 188 (2013)
Teretispora E.G. Simmons, CBS Biodiversity Series 6: 674 (2007)
Alternaria sect. Teretispora Woudenb. & Crous, Studies in Mycology 75: 202 (2013)
Botryomyces de Hoog & C. Rubio, Sabouraudia 20: 19 (1982)
Brachycladium Corda, Icones fungorum hucusque cognitorum 2: 14 (1838)
Sinomyces Yong Wang bis & X.G. Zhang, Fungal Biology 115 (2): 192 (2011)
Undifilum B.M. Pryor, Creamer, Shoemaker, McLain-Romero & Hambl., Botany 87 (2): 190 (2009)
Alternaria sect. Undifilum Woudenb. & Crous, Studies in Mycology 75: 206 (2013)
Eurotiomycetes
Eurotiomycetidae
Eurotiales
Aspergillaceae
Aspergillus P. Micheli ex Haller, Historia stirpium indigenarum Helvetiae inchoata 3: 113 (1768)
Taxon synonyms
Acmosporium Corda, Icones fungorum hucusque cognitorum 3: 11 (1839)
Alliospora Pim, J. Bot., London: 234 (1883)
Basidiella Cooke, Grevillea 6 (39): 118 (1878)
Briarea Corda, Deutschlands Flora, Abt. III. Die Pilze Deutschlands 2–6: 11 (1831)
Cladaspergillus Ritgen, Schr. Marb. Ges.: 89 (1831)
Euaspergillus F. Ludw., Lehrbuch der Niederen Kryptogamen: 258 (1892)
Gutturomyces Rivolta, Dei Parassiti Vegetali: 579 (1873)
Raperia Subram. & Rajendran, Kavaka: 133 (1976)
Rhodocephalus Corda, Icones fungorum hucusque cognitorum 1: 21 (1837)
Rhopalocystis Grove, J. Econ. Biol.: 40 (1911)
Sceptromyces Corda, Deutschlands Flora, Abt. III. Die Pilze Deutschlands 3 (11): 7 (1831)
Aspergillus P. Micheli, Nova Plantarum Genera: 212, t. 92 (1729)
Penicillium Link, Magazin der Gesellschaft Naturforschenden Freunde Berlin 3 (1): 16 (1809)
Taxon synonyms
Torulomyces Delitsch, Ergebnisse der theoretischen und angewandten Mikrobiologie: Band I: Systematik der Schimmelpilze: 91 (1943)
Penicillium sect. Torulomyces (Delitsch) Stolk & Samson, Advances in Penicillium and Aspergillus Systematics: 169 (1986)
Floccaria Grev., Scott. crypt. fl.: pl. 301 (1827)
Moniliger Letell. (1839)
Pritzeliella Henn., Beiblatt zur Hedwigia 42: 88 (1903)
Walzia Sorokin, Trudy Obshchestva ispytatelei prirody pri Imperatorskom Khar’kovskom universitê: 47 (1871)
Disclosure statement
No potential conflict of interest was reported by the author(s).
Additional information
Funding
References
- Abdollahzadeh J, Groenewald J, Coetzee M, Wingfield M, Crous P. 2020. Evolution of lifestyles in Capnodiales. Stud Mycol. 95:381–414. doi:https://doi.org/10.1016/j.simyco.2020.02.004.
- Ametrano CG, Grewe F, Crous PW, Goodwin SB, Liang C, Selbmann L, Lumbsch HT, Leavitt SD, Muggia L. 2019. Genome-scale data resolve ancestral rock-inhabiting lifestyle in Dothideomycetes (Ascomycota). IMA Fungus. 10(1):1–12. doi:https://doi.org/10.1186/s43008-019-0018-2.
- Araújo M, Viveiros R, Correia TR, Correia IJ, Bonifácio VD, Casimiro T, Aguiar-Ricardo A. 2014. Natural melanin: a potential pH-responsive drug release device. Int J Pharm. 469(1):140–145. doi:https://doi.org/10.1016/j.ijpharm.2014.04.051.
- Ascaso C, Wierzchos J, de Los Ríos A. 1995. Cytological investigations of lithobiontic microorganisms in granitic rocks. Bot Acta. 108(6):474–481. doi:https://doi.org/10.1111/j.1438-8677.1995.tb00524.x.
- Bentis CJ, Kaufman L, Golubic S. 2000. Endolithic fungi in reef-building corals (Order: Scleractinia) are common, cosmopolitan, and potentially pathogenic. Biol Bull. 198(2):254–260. doi:https://doi.org/10.2307/1542528.
- Beraldi-Campesi H. 2013. Early life on land and the first terrestrial ecosystems. Ecol Processes. 2(1):1–17.
- Bogomolova E, Olkhovaya E, Panina L, Soukharjevsky S. 2003. Experimental study of influence of rocks and minerals chemical composition and surface structure over the lithobiontic fungi colonies morphology. Микология И Фитопатология. 37(3):1–13.
- Breitenbach R, Silbernagl D, Toepel J, Sturm H, Broughton WJ, Sassaki GL, Gorbushina AA. 2018. Corrosive extracellular polysaccharides of the rock-inhabiting model fungus Knufia petricola. Extremophiles. 22(2):165–175. doi:https://doi.org/10.1007/s00792-017-0984-5.
- Bruns TD, White TJ, Taylor JW. 1991. Fungal molecular systematics. Annu Rev Ecol Syst. 22(1):525–564. doi:https://doi.org/10.1146/annurev.es.22.110191.002521.
- Bryan R, Jiang Z, Friedman M, Dadachova E. 2011. The effects of gamma radiation, UV and visible light on ATP levels in yeast cells depend on cellular melanization. Fungal Biol. 115(10):945–949. doi:https://doi.org/10.1016/j.funbio.2011.04.003.
- Caneva G, Lombardozzi V, Ceschin S, Municchia AC, Salvadori O. 2014. Unusual differential erosion related to the presence of endolithic microorganisms (Martvili, Georgia). J Cult Heritage. 15(5):538–545. doi:https://doi.org/10.1016/j.culher.2013.10.003.
- Caretta G, Tosi S, Piontelli E, de Hoog G. 2006. Phialophora sessilis, a lithobiont fungus. Mycotaxon. 95:281–284.
- Casadevall A, Cordero RJ, Bryan R, Nosanchuk J, Dadachova E. 2017. Melanin, radiation, and energy transduction in fungi. Microbiol Spectr. 5(2):5.2.05. doi:https://doi.org/10.1128/microbiolspec.FUNK-0037-2016.
- Chertov O, Gorbushina A, Deventer B. 2004. A model for microcolonial fungi growth on rock surfaces. Ecol Modell. 177(3–4):415–426. doi:https://doi.org/10.1016/j.ecolmodel.2004.02.011.
- Cole BJ, Tringe SG. 2021. Different threats, same response. Nat Plants. 7(5):544–545. doi:https://doi.org/10.1038/s41477-021-00915-z.
- Coleine C, Masonjones S, Selbmann L, Zucconi L, Onofri S, Pacelli C, Stajich JE. 2017. Draft genome sequences of the Antarctic endolithic fungi Rachicladosporium antarcticum CCFEE 5527 and Rachicladosporium sp. CCFEE 5018. Genome Announc. 5(27):e00397–00317. doi:https://doi.org/10.1128/genomeA.00397-17.
- Coleine C, Masonjones S, Sterflinger K, Onofri S, Selbmann L, Stajich JE. 2020. Peculiar genomic traits in the stress-adapted cryptoendolithic Antarctic fungus Friedmanniomyces endolithicus. Fungal Biol. 124(5):458–467. doi:https://doi.org/10.1016/j.funbio.2020.01.005.
- Coleine C, Stajich JE, de Los Ríos A, Selbmann L. 2021. Beyond the extremes: rocks as ultimate refuge for fungi in drylands. Mycologia. 113(1):108–133. doi:https://doi.org/10.1080/00275514.2020.1816761.
- Coleine C, Stajich JE, Zucconi L, Onofri S, Pombubpa N, Egidi E, Franks A, Buzzini P, Selbmann L. 2018. Antarctic cryptoendolithic fungal communities are highly adapted and dominated by Lecanoromycetes and Dothideomycetes. Front Microbiol. 9:1392. doi:https://doi.org/10.3389/fmicb.2018.01392.
- Cooney DG, Emerson R. 1964. Thermophilic fungi. Vol. 27. San Francisco: WH Freeman.
- Cordero RJ, Casadevall A. 2017. Functions of fungal melanin beyond virulence. Fungal Biol Rev. 31(2):99–112. doi:https://doi.org/10.1016/j.fbr.2016.12.003.
- Culka A, Jehlička J, Ascaso C, Artieda O, Casero CM, Wierzchos J. 2017. Raman microspectrometric study of pigments in melanized fungi from the hyperarid Atacama desert gypsum crust. J Raman Spectrosc. 48(11):1487–1493. doi:https://doi.org/10.1002/jrs.5137.
- Cunha MM, Franzen AJ, Seabra SH, Herbst MH, Vugman NV, Borba LP, de Souza W, Rozental S. 2010. Melanin in Fonsecaea pedrosoi: a trap for oxidative radicals. BMC Microbiol. 10(1):1–9. doi:https://doi.org/10.1186/1471-2180-10-80.
- Cuscó A, Catozzi C, Viñes J, Sanchez A, Francino O. 2018. Microbiota profiling with long amplicons using Nanopore sequencing: full-length 16S rRNA gene and the 16S-ITS-23S of the rrn operon. F1000Research. 7:1755. doi:https://doi.org/10.12688/f1000research.16817.1.
- Dadachova E, Bryan RA, Huang X, Moadel T, Schweitzer AD, Aisen P, Nosanchuk JD, Casadevall A. 2007. Ionizing radiation changes the electronic properties of melanin and enhances the growth of melanized fungi. PLoS One. 2(5):e457. doi:https://doi.org/10.1371/journal.pone.0000457.
- Dadachova E, Casadevall A. 2008. Ionizing radiation: how fungi cope, adapt, and exploit with the help of melanin. Curr Opin Microbiol. 11(6):525–531. doi:https://doi.org/10.1016/j.mib.2008.09.013.
- de Hoog GS, Hermanides-Nijhof E. 1977. The black yeasts and allied Hyphomycetes. Stud Mycol. 15:1–222.
- de Leo F, Antonelli F, Pietrini AM, Ricci S, Urzì C. 2019. Study of the euendolithic activity of black meristematic fungi isolated from a marble statue in the Quirinale Palace’s Gardens in Rome, Italy. Facies. 65(2):1–10. doi:https://doi.org/10.1007/s10347-019-0564-5.
- Dornieden T, Gorbushina A, Krumbein W. 2000. Biodecay of cultural heritage as a space/time-related ecological situation—an evaluation of a series of studies. Int Biodeterior Biodegradation. 46(4):261–270. doi:https://doi.org/10.1016/S0964-8305(00)00107-4.
- Egidi E, de Hoog G, Isola D, Onofri S, Quaedvlieg W, de Vries M, Verkley G, Stielow JB, Zucconi L, Selbmann L. 2014. Phylogeny and taxonomy of meristematic rock-inhabiting black fungi in the Dothideomycetes based on multi-locus phylogenies. Fungal Divers. 65(1):127–165. doi:https://doi.org/10.1007/s13225-013-0277-y.
- Favero-Longo SE, Gazzano C, Girlanda M, Castelli D, Tretiach M, Baiocchi C, Piervittori R. 2011. Physical and chemical deterioration of silicate and carbonate rocks by meristematic microcolonial fungi and endolithic lichens (Chaetothyriomycetidae). Geomicrobiol J. 28(8):732–744. doi:https://doi.org/10.1080/01490451.2010.517696.
- Favero-Longo SE, Viles HA. 2020. A review of the nature, role and control of lithobionts on stone cultural heritage: weighing-up and managing biodeterioration and bioprotection. World J Microbiol Biotechnol. 36(7):1–18. doi:https://doi.org/10.1007/s11274-020-02878-3.
- Flieger K, Knabe N, Toepel J. 2018. Development of an improved carotenoid extraction method to characterize the carotenoid composition under oxidative stress and cold temperature in the rock inhabiting fungus Knufia petricola A95. J Fungi. 4(4):124. doi:https://doi.org/10.3390/jof4040124.
- Freitas DF, Vieira-Da-Motta O, Mathias LDS, Franco RWDA, Gomes RDS, Vieira RAM, Rocha LOD, Olivares FL, Santos CDP. 2019. Synthesis and role of melanin for tolerating in vitro rumen digestion in Duddingtonia flagrans, a nematode-trapping fungus. Mycology. 10(4):229–242. doi:https://doi.org/10.1080/21501203.2019.1631896.
- Gadd GM. 2017. Fungi, rocks, and minerals. Elements. 13(3):171–176. doi:https://doi.org/10.2113/gselements.13.3.171.
- Gleason FH, Larkum AW, Raven JA, Manohar CS, Lilje O. 2019. Ecological implications of recently discovered and poorly studied sources of energy for the growth of true fungi especially in extreme environments. Fungal Ecol. 39:380–387. doi:https://doi.org/10.1016/j.funeco.2018.12.011.
- Golubic S, Friedmann EI, Schneider J. 1981. The lithobiontic ecological niche, with special reference to microorganisms. J Sediment Res. 51(2):475–478.
- Gonçalves V, Cantrell C, Wedge D, Alves T, Zani C, Galante D, Rodrigues F, Schaefer C, Rosa C, Rosa L. 2014. Bioprospection of rock-inhabiting fungi from extreme environments. Planta Med. 80(10): PC26. doi:https://doi.org/10.1055/s-0034-1382408.
- Gonçalves VN, Oliveira FS, Carvalho CR, Schaefer CE, Rosa CA, Rosa LH. 2017. Antarctic rocks from continental Antarctica as source of potential human opportunistic fungi. Extremophiles. 21(5):851–860. doi:https://doi.org/10.1007/s00792-017-0947-x.
- Gorbushina A. 2003. Microcolonial fungi: survival potential of terrestrial vegetative structures. Astrobiology. 3(3):543–554. doi:https://doi.org/10.1089/153110703322610636.
- Gorbushina AA. 2007. Life on the rocks. Environ Microbiol. 9(7):1613–1631. doi:https://doi.org/10.1111/j.1462-2920.2007.01301.x.
- Gorbushina AA, Andreas B, Schulte A. 2005. Microcolonial rock inhabiting fungi and lichen photobionts: evidence for mutualistic interactions. Mycol Res. 109(11):1288–1296. doi:https://doi.org/10.1017/S0953756205003631.
- Gorbushina AA, Broughton WJ. 2009. Microbiology of the atmosphere-rock interface: how biological interactions and physical stresses modulate a sophisticated microbial ecosystem. Annu Rev Microbiol. 63:431–450. doi:https://doi.org/10.1146/annurev.micro.091208.073349.
- Gorbushina AA, Krumbein WE. 2000. Rock dwelling fungal communities: diversity of life styles and colony structure. In: Seckbach, J, editor. Journey to diverse microbial worlds. Dordrecht: Springer; p. 317–334.
- Gorbushina AA, Krumbein WE, Volkmann M. 2002. Rock surfaces as life indicators: new ways to demonstrate life and traces of former life. Astrobiology. 2(2):203–213. doi:https://doi.org/10.1089/15311070260192273.
- Gorbushina A, Kotlova E, Sherstneva O. 2008. Cellular responses of microcolonial rock fungi to long-term desiccation and subsequent rehydration. Stud Mycol. 61:91–97. doi:https://doi.org/10.3114/sim.2008.61.09.
- Gorbushina A, Krumbein W, Hamman C, Panina L, Soukharjevski S, Wollenzien U. 1993. Role of black fungi in color change and biodeterioration of antique marbles. Geomicrobiol J. 11(3–4):205–221. doi:https://doi.org/10.1080/01490459309377952.
- Gostinčar C, Grube M, Gunde-Cimerman N. 2011. Evolution of fungal pathogens in domestic environments? Fungal Biol. 115(10):1008–1018. doi:https://doi.org/10.1016/j.funbio.2011.03.004.
- Gostinčar C, Gunde‐Cimerman N, Grube M. 2015. Polyextremotolerance as the fungal answer to changing environments. In: Bakermans C, editor. Microbial evolution under extreme conditions. Berlin: de Gruyter; p. 185–208.
- Gostinčar C, Muggia L, Grube M. 2012. Polyextremotolerant black fungi: oligotrophism, adaptive potential, and a link to lichen symbioses. Front Microbiol. 3:390. doi:https://doi.org/10.3389/fmicb.2012.00390.
- Gostinčar C, Stajich JE, Zupančič J, Zalar P, Gunde-Cimerman N. 2018a. Genomic evidence for intraspecific hybridization in a clonal and extremely halotolerant yeast. BMC Genomics. 19(1):1–12. doi:https://doi.org/10.1186/s12864-018-4751-5.
- Gostinčar C, Zajc J, Lenassi M, Plemenitaš A, de Hoog S, Al-Hatmi AM, Gunde-Cimerman N. 2018b. Fungi between extremotolerance and opportunistic pathogenicity on humans. Fungal Divers. 93(1):195–213. doi:https://doi.org/10.1007/s13225-018-0414-8.
- Gromov B. 1957. Microflora of rocky and primitive soils in certain northern regions of USSR. Mikrobiologiia. 26(1):52–59.
- Grube M, Muggia L, Gostinčar C. 2013. Niches and adaptations of polyextremotolerant black fungi. In: Seckbach, J, Oren, A, and Stan-Lotter, H, editors. Polyextremophiles. Dordrecht: Springer; p. 551–566.
- Gueidan C, Ruibal C, de Hoog G, Schneider H. 2011. Rock-inhabiting fungi originated during periods of dry climate in the late Devonian and middle Triassic. Fungal Biol. 115(10):987–996. doi:https://doi.org/10.1016/j.funbio.2011.04.002.
- Gueidan C, Villaseñor CR, de Hoog G, Gorbushina A, Untereiner W, Lutzoni F. 2008. A rock-inhabiting ancestor for mutualistic and pathogen-rich fungal lineages. Stud Mycol. 61:111–119. doi:https://doi.org/10.3114/sim.2008.61.11.
- Heinen W, Lauwers A. 1986. Mold on the rocks: a lithobiontic fungus from the sediments of radioactive thermal waters in the Gastein Valley, Austria. Acta Bot Neerl. 35(3):367–372. doi:https://doi.org/10.1111/j.1438-8677.1986.tb01299.x.
- Hubka V, Réblová M, Řehulka J, Selbmann L, Isola D, de Hoog SG, Kolařík M. 2014. Bradymyces gen. nov. (Chaetothyriales, Trichomeriaceae), a new ascomycete genus accommodating poorly differentiated melanized fungi. Antonie van Leeuwenhoek. 106(5):979–992. doi:https://doi.org/10.1007/s10482-014-0267-4.
- Isola D, Marzban G, Selbmann L, Onofri S, Laimer M, Sterflinger K. 2011. Sample preparation and 2-DE procedure for protein expression profiling of black microcolonial fungi. Fungal Biol. 115(10):971–977. doi:https://doi.org/10.1016/j.funbio.2011.03.001.
- Isola D, Selbmann L, de Hoog GS, Fenice M, Onofri S, Prenafeta-Boldú FX, Zucconi L. 2013. Isolation and screening of black fungi as degraders of volatile aromatic hydrocarbons. Mycopathologia. 175(5–6):369–379. doi:https://doi.org/10.1007/s11046-013-9635-2.
- Isola D, Zucconi L, Onofri S, Caneva G, de Hoog G, Selbmann L. 2016. Extremotolerant rock inhabiting black fungi from Italian monumental sites. Fungal Divers. 76(1):75–96. doi:https://doi.org/10.1007/s13225-015-0342-9.
- King Jr AD, Hocking AD, Pitt JI. 1979. Dichloran-rose bengal medium for enumeration and isolation of molds from foods. Appl Environ Microbiol. 37(5):959–964. doi:https://doi.org/10.1128/aem.37.5.959-964.1979.
- Kirtzel J, Siegel D, Krause K, Kothe E. 2017. Stone-eating fungi: mechanisms in bioweathering and the potential role of laccases in black slate degradation with the basidiomycete Schizophyllum commune. Adv Appl Microbiol. 99:83–101.
- Kiyuna T, An K-D, Kigawa R, Sano C, Sugiyama J. 2018. Two new Cladophialophora species, C. tumbae sp. nov. and C. tumulicola sp. nov., and chaetothyrialean fungi from biodeteriorated samples in the Takamatsuzuka and Kitora Tumuli. Mycoscience. 59(1):75–84. doi:https://doi.org/10.1016/j.myc.2017.08.008.
- Kuklinski P. 2009. Ecology of stone-encrusting organisms in the Greenland Sea—a review. Polar Res. 28(2):222–237. doi:https://doi.org/10.1111/j.1751-8369.2009.00105.x.
- Lakk H, Krijgsheld P, Montalti M, Woesten H 2018. Fungal based biocomposite for habitat structures on the moon and mars. 69th International Astronautical Congress Bremen, Germany.
- Lenassi M, Gostinčar C, Jackman S, Turk M, Sadowski I, Nislow C, Jones S, Birol I, Cimerman NG, Plemenitaš A. 2013. Whole genome duplication and enrichment of metal cation transporters revealed by de novo genome sequencing of extremely halotolerant black yeast Hortaea werneckii. PLoS One. 8(8):e71328. doi:https://doi.org/10.1371/journal.pone.0071328.
- Liberti D, Alfieri ML, Monti DM, Panzella L, Napolitano A. 2020. A melanin-related phenolic polymer with potent photoprotective and antioxidant activities for dermo-cosmetic applications. Antioxidants. 9(4):270. doi:https://doi.org/10.3390/antiox9040270.
- Luo Y, Wei X, Yang S, Gao Y-H, Luo Z-H. 2020. Fungal diversity in deep-sea sediments from the Magellan seamounts as revealed by a metabarcoding approach targeting the ITS2 regions. Mycology. 11(3):214–229. doi:https://doi.org/10.1080/21501203.2020.1799878.
- Lyu X, Shen C, Xie J, Fu Y, Jiang D, Hu Z, Tang L, Tang L, Ding F, Li K. 2015. A “footprint” of plant carbon fixation cycle functions during the development of a heterotrophic fungus. Sci Rep. 5(1):1–13. doi:https://doi.org/10.1038/srep12952.
- Martin-Sanchez PM, Nováková A, Bastian F, Alabouvette C, Saiz-Jimenez C. 2012. Two new species of the genus Ochroconis, O. lascauxensis and O. anomala isolated from black stains in Lascaux Cave, France. Fungal Biol. 116(5):574–589. doi:https://doi.org/10.1016/j.funbio.2012.02.006.
- Mikhailyuk TI. 2008. Terrestrial lithophilic algae in a granite canyon of the Teteriv River (Ukraine). Biologia. 63(6):824–830. doi:https://doi.org/10.2478/s11756-008-0104-1.
- Miura A, Urabe J. 2017. Changes in epilithic fungal communities under different light conditions in a river: a field experimental study. Limnol Oceanogr. 62(2):579–591. doi:https://doi.org/10.1002/lno.10445.
- Moreno LF, Vicente VA, de Hoog S. 2018. Black yeasts in the omics era: achievements and challenges. Med Mycol. 56(suppl_1):S32–S41. doi:https://doi.org/10.1093/mmy/myx129.
- Nagano Y, Miura T, Tsubouchi T, Lima AO, Kawato M, Fujiwara Y, Fujikura K. 2020. Cryptic fungal diversity revealed in deep-sea sediments associated with whale-fall chemosynthetic ecosystems. Mycology. 11(3):263–278. doi:https://doi.org/10.1080/21501203.2020.1799879.
- Nai C. 2014. Rock-inhabiting fungi studied with the aid of the model black fungus Knufia petricola A95 and other related strains. Bundesanstalt für Materialforschung und-prüfung (BAM).
- Nai C, Wong HY, Pannenbecker A, Broughton WJ, Benoit I, de Vries RP, Gueidan C, Gorbushina AA. 2013. Nutritional physiology of a rock-inhabiting, model microcolonial fungus from an ancestral lineage of the Chaetothyriales (Ascomycetes). Fungal Genet Biol. 56:54–66. doi:https://doi.org/10.1016/j.fgb.2013.04.001.
- Naranjo‐Ortiz MA, Gabaldón T. 2019. Fungal evolution: major ecological adaptations and evolutionary transitions. Biol Rev. 94(4):1443–1476. doi:https://doi.org/10.1111/brv.12510.
- Noack-Schönmann S, Bus T, Banasiak R, Knabe N, Broughton WJ, Den Dulk-Ras H, Hooykaas PJ, Gorbushina AA. 2014. Genetic transformation of Knufia petricola A95-a model organism for biofilm-material interactions. AMB Express. 4(1):1–6. doi:https://doi.org/10.1186/s13568-014-0080-5.
- Noack-Schönmann S, Spagin O, Gründer K-P, Breithaupt M, Günter A, Muschik B, Gorbushina A. 2014b. Sub-aerial biofilms as blockers of solar radiation: spectral properties as tools to characterise material-relevant microbial growth. Int Biodeterior Biodegradation. 86:286–293. doi:https://doi.org/10.1016/j.ibiod.2013.09.020.
- Omelon CR. 2008. Endolithic microbial communities in polar desert habitats. Geomicrobiol J. 25(7–8):404–414. doi:https://doi.org/10.1080/01490450802403057.
- Onofri S, Barreca D, Selbmann L, Isola D, Rabbow E, Horneck G, de Vera J, Hatton J, Zucconi L. 2008. Resistance of Antarctic black fungi and cryptoendolithic communities to simulated space and Martian conditions. Stud Mycol. 61:99–109. doi:https://doi.org/10.3114/sim.2008.61.10.
- Onofri S, de La Torre R, de Vera J-P, Ott S, Zucconi L, Selbmann L, Scalzi G, Venkateswaran KJ, Rabbow E, Sánchez Iñigo FJ. 2012. Survival of rock-colonizing organisms after 1.5 years in outer space. Astrobiology. 12(5):508–516. doi:https://doi.org/10.1089/ast.2011.0736.
- Onofri S, de Vera J-P, Zucconi L, Selbmann L, Scalzi G, Venkateswaran KJ, Rabbow E, de La Torre R, Horneck G. 2015. Survival of Antarctic cryptoendolithic fungi in simulated Martian conditions on board the International Space Station. Astrobiology. 15(12):1052–1059. doi:https://doi.org/10.1089/ast.2015.1324.
- Onofri S, Selbmann L, de Hoog G, Grube M, Barreca D, Ruisi S, Zucconi L. 2007. Evolution and adaptation of fungi at boundaries of life. Adv Space Res. 40(11):1657–1664. doi:https://doi.org/10.1016/j.asr.2007.06.004.
- Onofri S, Selbmann L, Pacelli C, de Vera JP, Horneck G, Hallsworth JE, Zucconi L. 2018. Integrity of the DNA and cellular ultrastructure of cryptoendolithic fungi in space or Mars conditions: a 1.5-year study at the International Space Station. Life. 8(2):23. doi:https://doi.org/10.3390/life8020023.
- Onofri S, Selbmann L, Zucconi L, Pagano S. 2004. Antarctic microfungi as models for exobiology. Planet Space Sci. 52(1–3):229–237. doi:https://doi.org/10.1016/j.pss.2003.08.019.
- Onofri S, Zucconi L, Isola D, Selbmann L. 2014. Rock-inhabiting fungi and their role in deterioration of stone monuments in the Mediterranean area. Plant Biosyst. 148(2):384–391. doi:https://doi.org/10.1080/11263504.2013.877533.
- Pacelli C, Bryan RA, Onofri S, Selbmann L, Shuryak I, Dadachova E. 2017a. Melanin is effective in protecting fast and slow growing fungi from various types of ionizing radiation. Environ Microbiol. 19(4):1612–1624. doi:https://doi.org/10.1111/1462-2920.13681.
- Pacelli C, Bryan RA, Onofri S, Selbmann L, Zucconi L, Shuryak I, Dadachova E. 2018a. The effect of protracted X‐ray exposure on cell survival and metabolic activity of fast and slow growing fungi capable of melanogenesis. Environ Microbiol Rep. 10(3):255–263. doi:https://doi.org/10.1111/1758-2229.12632.
- Pacelli C, Bryan RA, Onofri S, Selbmann L, Zucconi L, Shuryak I, Dadachova E. 2018b. Survival and redox activity of Friedmanniomyces endolithicus, an Antarctic endemic black meristematic fungus, after gamma rays exposure. Fungal Biol. 122(12):1222–1227. doi:https://doi.org/10.1016/j.funbio.2018.10.002.
- Pacelli C, Cassaro A, Maturilli A, Timperio AM, Gevi F, Cavalazzi B, Stefan M, Ghica D, Onofri S. 2020. Multidisciplinary characterization of melanin pigments from the black fungus Cryomyces antarcticus. Appl Microbiol Biotechnol. 104:6385–6395. doi:https://doi.org/10.1007/s00253-020-10666-0.
- Pacelli C, Selbmann L, Zucconi L, Coleine C, de Vera J-P, Rabbow E, Böttger U, Dadachova E, Onofri S. 2019. Responses of the black fungus cryomyces antarcticus to simulated Mars and space conditions on rock analogs. Astrobiology. 19(2):209–220. doi:https://doi.org/10.1089/ast.2016.1631.
- Pacelli C, Selbmann L, Zucconi L, de Vera J-P, Rabbow E, Horneck G, de La Torre R, Onofri S. 2017b. BIOMEX experiment: ultrastructural alterations, molecular damage and survival of the fungus Cryomyces antarcticus after the experiment verification tests. Origins Life Evol Biospheres. 47(2):187–202. doi:https://doi.org/10.1007/s11084-016-9485-2.
- Pacelli C, Selbmann L, Zucconi L, Raguse M, Moeller R, Shuryak I, Onofri S. 2017c. Survival, DNA integrity, and ultrastructural damage in Antarctic cryptoendolithic eukaryotic microorganisms exposed to ionizing radiation. Astrobiology. 17(2):126–135. doi:https://doi.org/10.1089/ast.2015.1456.
- Palmer F, Emery D, Stemmler J, Staley J. 1987. Survival and growth of microcolonial rock fungi as affected by temperature and humidity. New Phytol. 107(1):155–162. doi:https://doi.org/10.1111/j.1469-8137.1987.tb04889.x.
- Palmer F, Staley J, Ryan B. 1990. Ecophysiology of microcolonial fungi and lichens on rocks in Northeastern Oregon. New Phytol. 116(4):613–620. doi:https://doi.org/10.1111/j.1469-8137.1990.tb00546.x.
- Palmer R, Friedmann E. 1988. 2.9 incorporation of inorganic carbon by Antarctic cryptoendolithic fungi. Polarforschung. 58(2/3):189–191.
- Perry RS, Gorbushina A, Engel MH, Kolb VM, Krumbein WE, Staley JT. 2004. Accumulation and deposition of inorganic and organic compounds by microcolonial fungi. Third European Workshop on Exo-Astrobiology Madrid, Spain.
- Pinheiro AC, Mesquita N, Trovão J, Soares F, Tiago I, Coelho C, de Carvalho HP, Gil F, Catarino L, Piñar G. 2019. Limestone biodeterioration: a review on the Portuguese cultural heritage scenario. J Cult Heritage. 36:275–285. doi:https://doi.org/10.1016/j.culher.2018.07.008.
- Pombeiro-Sponchiado SR, Sousa GS, Andrade JC, Lisboa HF, Gonçalves RC. 2017. Production of melanin pigment by fungi and its biotechnological applications. Melanin. 47–75.
- Prenafeta-Boldú FX, Roca N, Villatoro C, Vera L, de Hoog GS. 2019. Prospective application of melanized fungi for the biofiltration of indoor air in closed bioregenerative systems. J Hazard Mater. 361:1–9. doi:https://doi.org/10.1016/j.jhazmat.2018.08.059.
- Réblová M, Hubka V, Thureborn O, Lundberg J, Sallstedt T, Wedin M, Ivarsson M. 2016. From the tunnels into the treetops: new lineages of black yeasts from biofilm in the Stockholm metro system and their relatives among ant-associated fungi in the Chaetothyriales. PLoS One. 11(10):e0163396. doi:https://doi.org/10.1371/journal.pone.0163396.
- Revankar SG. 2007. Dematiaceous fungi. Mycoses. 50(2):91–101. doi:https://doi.org/10.1111/j.1439-0507.2006.01331.x.
- Revankar SG, Sutton DA. 2010. Melanized fungi in human disease. Clin Microbiol Rev. 23(4):884–928. doi:https://doi.org/10.1128/CMR.00019-10.
- Ruibal C, Gueidan C, Selbmann L, Gorbushina A, Crous P, Groenewald J, Muggia L, Grube M, Isola D, Schoch C. 2009. Phylogeny of rock-inhabiting fungi related to Dothideomycetes. Stud Mycol. 64:123–133. doi:https://doi.org/10.3114/sim.2009.64.06.
- Ruibal C, Platas G, Bills G. 2008. High diversity and morphological convergence among melanised fungi from rock formations in the Central Mountain System of Spain. Persoonia. 21:93. doi:https://doi.org/10.3767/003158508X371379.
- Ruibal C, Platas G, Bills GF. 2005. Isolation and characterization of melanized fungi from limestone formations in Mallorca. Mycol Prog. 4(1):23–38. doi:https://doi.org/10.1007/s11557-006-0107-7.
- Ruibal C, Selbmann L, Avci S, Martin-Sanchez PM, Gorbushina AA. 2018. Roof-inhabiting cousins of rock-inhabiting fungi: novel melanized microcolonial fungal species from photocatalytically reactive subaerial surfaces. Life. 8(3):30. doi:https://doi.org/10.3390/life8030030.
- Scalzi G, Selbmann L, Zucconi L, Rabbow E, Horneck G, Albertano P, Onofri S. 2012. LIFE experiment: isolation of cryptoendolithic organisms from Antarctic colonized sandstone exposed to space and simulated Mars conditions on the International Space Station. Origins Life Evol Biospheres. 42(2):253–262. doi:https://doi.org/10.1007/s11084-012-9282-5.
- Scheerer S, Ortega‐Morales O, Gaylarde C. 2009. Microbial deterioration of stone monuments—an updated overview. Adv Appl Microbiol. 66:97–139.
- Schumacher J, Gorbushina AA. 2020. Light sensing in plant-and rock-associated black fungi. Fungal Biol. 124(5):407–417. doi:https://doi.org/10.1016/j.funbio.2020.01.004.
- Seiffert F, Bandow N, Bouchez J, Von Blanckenburg F, Gorbushina A. 2014. Microbial colonization of bare rocks: laboratory biofilm enhances mineral weathering. Procedia Earth Planet Sci. 10:123–129. doi:https://doi.org/10.1016/j.proeps.2014.08.042.
- Selbmann L, Benkő Z, Coleine C, de Hoog S, Donati C, Druzhinina I, Emri T, Ettinger CL, Gladfelter AS, Gorbushina AA, et al. 2020. Shed light in the DaRk LineagES of the fungal tree of life—STRES[J]. Life. 10(12):362. doi:https://doi.org/10.3390/life10120362.
- Selbmann L, de Hoog G, Mazzaglia A, Friedmann E, Onofri S. 2005. Fungi at the edge of life: cryptoendolithic black fungi from Antarctic desert. Stud Mycol. 51(1):1–32.
- Selbmann L, de Hoog G, Zucconi L, Isola D, Ruisi S, van Den Ende AG, Ruibal C, de Leo F, Urzì C, Onofri S. 2008. Drought meets acid: three new genera in a dothidealean clade of extremotolerant fungi. Stud Mycol. 61:1–20. doi:https://doi.org/10.3114/sim.2008.61.01.
- Selbmann L, Grube M, Onofri S, Isola D, Zucconi L. 2013. Antarctic epilithic lichens as niches for black meristematic fungi. Biology. 2(2):784–797. doi:https://doi.org/10.3390/biology2020784.
- Selbmann L, Isola D, Egidi E, Zucconi L, Gueidan C, de Hoog G, Onofri S. 2014. Mountain tips as reservoirs for new rock-fungal entities: saxomyces gen. nov. and four new species from the Alps. Fungal Divers. 65(1):167–182. doi:https://doi.org/10.1007/s13225-013-0234-9.
- Selbmann L, Pacelli C, Zucconi L, Dadachova E, Moeller R, de Vera J-P, Onofri S. 2018. Resistance of an Antarctic cryptoendolithic black fungus to radiation gives new insights of astrobiological relevance. Fungal Biol. 122(6):546–554. doi:https://doi.org/10.1016/j.funbio.2017.10.012.
- Selbmann L, Zucconi L, Isola D, Onofri S. 2015. Rock black fungi: excellence in the extremes, from the Antarctic to space. Curr Genet. 61(3):335–345. doi:https://doi.org/10.1007/s00294-014-0457-7.
- Sert HB, Sterflinger K. 2010. A new Coniosporium species from historical marble monuments. Mycol Prog. 9(3):353–359. doi:https://doi.org/10.1007/s11557-009-0643-z.
- Sert HB, Suembuel H, Sterflinger K. 2007a. A new species of Capnobotryella from monument surfaces. Mycol Res. 111(10):1235–1241. doi:https://doi.org/10.1016/j.mycres.2007.06.011.
- Sert HB, Suembuel H, Sterflinger K. 2007b. Sarcinomyces sideticae, a new black yeast from historical marble monuments in Side (Antalya, Turkey). Bot J Linn Soc. 154(3):373–380. doi:https://doi.org/10.1111/j.1095-8339.2007.00658.x.
- Sert HB, Suembuel H, Sterflinger K. 2011. Two new species of Capnobotryella from historical monuments. Mycol Prog. 10(3):333–339. doi:https://doi.org/10.1007/s11557-010-0706-1.
- Sert HB, Wuczkowski M, Sterflinger K. 2012. Capnobotryella isiloglui, a new rock-inhabiting fungus from Austria. Turk J Bot. 36(4):401–407.
- Shirakawa MA, Zilles R, Mocelin A, Gaylarde CC, Gorbushina A, Heidrich G, Giudice MC, Del Negro GM, John VM. 2015. Microbial colonization affects the efficiency of photovoltaic panels in a tropical environment. J Environ Manage. 157:160–167. doi:https://doi.org/10.1016/j.jenvman.2015.03.050.
- Silva-Bailao MG, Da Silva KLP, Dos Anjos LRB, de Sousa Lima P, de Melo Teixeira M, de Almeida Soares CM, Bailão AM. 2018. Mechanisms of copper and zinc homeostasis in pathogenic black fungi. Fungal Biol. 122(6):526–537. doi:https://doi.org/10.1016/j.funbio.2017.12.002.
- Singleton I, Tobin JM. 1996. Fungal interactions with metals and radionuclides for environmental bioremediation. Fungi and environmental change: symposium of the British Mycological Society, held at Cranfield University; March 1994. Cambridge [England]; New York: Published for the British Mycological Society ….
- Smith DF, Casadevall A. 2019. The role of melanin in fungal pathogenesis for animal hosts. In: Rodrigues, M, editor. Fungal physiology and immunopathogenesis. Cham: Springer; p. 1–30.
- Staley JT, Palmer F, Adams JB. 1982. Microcolonial fungi: common inhabitants on desert rocks? Science. 215(4536):1093–1095. doi:https://doi.org/10.1126/science.215.4536.1093.
- Sterflinger K. 1998a. Ecophysiology of rock inhabiting black yeasts with special reference to temperature and osmotic stress. Antonie van Leeuwenhoek. 74:271–281. doi:https://doi.org/10.1023/A:1001753131034.
- Sterflinger K. 1998b. Temperature and NaCl-tolerance of rock-inhabiting meristematic fungi. Antonie van Leeuwenhoek. 74(4):271–281.
- Sterflinger K, de Baere R, de Hoog G, de Wachter R, Krumbein WE, Haase G. 1997. Coniosporium perforans and C. apollinis, two new rock-inhabiting fungi isolated from marble in the Sanctuary of Delos (Cyclades, Greece). Antonie van Leeuwenhoek. 72(4):349–363. doi:https://doi.org/10.1023/A:1000570429688.
- Sterflinger K, de Hoog G, Haase G. 1999. Phylogeny and ecology of meristematic ascomycetes. Stud Mycol. 43:5–22.
- Sterflinger K, Gorbushina AA. 1997. Morphological and molecular characterization of a rock inhabiting and rock decaying dematiaceous fungus isolated from antique monuments of Delos (Cyclades, Greece) and Chersonesus (Crimea, Ukraine). Syst Appl Microbiol. 20(2):329–335. doi:https://doi.org/10.1016/S0723-2020(97)80080-X.
- Sterflinger K, Hain M. 1999. In situ hybridization with rRNA targeted probes as a new tool for the detection of black yeasts and meristematic fungi. Stud Mycol. 43:23–30.
- Sterflinger K, Krumbein W. 1995. Multiple stress factors affecting growth of rock‐inhabiting black fungi. Bot Acta. 108(6):490–496. doi:https://doi.org/10.1111/j.1438-8677.1995.tb00526.x.
- Sterflinger K, Lopandic K, Pandey RV, Blasi B, Kriegner A. 2014. Nothing special in the specialist? Draft genome sequence of Cryomyces antarcticus, the most extremophilic fungus from Antarctica. PLoS One. 9(10):e109908. doi:https://doi.org/10.1371/journal.pone.0109908.
- Sterflinger K. 2006. Black yeasts and meristematic fungi: ecology, diversity and identification. In: Péter, G, Rosa, C, editors. Biodiversity and ecophysiology of yeasts. Berlin, Heidelberg: Springer; p. 501–514.
- Sterflinger K, Piñar G. 2013. Microbial deterioration of cultural heritage and works of art—tilting at windmills? Appl Microbiol Biotechnol. 97(22):9637–9646. doi:https://doi.org/10.1007/s00253-013-5283-1.
- Sterflinger K, Tesei D, Zakharova K. 2012. Fungi in hot and cold deserts with particular reference to microcolonial fungi. Fungal Ecol. 5(4):453–462. doi:https://doi.org/10.1016/j.funeco.2011.12.007.
- Su C-J, Hsieh S-Y, Chiang MW-L, Pang K-L. 2020. Salinity, pH and temperature growth ranges of Halophytophthora isolates suggest their physiological adaptations to mangrove environments. Mycology. 11(3):256–262. doi:https://doi.org/10.1080/21501203.2020.1714768.
- Su L, Guo L, Hao Y, Xiang M, Cai L, Liu X. 2015. Rupestriomyces and Spissiomyces, two new genera of rock-inhabiting fungi from China. Mycologia. 107(4):831–844. doi:https://doi.org/10.3852/14-305.
- Sun W, Liu B, Fu R, Liu X, Xiang M. 2019. Two new rock-inhabiting species of Cyphellophora from karst habitats in China. Phytotaxa. 397(1):23–33. doi:https://doi.org/10.11646/phytotaxa.397.1.2.
- Sun W, Su L, Yang S, Sun J, Liu B, Fu R, Wu B, Liu X, Cai L, Guo L. 2020. Unveiling the hidden diversity of rock-inhabiting fungi: chaetothyriales from China. J Fungi. 6(4):187. doi:https://doi.org/10.3390/jof6040187.
- Taylor JW, Jacobson DJ, Kroken S, Kasuga T, Geiser DM, Hibbett DS, Fisher MC. 2000. Phylogenetic species recognition and species concepts in fungi. Fungal Genet Biol. 31(1):21–32. doi:https://doi.org/10.1006/fgbi.2000.1228.
- Teixeira M, Moreno LF, Stielow B, Muszewska A, Hainaut M, Gonzaga L, Abouelleil A, Patané J, Priest M, Souza R. 2017. Exploring the genomic diversity of black yeasts and relatives (Chaetothyriales, Ascomycota). Stud Mycol. 86:1–28. doi:https://doi.org/10.1016/j.simyco.2017.01.001.
- Tesei D, Marzban G, Zakharova K, Isola D, Selbmann L, Sterflinger K. 2012. Alteration of protein patterns in black rock inhabiting fungi as a response to different temperatures. Fungal Biol. 116(8):932–940. doi:https://doi.org/10.1016/j.funbio.2012.06.004.
- Tesei D, Quartinello F, Guebitz GM, Ribitsch D, Nöbauer K, Razzazi-Fazeli E, Sterflinger K. 2020. Shotgun proteomics reveals putative polyesterases in the secretome of the rock-inhabiting fungus Knufia chersonesos. Sci Rep. 10(1):1–15. doi:https://doi.org/10.1038/s41598-019-56847-4.
- Tonon C, Breitenbach R, Voigt O, Turci F, Gorbushina AA, Favero-Longo SE. 2021. Hyphal morphology and substrate porosity-rather than melanization-drive penetration of black fungi into carbonate substrates. J Cult Heritage. 48:244–253. doi:https://doi.org/10.1016/j.culher.2020.11.003.
- Trovão J, Tiago I, Soares F, Paiva DS, Mesquita N, Coelho C, Catarino L, Gil F, Portugal A. 2019. Description of Aeminiaceae fam. nov., Aeminium gen. nov. and Aeminiumludgeri sp. nov. (Capnodiales), isolated from a biodeteriorated art-piece in the Old Cathedral of Coimbra, Portugal. MycoKeys. (45):57. doi:https://doi.org/10.3897/mycokeys.45.31799.
- Urzì C, de Leo F, de Hoog S, Sterflinger K. 2000. Recent advances in the molecular biology and ecophysiology of meristematic stone-inhabiting fungi. In: Ciferri, O, Tiano, P, Mastromei, G, editors. Of microbes and art. Boston (MA): Springer; p. 3–19 doi:https://doi.org/10.1007/978-1-4615-4239-1_1.
- Urzì C, Wollenzien U, Criseo G, Krumbein WE. 1995. Biodiversity of the rock inhabiting microflora with special reference to black fungi and black yeasts. Microbial diversity and ecosystem function. 16:289–302
- Vahidzadeh E, Kalra AP, Shankar K. 2018. Melanin-based electronics: from proton conductors to photovoltaics and beyond. Biosens Bioelectron. 122:127–139. doi:https://doi.org/10.1016/j.bios.2018.09.026.
- Vasileiou T, Summerer L. 2020. A biomimetic approach to shielding from ionizing radiation: the case of melanized fungi. PLoS One. 15(4):e0229921. doi:https://doi.org/10.1371/journal.pone.0229921.
- Vázquez-Nion D, Rodríguez-Castro J, López-Rodríguez M, Fernández-Silva I, Prieto B. 2016. Subaerial biofilms on granitic historic buildings: microbial diversity and development of phototrophic multi-species cultures. Biofouling. 32(6):657–669. doi:https://doi.org/10.1080/08927014.2016.1183121.
- Voigt O, Knabe N, Nitsche S, Erdmann EA, Schumacher J, Gorbushina AA. 2020. An advanced genetic toolkit for exploring the biology of the rock-inhabiting black fungus Knufia petricola. Sci Rep. 10(1):1–14. doi:https://doi.org/10.1038/s41598-020-79120-5.
- Volkmann M, Whitehead K, Rütters H, Rullkötter J, Gorbushina AA. 2003. Mycosporine‐glutamicol‐glucoside: a natural UV‐absorbing secondary metabolite of rock‐inhabiting microcolonial fungi. Rapid Commun Mass Spectrom. 17(9):897–902. doi:https://doi.org/10.1002/rcm.997.
- Wollenzien U, de Hoog G, Krumbein W, Uijthof J. 1997. Sarcinomyces petricola, a new microcolonial fungus from marble in the Mediterranean basin. Antonie van Leeuwenhoek. 71(3):281–288. doi:https://doi.org/10.1023/A:1000157803954
- Wollenzien U, de Hoog G, Krumbein W, Urzi C. 1995. On the isolation of microcolonial fungi occurring on and in marble and other calcareous rocks. Sci Total Environ. 167(1–3):287–294. doi:https://doi.org/10.1016/0048-9697(95)04589-S.
- Wu B, Hussain M, Zhang W, Stadler M, Liu X, Xiang M. 2019. Current insights into fungal species diversity and perspective on naming the environmental DNA sequences of fungi. Mycology. 10(3):127–140. doi:https://doi.org/10.1080/21501203.2019.1614106.
- Zakharova K, Marzban G, de Vera J-P, Lorek A, Sterflinger K. 2014. Protein patterns of black fungi under simulated Mars-like conditions. Sci Rep. 4(1):1–7.
- Zakharova K, Tesei D, Marzban G, Dijksterhuis J, Wyatt T, Sterflinger K. 2013. Microcolonial fungi on rocks: a life in constant drought? Mycopathologia. 175(5–6):537–547. doi:https://doi.org/10.1007/s11046-012-9592-1.
- Zhang Z-F, Pan Y-P, Liu Y, Li M. 2020. Pacific Biosciences Single-molecule Real-time (SMRT) Sequencing reveals high diversity of basal fungal lineages and stochastic processes controlled fungal community assembly in mangrove sediments.