ABSTRACT
Four dimeric diketopiperazine stereoisomers (1–4) including two new ones (1–2) had been isolated from the culture broth of one marine-derived fungus Aspergillus sp. Z3, which was found in the gut of a marine isopod Ligia exotica. The planner structures and absolute configurations of the new compounds were determined by combination of NMR, HRESIMS, electronic circular dichroism calculation, Marfey’s method as well as single-crystal X-ray diffraction. Their cytotoxicity against the prostate cancer PC3 cell line was assayed by the MTT method.
1. Introduction
Indole diketopiperazines, which were formed by the condensation of tryptophan with other different amino acids, are an important family of secondary metabolites commonly produced by fungi. Most of these alkaloids are characterised by diverse structural scaffolds and ring systems derived from dimerisation by two indole units and conjugation with another building block as cyclic dipeptides (Li et al. Citation2009; Ma et al. Citation2016; Klas et al. Citation2018). This class of alkaloids possess diverse biological activities such as antimicrobial (Zheng et al. Citation2007; Jia et al. Citation2019; Yang et al. Citation2021), anticancer (Wang et al. Citation2015; Zhang et al. Citation2019), immunomodulatory (Fujimoto et al. Citation2004), anti-inflammatory (Barrow et al. Citation1993; Wen et al. Citation2018; Yang et al. Citation2021), antioxidant (Sun et al. Citation2018) and antiviral (Wibowo et al. Citation2021; Nishiuchi et al. Citation2022), which attracted much attention. Our ongoing research on marine fungal metabolites revealed one diketopiperazine dimer WIN 64821 (4), a competitive antagonist to substance P (Barrow et al. Citation1993) and an antagonist of cholecystokinin type-B receptor (Sedlock et al. Citation1994), with a series of its stereoisomers (1–3) from the marine-derived fungus Aspergillus sp. Z3 (). Compounds 1 and 2 are new ones. Herein, the purification, structure elucidation, and biological activities of the four compounds are described.
2. Materials and methods
2.1. General experimental procedures
Optical rotation was measured on an Autopol I polarimeter (Rudolph Research Analytical, Hackettstown, NJ, USA). The ultraviolet (UV) absorption spectra were measured in MeOH on a METASH UV-8000 spectrophotometer (Shanghai METASH Instruments Co. Ltd., Shanghai, China). The electronic circular dichroism (ECD) was measured on a JASCO J-815 spectropolarimeter (JASCO Co. Tokyo, Japan). The infrared (IR) spectra were recorded using a Bruker Vector 32 spectrometer (Brucker, Karlsruhe, Germany). NMR spectra were recorded in CDCl3 (ALDRICH, St. Louis, MO, USA) or MeOH-d4 (ALDRICH, St. Louis, MO, USA) with tetramethylsilane (TMS) as an internal standard, using a Bruker AV III 500 MHz NMR spectrometer. HR-ESIMS data were obtained on an Agilent 6224 TOF LC/MS. Single crystal X-ray crystallography was determined on an Xcalibur Atlas Gemini Ultra diffractometer (Agilent Technologies). Column chromatography (CC) was performed with silica (100–200 and 200–300 mesh, Qingdao Haiyang Chemical Co., Ltd., Qingdao, China).
2.2. Fungal material and fermentation
The fungal colony (Z3) was isolated from marine isopod Ligia exotica which was collected in seaside of Dinghai in Zhoushan, Zhejiang Province of China, in December 2011. The fungal Z3 was determined as Aspergillus sp. by ITS analysis.
The strain (Z3) was static cultured at 28 °C for 30 days in 500 mL Erlenmeyer-flasks (200 × 250 mL, a total of 50 L) each containing 250 mL of 2216E liquid media (QingDao Hopebio-Technology Co., Ltd., Qingdao, China).
The culture broth (50 L) was filtered and extracted with equivoluminal EtOAc for 3 times to obtain 5 g metabolites extract, which was fractioned by silica gel column chromatography (CC) eluted in gradient from cyclohexane- EtOAc (20:1–10:1) to CH2Cl2- MeOH(100:1–5:1). Ten fractions (Frs. 1–10) were collected based on TLC analysis. Fr. 8 (500 mg) was purified by preparative ODS-HPLC (Agilent Persuit XRs C18, 10 μm, 21.2 × 250 mm, 10 mL/min, 60% MeOH in H2O) to obtain compound 3 (44.2 mg), 4 (44.2 mg) and 1 (6.2 mg). Fr. 9 (230 mg) was purified by semi-preparative ODS-HPLC (COSMOSIL PACKED COLUMN, 5C18-MS-II column, 10ID×250 mm, 4 mL/min, 53% MeOH in H2O) to obtain compound 2 (7.2 mg).
2.2.1. Physicochemical and spectral data
Compound 1:white solid; [α] 25D +478.0 (c 1.1, CHCl3); UV (MeOH) λmax (logε): 210 (3.9), 241 (2.3), 300 (1.4) nm; IR (film) νmax: 3380, 2924, 1667, 1408, 1340, 1255 cm−1; 1H and 13C NMR data see ; (+)-HRESIMS [M + H]+ m/z 665.2868 (calcd. 665.2871 for C40H37N6O4).
Table 1. 1H NMR data for compounds 1–2 (1H NMR at 500 MHz).
Table 2. 13C NMR data for compounds 1–2 (13C NMR at 125 MHz).
Compound 2:white solid; [α] 25D +228.4 (c 0.90, CHCl3); UV (MeOH) λmax (logε): 210 (4.3), 242 (2.6), 301 (1.7) nm; IR (film) νmax: 3378, 2925, 1670, 1437, 1316, 1097 cm−1; 1H and 13C NMR data see ; (+)-HRESIMS [M + H]+ m/z 665.2863 (calcd. 665.2871 for C40H37N6O4).
Compound 3:white solid; [α] 25D +316.8 (c 0.95, CHCl3); UV (MeOH) λmax (logε): 210 (3.9), 242 (2.2), 301 (1.1) nm; IR (film) νmax: 3385, 2923, 2851, 1667, 1435, 1254 cm−1; 1H and 13C NMR data see Table S1 and S2, Supplementary data; (+)-HRESIMS [M + H]+ m/z 665.2860 (calcd. 665.2871 for C40H37N6O4).
WIN 64821 (4):white solid; [α] 25D +397.3 (c 0.95, CHCl3); UV (MeOH) λmax (logε): 210 (4.3), 242 (2.6), 301 (1.7) nm; IR (film) νmax: 3405, 2930, 1635, 1455, 1341, 1248 cm−1; 1H and 13C NMR data see Table S1 and S2, Supplementary data; (+)-HRESIMS [M + H]+ m/z 665.2894 (calcd. 665.2871 for C40H37N6O4).
2.3. Marfey’s method
Compounds 1–3 (0.5 mg) were hydrolysed in 1 mL HCl (6 mol/L) at 110 °C for 24 h, respectively. The cooled reaction mixture was evaporated to dryness and HCl was removed. The dried hydrolysate was dissolved in 120 μL ddH2O and then 20 μL NaHCO3 (1 mol/L) and 400 μL 1% (w/v) FDAA in acetone were added to the solution of the hydrolysate. The mixture was stirred at 42 °C for 2 h and the reaction was terminated by an addition of 10 μL HCl (1 mol/L). The reaction product was evaporated to dryness and dissolved with 1 mL MeOH to give amino acid-FDAA derivatives for the HPLC analysis. Each standard amino acid (0.5 mg) of L-phenylalanine and D-phenylalanine was also converted to its FDAA derivatives. For Marfey′s analysis, each of the amino acid-FDAA derivatives (15 μL) was analysed by HPLC (SHIMADZU column: C18, 5 μm, 4.6 × 250 mm; flow rate: 1.0 mL/min; detection wavelength: 340 nm) with the linear gradient programme from 10% to 100% acetonitrile in ddH2O over 60 min. Each chromatographic peak was identified by comparing its retention time with the FDAA derivatives of the standard amino acids. The retention time of the standard derivatives was as follows: 23.2 min for FDAA, 28.8 min for L-phenylalanine and 31.1 min for D-phenylalanine.
2.4. Crystallographic data of compound 1
Yellow crystals of 1 was obtained from MeOH. X-ray diffraction analysis was carried out on an Xcalibur Atlas Gemini Ultra diffractometer (Agilent Technologies) with Cu Kα radiation (λ = 1.54184 Å) at 293 (2) K. Crystal data for compound 1: C42H44N6O6, M = 728.83; needle crystal (0.48 × 0.29 × 0.18 mm3), orthorhombic system, space group P 2(1), a = 9.3167 (2) Å, b = 19.4360 (3) Å, c = 21.0342 (3) Å, α = 90°, β = 90°, γ = 90°, V = 3808.86 (11) Å3, Z = 4, d = 1.271 g/cm3, F(000) = 1544. A total of 6701 reflections were collected in the range 6.2 ≤ 2θ ≤ 134.0, of which 5935 unique reflections with I > 2σ(I) were collected for the analysis. The structure was refined by full-matrix least squares on F 2 using SHELXL-2015 package software. The final reliability factors are: R = 0.0526, wR2 = 0.1451, Flack parameter = 0.07 (16), and the goodness of fit on F2 was equal to 1.037. Crystallographic data for the structure reported in this paper have been deposited at the Cambridge Crystallographic Data Centre under the reference number CCDC 1819264. Copies of the data can be obtained, free of charge, on application to CCDC, 12 Union Road, Cambridge CB2 1EZ, UK (fax: +44-(0)1223–336033 or email: [email protected]).
2.5. ECD calculation
The CIF structure of compound 1was initially optimised at B3LYP/6-31 + g (d, p) level in MeOH using the CPCM polarisable conductor calculation model. The theoretical calculation of ECD was conducted in MeOH using Time-dependent Density functional theory (TD-DFT) at the B3LYP/6-31 + g (d, p) level for one conformer of compound 1. Rotatory strengths for a total of 30 excited states were calculated. ECD spectrum were generated using the program SpecDis 1.6 (University of Würzburg, Würzburg, Germany) and GraphPad Prism 5 (University of California San Diego, USA) from dipole-length rotational strengths by applying Gaussian band shapes with sigma = 0.3 eV.
2.6. Cytotoxicity bioassays
The cytotoxicity was measured by the MTT assay against the prostate cancer PC3 cell line. Tumour cell lines were seeded in 96-well plates (4 × 103 per well in 100 μL). After 24 h of incubation cells were treated with gradient concentrations (100 μmol/L, 50 μmol/L, 25 μmol/L, 12.5 μmol/L, 6.25 μmol/L, 3.125 μmol/L) for another 72 h. Afterwards, MTT solution (5.0 mg/mL in RPMI-1640 media, Sigma, St. Louis, MO, USA) was added (20 μL/well) and then plates were incubated for another 4 h at 37 °C. The compounds were dissolved in DMSO and cell growth inhibition assay was performed as reported previously. The growth inhibitory ability of the compounds was calculated and expressed using the IC50 value by the software of Dose-Effect Analysis. Doxorubicin (ADR) was used as a positive control (Wang et al. Citation2006).
3. Results and discussion
Compound 1 was purified as white powder. The 1H NMR and 13C NMR spectra showed 16 protons and 20 carbon signals, respectively (, Figure S3 and S4, Supplementary data). While the quasi-molecular ion peak at 665.2868 for [M + H]+ obtained from ESI-HRMS revealed a molecular formula of C40H36N6O4 (calcd.665.2871 for C40H37N6O4), suggesting 1 to be a symmetric dimer. 1H-1H COSY cross peaks for H-5 (δH 6.94), H-6 (δH 6.72), H-7 (δH 7.19) and H-8 (δH 6.71), together with HMBC correlations from H-7 and H-8 to C-9 (δC 150.1), H-5 and H-6 to C-4 (δC 125.7) concluded one ortho-disubstituted benzene fragment. The tryptophan-like moiety (Unit A) was established by 1H-1H COSY cross peaks for H-12 (δH 2.17, 2.29) and H-11 (δH 1.89), combined with the key HMBC correlations of H-2 (δH 5.03) with C-3 (δC 58.0) and C-9, H-5 with C-3 and C-4, H-11 with C-3 and C-16 (δC 166.1), H-12 with C-13 (δC 169.5), C-2 (δC 78.2), C-3 and C-4 (). The left signals of six olefinic carbon at δC 133.8 (C-18), 129.6 (C-19/C-23), 128.3 (C-20/C-22) and 126.8 (C-21), combined with the five olefinic protons at δH 6.98 (H-19/H-23), 6.85 (H-20/H-22), 6.84 (H-21) lead to a monosubstituted benzene fragment, which was connected to be a phenylalanine residue (Unit B) by the 1H-1H COSY cross peaks for H-15 (δH 4.13) and H-17 (δH 3.16, 2.83), together with HMBC correlations from H-15 to C-16 and C-18, H-17 to C-16, C-18 and C-19 (). The HMBC correlations of H-15 with C-13 and H-2, H-12 with C-16 connected unit A with B, possessing an indole diketopiperazine monomer (). The only left C-3 site was the position that connected the two monomers. Therefore, the planner structure of 1 was finally determined to be a twofold symmetrical dimer (), which was the same as the known compound 4 (WIN 64821) (Barrow et al. Citation1993).
Comparison of the 1H-NMR data between compounds 1 and 4 revealed a significant difference that there was an unusual up-field proton signal for H-11 (δH 1.89) in compound 1. It was possibly caused by the positive shielding effect of the adjacent aromatic ring as the α-H of amino acid residue found in asperazine (Varoglu et al. Citation1997). It was reported that the positive or negative cotton effect at 254 nm and 300 nm in the electronic circular dichroism (ECD) spectrum depended on the stereochemistry of C-2/C-2’ and C-3/C-3’ (Barrow et al. Citation1993). Compound 1 showed positive cotton effect at 254 nm and 300 nm, same as WIN 64821, which indicated the chirality of C-2/C-2’ and C-3/C-3’ has not changed, both were R configuration (). The calculated ECD spectrum of this configuration at 254 nm was the same as experiment result, while the theoretical data at 300 nm did not yield (). It is speculated that the n-π transition signal of carbonyl is about 300 nm. The theoretical calculations suggested the carbonyl group had little effect on the configuration change may be the reason that no calculation results were obtained at 300 nm. The acid hydrolysates of compound 1 reacted with Marfey’s reagent (FDAA) showed the presence of L-phenylalanine residue by HPLC analysis (Figure S10, Supplementary data). Therefore, the absolute configuration at C-15/C-15’ was determined as S. The up-field shifting was speculated to be caused by the positive shielding effect of the aromatic ring of phenylalanine residue, so that H-11 and H-15 were in the opposite side of the piperazine ring. Because there was no change of chirality occurred at C-15/C-15’, the configuration at C-11/C-11’ were inverse to R, which was different from the reported compound WIN 64821. Finally, the speculation described above was proved by the X-ray crystallographic data () and the absolute stereochemistry of compound 1, the symmetrical dimer, was determined as 2 R, 2ʹR, 3 R, 3ʹR, 11 R, 11ʹR, 15S, 15ʹS.
Figure 3. (a) ECD spectra of compounds 1 and 4. (b) Experimental and calculated ECD spectra of compound 1.
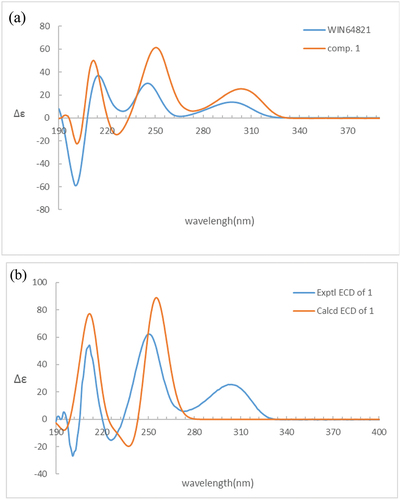
Compound 2 was isolated as white powder. The quasi-molecular ion peak at 665.2863 for [M + H]+ obtained from ESI-HRMS revealed a same molecular formula of C40H36N6O4 (calcd. 665.2871 for C40H37N6O4) as compound 1. The 13C NMR spectrum showed 40 carbon signals, combined analysis with DEPT and HSQC spectra revealed the presence of four amide carbonyls (δC 167.2, 168.9, 168.7, 165.7), four methylene carbons (δC 35.4, 36.7, 40.2, 40.4), six aliphatic methine carbons (δC 54.8, 56.6, 58.7, 58.3, 78.3, 80.3), two aliphatic quaternary carbons (δC 58.1, 58.5), six olefinic quaternary carbons (δC 126.4, 129.7, 148.4, 150.1, 134.5, 134.0) and eighteen olefinic methine carbons (). It was found that the 1H and 13C NMR signals were twice that of compounds 1 and 4, suggesting 2 to be an asymmetric dimer composed of two stereochemistry different monomers. The planner structure of compound 2 was elucidated by 1D and 2D NMR spectra (). The 1H-1H COSY cross peaks for H-12 (δH 2.60, 2.24) and H-11 (δH 2.31), combined with the key HMBC correlations of H-2 (δH 4.83) with C-3 (δC 58.1) and C-9 (δC 148.4), H-5 (δH 7.08) with C-3 and C-4 (δC 129.7), H-11 with C-2 (δC 78.3), C-3 and C-13 (δC 168.7), H-12 with C-13 (δC 168.9), C-2 (δC 80.3), C-3 and C-4 constructed the tryptophan-like moiety (). The 1H-1H COSY cross peaks for H-15 (δH 4.10) and H-17 (δH 3.19, 2.83), together with HMBC correlations from H-15 to C-16 (δC 167.2) and C-18 (δC 134.5), H-17 to C-16, C-18 and C-19 (δC 129.1) lead to a phenylalanine residue (). The HMBC correlations of H-15 with C-13 and H-2, H-12 with C-16 connected tryptophan-like moiety with phenylalanine residue, possessing an indole diketopiperazine monomer (). The left signals were used to elucidate the other monomer. Then the left C-3 site was the position that connected the two monomers. Therefore, the planner structure of 2 was finally determined, which was the same as compound 1 mentioned above.
Detailed analysis of the 1H NMR spectrum of compound 2 revealed two unusual up-field proton signals for H-11 (δH 2.31) and H-11’ (δH 2.21), which were estimated to be affected by the positive shielding effect of the phenyl ring of phenylalanine residue as well according to the configuration of compound 1. Therefore, H-11 and H-15, H-11’ and H-15’ were on the opposite side of the respective piperazine ring. Hydrolysis in HCl, followed by Marfey’s derivatisation, showed the presence of D- and L-phenylalanine residues in the structure of 2 (Figure S19, Supplementary data), which indicated the absolute configurations at C-15 and C-15’ were different. The ECD spectrum of compound 2 showed positive cotton effect at 254 nm and 300 nm (), suggesting the chirality of C-2/C-2’ and C-3/C-3’ has not changed, still were R. Then the NOESY cross peak of H-2 (δH 4.83) and H-11 suggested the cis-orientation of the two protons, which further defined the absolute configuration at C-11 as S. For H-11 and H-15 were trans-orientation, the chirality of C-15 was determined as R. Simultaneously, C-15’ had the S configuration followed the results of Marfey’s method and the stereochemistry of C-11’ was deduced as R. Finally, the absolute configuration of compound 2 was defined as 2 R, 2ʹR, 3 R, 3ʹR, 11S, 11ʹR, 15 R, 15ʹS ().
Compound 3, sharing the same planner structure of the afore-mentioned compounds, was an asymmetric dimer (). 1H NMR spectrum revealed one unusual up-field proton signals for H-11 (δH 1.98) and one normal proton for H-11’ (δH 4.02) (Table S1, Supplementary data). The up-field shifting was estimated to be caused by the same reason as that for compounds 1 and 2, the positive shielding effect of the phenyl ring. The absolute configurations at C-15/C-15’ were both defined as S depending on Marfey’s results (Figure S29, Supplementary data). Thus, the chirality of C-11 and C-11’ was determined as R and S, respectively. The ECD spectrum of compound 3 showed positive cotton effect at 254 nm and 300 nm (Figure S30, Supplementary data), suggesting the chirality of C-2/C-2’ and C-3/C-3’ has not changed, still were R. Therefore, the absolute configuration of compound 3, which was consistent with the compound reported in the literature (Gu et al. Citation2019), was defined as 2 R, 2ʹR, 3 R, 3ʹR, 11 R, 11ʹS, 15S, 15ʹS.
All the four compounds were tested for their in vitro cytotoxicity against the prostate cancer PC3 cell line by MTT method. Unfortunately, all of them were found to be inactive, displaying IC50 values >40 μM. Complex structural and stereoisomeric modification might influence the biological effects of the target compounds through the alteration of the shape or rigidity of the scaffold (Giessen et al. Citation2013; Giessen and Marahiel Citation2015). As some members of dimeric diketopiperazine encompass a vast spectrum of biological properties, it is suggested that the four compounds’ probability possesses an alternative and undefined biology that can be studied in further research.
4. Conclusions
Diketopiperazine scaffold, the smallest of all cyclopeptides, frequently embedded in complex metabolic architectures. Dimeric diketopiperazines are a relevant variation of this class of natural products occupying an enormous chemical complexity. So far, the biosynthesis of mainframe structure of diketopiperazine was found to be catalysed by nonribosomal peptide synthetases (NRPSs) and cyclodipeptide synthases (CDPSs) (Gondry et al. Citation2009; Belin et al. Citation2012; Borgman et al. Citation2019). Tailoring enzymes, including oxidoreductases and prenyltransferases, modify the initially assembled diketopiperazine, and cytochrome P450 responsible for the dimerisation of diketopiperazine monomers (Mishra et al. Citation2017; Shende et al. Citation2020). Compounds 1–4 were dimeric diketopiperazine stereoisomers, whose configuration was mainly different at C-11/C-11’ and C-15/C-15’ positions, while C-2/C-2’ and C-3/C-3’ positions were identical, indicating the biosynthesis of the four stereoisomers might be carried out by using different chiral phenylalanine and tryptophan as substrates, then dimerised by a possible P450 enzyme at C-3/C-3’ spot. Although the four dimeric diketopiperazine stereoisomers showed noncytotoxic against prostate cancer PC3 cell line, due to the diverse biological activities this class of compounds possess, they still provide attractive new targets for developing on the diketopiperazine scaffold to support future drug discovery.
Acknowledgements
We thank Ms. Jianyang Pan (Pharmaceutical Informatics Institute, Zhejiang University) for performing NMR spectrometry for structure elucidation. We are grateful to Mr. Jiyong Liu (Department of Chemistry, Zhejiang University) for the test and analysis of the X-ray diffraction.
Disclosure statement
No potential conflict of interest was reported by the author(s).
Additional information
Funding
References
- Barrow CJ, Cai P, Snyder JK, Sedlock DM, Sun HH, Cooper R. 1993. WIN 64821, a new competitive antagonist to substance P, isolated from an Aspergillus species: structure determination and solution conformation. J Org Chem. 58(22):6016–6021. doi:10.1021/jo00074a031.
- Belin P, Moutiez M, Lautru S, Seguin J, Gondry M. 2012. The nonribosomal synthesis of diketopiperazines in trna-dependent cyclodipeptide synthase pathways. Nat Prod Rep. 29(9):961–979. doi:10.1039/c2np20010d.
- Borgman P, Lopez RD, Lane AL. 2019. The expanding spectrum of diketopiperazine natural product biosynthetic pathways containing cyclodipeptide synthases. Org Biomol Chem. 17(9):2305–2314. doi:10.1039/c8ob03063d.
- Fujimoto H, Sumino M, Okuyama E, Ishibashi M. 2004. Immunomodulatory constituents from an Ascomycete, Chaetomium seminudum. J Nat Prod. 67(1):98–102. doi:10.1021/np0302201.
- Giessen TW, Marahiel MA. 2015. Rational and combinatorial tailoring of bioactive cyclic dipeptides. Fron Microbiol. 6:785. doi:10.3389/fmicb.2015.00785.
- Giessen TW, Tesmar AMV, Marahiel MA. 2013. A trna-dependent two-enzyme pathway for the generation of singly and doubly methylated ditryptophan 2,5-diketopiperazines. Biochemistry. 52(24):4274–4283. doi:10.1021/bi4004827.
- Gondry M, Sauguet L, Belin P, Thai R, Amouroux R, Tellier C, Tuphile K, Jacquet M, Braud S, Courçon M, et al. 2009. Cyclodipeptide synthases are a family of trna-dependent peptide bond-forming enzymes. Nat Chem Biol. 5(6):414–420. doi:10.1038/nchembio.175.
- Gu BB, Gui YH, Liu L, Su ZY, Jiao WH, Li L, Sun F, Wang SP, Yang F, Lin HW. 2019. A new asymmetric diketopiperazine dimer from the sponge-associated fungus Aspergillus versicolor 16F-11. Magn Reson Chem. 57(1):49–54. doi:10.1002/mrc.4780.
- Jia B, Ma YM, Liu B, Chen P, Hu Y, Zhang R. 2019. Synthesis, antimicrobial activity, structure-activity relationship, and molecular docking studies of indole diketopiperazine alkaloids. Front Chem. 7:837. doi:10.3389/fchem.2019.00837.
- Klas KR, Kato H, Frisvad JC, Yu F, Newmister SA, Fraley AE, Sherman DH, Tsukamoto S, Williams RM. 2018. Structural and stereochemical diversity in prenylated indole alkaloids containing the bicyclo[2.2.2]diazaoctane ring system from marine and terrestrial fungi. Nat Prod Rep. 35(6):532–558. doi:10.1039/C7NP00042A.
- Li GY, Yang T, Luo YG, Chen XZ, Fang DM, Zhang GL. 2009. Brevianamide J, a new indole alkaloid dimer from fungus Aspergillus versicolor. Org Lett. 11(16):3714–3717. doi:10.1021/ol901304y.
- Ma YM, Liang XA, Kong Y, Jia B. 2016. Structural diversity and biological activities of indole diketopiperazine alkaloids from fungi. J Agric Food Chem. 64(35):6659–6671. doi:10.1021/acs.jafc.6b01772.
- Mishra A, Choi J, Choi SJ, Baek KH. 2017. Cyclodipeptides: an overview of their biosynthesis and biological activity. Molecules. 22(10):1796. doi:10.3390/molecules22101796.
- Nishiuchi K, Ohashi H, Nishioka K, Yamasaki M, Furuta M, Mashiko T, Tomoshige S, Ohgane K, Kamisuki S, Watashi K. 2022. Synthesis and antiviral activities of neoechinulin B and its derivatives. J Nat Prod. 85(1):284–291. doi:10.1021/acs.jnatprod.1c01120.
- Sedlock DM, Barrow CJ, Brownell JE, Hong A, Gillum AM, Houck DR. 1994. WIN 64821, a novel neurokinin antagonist produced by an Aspergillus sp. II. Biological activity. J Antibiot. 47(4):399–410. doi:10.7164/antibiotics.47.399.
- Shende VV, Khatri Y, Newmister SA, Sanders JN, Lindovska P, Yu F, Doyon TJ, Kim J, Houk KN, Movassaghi M, et al. 2020. Structure and function of nzeb, a versatile C–C and C-N bond forming diketopiperazine dimerase. J Am Chem Soc. 142(41):17413–17424. doi:10.1021/jacs.0c06312.
- Sun S, Ma K, Tao Q, Han J, Bao L, Liu L, Liu H. 2018. Diketopiperazines and 2h-pyran-2-ones with antioxidant activity from the rice fermented with aspergillus luchuensis. Fitoterapia. 125:266–272. doi:10.1016/j.fitote.2018.01.016.
- Varoglu M, Corbett TH, Valeriote FA, Crews P. 1997. Asperazine, a selective cytotoxic alkaloid from a sponge-derived culture of aspergillus niger. J Org Chem. 62(21):7078–7079. doi:10.1021/jo970568z.
- Wang F, Hua H, Pei Y, Chen D, Jing Y. 2006. Triterpenoids from the resin of styrax tonkinensis and their antiproliferative and differentiation effects in human leukemia HL-60 cells. J Nat Prod. 69(5):807–810. doi:10.1021/np050371z.
- Wang FQ, Tong QY, Ma HR, Xu HF, Hu S, Ma W, Xue YB, Liu JJ, Wang JP, Song HP, et al. 2015. Indole diketopiperazines from endophytic Chaetomium sp 88194 induce breast cancer cell apoptotic death. Sci Rep. 5:9294. doi:10.1038/srep09294.
- Wen H, Liu X, Zhang Q, Deng Y, Zang Y, Wang J, Liu J, Zhou Q, Hu L, Zhu H. 2018. Three new indole diketopiperazine alkaloids from aspergillus ochraceus. Chem Biodivers. 15(4):e1700550. doi:10.1002/cbdv.201700550.
- Wibowo JT, Ahmadi P, Rahmawati SI, Bayu A, Putra MY, Kijjoa A. 2021. Marine-derived indole alkaloids and their biological and pharmacological activities. Mar Drugs. 20(1):3. doi:10.3390/md20010003.
- Yang J, Gong L, Guo M, Jiang Y, An F. 2021. Bioactive indole diketopiperazine alkaloids from the marine endophytic fungus Aspergillus sp. YJ191021. Mar Drugs. 19(3):157. doi:10.3390/md19030157.
- Zhang YH, Geng C, Zhang XW, Zhu HJ, Shao CL, Cao F, Wang CY. 2019. Discovery of bioactive indole-diketopiperazines from the marine-derived fungus penicillium brasilianum aided by genomic information. Mar Drugs. 17(9):514. doi:10.3390/md17090514.
- Zheng CJ, Park SH, Koshino H, Kim YO, Kim WG. 2007. Verticillin G, a new antibacterial compound from bionectra byssicola. J Antibiot. 60(1):61–64. doi:10.1038/ja.2007.8.