Abstract
In mountain regions, ecosystem services provision is strongly linked to land use, topography and climate, where impacts can be expected under global change. For our study site in the Austrian Alps, we examined the relationship between agricultural activities and multiple ecosystem services on landscape scale from past to future. Modelling of future land-use patterns was based on stakeholder workshops considering different socio-economic and climate scenarios. In the past, land-use intensity was reduced resulting in less forage provision but better regulating services. Future scenarios predict contrasting developments; under conditions of global change, farmers shift the focus of their activities towards tourism, but in times of global economic crisis farming becomes more important again. Developing the local economy facilitates new markets for agricultural products, but projected drought periods will cause an abandonment of farmland. While forest regeneration is valuable for regulating services, it reduces the aesthetic value. Both regulating and cultural services decrease when forage provision is optimized. To ensure multiple ecosystem service provision, agricultural management should be related to ecosystem services and included into land-use policies and agricultural incentives.
Introduction
The management of agricultural ecosystems (1) ensures the provision of food, fibre and fuel; (2) regulates the water and carbon balance; (3) guards against natural hazards and (4) preserves the aesthetic value of the landscape (Walz et al. Citation2007; de la Vega-Leinert et al. Citation2008; Leitinger et al. Citation2008; Tappeiner et al. Citation2008; Lavorel et al. Citation2011). Agricultural modernization with specialization, rationalization and mechanization and other more profitable sources of income (Rudel et al. Citation2005) have led to a decrease of the used agricultural area in Europe since the mid-twentieth century (Rabbinge and van Diepen Citation2000). The European Alps are particularly affected by dramatic land-use changes with more intensive use of favourable areas (good climate conditions for agriculture and accessibility with machinery) and a reduction of less favourable areas remaining in use, especially on alpine pastures (Rutherford et al. Citation2008). Abandonment of agricultural areas results in forest re-growth, leading to more homogeneous landscape patterns (Tasser et al. Citation2007). By the end of the twentieth century, change rates seem to decelerate (Schneeberger et al. Citation2007), and Tilman et al. (Citation2001) even predicted an increase of arable land and pastures.
Agricultural productivity relies on a range of supporting and regulating services (Swinton et al. Citation2007; Zhang et al. Citation2007). At the same time, agricultural ecosystems on their part influence ecological functions (Swift et al. Citation2004; Stallman Citation2011) and management activities can lead to altered ecosystem services (Lavorel et al. Citation2011), e.g. downstream water quality (Gordon et al. Citation2010), nitrogen and phosphorus soil balances (Bouwman et al. Citation2009), CH4 and N2O emissions (Pitesky et al. Citation2009). Land-use changes related to an intensification of agricultural production are considered a major threat for the capacity of ecosystems to provide multiple ecosystem services (Foley et al. Citation2005; Metzger et al. Citation2006). While the increasing demand for food, energy and water leads to changes in land-use pattern, climate change causes water stress and vegetation shifts (Theurillat and Guisan Citation2001; Schröter et al. Citation2005). To analyse future impacts of human activities on ecosystem services, management scenarios for decision-making are used (de Groot et al. Citation2010; Swetnam et al. Citation2011).
An increasing number of studies have examined ecosystem services on a global or continental scale (Millennium Ecosystem Assessment Citation2005; Metzger et al. Citation2006; Naidoo et al. Citation2008; Kienast et al. Citation2009), as well as on a regional scale (Chan et al. Citation2006; Egoh et al. Citation2009; Eigenbrod et al. Citation2010). While several studies have addressed the spatial distribution of multiple ecosystem services at the level of a landscape (Troy and Wilson Citation2006; Gimona and van der Horst Citation2007; Egoh et al. Citation2008; Naidoo et al. Citation2008; Nelson et al. Citation2009), few studies were carried out in mountain regions (Grêt-Regamey et al. Citation2008; Lavorel et al. Citation2011). Moreover, there is the need to examine the interdependence of multiple ecosystem services in terms of their synergies and trade-offs (Bennett et al. Citation2009; Nelson et al. Citation2009; Raudsepp-Hearne et al. Citation2010).
In the current study, we aimed our efforts at examining historical and future impacts of altered agricultural activities on multiple ecosystem services of mountain regions. Since ecological functions rely greatly on topography, climatic conditions and vegetation patterns (Dorner et al. Citation2002; Mottet et al. Citation2006; Gellrich and Zimmermann Citation2007), we adapted existing modelling approaches and developed new methods to quantify ecosystem services to the specific characteristics of mountain regions based on a geographic information system (GIS). Future multiple ecosystem services provision was projected based on socio-economic and climate scenarios. The specific objectives were as follows: (1) assessment of socio-economic and climate scenarios by a participatory approach; (2) GIS-based quantification and spatial modelling of multiple ecosystem services; (3) impact analysis of ecosystem services based on historical and future land-use pattern and (4) trade-off analysis of multiple ecosystem services.
Materials and methods
Study area and data collection
The Long Term Ecological Research (LTER) Site Kaserstattalm (11°15′–11°20′ E, 47°5′–47°10′ N) is located in the Stubai Valley in Austria on moderate to steep slopes facing south and southeast (). It extends over an area of 5.1 km² and altitude ranges from 970 to 2535 m. The average annual air temperature and precipitation are 2.4°C and 1100 mm at 1750 m a.s.l., respectively. Agricultural use has shaped the landscape for centuries. The upper part is covered by alpine meadows and pastures managed with different intensities. More than half of these grasslands have been abandoned in recent decades. They are bounded below by open larch forest that changes to dense forest further down. The lower slopes and the valley floor are intensively used and covered mainly by grassland for forage production.
Figure 1. Location of study area in Europe (a) and in the Stubai Valley (b). Land-use pattern for the status quo of the study site ‘Kaserstattalm’ (c).
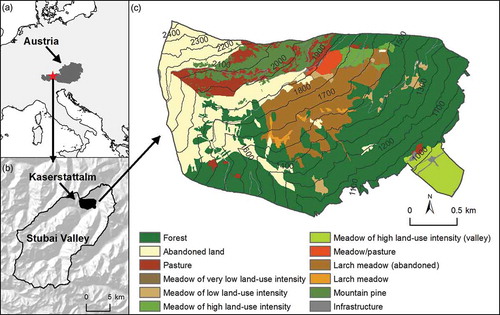
Ecosystem services were modelled based on historical and future land-use pattern, vegetation distribution and topography. Historical land-use maps (1954, 1960, 1970, 1980, 1990, 2001 and 2011) were determined on the basis of orthophotos and historical maps as well as of interviews with farmers. Interpretation and mapping of land-use distribution was done by on-screen digitizing. Missing time periods were integrated using historical data (agricultural census and village chronicles) and free response interviews with all 43 landowners and farmers to get detailed information about land use, i.e. differentiation between meadows and pastures, number of mowings and grazing period. All information was included in the maps that were then checked by the farmers for accuracy (geometry and attributes). For more details on the mapping method used, see Tasser et al. (Citation2009). The vegetation was mapped in the field at a scale of 1:5000, based on orthophotos with a minimum plot-size of 25 m2 for herbaceous plant communities and ∼250 m2 for forests. For more details on the mapping method used, see Tasser and Tappeiner (Citation2002). To derive topographical characteristics (elevation, slope and aspect), which were important for modelling biophysical and climate-dependent factors, a digital elevation model (DEM) with a spatial resolution of 5 m × 5 m was used, provided by the Tyrolean Information System (tiris©) of the State Government of the Tyrol.
Scenarios
To assess future trends, we used different scenarios for (1) socio-economic conditions and (2) climate change.
Socio-economic scenarios
Based on current trends of dynamics in the local development of settlements and job opportunities described by the Intergovernmental Panel on Climate Change (IPCC), the local demand for touristic services and the regional and international market opportunities for agricultural products (Intergovernmental Panel on Climate Change Citation2000), we defined two contrasting socio-economic scenarios: (1a) localized scenario and (1b) globalized scenario. The terms ‘localized’ and ‘globalized’ do not refer to any spatial scale but to the direction of socio-economic development. In the localized scenario, the economic development path assumes rather closed local and regional economic cycles and is largely independent of global economic changes (IPCC storyline B2). In contrast, for a globalized scenario, global competition on mass markets is more important than local and regional economic relations (IPCC storyline A1). Based on stakeholder consultations through expert interviews and group discussions (Lamarque et al. Citation2011), the IPCC scenarios were adapted to the specific conditions of the study area.
To present the scenarios to stakeholders, the following storylines were designed:
• | The ‘localized’ socio-economic scenario (1a) suggests a development that focuses on the local/regional economy and proposes an increase in the number of commuters to the regional capital (within a distance of 20 km) in the lower part of the valley and a focus on sustainable tourism activities (farm holidays, hiking, tobogganing and backcountry skiing in winter). Locally produced food is increasingly in demand and regional and organic products fetch high prices. Farmers receive payments out of the second pillar of the Common Agricultural Policy (CAP) for rural development and agri-environmental measures. National and regional subsidies complement the CAP and also address regionally targeted environmental specifications. The regional and the local administrations contribute financial support for infrastructure (road maintenance, joint processing and marketing facilities) and join use of special agricultural equipment. | ||||
• | The ‘globalized’ socio-economic scenario (1b) is based on the assumption that the study area will aim for competitiveness on a world market. It also forecasts an increasing number of commuters to the regional capital. In the upper part of the valley, tourism is based on an increasing number of ski runs for winter activities, glacier skiing all year round and outdoor adventure activities in summer. This results in mass tourism and new hotel complexes. The farmers are only paid to manage agricultural land according to the recreational demands in specially designated locations. As both residents and tourists are assumed to be price-conscious in their purchasing behaviour for food products, local origin and quality become rather irrelevant. Therefore, local food production is assumed to be not competitive because of declining producer prices on the global market. Agricultural produce, as far as it can be competitive on the world market, is processed in highly centralized facilities outside the valley. Agricultural transfer payments are assumed to have fallen on average by about 20% for mountain farms (European Commission Citation2010). Out of the compensation payments of the second pillar of the CAP for farmers in mountain areas, every farmer still receives a small basic income that increases with the provision of global ecosystem services, such as preservation and enhancement of biodiversity, water quality and plants for CO2 sequestration. These assumptions were based on ‘policy option 3’ as presented in the Communication from the European Commission (European Commission Citation2010). |
Climate scenarios
Climate change scenarios of both General Circulation Models (GCMs) and Regional Climate Models (RCMs) suffer from uncertainties, especially in mountain regions (Beniston Citation2006). To account for regional climate patterns, we used a daily climate change dataset for Austria with a spatial resolution of 1 km² provided by Strauss et al. (Citation2012). The datasets were projected for the period 2008–2040 with ACLiReM (Austrian Climate change Model using Linear Regression, Strauss et al. Citation2011) based on historical daily weather station data from 1975 to 2007 and linear regression with repeated bootstrapping. Starting from the status quo (SQ), we defined three different climate scenarios for our study site: (2a) 5-year scenario, (2b) 20-year scenario and (2c) worst-case scenario. Strauss et al. (Citation2012) calculated three different temperature scenarios. We selected the ‘high’ temperature scenario (i.e. maximum mean temperature for the period 2008–2040). For changing precipitation rates, there is no clear signal for the next three decades and Strauss et al. (Citation2012) provide 17 different scenarios for precipitation. In line with Beniston (Citation2006), we selected increasing daily winter precipitation (September–February) by 10% and decreasing daily summer precipitation (March–August) by 10% for the 5-year and 20-year scenarios. For the worst-case scenario, we used decreasing daily precipitation by 20%.
To present the scenarios to stakeholders, the following storylines were designed:
• | In the 5-year scenario (2a), dry years are alternating with normally wet years and occur in three out of 5 years. For dry years, spring-time conditions are dry with very little run-off due to missing melting water from the tops. The summers are characterized by little rainfall and high temperatures (). Precipitation in winter falls almost exclusively as rain and leads to a shorter period of solid snow cover. In dry years, vegetation below ∼1300 m experiences extreme drought stress and hay yield is only 50% of the usual amount (Egger et al. Citation2005). Table 1. Mean annual temperature, annual precipitation and summer precipitation (April–September) of the status quo and scenarios for normal and dry years | ||||
• | For the 20-year scenario (2b), the trend with increased temperatures and variable precipitation patterns continues and leads to warm winter months with very little snow (). We assume that dry years occur in 7 out of 10 years (Abegg Citation1996; Steiger Citation2010). Mainly on alpine meadows and pastures, average years produce little loss of yield (∼–10%), while dry years reduce agricultural yields by about 50% (Egger et al. 2005; Pfurtscheller 2012, personal communication). Snow cover becomes unreliable below 1500 m a.s.l. and the conditions for artificial snow-making are getting poorer. Ski tourism is only profitable above 1800 m a.s.l. (Steiger Citation2010). | ||||
• | In the worst-case scenario (2c), most years are very dry, with climatic conditions similar to the dry years of the 20-year scenario. As snow reliability fails (Abegg Citation1996; Steiger Citation2010) and springs are dry, winter tourism collapses and hay yields are rarely good enough to make farming feasible. |
Workshops
The two socio-economic scenarios (termed ‘localized’ and ‘globalized’, see above) were combined with the climate scenarios, resulting in six different overall scenarios: (1) localized 5-year LN(5), (2) localized 20-year LN(20), (3) localized worst-case LW(20), (4) globalized 5-year GN(5), (5) globalized 20-year GN(20) and (6) globalized worst-case GW(20). To assess the management implications of the different scenarios, two workshops, one for the localized scenarios and another one for the globalized scenarios, were organized with 4–6 farmers from the Stubai Valley in each session. A qualitative approach was used to select farmers that represent typical situations to get a ‘public opinion, collective attitudes and ideologies’ (Mayring Citation2002) in the context of future land-use management.
To capture various opinions and solutions, the farmers were selected by their socio-economic profiles, characterized by their modern or traditional habitus (Schallberger Citation1999) and their approach towards market and nature. This was reflected in different farming styles (Van der Ploeg and Long Citation1994), combining production patterns (organic or conventional, high or low mechanization) with market approaches (direct/indirect marketing channels) and different employment situation (i.e. part-time, or full-time, employed or self-employed). During the workshops, the farmers were asked to develop a joint strategy to react to the specific scenarios. In addition, they were to explain related land-use changes. For the study area, spatial mapping of the scenarios was done on orthophotos by the farmers and later digitalized.
Modelling ecosystem services
Lamarque et al. (Citation2011) identified a common set of ecosystem services of mountain grasslands that were considered important by local stakeholders and farmers for the Stubai Valley (aesthetic value, carbon sequestration, forage quality, forage quantity, natural hazard regulation, pollination, recreation, soil stability, water quality and water quantity). Accordingly, we modelled a selection of ecosystem services for our study site ‘Kaserstattalm’ based on land-use pattern, vegetation distribution and topographic characteristics using 5 m × 5 m resolution data. We adapted the different GIS-based modelling approaches, which are described in the following in detail, to the specific topographic conditions of mountain regions to quantify the ecosystem services on a landscape scale. Raster maps were created for historical dates and future scenarios and rescaled to values ranging from 0 to 100. For reporting, we calculated mean values of the total study area.
Aesthetic value
The aesthetic value was assessed by a photo survey (see supplementary online material http://informahealthcare.com/doi/suppl/[10.1080/21513732.2012.751936]). The standardized questionnaire was composed of five photographic series, each consisting of four photographs, related to (1) different management types of alpine grassland (pasture, meadow and abandoned land), (2) different management intensities of meadows (number of mowings and fertilization), (3) different successional stages of abandoned land, (4) different management types of alpine grassland at flowering season (meadow of low/high land-use intensity, pasture and abandoned land) and (5) different management types of alpine grassland after cutting or grazing. A total of 78 persons, locals and tourists, were interviewed at the alpine hut, which is located at 1900 m a.s.l. on our study site ‘Kaserstattalm’ and asked to give scores to the pictures of each series according to their preferences (1 for least preferred and 4 for most preferred). To map the aesthetic value, we linked the resulting preference values to the different land-use categories.
Carbon sequestration
Carbon density (Mg C ha−1) assigned to each vegetation type was derived from above-ground and below-ground phytomass (Mg ha−1) and C-stocks (g g−1) resulted from project-area-relevant literature and own measurements. Above-ground phytomass was measured by harvesting or cutting the vegetation of defined plots. Below-ground phytomass was determined by taking soil cores and manual excavation of roots. For further details, refer to Tappeiner et al. (Citation2008). Phytomass and C-content were multiplied and an area-weighted mean value for each land-use category was obtained by overlaying the vegetation and the land-use maps.
Forage quality
Each vegetation type was assigned a forage quality value according to Klapp et al. (Citation1953). By overlaying the vegetation and the land-use maps, an area-weighted mean value for each land-use category was obtained. To account for changing climate conditions within land-use types, each raster cell was corrected by the elevation according to Egger (Citation1999), where forage quality increases below 1800 m a.s.l. (10% per 100 m) and decreases above 2200 m a.s.l. (10% per 200 m). This leads to a differentiation of our community-weighted mean for climatic and soil differences.
Forage quantity
Forage quantity is a function of topography and land-use pattern and was estimated on the basis of productivity type of grassland, determined by plant species, and the growing season (Egger et al. Citation2005). Using the DEM, the days with vegetation growth were determined according to Harflinger and Knees (Citation1999) for the climate zone inner-alpine west. Higher mean temperatures of the scenarios are considered by lowering the DEM with the temperature lapse rate of 6°C per km of elevation (Körner Citation2003). The forage quantity was corrected by topography, i.e. slope and aspect, which can accelerate or diminish vegetation growth. As precipitation requirements for forage are 100 mm precipitation per 1000 kg forage (Egger et al. Citation2005), the maximum possible forage quantity is limited by the total amount of summer precipitation (April–September).
Natural hazard regulation
Natural hazards in mountain regions are mainly mass movements, i.e. rock fall and landslides, debris flows and avalanches. As rock fall is no major issue in our study area, natural hazard regulation was modelled on the basis of (1) snow gliding, (2) surface water run-off and (3) root density. Avalanches are often caused by snow gliding (Clarke and McClung Citation1999). To predict snow gliding, six key drivers (forest stand, slope angle, winter precipitation, surface roughness, slope aspect west and slope aspect east) were used (Leitinger et al. Citation2008). Snow gliding also increases the probability of landslides (Tasser et al. Citation2003). Surface water run-off, contributing to landslides, mudflows and floods, is a function of above-ground phytomass, skeleton fraction-soil stone content in 0–0.1 m soil depth, annual precipitation and elevation (Leitinger et al. Citation2010). Root density is related to slope stability (Reubens et al. Citation2007). We mapped root mass associated to each vegetation type (Tappeiner et al. Citation2008) and obtained an area-weighted mean value for each land-use category by overlaying the vegetation and the land-use maps. To determine natural hazard regulation, the three input factors snow gliding, surface water run-off and root density were added for each raster cell.
Soil stability
To predict the soil stability, we used the universal soil loss equation (USLE, Wischmeier and Smith Citation1978). The rate of soil erosion is a function of land-use pattern, soil type, precipitation and topography. The erosivity factor – the greater the intensity and duration of the rain storm, the higher the erosion potential – was assumed to be equal throughout the study area and therefore excluded from calculation as we found no significant differences in rainfall intensity (mm per time unit) between our climate stations at 970, 1750, 1850 and 2000 m a.s.l. Slope characteristics were derived from the DEM. As no data about soil characteristics were available, we used root density, which is related to soil stability (Reubens et al. Citation2007). Root mass associated with each vegetation type (Tappeiner et al. Citation2008) was overlaid on the land-use map and an area-weighted mean value for each land-use category calculated.
Multiple ecosystem services analysis
A multiple ecosystem services map was obtained by the sum of all ecosystem services using 0–100 scaled values. Impacts of land-use change were visualized by creating maps of ecosystem service change. To understand trade-offs and synergies between different ecosystem services, a principal component analysis (PCA) was performed in ArcGIS™ (Version 9.3.1, Spatial Analyst, ESRI) for historical dates and future scenarios. Using the six different ecosystem services maps as input raster layers, eigenvectors, eigenvalues, a covariance matrix and a correlation matrix were calculated, and maps of the components were generated.
Results
Scenario workshop
During the workshop, the farmers specified that their decisions about intensity and type of agricultural use are driven by different key factors: agricultural policies, climate conditions, nature protection, topography and economic motivations. The general strategies for responding to the different socio-economic and climate scenarios are representative for the whole region. Spatial distribution of land-use patterns was mapped only for the study area ‘Kaserstattalm’.
In the globalized scenarios, the farmers shift their activities towards tourism (in which most of them are already engaged part-time) and continue managing alpine grasslands and pastures for landscape conservation. Large meadows are managed as long as they can be mown by machinery; otherwise, they are transformed into pastures. The farmers react to global pressure and decreasing market-prices with a shift in livestock, i.e. from cattle to sheep and goats. Higher temperatures due to climate change allow a diversification of products, i.e. vegetables or wine-growing, and animals can stay longer on pastures. Irrigation might become necessary for agricultural use on the valley floor, but the farmers were convinced that the glacier will continue to provide the required amount of irrigation water, even in times of drought. For the globalized worst-case scenario GW(20) farmers assume a global financial crisis. In times of economic crisis, with scarce employment opportunities, farming becomes more important. Hence, almost any available area is used for agriculture to cover the subsistence food requirements of the region.
During the workshop on the localized scenarios, farmers rated the market opportunities of this scenario as good and considered new crops like pumpkins on the valley floor and new production niches, like dairy sheep farming on the slope. The farmers considered water scarcity due to climate change as the biggest problem. Irrigation, however, was seen as a solution only for some farmers in the valley on areas of high land-use intensity. Farmers on dry slopes would not benefit from irrigation as pumping up water for irrigation was not thought feasible. The use of favourable areas is intensified and diversified for products needed on local markets (e.g. wine, fruits and vegetables). Less favourable areas, especially on hill sites, are largely abandoned or used for grazing with sheep. Livestock farming remains more important than arable farming, even if the proportion of arable land grows. The farmers continue managing their agricultural land as long as it is profitable (also due to payments for landscape preservation) and combine agricultural activities with other occupations, which still allow part-time farming. Continued transfer payments were thought necessary, but the restrictions imposed by the compulsory 5-year period of the contracts were severely criticized. With the prospect of weather conditions possibly varying more frequently from year to year, farmers requested more freedom in their management decisions. As tourism is likely to increase, especially during summer, some areas might be adapted for touristic use, e.g. with golf courses and small artificial lakes. There is no joint strategy for facing possible future socio-economic and climate changes but rather individual solutions that need to be developed and for each farm to find its own niche. The localized worst-case scenario is dominated by an abandonment of farms due to prolonged drought periods and overregulation. Farmers complained that the existing regulations of contractual commitments for transfer payments in the agri-environmental programme (no land-use change within the 5-year period) already hinder innovations. This leads to critical situations, especially in the course of farm succession.
Land-use change
Historical changes
From 1954 to 2011, agricultural use generally decreased (). The main period of abandonment occurred in the 1960s and 1970s. Larch meadows in particular were almost completely abandoned until 2011, leading to an increase of dense forest after several decades. Alpine pastures and meadows were reduced to 38% of the area in 1954, meadows decreasing more rapidly than pastures. On the valley floor, arable land disappeared completely in 1970 and changed to grassland of high land-use intensity.
Table 2. Land-use distribution of the study area ‘Kaserstattalm’ for historical dates, the status quo and the scenarios
Future changes
Land-use changes for the scenarios GN(5) and LN(5) coincide and land-use distribution is similar to the SQ (). This is not astonishing as farmers depend on the transfer payments from agri-environmental programmes with a 5-year contractual period. Half of the abandoned land is used again as alpine pastures and the land-use intensity of meadows has increased. The change from abandoned land to alpine pastures continues with a lower transformation rate for the 20-year scenarios. Future changes are expected to result from either a collapse of the world economy (scenario GW(20)) or over-regulation in times of increased climatic variation. The results of both the scenarios are entirely different. In GW, the post-World War II landscape is re-established, while in scenario LW(20) large parts are expected to be reforested and abandoned. The GW scenario forecasts massive intensification of use and a reversal of almost the entire area to agricultural use. Higher areas are dominated by pastures and meadows of low land-use intensity. On favourable areas, land-use intensity of meadows is high. Even large areas of previously abandoned larch meadows are used again, especially in areas of higher elevation where forest regeneration occurs more slowly. On the valley floor, 30% of the grassland is transformed to arable land. The farmers considered LN(20) as the maximum likely reduction of land use as long as the local conditions allow farming. However, future generations might change occupation and abandon all alpine grassland, leading to an increase of forest and abandoned land. The valley floor is still managed by big farmers.
Ecosystem services
Landscape pattern
Spatial distribution of ecosystem services is related to land-use pattern and topography ( and ). The aesthetic value is highest for meadows of low land-use intensity, followed by abandoned larch meadows. Alpine pastures and meadows are preferred to abandoned land. Least attractive are meadows of high land-use intensity and forest. Carbon sequestration is highest in forests and reaches good values in larch meadows. Meadows of very low land-use intensity and abandoned land store more carbon than alpine pastures, whereas meadows of high land-use intensity and arable land store the least carbon. Meadows of high land-use intensity on the valley floor produce highest forage quantities and have the best forage quality. Production rates and quality diminish with decreasing management intensity and increasing elevation. Pastures have lower forage quality than meadows. Natural hazard regulation is lower for alpine pastures than for meadows of low land-use intensity or abandoned land due to higher soil compaction and higher surface run-off. Forest and larch meadows have highest natural hazard regulation values. Soil stability depends highly on the slope gradient and the vegetation cover. Abandoned land has higher root densities than managed grasslands. Lowest erosion risk arises on the flat valley floor and in forests, followed by larch meadows and abandoned land on moderate slopes.
Historical impacts
Historical land-use changes have led to an increase of the total ecosystem service value but have influenced the analysed ecosystem services to different degrees (). The aesthetic value increased between 1954 and 1990, primarily due to the abandonment of larch meadows, whereas the subsequent decrease was caused by forest regeneration. An increase in carbon sequestration occurred after 1990 because of forest growth. Mean values of forage quality and quantity have risen continuously, particularly between 1970 and 1980. Large areas of alpine pastures and meadows were abandoned and only areas with higher production rates and better forage quality continued to be managed. The total forage amount of the entire study site has decreased by 47% since 1954. Natural hazard regulation and soil stability increased after 1990, when forest replaced larch meadows, and managed grasslands were more and more abandoned.
Future impacts
The total ecosystem service value for all scenarios is lower than the SQ except LW(20) and decreases with time for the globalized scenarios, while decelerating for the localized scenarios (). In the 5-year scenarios, only small changes are predicted for carbon sequestration and soil stability. Less precipitation and higher temperatures generally lower the risk of natural hazards because of less snow cover and reduced surface run-off. Areas for forage production are extended, but mean forage quality and quantity decrease because pastures are lower in forage quality and quantity than meadows. Higher impacts are estimated for the 20-year scenarios. Carbon sequestration, natural hazard regulation and soil stability increase, while mean forage quality and quantity continue to decrease. Highest impacts occur in GW(20), where all ecosystem service values decrease except aesthetic value and natural hazard regulation. Forage production is limited due to significantly lower precipitation. Loss of areas with high land-use intensity for forage production is compensated for by an enormous increase of used areas, especially pastures. Mean forage quantity and quality are therefore lower, but the total forage amount produced by the entire study site reaches more than double that of SQ. Impacts in LW(20) are contrary to GW(20), with a decrease in the aesthetic value and improved regulating services. Agricultural activities continue only on the valley floor. Although provisioning services improve significantly, the total forage amount of the entire study site is only about 17% of that in SQ.
Trade-offs
We applied a PCA for all time steps to assess trade-offs and synergies between multiple ecosystem services. The first two components explain 85–89% of the variance (for SQ, see ). The relationships between the different ecosystem services indicate only little changes across time but differ between different land-use/cover categories (). Thus, we concentrate on the SQ. The first axis is driven by contrasts between regulating ecosystem services, dominated by carbon sequestration and the aesthetic value and forage provision (, ). Regarding different land-use types, the first component has high values for forest, while pastures and meadows have low values (). The second axis is driven by contrasts between the aesthetic value and forage provision (, ). High aesthetic values are associated to extensive
Table 3. Correlation matrix of PCA for the status quo
Discussion
Scenario analysis has often been linked with participatory approaches to understand socio-economic, administrative, cultural, political and environmental dynamics within a particular region (Kok et al. Citation2006; Walz et al. Citation2007). We asked local farmers of the Stubai Valley to specify the management implications arising in different socio-economic conditions under climate change. As key drivers for land-use change the farmers identified agricultural funding policies, market prices and dryer climate conditions. While the farmers keep managing agricultural land in the globalized scenarios and respond to global pressure by diversification of products and livestock, agricultural land is partly abandoned in the localized scenarios (especially on the slopes and on alpine pastures) or transformed into touristic areas. During the next 20 years, about 11% of the area will be subject to land-use changes in GN(20) and 9% in LN(20). GW(20), predicts even changes for 43% of the total area. The farmers considered LW(20), which affects 23% of the area, not very likely to occur since they keep managing agricultural land as long as the local economy allows. However, future generations might decide differently. During the last decade, agriculturally used areas have been widely abandoned in the European Alps, especially in southern Italian and French Alps (Höchtl et al. Citation2005; Giupponi et al. Citation2006; Zimmermann et al. Citation2010). The resulting land-use changes for our study site correspond generally to Europe-wide land-use/cover scenarios (Rounsevell et al. Citation2006; Bayfield et al. Citation2008). Since the Stubai Valley was identified by Tappeiner et al. (Citation2003) as an Alpine ‘standard region’, characterized by agricultural use with a specialization in livestock farming, which is representative for 22% of the European Alps, the study results are likely to correspond to the development of other comparable regions within the European Alps.
Table 4. Eigenvectors of PCA for the status quo
When analysing ecosystem services and their trade-offs, spatial and temporal scales have to be considered (Rodriguez et al. Citation2006). We used different GIS-based modelling approaches to quantify the provision of multiple ecosystem services for historical dates and future scenarios on a landscape scale. In mountain regions, ecosystem services are influenced by topographic characteristics. For provisioning services, we found strong dependencies to topography. As confirmed by Chen et al. (Citation2007), forage quantity decreases with increasing elevation. Many regulating services are also determined by topography, e.g. natural hazard regulation and slope stability are better for smaller slope gradients. Consistent with other studies, different ecosystem services correspond to landscape pattern (Gimona and van der Horst Citation2007; Naidoo et al. Citation2008; Egoh et al. Citation2009). The aesthetic value is higher for alpine pastures and meadows of low land-use intensity than for forest or meadows of high land-use intensity. Carbon sequestration is higher for forest than for grassland. Service hotspots are larch meadows, which have, however, almost completely disappeared in recent decades. Impacts on multiple ecosystem services can be related to land-use/cover change. Previous land-use changes have led to an increase of all ecosystem service values except the aesthetic value. The scenarios predict a trend reversion for most ecosystem services, apart from natural hazard regulation and the aesthetic value. For modelling future scenarios, we assumed that plant species distribution is only influenced by land use. However, species distribution shifts and even extinction of alpine plants can be expected due to climate change (Thuiller et al. Citation2005; Grabherr Citation2009).
We found trade-offs between provisioning ecosystem services and both regulating and cultural ecosystem services, also confirmed by other studies (Bennett et al. Citation2009; Raudsepp-Hearne et al. Citation2010). Forested areas cannot be used for forage production but are very valuable for carbon sequestration. The aesthetic value is negatively correlated to forage quantity and forage quality, i.e. increasing management intensity leads to higher forage production but reduces the aesthetic value. Comparable to Badgley et al. (Citation2007), who found that trade-offs between agricultural production and many ecosystem services can be avoided by using sustainable management practices, our research findings indicate that extensive use has a positive influence on regulating and cultural ecosystem services. We also found trade-offs between regulating and cultural ecosystem services. While the aesthetic value of dense forest is lower than for grasslands, carbon sequestration and natural hazard regulation are higher. Since managed landscapes are areas of cultural importance, the local population is critical of any shift from rural landscapes to forests (Höchtl et al. Citation2005; Bauer et al. Citation2009), but people living outside the Alps perceive forest regeneration less negatively (Hunziker et al. Citation2008). The results of the survey indicate that tourists appreciate abandoned land and forest better than the local population. We found the highest aesthetic values related to extensively managed grassland or larch meadows and lower values for meadows of high land-use intensity or abandoned land.
In our study, we focused on a selection of ecosystem services to which local stakeholders and farmers attached importance (Lamarque et al. Citation2011). Several ecosystem services related to agriculture have been neglected, including pollination, water quantity and quality and recreation. Moreover, management practices control disservices from agriculture, e.g. habitat loss, nutrient run-off, pesticide poisoning of non-target species (Zhang et al. Citation2007) and future efforts will be concentrated on modelling additional services and disservices to agriculture.
Conclusions
Ecosystem services related to agriculture contribute to human well-being. Provisioning services are directly related to land use, but also regulating and cultural services depend on management practices. Future land-use policies should take into account that ecosystem services in mountain regions are closely linked to topographic and climatic conditions and a more flexible system for financial support could improve the farmers’ options for reacting to climatic variations. Trade-offs are related to land use and occur between provisioning, regulating and cultural services. Service hotspots and multiple ecosystem service provision can be enhanced by sustainable agricultural management. Tourism is likely to be strengthened under both the globalized and the localized scenario and is positively influenced by extensive agricultural management enhancing the aesthetic value. With regard to economic benefits deriving from tourism, landscape preferences linked to agricultural practices should be integrated into land-use policies and agricultural incentives for a sustainable development of mountain regions.
TBSM_A_751936_Supplementary material
Download PDF (1.3 MB)Acknowledgements
We thank the farmers of the Stubai Valley for participating in the scenario workshop and for their valuable inputs. We wish to thank Michael Heinl for assistance during the workshop and for data analyses and Christian Newesely, Stefanie Rauscher and Dagmar Rubatscher for providing data. Three anonymous reviewers are acknowledged for their helpful comments. We also thank Brigitte Scott for language editing. This research was funded by the ERA-Net BiodivERsA, with the national funder FWF, part of the 2008 BiodivERsA call for research proposals. This study was conducted on the LTER site ‘Stubai Valley’, a member of the Austrian LTSER Platform ‘Tyrolean Alps’. The institutions involved are part of the interdisciplinary research centres ‘Ecology of the Alpine Region’ and ‘Mountain Agriculture’ within the research area ‘Alpine Space – Man and Environment’ at the University of Innsbruck.
Notes
Uta Schirpke and Georg Leitinger are joint first authors.
References
- Abegg , B. 1996 . Klimaänderung und Tourismus. Klimafolgenforschung am Beispiel des Wintertourismus in den Schweizer Alpen , Zürich , , Switzerland : vdf Hochschulverlag AG .
- Badgley , C , Moghtader , J , Quintero , E , Zakem , E , Chappell , MJ , Avilés-Vázquez , K , Samulon , A and Perfecto , I. 2007 . Organic agriculture and the global food supply . Renew Agr Food Sys , 22 ( 2 ) : 86 – 108 .
- Bauer , N , Wallner , A and Hunziker , M. 2009 . The change of European landscapes: human-nature relationships, public attitudes towards rewilding, and the implications for landscape management in Switzerland . J Environ Manage , 90 ( 9 ) : 2910 – 2920 .
- Bayfield , N , Barancok , P , Furger , M , Sebastià , MT , Domínguez , G , Lapka , M , Cudlinova , E , Vescovo , L , Ganielle , D , Cernusca , A and al , et . 2008 . Stakeholder perceptions of the impacts of rural funding scenarios on mountain landscapes across Europe . Ecosystems , 11 ( 8 ) : 1368 – 1382 .
- Beniston , M. 2006 . Mountain weather and climate: a general overview and a focus on climatic change in the Alps . Hydrobiologia , 562 ( 1 ) : 3 – 16 .
- Bennett , EM , Peterson , GD and Gordon , LJ. 2009 . Understanding relationships among multiple ecosystem services . Ecol Lett , 12 ( 12 ) : 1394 – 1404 .
- Bouwman , AF , Beusen , AHW and Billen , G. 2009 . Human alteration of the global nitrogen and phosphorus soil balances for the period 1970–2050 . Global Biogeochem Cycles , 23 : GB0A04
- Chan , KMA , Shaw , MR , Cameron , DR , Underwood , EC and Daily , GC. 2006 . Conservation planning for ecosystem services . PLoS Biol , 4 ( 11 ) : e379
- Chen , XF , Chen , JM , An , SQ and Ju , WM. 2007 . Effects of topography on simulated net primary productivity at landscape scale . J Environ Manage , 85 ( 3 ) : 585 – 596 .
- Clarke , J and McClung , DM. 1999 . Full-depth avalanche occurrences caused by snow gliding, Coquihalla, British Columbia, Canada . Canada J Glaciol , 45 ( 151 ) : 539 – 546 .
- de Groot , RS , Alkemade , R , Braat , L , Hein , L and Willemen , L. 2010 . Challenges in integrating the concept of ecosystem services and values in landscape planning, management and decision making . Ecol Complex , 7 ( 3 ) : 260 – 272 .
- de la Vega-Leinert , A , Schröter , D , Leemans , R , Fritsch , U and Pluimers , J. 2008 . A stakeholder dialogue on European vulnerability . Reg Environ Change , 8 ( 3 ) : 109 – 124 .
- Dorner , B , Lertzman , K and Fall , J. 2002 . Landscape pattern in topographically complex landscapes: issues and techniques for analysis . Landscape Ecol , 17 ( 8 ) : 729 – 743 .
- Egger , G. 1999 . Studie im Auftrag von Bundesministerium für Umwelt, Jugend und Familie, Klagenfurt Biotopkartierung Nationalpark Hohe Tauern. Erhebung, Bewertung und Maßnahmenentwicklung ausgewählter Biotope der Außenzone des Nationalparks Hohe Tauern (Tirol)
- Egger , G , Angermann , K , Aigner , S and Buchgraber , K. 2005 . GIS-Gestützte Ertragsmodellierung zur Optimierung des Weidemanagements auf Almweiden , Gumpenstein : BAL – Bundesanst. für Alpenländ. Landwirtschaft .
- Egoh , B , Reyers , B , Rouget , M , Bode , M and Richardson , D. 2009 . Spatial congruence between biodiversity and ecosystem services in South Africa . Biol Conserv , 142 ( 3 ) : 553 – 562 .
- Egoh , B , Reyers , B , Rouget , M , Richardson , DM , Le Maitre , DC and van Jaarsveld , AS. 2008 . Mapping ecosystem services for planning and management . Agric Ecosyst Environ , 127 ( 1–2 ) : 135 – 140 .
- Eigenbrod , F , Armsworth , PR , Anderson , BJ , Heinemeyer , A , Gillings , S , Roy , DB , Thomas , CD and Gaston , KJ. 2010 . Error propagation associated with benefits transfer-based mapping of ecosystem services . Biol Conserv , 143 ( 11 ) : 2487 – 2493 .
- European Commission. 2010. Brussels (Belgium): the CAP towards 2020: meeting the food, natural resources and territorial challenges of the future [Internet]. [cited 2012 Aug 16]. Available from: http://eur-lex.europa.eu/LexUriServ/LexUriServ.do?uri=COM:2010:0672:FIN:en:PDF (http://eur-lex.europa.eu/LexUriServ/LexUriServ.do?uri=COM:2010:0672:FIN:en:PDF)
- Foley , JA , DeFries , R , Asner , GP , Barford , C , Bonan , G , Carpenter , SR , Chapin , FS , Coe , MT , Daily , GC , Gibbs , HK and al , et . 2005 . Global consequences of land use . Science , 309 ( 5734 ) : 570 – 574 .
- Gellrich , M and Zimmermann , NE. 2007 . Investigating the regional-scale pattern of agricultural land abandonment in the Swiss mountains: a spatial statistical modelling approach . Landscape Urban Plan , 79 ( 1 ) : 65 – 76 .
- Gimona , A and van der Horst , D. 2007 . Mapping hotspots of multiple landscape functions: a case study on farmland afforestation in Scotland . Landscape Ecol , 22 ( 8 ) : 1255 – 1264 .
- Giupponi , C , Ramanzin , M , Sturaro , E and Fuser , S. 2006 . Climate and land use changes, biodiversity and agri-environmental measures in the Belluno province, Italy . Environ Sci Policy , 9 ( 2 ) : 163 – 173 .
- Gordon , LJ , Finlayson , CM and Falkenmark , M. 2010 . Managing water in agriculture for food production and other ecosystem services . Agric Water Manage , 97 ( 4 ) : 512 – 519 .
- Grabherr , G. 2009 . Biodiversity in the high ranges of the Alps: ethnobotanical and climate change perspectives . Global Environ Change , 19 ( 2 ) : 167 – 172 .
- Grêt-Regamey , A , Bebi , P , Bishop , ID and Schmid , WA. 2008 . Linking GIS-based models to value ecosystem services in an Alpine region . J Environ Manage , 89 ( 3 ) : 197 – 208 .
- Harlinger , O and Knees , G. 1999 . Klimahandbuch Der Österreichischen Bodenschätzung. Klimatographie. 1. Teil , 1st , Innsbruck (Austria) : Universitätsverlag Wagner .
- Höchtl , F , Lehringer , S and Konold , W. 2005 . “Wilderness”: what it means when it becomes a reality—a case study from the southwestern Alps . Landscape Urban Plan , 70 : 85 – 95 . 1–2
- Hunziker , M , Felber , P , Gehring , K , Buchecker , M , Bauer , N and Kienast , F. 2008 . Evaluation of landscape change by different social groups . Mt Res Dev , 28 ( 2 ) : 140 – 147 .
- 2000 . Emissions scenarios. A special report of working group III of the intergovernmental panel on climate change , Cambridge (UK) : Cambridge University Press . Intergovernmental Panel on Climate Change
- Kienast , F , Bolliger , J , Potschin , M , de Groot , R , Verburg , P , Heller , I , Wascher , D and Haines-Young , R. 2009 . Assessing landscape functions with broad-scale environmental data: insights gained from a prototype development for Europe . Environ Manage , 44 ( 6 ) : 1099 – 1120 .
- Klapp , E , Boeker , P , König , F and Stählin , A. 1953 . Das Grünland , Hannover : Schaper . Wertzahlen der Grünlandpflanzen; p. 38–40
- Kok , K , Rothman , DS and Patel , M. 2006 . Multi-scale narratives from an IA perspective: part I. European and Mediterranean scenario development . Futures , 38 ( 3 ) : 261 – 284 .
- Körner , C. 2003 . Alpine plant life: functional plant ecology of high mountain ecosystems , 2nd , Heidelberg (Germany) : Springer .
- Lamarque , P , Tappeiner , U , Turner , C , Steinbacher , M , Bardgett , R , Szukics , U , Schermer , M and Lavorel , S. 2011 . Stakeholder perceptions of grassland ecosystem services in relation to knowledge on soil fertility and biodiversity . Reg Environ Change , 11 ( 4 ) : 791 – 804 .
- Lavorel , S , Grigulis , K , Lamarque , P , Colace , M , Garden , D , Girel , J , Pellet , G and Douzet , R. 2011 . Using plant functional traits to understand the landscape distribution of multiple ecosystem services . J Ecol , 99 ( 1 ) : 135 – 147 .
- Leitinger , G , Höller , P , Tasser , E , Walde , J and Tappeiner , U. 2008 . Development and validation of a spatial snow-glide model . Ecol Model , 211 ( 3–4 ) : 363 – 374 .
- Leitinger , G , Tasser , E , Newesely , C , Obojes , N and Tappeiner , U. 2010 . Seasonal dynamics of surface runoff in mountain grassland ecosystems differing in land use . J Hydrol , 385 ( 1–4 ) : 95 – 104 .
- Mayring , P. 2002 . Einführung in die Qualitative Sozialforschung , 5th revised , Weinheim (Germany) : Beltz Verlag .
- Metzger , MJ , Rounsevell , M , Michlik , A , Leemans , R and Schröter , D. 2006 . The vulnerability of ecosystem services to land use change . Agric Ecosyst Environ , 114 ( 1 ) : 69 – 86 .
- Millennium Ecosystem Assessment . 2005 . Ecosystems and human well-being: synthesis , Washington , DC : Island Press .
- Mottet , A , Ladet , S , Coqué , N and Gibon , A. 2006 . Agricultural land-use change and its drivers in mountain landscapes: a case study in the Pyrenees . Agric Ecosyst Environ , 114 ( 2–4 ) : 296 – 310 .
- Naidoo , R , Balmford , A , Costanza , R , Fisher , B , Green , RE , Lehner , B , Malcolm , TR and Ricketts , TH. 2008 . Global mapping of ecosystem services and conservation priorities . Proc Natl Acad Sci USA , 105 ( 28 ) : 9495 – 9500 .
- Nelson , E , Mendoza , G , Regetz , J , Polasky , S , Tallis , H , Cameron , DR , Chan , KMA , Daily , GC , Goldstein , J , Kareiva , PM and al , et . 2009 . Modeling multiple ecosystem services, biodiversity conservation, commodity production, and tradeoffs at landscape scales . Front Ecol Environ , 7 ( 1 ) : 4 – 11 .
- Pitesky , ME , Stackhouse , KR and Mitloehner , FM. 2009 . “ Clearing the air: livestock's contribution to climate change ” . In Sparks D, editor. Advances in agronomy , 1 – 40 . Burligton (MA) : Academic Press. p .
- Rabbinge , R and van Diepen , CA. 2000 . Changes in agriculture and land use in Europe . Eur J Agron , 13 ( 2–3 ) : 85 – 99 .
- Raudsepp-Hearne , C , Peterson , GD and Bennett , EM. 2010 . Ecosystem service bundles for analyzing tradeoffs in diverse landscapes . Proc Natl Acad Sci USA , 107 ( 11 ) : 5242 – 5247 .
- Reubens , B , Poesen , J , Danjon , F , Geudens , G and Muys , B. 2007 . The role of fine and coarse roots in shallow slope stability and soil erosion control with a focus on root system architecture: a review . Trees Struct Funct , 21 ( 4 ) : 385 – 402 .
- Rodriguez , JP , Beard , TD , Bennett , E , Cumming , GS , Cork , S , Agard , J , Dobson , A and Peterson , G. 2006 . Trade-offs across space, time, and ecosystem services . Ecol Soc , 11 ( 1 ) : 28
- Rounsevell , MDA , Reginster , I , Araújo , MB , Carter , TR , Dendoncker , N , Ewert , F , House , JI , Kankaanpää , S , Leemans , R , Metzger , MJ and al , et . 2006 . A coherent set of future land use change scenarios for Europe . Agric Ecosyst Environ , 114 ( 1 ) : 57 – 68 .
- Rudel , TK , Coomes , OT , Moran , E , Achard , F , Angelsen , A , Xu , J and Lambin , E. 2005 . Forest transitions: towards a global understanding of land use change . Global Environ Change , 15 ( 1 ) : 23 – 31 .
- Rutherford , GN , Bebi , P , Edwards , PJ and Zimmermann , NE. 2008 . Assessing land-use statistics to model land cover change in a mountainous landscape in the European Alps . Ecol Model , 212 ( 3–4 ) : 460 – 471 .
- Schallberger , P. 1999 . “ Wovon handeln bäuerliche Zukunftsvorstellungen? Determinanten, Dimensionen und Typen (German manuscript of the paper: De quel avenir parlent les paysans?) ” . In On achève bien les paysans. Reconstruire une identité paysanne dans un monde incertain , Edited by: Droz , Y and Mieville-Ott , V . 103 – 126 . Genève (Switzerland) : Edition Georg. p . editors.
- Schneeberger , N , Bürgi , M and Kienast , F. 2007 . Rates of landscape change at the northern fringe of the Swiss Alps: historical and recent tendencies . Landscape Urban Plan , 80 ( 1–2 ) : 127 – 136 .
- Schröter , D , Cramer , W , Leemans , R , Prentice , IC , Araújo , MB , Arnell , NW , Bondeau , A , Bugmann , H , Carter , TR , Gracia , CA and al , et . 2005 . Ecosystem service supply and vulnerability to global change in Europe . Science , 310 ( 5752 ) : 1333 – 1337 .
- Stallman , HR. 2011 . Ecosystem services in agriculture: determining suitability for provision by collective management . Ecol Econ , 71 : 131 – 139 .
- Steiger , R. 2010 . The impact of climate change on ski season length and snowmaking requirements in Tyrol, Austria . Clim Res , 43 ( 3 ) : 251 – 262 .
- Strauss , F , Formayer , H and Schmid , E. 2012 . High resolution climate data for Austria in the period 2008–2040 from a statistical climate change model . Int J Climatol , DOI: 10.1002/joc.3434
- Strauss , F , Schmid , E , Moltchanova , E , Formayer , H and Wang , X. 2011 . Modeling climate change and biophysical impacts of crop production in the Austrian Marchfeld region . Climatic Change , 111 ( 3 ) : 641 – 664 .
- Swetnam , RD , Fisher , B , Mbilinyi , BP , Munishi , PKT , Willcock , S , Ricketts , T , Mwakalila , S , Balmford , A , Burgess , ND , Marshall , AR and al , et . 2011 . Mapping socio-economic scenarios of land cover change: a GIS method to enable ecosystem service modeling . J Environ Manage , 92 ( 3 ) : 563 – 574 .
- Swift , MJ , Izac , AN and van Noordwijk , M. 2004 . Biodiversity and ecosystem services in agricultural landscapes—are we asking the right questions? . Agric Ecosyst Environ , 104 ( 1 ) : 113 – 134 .
- Swinton , SM , Lupi , F , Robertson , GP and Hamilton , SK. 2007 . Ecosystem services and agriculture: cultivating agricultural ecosystems for diverse benefits . Ecol Econ , 64 ( 2 ) : 245 – 252 .
- Tappeiner , U , Tappeiner , G , Hilbert , A and Mattanovich , E. 2003 . The EU agricultural policy and the environment: evaluation of the alpine region , Oxford (UK) : Blackwell .
- Tappeiner , U , Tasser , E , Leitinger , G , Cernusca , A and Tappeiner , G. 2008 . Effects of historical and likely future scenarios of land use on above- and belowground vegetation carbon stocks of an alpine valley . Ecosystems , 11 ( 8 ) : 1383 – 1400 .
- Tasser , E , Mader , M and Tappeiner , U. 2003 . Effects of land use in alpine grasslands on the probability of landslides . Basic Appl Ecol , 4 ( 3 ) : 271 – 280 .
- Tasser , E , Ruffini , F and Tappeiner , U. 2009 . An integrative approach for analysing landscape dynamics in diverse cultivated and natural mountain areas . Landscape Ecol , 24 ( 5 ) : 611 – 628 .
- Tasser , E and Tappeiner , U. 2002 . Impact of land use changes on mountain vegetation . Appl Veg Sci , 5 ( 2 ) : 173 – 184 .
- Tasser , E , Walde , J , Tappeiner , U , Teutsch , A and Noggler , W. 2007 . Land-use changes and natural reforestation in the Eastern Central Alps . Agric Ecosyst Environ , 118 ( 1–4 ) : 115 – 129 .
- Theurillat , J and Guisan , A. 2001 . Potential impact of climate change on vegetation in the European Alps: a review . Climatic Change , 50 ( 1 ) : 77 – 109 .
- Thuiller , W , Lavorel , S , Araújo , MB , Sykes , MT and Prentice , IC. 2005 . Climate change threats to plant diversity in Europe . Proc Natl Acad Sci USA , 102 ( 23 ) : 8245 – 8250 .
- Tilman , D , Fargione , J , Wolff , B , D'Antonio , C , Dobson , A , Howarth , R , Schindler , D , Schlesinger , WH , Simberloff , D and Swackhamer , D. 2001 . Forecasting agriculturally driven global environmental change . Science , 292 ( 5515 ) : 281 – 284 .
- Troy , A and Wilson , MA. 2006 . Mapping ecosystem services: practical challenges and opportunities in linking GIS and value transfer . Ecol Econ , 60 ( 2 ) : 435 – 449 .
- Van der Ploeg , JD and Long , A. 1994 . Born from within: practice and perspectives of endogenous rural development, Van Gorcum, Assen . Styles of farming: an introductory note on concepts and methodology , : 7 – 30 .
- Walz , A , Lardelli , C , Behrendt , H , Grêt-Regamey , A , Lundström , C , Kytzia , S and Bebi , P. 2007 . Participatory scenario analysis for integrated regional modeling . Landscape Urban Plan , 81 ( 1–2 ) : 114 – 131 .
- Wischmeier , WH and Smith , DD. 1978 . Predicting rainfall erosion losses – a guide to conservation planning , Washington , DC : US Department of Agriculture .
- Zhang , W , Ricketts , TH , Kremen , C , Carney , K and Swinton , SM. 2007 . Ecosystem services and dis-services to agriculture . Ecol Econ , 64 ( 2 ) : 253 – 260 .
- Zimmermann , P , Tasser , E , Leitinger , G and Tappeiner , U. 2010 . Effects of land-use and land-cover pattern on landscape-scale biodiversity in the European Alps . Agric Ecosyst Environ , 139 ( 1–2 ) : 13 – 22 .