Abstract
The white-tailed deer (Odocoileus virginianus) is one of the most important species related to sport hunting in northern Mexico. During the last decade, this species has been subjected to intensive breeding to achieve improvements in certain desired traits (i.e., antlers). This alleged intensive management of bringing originally wild populations into captivity might have harmful consequences on genetic diversity. In this short research paper we estimate and discuss the consequences of that transition, as assessed by a microsatellite genetic marker analysis. The results show that no short-term changes in genetic diversity parameters were promoted by captivity; however, a genetic diversity condition maintained by artificial genetic flow was identified, perhaps allowing for the required introgression of gene diversity into this closed population. A wider analysis is recommended and the implications are discussed. Within a realistic forecast of expanding sport hunting, the achievement of useful, pragmatic, and strict conservancy programs of this species, considering approaches such as those used here, will be necessary.
Introduction
Under the Federal Wildlife Law (DOF 2002) of Mexico, the creation of wildlife conservancy and sustainable management units (UMAs for its Spanish acronym) has promoted the diversification of livestock production systems, thereby permitting the exploitation of certain species such as the White-Tailed deer (WTD), Odocoileus virginianus, and allowing its conservancy in closed areas of preservation. Persuaded by this opportunity, some cattle ranchers have transitioned into WTD breeding for game purposes, obtaining substantially more profits than with raising cattle alone. For example, in Tamaulipas, a border state with the United States, the 2008 game season produced over 16 million dollars in net profit (FECANACO 2011).
The creation of UMAs can induce the transition of wild populations into relatively closed populations and can allow for management intensification. Theoretically, this practice could trigger important consequences on genetic variation, promoting genetic drift and reducing the flow of genes (Falconer & MacKay Citation1996; Demandt Citation2010). However, due to a strong interest of the producers in the preservation and improvement of superior trophy animals, restocking is frequent. Records of this practice began in the late 1970s through 1990s, after the original WTD populations were considered almost in extinction (Villarreal Citation1999). In northern Mexico, although subsistence hunting is not as frequent as in the southern and central regions of Mexico (De la Rosa et al. Citation2012), a main concern is a decrease in genetic variability due to an intensification of WTD breeding and management.
Although it is presumed that wildlife ranching has important biological and ecological consequences including diseases or genetic bottlenecks, little research has been focused on this matter (Butler et al. Citation2005). Currently, conservation genetics represent a valuable tool for monitoring the genetic variability of wild animal populations facing management or exploitation risks. Through the use of genetic markers such as microsatellites (DeYoung et al. Citation2003), it is possible to implement strategies to determine genetic variability dynamics over a temporal perspective and to diagnose genetic diversity issues in model populations.
Using a UMA as a current farm model, we compared genetic diversity estimated through microsatellite marker loci in a transitional WTD population to identify evidence of genetic variability loss, inbreeding shifts, and/or effective population size reduction and discuss some of their implications.
Materials and methods
Study population and sample source
The UMA studied is located at km 24.5 of Nuevo Laredo–Anahuac Road, in the Nuevo Laredo municipality of Tamaulipas. The ranch extends to approximately 1000 ha and is surrounded by a high fence. The climate of this region is semiarid with an annual mean rainfall of 40 cm. Vegetation has been classified as Tamaulipan thorn scrub of the south Texas plain (Fulbright & Ortega-S Citation2006).
WTD samples were collected from 2001 to 2008. The organization of ranch management began in 1999 with construction of the first management pens and confinement of animals to the same ranch area. Direct mating and natural inseminations were used since then until 2005. From 2006 to date, assisted reproduction was implemented formerly by intra-cervical insemination and later by laparoscopic inseminations.
The population was structured on the basis of samples collected and grouped into three UMA stages or periods: original (samples collected from 2001 to 2003 of animals in the area surrounding the UMA but outside of the intensive installations), transition (samples collected from 2004 to 2005 while in the process of intensive management), and captive (samples collected from 2006 to 2008 from animals under intensive feeding and reproductive management strategies including artificial insemination and selection). Samples were obtained from adult specimens of both sexes and verified to be randomly selected and to have no direct familiar relationship.
Sample processing
Three types of sample were collected, and genomic DNA extraction was performed according to the sample nature using different commercial kits; tissue and hair follicle DNA was extracted using the Genelute Mammalian Genomic DNA Kit (Cat. G1N350, Sigma-Aldrich Co. LLC, St. Louis, Missouri, USA), and older blood-stain sample DNA from original populations was extracted with a Promega Kit (Cat. A7710, Promega Corp., Madison, Wisconsin, USA).
Genotyping of samples was performed using the 15-microsatellite panel reported by Anderson et al. (Citation2002) and DeYoung and Honeycutt (Citation2005) for WTD. Due to technical limitations, markers were divided into two groups for polymerase chain reaction (PCR) product analysis. The first group of four microsatellites (MAF36, MAF70, D15, and MSTN01) was visualized in a LI-COR 42001G semi-automated sequencer, while the second group of ten markers (BM848, BM6438, D, K, OCAM, CERVID, INRA01, Q, N, BM4208, and ILST011) was visualized by an automated ABI-PRISM™ 3130 sequencer. Optimization of specific PCR was achieved by examining different combinations of DNA, magnesium chloride, and primer concentrations.
Data analysis
Microsatellite genotypes were previously analyzed by MICRO-CHECKER (Van Oosterhout et al. Citation2004) to identify genotyping errors in the microsatellite panel used. Data were then analyzed to estimate the effect of the population’s transition into captivity on the genetic variability and diversity parameters. Expected (HE) and observed (HO) heterozygosities and inbreeding coefficients (FIS) were estimated using GENEPOP’s version 4.0 (Rousset Citation2008; LGE, Montpellier, France). An estimation of private allele frequencies was done using CONVERT software (Glaubitz Citation2004; Purdue University, West Lafayette, IN, USA). Complementarily, genic diversity (G) and allelic richness (Ar) were estimated by FSTAT ver. 2.9.3 (Goudet Citation2001; Lausanne University, Lausanne, Switzerland).
The normality distribution of the parameters was tested by a Shapiro–Wilk W test, and after normality distribution was confirmed, all parameters were analyzed by a GLM procedure to detect significant changes temporally. All analyses were performed using SAS (SAS Institute Inc. 2001, Cary, NC, USA) software.
Genetic differentiation by FST pairwise analysis was computed by ARLEQUIN version 3.11 (Excoffier et al. Citation2005; CMPG, Institute of Ecology and Evolution, University of Bern, Bern, Switzerland) after an AMOVA analysis among the UMA stages. Finally, the effective population size, Ne, was estimated using NeESTIMATOR software (Ovenden et al. Citation2007; Molecular Fisheries Laboratory, University of Queensland, Brisbane, Australia), through linkage disequilibrium test for individual subpopulations (LD Ne). A test to detect recent effective population size reductions under Infinite Allele and Stepwise Mutation models was performed using the BOTTLENECK program (Cornuet & Luikard Citation1997; CBGP, INRA, Montpellier, France).
Results
Genetic parameter estimations and P-values of the mean comparisons are shown in None of the comparisons had significant differences, although a slight reducing trend was observed in all parameters except for FIS. This trend (p > 0.05) is apparent in , with estimates of HO and HE trending toward a slight reduction of parameters in the transition population.
Figure 1. Least square means ± standard errors of expected (HE) and observed (HO) heretozygosities from original to captive white-tailed deer subpopulations.
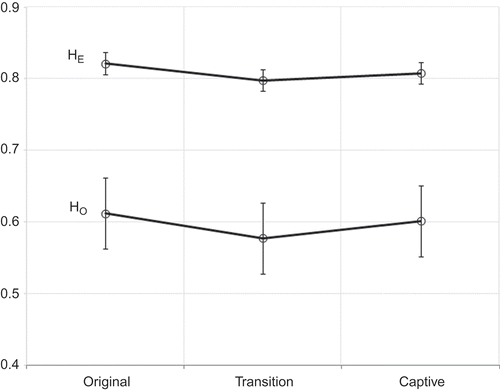
Table 1. Least square means ± standard errors of the genetic variability and diversity parameters of the studied subpopulations.
It is important to remark that the inbreeding coefficient means estimated for the three subpopulations were of considerable size. Because this parameter is a corrected average of the FIS by locus, attention was paid in their computing and comparison. However, no significant differences were identified through population transition. Genetic equilibrium as a consequence was deviated in all three subpopulations for all loci except BM848, CERVID, INRA01, and Q; D and INRA01; and BM6438, D, INRA01, and ILST11 for the original, transition, and captive populations, respectively. An additional comparison showed that the extreme subpopulations had the highest number of private alleles. The original subpopulation exhibited 13 private alleles and the captive subpopulation had 14 private alleles, all within 8 loci. Conversely, the transition population showed only three private alleles in the D15, N, and BM4208 loci.
Furthermore, genetic structure analysis, including a comparison of the temporally separated subpopulations, showed no substantial differentiation between them; the main extent of genetic variability was found within the compared populations (). Subsequent analyses of pairwise FST complemented previous structure analyses supporting significant (p < 0.001) but undifferentiated groups of animals (). Interestingly, a slightly elevated trend in fixation differentiation was observed between the original and captive populations.
Table 2. Analyses of the molecular variance of a transitional population of white-tailed deer.
Table 3. Subpopulation pairwise FST and respective p-values.
Undoubtedly, possible shifting in effective population sizes was an important concern in this analysis. LD Ne estimation showed a positive shift to the captive subpopulation after a reduction in size from the original to transition population that might be supported by gene introgression through reproductive strategies (). No bottleneck events were identified in the adjusted models (p > 0.05), and all populations had normal L-shaped distributions.
Table 4. Linkage disequilibrium of the effective population size of the studied subpopulations.
Discussion
In the present work, genetic marker and statistical strategies were conducted to identify the consequences of WTD population transition in a model population going from natural to farmed one. Under the present analysis of results, no evidence of substantial negative genetic variability shifting was identified. No significant reduction in genetic diversity parameters was estimated (p > 0.05), and although a moderate and sustained level of FIS was found since the captured WTD became the original population, an increase in the effective population size indicated under the conditions of the model farm studied that captivity is not a risk factor for diminishing the genetic variability of original populations of WTD.
Cronin et al. (Citation1995, 2003, Citation2006) have directed their efforts to determining the effects of wildlife ranching or ‘domestication’ on the genetic composition of different deer species. They consider the selection and reduction of populations under domestication a risk factor for genetic variability loss because of founder effects, genetic drift, and selection in populations with small effective sizes (Cronin et al. Citation2009). However, some of their results have shown that genetic variation can be maintained in specific domestic and wild populations (e.g., elk) with appropriate management, and the same might be assumed for other deer species.
For WTD, particular attention would be focused on intensification or induced ‘domestication’ conditions. As Messner (Citation2011) analyzed, a justification by which WTD might be considered in the domestication process is the mutualism identified by its human relationship. Because humans focus upon a morphological trait (i.e., antler development), domestication, would be fulfilled by breeding animals in captivity with specific objectives. Additionally, by subjugation of captive animals through enhanced feeding (nutritional) and water availability and intensified reproductive, selection, and breeding management through mating choice, secondary traits would be favored (Driscoll et al. Citation2009). Although not addressed by the present study, likewise would lost genetic diversity be, an additionally and genuine issue related to the confinement and/or mobilization of breeding stock for genetic improvement purposes as a critical concern is the spreading risk of Chronic Wasting Disease and its catastrophic consequences not only for captive populations but for free ranging animals (Miller Citation2012).
With the realistic forecast of expanding sport hunting, the achievement of useful and pragmatic conservancy programs is necessary. Because large amounts of income are involved in this increasing industry, other national regions will likely explore its potential as an opportunity to diversify livestock production systems. Additionally, the translocation of animals from other states for breeding purposes would become a constant interest. This is not new; as a precedent, animal movement from state to state often introduces subspecies from diverse regions in North America (Marchinton et al. Citation1995). In Mexico, some evidence exists regarding the reintroduction movement of the Texanus WTD subspecies, allowing population recovery by the 1970s (Villarreal Citation1999). Similarly, current border interchange of germplasm from the United States to the border states of Mexico is often used for genetic improvement purposes. The same interaction occurs from Canadian WTD farms that provide ‘shooter bucks’ to the demanding market of the United States (Hudson Citation2009).
Information on the genetic variability dynamics of closed or captive populations of WTD would acknowledge an understanding of its possible consequences, allowing for policy making and a comprehensive application of information for regularly monitoring stock movement or artificial flow (extractive or introductive) and allowing the maintenance of initial genetic diversity.
The analyzed population, as a model UMA, began with captured animals being confined for reproductive purposes. After almost a decade, we can illustrate a four-generational diagnosis of genetic variability, considering an intergenerational interval of 2 years (DeYoung et al. Citation2003). As captive populations are usually founded with small numbers of individuals and with annual culling rates equaling the rate of population increase, allelic frequencies may depart from natural conditions (Hartl et al. Citation2003), and genetic flow and introgression may become a frequent occurrence.
To find a balance between genetic improvement (breeding management) and genetic diversity is a complex task to achieve; it is known that intensive artificial selection may have undesirable consequences, such as decreased effective population size due to the intensive selection of better bucks and closing populations (Falconer & MacKay Citation1996). Since the original breeding stock used by direct mating and AI was necessarily replaced at assessed period (i.e., 8 years), as hypothesized, at least a reduction in genetic estimators was expected. The present study showed reduced heterozygosity and deviation from genetic equilibrium. As theoretically presumed, this was induced by a reduced amount of founders (Hartl et al. Citation2003); therefore, a main precept for captive populations must be in accounting for the enclosure of a sufficient number of founders with a healthy genetic diversity estimator. However, no critical changes in declining genetic variability were observed. It is important to comment that the short period evaluated might not be sufficient to perceive a significant diversity lost; therefore, further monitoring and evaluation of these presented indicators is recommended to clarify these assumptions.
Research with approaches such as those presented here will potentially allow for the creation of the necessary information to justify policy making toward regularization of the intensive management of WTD populations and the revalorized use of other WTD subspecies. In the future, further analyses assessing wider populations and periodically longer periods of UMAs and wild populations might elucidate the reliability of these acknowledged outcomes. Furthermore, as more genomic resources (e.g., SNPs) for WTD species could be available, their inclusion in evaluation studies might provide the better examining of population dynamics and structure (Helyar et al. Citation2011; Kirk & Freeland Citation2011).
Almost all studies involving WTD in Mexico are focused on population density estimations from different regions with conservancy purposes (Mandujano & Gallina Citation1993; Sánchez-Rojas et al. Citation1997; López-Soto & Badii 2000; Mandujano Citation2004; Gallina Citation2010). A widespread consensus involving variability maintenance and preservation, however generalizing, suggests that under actual conditions of UMA regulation almost all WTD distribution regions are in constant threat. Nonetheless, as no data are available regarding furtive hunting in Mexico and considering hunting as the most important source of human-caused mortality (Collins & Kays Citation2011), we might suggest UMA as a realistic method of hunting control under Mexico’s wildlife perspective.
A comprehensive approach would also consist of supporting studies to elucidate the genetic relationships among the presumed 14 WTD subspecies in Mexico. Only a few efforts have been completed. De la Rosa et al. (2012) found evidence of differentiation between the Texanus, Carminis, Veracrusis, Yucatanensis, and Sinaloe WTD subspecies, and Ambriz (Citation2012) found specific variations in genes at mitochondrial level among those subspecies. These findings might suggest the specific genetic or lineage background and necessity for protecting their own genetic diversity; the same assumptions can be made in regions without a geographic barrier and with the same agro-climatic condition, but analyses remain to be done. Documentation of these relationships and the consequences of translocations or reintroductions using the strategies presented here may prevent and avoid further and deeper issues, such as those presented by Hartl et al. (Citation2003) regarding European red deer populations in danger of losing their specific identity.
Conclusions
As the current results showed, no short-term changes in genetic variability were promoted by the captivity of this WTD population, perhaps because artificial genetic flow allowed the required introgression of genetic diversity into this ‘closed population’. This postulation is supported by the clear consequence founded here: the loss and reintroduction of private alleles from the original to captive populations. Further analyses should consider a wider sampling of UMAs to corroborate current inferences and possible negative shifts in other WTD populations. Inclusion of a large number of UMA models will further validate estimations and confirm assumptions.
Acknowledgments
The authors would like to thank the Instituto Politécnico Nacional through the SIP20090633-20100513 project grant. We also thank the Asociación Nacional de Criadores de Cervidos de Mexico A.G. for their genuine support of this research.
References
- Ambriz MP. 2012. Secuenciación y análisis del genoma mitocondrial de subespecies de Venado Cola Blanca (Odocoileus virginianus) [MSc thesis]. Reynosa: National Polytechnic Institute; p. 87.
- Anderson JD, Honeycutt RL, Gonzales RA, Gee KL, Skow LC, Gallagher RL, Honeycutt DA, DeYoung RW. 2002. Development of microsatellite DNA markers for the automated genetic characterization of White-Tailed deer populations. J Wildl Manage. 66:67–74.
- Butler MJ, Teaschner AP, Ballard WB, McGee BK. 2005. Wildlife ranching in North America: arguments, issues, and perspectives. Wild Soc Bull. 33:381–389.
- Collins C, Kays R. 2011. Causes of mortality in North American populations of large and medium-sized mammals. Anim Cons. 14:474–483.
- Cornuet JM, Luikart G. 1997. Description and power analysis of two test for detecting recent population bottlenecks from allele frequency data. Genetics. 144:2001–2014.
- Cronin MA, MacNeil MD, Patton JC. 2006. Mitochondrial DNA and microsatellite DNA variation in domestic reindeer (Rangifer tarandus tarandus) and relationships with wild caribou (Rangifer tarandus granti, Rangifer tarandus groenlandicus, and Rangifer tarandus caribou). J Hered. 97:525–530.
- Cronin MA, Patton JC, Balmysheva N, MacNeil MD. 2003. Genetic variation in caribou and reindeer (Rangifer tarandus). Anim Gen. 34:33–41.
- Cronin MA, Renecker LA, Patton JC. 2009. Genetic variation in domestic and wild elk (Cervus elaphus). J Anim Sci. 87:829–834.
- Cronin MA, Renecker LA, Pierson BJ, Patton JC. 1995. Genetic variation in domestic reindeer and wild caribou in Alaska. Anim Gen. 26:427–434.
- De la Rosa–Reyna X, Calderon–Lobato RD, Parra-Bracamonte GM, Sifuentes-Rincón AM, De Young R, García-De León F, Arellano-Vera W. 2012. Genetic diversity and structure 400 among subspecies of white–tailed deer in México. J Mammal. 93:1158–1168.
- Demandt MH 2010. Temporal changes in genetic diversity of isolated populations of perch and roach. Cons Genet. 11:249–255.
- DeYoung RW, Demarias S, Honeycutt RL, Gonzalez RA, Gee KL, Anderson JD. 2003. Evaluation of a DNA microsatellite panel useful for genetic exclusion studies in white-tailed deer. Wild Soc Bull. 31:220–232.
- DeYoung RW, Honeycutt RL. 2005. The molecular toolbox: genetic techniques in wildlife ecology and management. J Wild Man. 69:1362–1384.
- Driscoll CA, MacDonald DW, O’Brian JO. 2009. From animals to domestic pets, an evolutionary view of domestication. Proc Natl Acad Sci. 106:9971–9978.
- Excoffier L, Laval G, Schneider S. 2005. Arlequin ver. 3.0: an integrated software package for population genetics data analysis. Evol Bioinform Online. 1:47–50.
- Falconer DS, MacKay TFC. 1996. Introducción a la genética cuantitativa. Zaragoza: Acribia; 469 p.
- Federación de Cámaras Nacionales de Comercio. 2011. Tamaulipas perdió en turismo cinegetico 11 mdd en tres años [Internet]. Tamaulipas en Linea, México; [cited 2010 Dec 14]. Available from: http://tamaulipasenlinea.mx/Tamaulipas-perdio-en-turismo-cinegetico-11-mdd-en-tres-anos/
- Fulbright TE, Ortega-S JA. 2006. White–tailed deer habitat: ecology and management on rangelands. Kingsville (TX): A & M University Press; p. 256.
- Gallina S. 2010. UMAs reto para la conservación: situación problemática y perspectivas, con el ejemplo de aprovechamiento del venado cola blanca. In Conference Memories, XII Simposio Nacional de Venados de México, UNAM 23 al 25 de julio del 2010.
- Glaubitz JC. 2004. CONVERT: a user friendly program to reformat diploid genotypic data for commonly used population genetic software packages. Mol Ecol Notes. 4:309–310.
- Goudet J. 2001. FSTAT, a program to estimate and test gene diversities and fixation indices [Internet]. Version 2.9.3 [cited 2012 Dec 17]. Available from: http://www.unil.ch/izea/softwares/fstat.html.
- Hartl GB, Zachos F, Nadlinger K. 2003. Genetic diversity in European red deer (Cervus elaphus L.): antropogenic influences on natural populations. CR Biol. 326:S37–S42.
- Helyar SJ, Hemmer-Hansen J, Bekkevold D, Taylor MI, Ogden R, Limborg MT, Cariani A, Maes GE, Diopere E, Carvalho GR, Nielsen EE. 2011. Application of SNPs for population genetics of nonmodel organisms: new opportunities and challenges. Mol Ecol Res. 11:123–136.
- Hudson RJ, editor. 2009. Livestock diversification: issues and trends. In: Management of agricultural, forestry and fisheries enterprises. Developed under the Auspices of the UNESCO. Oxford: Eolss Publishers; 440 p.
- Kirk H, Freeland JR. 2011. Applications and implications of neutral versus non-neutral markers in molecular ecology. Int J Mol Sci. 12:3966–3988.
- Lopez-Soto JH, Badii MH. 2000. Depredación en crías de venado cola blanca (Odocoileus virginianus texanus) por coyote (Canis latrans) en una unidad de manejo y aprovechamiento del norte de Nuevo León, Mexico. Act Zool Mex (ns). 81:135–138.
- Mandujano S. 2004. Análisis bibliográfico de los estudios de venados en México. Acta Act Zool Mex (ns). 20:211–251.
- Mandujano S, Gallina S. 1993. Densidad del Venado cola blanca basada en conteos en transectos en un bosque tropical de Jalisco. Act Zool Mex (ns). 56:1–37.
- Marchinton RL, Miller KV, McDonald SJ. 1995. Quality Whitetails, the why and how of quality deer management. Mechanicsburg (PA): Stackpole Books. Genetics; p. 169–189.
- Messner TC. 2011. White-tailed deer management strategies and domestication processes. Hum Ecol. 39:165–178.
- Miller JE. 2012. A growing threat: how breeding could put public trust wildlife at risk. The Wildlife Professional, Winter; p. 22–27.
- Ovenden JR, Peel D, Street R, Courtney A, Hoyle S, Peel S, Podlich H. 2007. The genetic effective and adult census size of an Australian population of tiger prawns (Penaeus escullentus). Mol Ecol. 16:127–138.
- Rousset F. 2008. Genepop’007: a complete reimplementation of the Genepop software for Windows and Linux. Mol Ecol Res. 8:103–106.
- Sanchez-Rojas G, Gallina S, Mandujano S. 1997. Area de actividad y uso del hábitat de dos venados cola blanca (Odocoileus virginianus) en un bosque tropical de la costa de Jalisco, México. Acta Act Zool Mex (ns). 72: 39–54.
- Van Oosterhout C, Hutchinson WF, Wills DPM, Shipley P. 2004. MICRO-CHECKER: software for identifying and correcting genotyping errors in microsatellite data. Mol Ecol Res. 4:535–538.
- Villarreal GJG. 1999. Venado Cola Blanca, manejo y aprovechamiento cinegético. General Bravo: Unión Ganadera Regional de Nuevo León; 401 p.