Abstract
Coastal human settlements are becoming increasingly vulnerable to natural disasters such as tsunamis and cyclones. Recent events, including the Indian Ocean Tsunami in 2004 and Typhoon Haiyan in 2013, have brought the issue of coastal protection to the forefront in many countries across the globe. We conducted a review of recent research regarding the extent of coastal protection provided by mangroves that includes observational studies, numerical modeling, and laboratory experiments. We described our findings in a unique outline based on the methodology and event type and concluded that observational studies have not provided conclusive results on the extent of coastal protection provided by mangroves from extreme natural disasters. However, results from several recent numerical and physical models support the mitigating capabilities of mangroves for cyclone storm surges and small tsunamis. Studies on the economic valuation of mangroves have estimated coastal protection to be a major portion of their total value. Further research utilizing robust datasets for multivariate statistical analyses and validation of numerical models is still needed to provide a better assessment of the feasibility of incorporating mangroves into coastal protection plans.
1. Introduction
An estimated 40% of the human population lives in coastal regions (CIESIN Citation2007). Many of these coastal communities are at risk of being affected by tsunamis, cyclones, or both (NOAA Citation2012, Citation2013). These natural disasters can cause either large destructive waves or storm surges, the dangers of which are exacerbated by coastal erosion and sea level rise (Nicholls & Cazenave Citation2010). As aging infrastructure and engineered coastal protection become more outdated, the potential for extremely costly natural disasters increases (Turner et al. Citation1996). In the past decade, coastal communities across the planet have been impacted: The Indian Ocean Tsunami caused severe damage in 2004 (Check Citation2005; Danielsen et al. Citation2005), Hurricane Katrina devastated the Gulf of Mexico coast of the United States in 2005 (Costanza et al. Citation2006; Day et al. Citation2007), and Typhoon Haiyan caused significant destruction in the Philippines and Southeast Asia in 2013. While these events and the possibility of more frequent, powerful tropical cyclones due to climate change remain fresh in the public mind, the establishment of effective and sustainable coastal protection is a pressing concern (Webster Citation2005; Knutson et al. Citation2010). The conservation, restoration, and creation of mangrove forests may be one solution as many of the areas vulnerable to tsunamis and cyclones are also home to mangrove forests (). Moreover, an estimated 64% of the world’s mangrove forests are within 25 km of large urban centers, and are threatened by future urban development (MEA Citation2005).
Figure 1. Global mangrove locations with typical cyclone paths and areas of high tsunami-causing earthquake (seaquake) frequency (Giri et al. Citation2011; NOAA Citation2012, Citation2013).
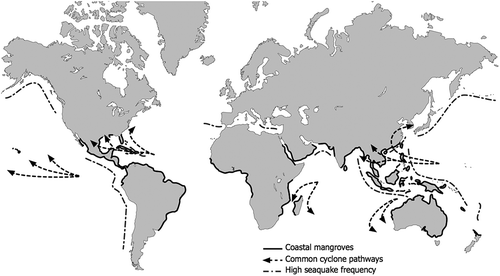
Mangroves are a group of halophytic tree and shrub species that are commonly found in the coastal areas of regions with tropical and subtropical climates (). There are approximately 70 different species of mangroves that span 19 families and 28 genera with a range of different morphologies and physiologies (Duke et al. Citation1998). Due to low tolerance of cold temperatures, their global distribution is primarily within the 30° north and south latitudes (Alongi Citation2002). They are characterized by adaptations to the salinity, anoxia, and tidal hydrology found in coastal ecosystems (Mitsch et al. Citation2009; Mitsch & Gosselink Citation2015). Some species such as Avicennia germinans (found on American and African coasts) and Heritiera fomes (dominant in the Sundarbans of India and Bangladesh) have pneumatophores; aboveground extensions of root branches that aid in the exchange of atmospheric gas (Spalding et al. Citation2010). Other species such as Rhizophora mangle (found on American and African Coasts) and Bruguiera gymnorhiza (found on the coasts of the Indian and Pacific Oceans) grow extensive prop root complexes for enhanced stability (Spalding et al. Citation2010). Both of these adaptations also may improve the capacity of mangroves to provide coastal protection through wave attenuation and soil stabilization (Alongi Citation2008; Ostling et al. Citation2009; Gedan et al. Citation2011). Mangroves also provide many other valuable ecosystem services such as raw materials, maintenance of fisheries, recreation, and carbon sequestration (Barbier et al. Citation2011; Mitsch & Gosselink Citation2015).
The extent of protection which the planting, conservation, and/or restoration of mangrove forests can provide from tsunamis and cyclones is still uncertain (Danielsen et al. Citation2005; Kerr & Baird Citation2007; Baird et al. Citation2009; Das & Vincent Citation2009; Feagin et al. Citation2010). An accurate assessment of how well mangroves can protect coasts from destructive tsunami waves and storm surges is required for proper coastal protection management decisions as well as for the correct economic valuation of the ecosystem services provided by mangroves. This knowledge may provide a case for conservation and restoration of coastal ecosystems that the public and policymakers can utilize (Costanza & Farley Citation2007; Barbier et al. Citation2011).
There have been review articles written on similar topics and focusing on countries such as India and Indonesia (Alongi Citation2008; Cochard et al. Citation2008; Tanaka Citation2009; Feagin et al. Citation2010; Gedan et al. Citation2011). Our review aims to complement these reviews with case studies of mangrove forest management from additional countries and the inclusion of more recently published studies. We focus on common conclusions from research on mangrove coastal protection from tsunamis and cyclones, two types of natural disasters with different causes and physical features but similar coastal impacts. Observational studies, numerical model studies, and physical model studies are all discussed so that results from these different approaches can be compared and contrasted. We also address the economic valuation of mangroves as this is an important consideration in planning and policy. Ultimately, this review synthesizes the results of current research on the capability of mangrove forests to protect coastal areas from the damage caused by tsunamis and cyclones and suggests future directions of research.
2. History of mangrove management
Mangrove ecosystems are threatened across the globe, with an estimated 35% of the world’s mangroves lost due to human activities between 1980 and 2000 (Valiela et al. Citation2001). In many countries, they are destroyed for aquacultural practices such as shrimp farming, whereas in others, they are adversely impacted by commercial and residential development on coveted coastal land (Duke et al. Citation2007). Increasing sea levels due to climate change has also brought the threat of a ‘coastal squeeze’ on mangroves due to rising water on one side and human development on the other (Gilman et al. Citation2007; Mitsch & Gosselink Citation2015). Additionally, the same coastal natural disasters that affect humans are also a primary cause of disturbance to mangroves (Cahoon et al. Citation2003; Davis et al. Citation2004; Porwal et al. Citation2012). However, mangroves have been shown to be resilient to disturbance, which has prompted much inquiry into their future management and probable response to climate change (Alongi Citation2008). Case studies of mangrove disturbances and management in three countries, Bangladesh, the Philippines, and the United States, with extensive histories of coastal natural disasters are provided below.
2.1 Bangladesh
Management of mangrove forests for the benefit of society has been practiced for decades in Bangladesh. In 1966, the Bangladesh Forest Department initiated a program of planting mangroves along the country’s coastline to provide coastal stabilization and protection (Saenger & Siddiqi Citation1993). This initiative was in response to a series of severe cyclones that hit the country in the early 1960s. The planting of mangroves in these coastal areas was found to reduce erosion, causing the land to stabilize and expand enough to support agriculture (Saenger & Siddiqi Citation1993). In the late 1970s, however, the shrimp farming industry grew rapidly in Bangladesh, and by the late 1990s, all 7500 ha of the Chakaria Sundarbans forest, the oldest mangrove forest in the Indian subcontinent, had been cleared primarily for shrimp farming (Hossain et al. Citation2001). As population pressure increases, The Bangladesh Forest Department and the forest-dependent communities continue to struggle over property rights in the rest of the Sundarbans (Roy et al. Citation2012). However, the restoration and conservation of the country’s mangroves have become issues of growing concern as evidenced by the program of action put forth by the Bangladesh Department of Environment. This program outlines strategies of protecting mangroves for the livelihood they provide and the coastal protection they may offer (DOE Citation2006). The drastic changes in valuation of the country’s mangrove forests over these few decades demonstrate how the public’s and government’s perception of these ecosystems depend largely on the socioeconomic situation.
2.2 Philippines
The Philippines have also had a long history of mangrove management. On Banacon Island, the mangrove species Rhizophora stylosa, was planted and harvested extensively for its timber beginning in the 1940s (Walters Citation2003). A national political movement to protect mangroves resulted in legislation being passed in 1979 that established protected wilderness areas in critical mangrove forests where harvesting was made illegal. Although they could no longer harvest them, residents still continued to plant new mangroves, and the government eventually allowed harvesting to resume because Banacon had become a national display of sustainable, community-based mangrove management (Walters Citation2003). Elsewhere in the Philippines, such as in Bais Bay, mangroves were cleared throughout most of the twentieth century for fish ponds, firewood, and construction wood (Samson & Rollon Citation2008). More recently, mangroves have been replanted in some areas to protect fish ponds from wave damage or for restoration purposes (Walters Citation2003; Samson & Rollon Citation2008). Since the devastating Typhoon Haiyan hit the Philippines in 2013, the Philippine government has started a ‘comprehensive program of environmental protection’ including establishment of ‘no-build zones’ in coastal areas and the restoration of mangroves (Straits Times Citation2013). This program demonstrates the Philippine Government’s view that mangroves may provide protection against powerful cyclones like Typhoon Haiyan occurring in the future.
2.3 Southern USA
In the United States, it has been estimated that 28% of the mangrove forests present in 1980 were lost by 2001 (FAO Citation2007). The majority of mangrove forests in the United States exist in the state of Florida with an estimated 190,000 ha (FDEP Citation2012). In Florida, mangroves have been threatened chiefly by commercial and residential development (FDEP Citation2012). In 1975, the US Army Corps of Engineers gained the ability to regulate the dredging and filling of wetlands, including mangroves, under Section 404 of the Clean Water Act (Mitsch & Gosselink Citation2015). More recently, the Mangrove Trimming and Preservation Act, passed in Florida in 1996, protects mangroves from outright clearing, but allows to permit for the trimming of mangroves near waterfront property for aesthetic purposes (FDEP Citation1996). Furthermore, unpermitted impacts on mangroves require compensatory on-site restoration or off-site mitigation to be performed (FDEP Citation1996). Mangrove restoration was also addressed at the federal government level in 2000 with the passing of the Estuary and Clean Water Act, which provided funds to restore estuarine habitat including mangrove forests (U.S. Congress Citation2000). While mangroves are now protected in Florida, most of the larger cities along the Gulf of Mexico and the southern Atlantic coast have already developed to the coastline, leaving minimal space for mangroves to be planted. Biscayne Bay in Miami, Florida, is one of the few cases in which a mangrove forest has been preserved on a section of the coastal side of a large city (Xu et al. Citation2010). Typically though, only smaller or still-developing coastal urban areas will likely have mangrove planting and/or conservation as a possible option for coastal zone protection.
From the case studies in these three countries, it can be seen that coastal communities and governments have invested in maintaining mangroves whether for the potential benefits of silviculture, habitat provision, carbon sequestration, or coastal protection (Barbier et al. Citation2011). This demonstrates the need for accurate assessments of how much coastal protection mangroves can provide and the potential economic costs and benefits of this protection. With this information, decision-makers can better understand the advantages and disadvantages of this coastal protection option.
3. Characteristics of tsunamis and cyclones
A distinction between the different damaging components of tsunamis and cyclones is integral to the evaluation of a mangrove forest’s capacity to mitigate either natural disaster. Tsunamis are a series of large waves typically caused by underwater earthquakes and volcanoes that occur along fault lines () and displace large amounts of water. Cyclones, which may also be called hurricanes or typhoons depending on their geographical location, are low pressure, circular storms that can cause coastal damage through storm surges, wind waves, swell waves, and strong winds. Tsunami waves and cyclone storm surges vary in velocity, height, duration, and recurrence, all essential factors in determining a mangrove forest’s ability to attenuate them (Kerr et al. Citation2006; Mazda et al. Citation2006).
Tsunami waves begin offshore as low-amplitude waves with large wavelengths, then increase in amplitude and decrease in wavelength as they approach shore due to an effect known as wave shoaling (Komar Citation1998). The period of a tsunami wave as it hits the shore is typically in the range of 10–60 min, meaning the coastal inundation will last at least that long (Mirchina & Pelinovsky Citation1981). The highest measured point reached on land by water after a tsunami hits shore is known as run-up. When analyzing maximum run-up heights of catastrophic tsunamis in the 1990s, Choi et al. (Citation2002) found a range of 2.8–18.4 m above mean sea level. Recurrence patterns are also an important consideration as large tsunamis can consist of several waves. A series of smaller waves may prove more destructive than one large wave because the first can clear vegetation and allow subsequent waves to reach further inland (Kerr et al. Citation2006). For reference, the Indian Ocean tsunami of 2004 is considered one of the most destructive natural disasters in recorded history and had three main waves in most areas, with periods of 40–50 min, with run-ups up to 40 m (Choi et al. Citation2006; Rabinovich & Thomson Citation2007).
Cyclones, on the other hand, may create the rise in coastal water level known as storm surges, which can result in loss of life and damage to property. These surges are created by a combination of a storm’s winds and local low pressures increasing water levels above predicted tidal levels (McIvor et al. Citation2012). These surges can last for several hours and commonly reach heights ranging from 1 to 3 m above mean sea level for powerful cyclones although in extreme cases, they have been observed to reach up to 9 m (NCDC Citation2005; Storch & Woth Citation2008; Smith et al. Citation2009; Wang et al. Citation2012). Thus, in comparison to tsunami waves, storm surges typically have longer inundation periods but a lower inundation depth. The winds of cyclones can also be very destructive with 1-min sustained wind speeds of 33 m/s for Category 1 hurricanes and >70 m/s for Category 5 hurricanes (NHC Citation2013). For reference, Hurricane Katrina, a Category 5 hurricane, had storm surges of 6–9 m with sustained winds of 76 m/s (NCDC Citation2005).
4. Mechanisms of protection
Mangroves both directly and indirectly attenuate waves and surges. Directly, they can provide additional drag against wave energy through their trunks, leaves, root systems, and pneumatophores () (Mazda et al. Citation2006). It should be noted that tsunamis and surges behave differently than the tidal and wind waves that Mazda et al. (Citation2006) focused on, and further study is needed into how tsunamis and surges behave as they pass through mangroves. For instance, the long period of tsunami waves may cause any mitigation provided by mangroves to be minor, as the wave will continue to penetrate through the forest long after the mangroves have already been potentially uprooted or damaged by the tsunami’s hydrodynamic forces (Yanagisawa et al. Citation2009). Also, as the water height of severe tsunamis and surges increases, the ability of mangroves to provide significant protection against them is likely reduced (Das & Vincent Citation2009; Yanagisawa et al. Citation2010). Factors within the forest itself that likely determine the amount of drag include the specific characteristics of the species (e.g., presence of pneumatophores or complex prop root systems), the density of the forest, and the diameter of the tree stems (Ewel et al. Citation1998; Alongi Citation2008). The spatial dimensions of the forest, in particular how far it reaches inland from the shore, are considered some of the most important determinants of protective capabilities with regards to tsunamis and cyclones (Olwig et al. Citation2007; Das & Vincent Citation2009). Mangroves may also be able to reduce wind-related damage from large cyclone storms by directly attenuating the energy of wind that passes through their dense canopies, but further research is needed on this potential capability (Das & Crépin Citation2013).
Figure 2. Direct and indirect mechanisms of coastal protection from tsunamis and hurricanes (adapted from Massel et al. Citation1999; Cochard et al. Citation2008; Gedan et al. Citation2011; Gelfenbaum et al. Citation2011; Das & Crépin Citation2013).
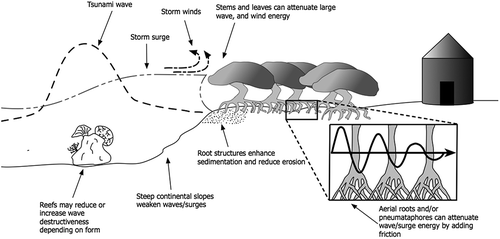
Indirectly, mangroves can promote the stabilization and establishment of coastal soil with their complex root systems (Furukawa et al. Citation1997; Alongi Citation2008). Mangrove roots, like those of many coastal species, can potentially counteract coastal erosion by increasing sediment accretion within their complex structures through friction and reduced turbulence (Kathiresan Citation2003; Thampanya et al. Citation2006). Over time, mangroves may also add significant amounts of organic matter in the form of both roots and leaf litter to the littoral zone, raising the soil surface elevation and reducing erosion due to the stabilizing attributes of the organic matter (McKee & Vervaeke Citation2009). By increasing accretion while reducing erosion, mangroves are able to expand coasts and have been used successfully for this purpose as was seen in Bangladesh in the 1960s (Saenger & Siddiqi Citation1993). Ultimately, this stabilization and expansion of the coast may, in turn, reduce both storm surges and tsunami wave impacts further inland (Alongi Citation2008; Gedan et al. Citation2011).
It is important to be aware of other coastal characteristics that may contribute to wave mitigation that are not implicit to mangroves and may provide confounding effects in studies. For instance, flooding and damage from tsunami waves may be reduced if the continental slope is steeper (Chatenoux & Peduzzi Citation2007; Kerr et al. Citation2009). Also, the presence of coral reefs offshore may either reduce or increase wave destructiveness depending on the reef characteristics and coastal morphology (Gelfenbaum et al. Citation2011). Additionally, when attempting to determine the contribution of mangroves to damage mitigation, the distance inland and the elevation above sea level of an impacted area are important variables and thus should be controlled for in multivariate statistical models (Kerr et al. Citation2006). If these variables are not controlled for in statistical analyses and models, then it becomes difficult to isolate the protection provided solely by the presence of mangroves.
The damage sustained from storms and tsunamis by the mangrove forests themselves is another necessary consideration when attempting to assess their feasibility as part of a coastal protection plan. For instance, tsunamis with multiple large waves may have more impact inland as the initial waves will damage the mangroves by reducing their protective capabilities for subsequent waves (Kerr et al. Citation2006). Mangroves can be destroyed by natural disasters, and if they cannot be reestablished (either naturally or by planting) before another natural disaster occurs, they might not be an appealing option for coastal protection. After examining the extent of mangrove destruction and subsequent recovery in Phang Nga, Thailand after the 2004 Indian Ocean tsunami, Kamthonkiat et al. (Citation2011) found that the tsunami reduced mangrove area by ~5% (1054 ha). Mangrove area continued to decline in 2006 as indirect effects of the tsunami, such as coastal elevation changes and soil degradation, took their toll. By 2010, however, the mangrove area had begun to increase again likely due to reforestation programs (Kamthonkiat et al. Citation2011). Smith et al. (Citation2009) performed a similar study on the impacts of the 2005 Hurricane Wilma on Florida mangroves and estimated that 1250 ha of mangroves were initially lost in this storm. They also reported that frequent hurricanes hitting the area have drastically altered the mangrove ecosystems by even changing some of them to mudflats. Numerical modeling studies can also aid in the assessment of mangroves to withstand damage. For example, Yanagisawa et al. (Citation2010) used data of mangrove damage from the 2004 Indian Ocean tsunami and a numerical model to determine a ‘fragility function’ that predicted mangrove destruction by tsunamis. Insights into the durability of mangroves and their ability to be reestablished can provide decision makers with useful information about the efficacy and potential costs of maintaining coastal protection programs that involve mangroves.
5. Observational studies on coastal protection by mangroves
Due to the low predictability and destructiveness of natural disasters, it is inherently difficult to empirically study their impacts and to have adequate baseline data for comparisons after they occur. Because of this, researchers typically utilize remote sensing and geographic information system (GIS) applications for gathering data from pre-disaster and post-disaster sites. Examples of data that can be effectively gathered in this manner include topography, land use maps, and damage assessments. There still exists a need to ground truth these data for rigorous analyses, but when combined with appropriate statistical analyses, insight can be gained into the relative importance of mangroves in coastal protection.
5.1 Protection from tsunamis
There have been several studies using these remote sensing and GIS techniques to assess the role of mangrove forests in mitigating damage to villages during the 2004 Indian Ocean tsunami. Danielsen et al. (Citation2005) used satellite imagery to investigate the relative damage done by the tsunami to 12 villages on the southeast coast of India that were behind areas of dense tree vegetation, open tree vegetation, or no trees. They found that villages behind dense or open mangrove forests experienced markedly less damage than those behind no trees. Dahdouh-Guebas and Koedam (Citation2006) commented on the analysis done by Danielsen et al. (Citation2005), noting that it did not consider the potential degradation of the forests, which was not captured in satellite imagery. Furthermore, it did not consider how the amount of damage sustained by a village was influenced by the different construction materials used or by the distance of each village from the coastline (Dahdouh-Guebas & Koedam Citation2006). Danielsen et al. (Citation2006) replied the same issue, acknowledging the shortcomings but holding to the conclusion of the need for a protection strategy focused on maintaining and restoring coastal ecosystems. While the study by Danielsen et al. (Citation2005) was one of the first published studies on the topic of mangroves potentially providing protection from the 2004 Indian Ocean tsunami, its statistical analysis left out several potentially important factors. Nonetheless, the study provided a framework for future studies and initiated a much-needed debate on the topic.
In an extension of the Danielsen et al.’s (Citation2005) study, Olwig et al. (Citation2007) used remote-sensing techniques and ground truthing to create 100 profiles along the southeast coast of India, and for each, compared the length of woody vegetation present to the length of damage behind this vegetation. They concluded that woody vegetation could provide coastal protection, but possibly only for waves with amplitudes up to 10 m. Kerr et al. (Citation2009) commented on the study by Olwig et al. (Citation2007) and concluded that it did not consider other confounding variables that also may cause tsunami damage such as elevation, near-shore bathymetry, and land use. Rasmussen et al. (Citation2009) responded by reaffirming the findings of Olwig et al. (Citation2007) and emphasized that while Kerr et al. (Citation2009) disputed the homogeneity of elevation and near-shore bathymetry between the study sites, they provided no evidence to the contrary. They also stated that only the northern part of the study area was criticized, while the southern part still provided convincing evidence of the protective capabilities of woody vegetation. Rasmussen et al. (Citation2009) did acknowledge that more research is certainly needed and that debate on the topic is very beneficial to the advancement of the science.
The debate over the amount of protection the tsunami provided by mangroves was continued with the critiques and subsequent responses for two more studies based on the 2004 Indian Ocean tsunami (Kathiresan & Rajendran Citation2005, Citation2006; Kerr et al. Citation2006; Iverson & Prasad Citation2007, Citation2008; Baird & Kerr Citation2008). The common critique for both studies was that they did not adequately account for other tsunami mitigating factors such as distance from shore and topography and thus could not draw conclusions on the contribution of mangroves alone. The overall message of these critiques was that while mangroves are valuable ecosystems for their provided goods and services, they may only provide a false sense of security against extreme waves and thus the money invested into their establishment may be better spent on developing ‘early-warning systems, community education, and emergency planning’ (Kerr & Baird Citation2007).
These critiques demonstrate the need for multivariate statistical analyses when assessing the protection provided by mangroves. Chatenoux and Peduzzi (Citation2007) performed such an analysis on the 2004 Indian Ocean tsunami using data from 62 sites in the countries of Indonesia, Thailand, India, Sri Lanka, and Maldives. Included in their datasets were topography, near-shore bathymetry, and distributions of coral reefs, seagrass beds, and mangrove forests. Using regression analysis, they found the following variables to be significantly negatively correlated with the width of flooded land: distance from subduction fault line (tsunami origin), average water depth 10 km from shore, and percent coverage by seagrass beds. On the topic of mangroves providing protection, they made the point that mangroves tend to be located only in the sheltered coastal areas such as protected bays and estuaries. This made it impossible for them to isolate the effect of mangroves from other sheltering factors. This is an important consideration in future observational studies on mangrove protection, and Chatenoux and Peduzzi (Citation2007) suggested comparing areas where mangroves have been removed to areas where they have not may be a solution to this issue.
A more recent study by Laso Bayas et al. (Citation2011) aimed to reassess the contribution of coastal vegetation to the protection of Aceh, Indonesia from the 2004 tsunami, taking into account the common critiques of previous studies on the same topic. They included elevation changes as well as near-shore bathymetry in their multivariate statistical models for assessing the controls of tsunami casualties and structural damage. A factor called ‘vegetation resistance coefficient’ was also included which was equal to the product of the vegetation height, the square of the stem diameter at breast height, and the planting density (Laso Bayas et al. Citation2011). This coefficient was then turned into an area-weighted variable called ‘land cover roughness.’ In their multivariate statistical analyses, they found that the distance inland of a site was the major determinant of casualties and structural damage. Additionally, forests located in front of settlements reduced casualties by an average of 8% but did not reduce structural damage. Interestingly, forests located behind settlements were found to increase casualties and structural damage, possibly due to the entrapment and subsequent backwash of debris. They concluded that shifting the location of settlements further inland might be impractical and instead recommend planting vegetation between the settlement and the coast that can provide livelihood as well as protection. Furthermore, due to their findings of a limited ability of vegetation to mitigate large tsunamis, they ultimately recommend combining planting with early warning systems and other risk mitigating actions (Laso Bayas et al. Citation2011).
5.2 Protection from cyclones
While the ability of mangroves to protect from the extreme waves caused by severe tsunamis may still be debatable, their ability to mitigate the relatively more common and less extreme storm surges caused by cyclones is also of interest. Das and Vincent (Citation2009) looked at 409 villages on the east coast of India that were hit by a super cyclone in 1999, which killed almost 10,000 people. They concluded that there was a significant negative correlation between the number of deaths in a village and the width of mangrove forest between that village and the coast. However, as noted by Cochard (Citation2013), the study did not find a similarly significant negative correlation between house damage and mangrove forest width. The methods and results of the study by Das and Vincent (Citation2009) were critiqued by Baird et al. (Citation2009), who stated that elevation and distance inland were much more likely important factors in village mortality rates, and that the cost of mangrove revegetation was high compared with that of an early warning system. Vincent and Das (Citation2009) made a response to this critique, clarifying that topography and distance inland were accounted for in their methods and reaffirming the results of the study. They also made the point that in the state of Orissa, people were warned to evacuate, but decided to stay due to various reasons and that in this case, the presence of mangroves helped save their lives (Vincent & Das Citation2009). While the study by Das and Vincent (Citation2009) did indeed account for topography in their regression analysis, only a dummy variable was used to identify three different types of topography, future studies using more detailed topographical data such as was seen in the study by Chatenoux and Peduzzi (Citation2007) should provide more robust conclusions.
More direct empirical observations of the interaction between mangroves and cyclone storm surges have been made in the Southeastern USA, where hurricanes pose a considerable threat to many coastal cities. In Florida, Krauss et al. (Citation2009) recorded storm surge reductions through mangrove forests during Hurricane Charley in 2004 and Hurricane Wilma in 2005. During surges with maximum water levels ranging from 79 to 104 cm above ground level, they found surge reductions of 4.2 and 9.4 cm/km in a riverine mangrove and a mangrove interior marsh, respectively. However, they could not conclude what the relative contribution of mangrove trees were on the attenuation as it may have also been controlled by other factors such as elevation changes (Krauss et al. Citation2009).
Hurricane Wilma was also part of a unique, unplanned experiment by Granek and Ruttenberg (Citation2007) in which they were able to directly assess the protective ability of mangroves due to two tropical storms hitting an ongoing experimental study site in Belize. They analyzed how much experimental equipment was lost from sites that were either cleared of mangroves or still had intact forests. Cleared sites lost significantly more of all types of equipment than those sites that still had intact mangrove forests. Looking forward, valuable field observations like those in the studies by Krauss et al. (Citation2009) and Granek and Ruttenberg (Citation2007) should be compiled and combined with statistical and numerical models to more effectively assess differences in damage between sites with and without mangrove protection. In order to control for variables such as coastal topography and surge characteristics, these studies would ideally include a variety of sites and storm events so that the role mangroves play in coastal protection may be better distinguished.
6. Numerical and physical models of mangrove coastal protection
Another approach to dealing with the low predictability of tsunamis and cyclones is to use techniques such as numerical models and laboratory-scale physical models. Numerical models typically consist of a set of differential equations that can be run in a computer to simulate phenomena such as hydrodynamics over time. On the other hand, laboratory-scale physical models use real-life, scaled-down systems such as flumes to simulate natural phenomena. Both these types of models are beneficial in their ability to more effectively separate the effects of vegetation on wave and surge attenuation from correlated variables than statistical analysis alone can (Zhang et al. Citation2012).
6.1 Numerical models of protection from tsunamis
A crucial component of many numerical models of coastal protection is the Manning’s roughness coefficient (n) used for different types of land cover (Xu et al. Citation2010; Yanagisawa et al. Citation2010; Kaiser et al. Citation2011; Zhang et al. Citation2012; Liu et al. Citation2013). This coefficient, which represents the amount of friction provided for reducing the height and velocity of surges and waves, can be used to analyze the effects of mangroves on coastal protection (Mattocks & Forbes Citation2008). The value of n can vary with factors such as mangrove species, condition (e.g. age, health, damaged or not damaged), stem diameter, and forest density (Yanagisawa et al. Citation2010; Kaiser et al. Citation2011). A more comprehensive understanding of the value of this coefficient for different scenarios would reduce uncertainty in numerical modeling efforts and should be a goal for future research.
Many models were prompted by the 2004 Indian Ocean Tsunami, such as the numerical hydrodynamic model described in the study by Yanagisawa et al. (Citation2010), which was validated using tide gage data and field measurements of the depth and extent of inundation in Banda Aceh, Indonesia. They calculated a ‘fragility function’ to predict mangrove loss from tsunamis, but they also modeled the reduction of tsunami hydrodynamic force and inundation depth by mangroves. The fragility function allowed the model to recalculate n values at each time step depending on the flow condition and tree destruction. The model compared scenarios with and without mangroves by using identical, simplified topographies of the Banda Aceh coast. Mangrove forests of 10, 20, and 30 years of age were separately modeled, with trunk diameter increasing and tree density decreasing with increased age. Their numerical model results showed that 10-year-old mangroves performed best for tsunami waves <3 m, reducing maximum inundation depth by 38% and hydrodynamic force by 70% behind the forest. Furthermore, 20- and 30-year-old mangroves performed better for greater tsunami wave heights, indicating the possible importance of conserving mangroves over long periods of time to enhance the protection they provide. Additionally, they found that any tsunami waves in the range of 6–9 m were largely unmitigated by the presence of mangroves of any age due to high probability of a significant amount of the trees being destroyed. While their results may provide some preliminary evidence for the protective role of mangroves for smaller tsunami waves, the model should ideally be run for more scenarios of different locations and tsunami characteristics to allow for more general conclusions to be made (Yanagisawa et al. Citation2010).
Kaiser et al. (Citation2011) used an n value of 0.0951 for mangroves in their use of the numerical models ComMIT and Mike 21 FM to simulate tsunami inundation in Thailand. Their model was validated using recorded water-level data and water marks from tsunami run-up observed in the field and was found to be sufficiently accurate. In their simulations, mangroves were found to have little impact on the area of inundation (352 ha without mangroves compared with 348 ha with mangroves). However, inundation depth was found to vary with the presence of mangroves: in simulations with mangroves, the depth in front of the forest increased by up to 0.4 m, while the depth behind the forest was up to 0.65 m lower than that from simulations run without the presence of mangroves. This study is one of the few numerical models of land cover influencing tsunami inundation that had high-quality field data available for validation, but the authors still stressed the need for better spatial information on water depths and velocities to improve pre-tsunami and post-tsunami damage analyses (Kaiser et al. Citation2011).
6.2 Numerical models of protection from cyclones
Protection from cyclones by coastal vegetation has also been simulated in numerical models using methods similar to those used for tsunamis. Xu et al. (Citation2010) performed extensive hydrodynamic simulations of storm surges from Hurricane Andrew in Biscayne Bay, Florida. They found that when the roughness of the mangrove forest area was not accounted for in their model, their simulations significantly overestimated the observed inundation area. To correct this, they ran three separate simulations using varying n values of 0.05, 0.1, and 0.15 for the 1–4 km wide mangrove forest areas located on the coast of the bay. These additional simulations found that an n value of 0.15 for mangroves provided the best match to observed inundation areas. While it is not certain that neglecting the effects of mangroves was the reason for their initial simulations to be inaccurate, this study does demonstrate how hydrodynamic models may aid in assessing the capability of mangroves to mitigate storm surges. Further field observations including spatial water-level data during the storms would allow for better estimation of n values and would better verify their conclusions (Xu et al. Citation2010).
Zhang et al. (Citation2012) utilized data from Hurricane Wilma in 2005 and the Coastal and Estuarine Storm Tide numerical hydrodynamic model to simulate storm surges on the coast of Florida from hurricanes of varying strength. The simulated surge heights of their numerical model were calibrated with surge heights recorded from Hurricane Wilma, a Category 3 hurricane with a maximum storm surge of 5 m. Their simulations used an n value of 0.14 for mangroves and found that ‘widths of mangrove zones affect surge attenuation in a nonlinear fashion with large reduction at the initial increment of widths and small reduction at subsequent increments’ (Zhang et al. Citation2012, p. 22). They concluded that a 7–8 km wide mangrove forest could reduce a Category 3 hurricane’s surges by ~80%. They also noted that the velocity of surges is an important factor in their potential to be reduced by mangroves, with faster surges being more restricted in the mangroves and slower surges penetrating deeper inland. In an extension of this study, Liu et al. (Citation2013) analyzed the same model and concluded that storm intensity, size, and direction were additional factors that may determine the ability of mangroves to reduce storm surge and flooding. These two studies exhibit how a plethora of water-level data from a cyclone’s storm surges can be used to develop a calibrated numerical model that can estimate the influence of land cover on surge hydrodynamics. Further modeling efforts like these can potentially be important tools in risk assessment and determining the protective capabilities of mangroves.
6.3 Physical models of coastal protection
Laboratory-scale physical models provide another method of assessing the protective capabilities of mangrove forests. These models can be operated in different scenarios to calibrate and validate the drag-related coefficients of mangrove trees. Harada et al. (Citation2002) placed various permeable structures in a wave-generating open channel to simulate reduction of tsunamis by different land cover types. The structure designed to simulate mangroves had changing porosities in the vertical direction, corresponding to the roots, trunks, and leaves of a typical mangrove tree. Their experiments found all the simulated land cover types including mangroves, reduced water elevation, current, and pressure behind them (Harada et al. Citation2002). Husrin et al. (Citation2012) and Strusińska-Correia et al. (Citation2013) used extensively parameterized mangrove structures in a flume to simulate mangrove effects on a variety of wave types. Husrin et al. (Citation2012) found that the mangrove models were better at attenuating breaking waves than nonbreaking waves and that the widest forest model performed the best. Strusińska-Correia et al. (Citation2013) had similar methods but found somewhat contrasting results in which topography played a much more significant role in tsunami wave attenuation than the simulated mangroves. However, they mentioned that their research with this system is still ongoing and that future results will provide more insights (Strusińska-Correia et al. Citation2013).
The findings of these numerical models and laboratory-scale physical models generally support that mangrove forests have the potential to reduce water height and velocity from both tsunamis and storm surges. In many cases, they can show this more effectively than observational studies due to their ability to better isolate the effects of mangroves through sensitivity analyses or direct experimental controls. However, these are still models of complex real-world phenomena, and many assumptions and generalizations must be made both in their creation and interpretation of outputs. For example, the numerical models can only draw strong conclusions for the locations and coastal events they simulated and should be validated across more scenarios to draw more robust general conclusions about the extent of coastal protection provided by mangroves. With regards to laboratory-scale physical models, it must be assumed that the hydrodynamic flumes are accurately simulating tsunami or surge conditions, which again is difficult due to the limited amount of data available on the behavior of these phenomena as they occur.
7. Valuation of mangrove ecosystems
An estimated economic value of mangrove forests is a vital piece of information with regards to decision-making within coastal protection plans. Balmford et al. (Citation2002) found only five studies worldwide where economic estimates were available for both intact ecosystems and the same landscape, but heavily managed. Two of those five case studies were of wetlands with one of those two from a mangrove swamp. Balmford et al.’s (Citation2002) economic analysis of a mangrove swamp in Thailand, based on the work of Sathirathai (Citation1998), showed that conversion of a swamp to aquaculture made economic sense in the short term, but in the long term, the total economic value of an intact mangrove swamp was US$ 60,400 ha−1, about 3.6 times that of the value of converting the swamp to shrimp aquaculture. The values for the natural mangrove swamp used in this study included timber, charcoal, non-timber forest products, offshore fisheries, and coastal protection. The value of coastal protection was calculated using the construction cost to replace lost mangroves with breakwater structures and was found in different cases to range from 73 to 93% of the total estimated economic value of the mangrove forest (Sathirathai Citation1998).
In a related study, Barbier (Citation2007) estimated the value of mangroves in Thailand by summing the value of the forest products they provide, the value of their enhancement to fisheries, and the value of their coastal protection. These totaled to an estimated value in the range of US$ 10,158–12,392 ha−1, of which US$ 8,966–10,821 ha−1 (~87%) was attributed to storm protection service. The value of the protection service was calculated using an alternative to the replacement cost approach known as an ‘expected damage function’ which estimates how changes in an asset will influence the chance of a damaging event occurring. This function was created using 1979–1996 data from Thailand of average annual mangrove loss and real economic damages incurred by coastal disasters (Barbier Citation2007).
Costanza et al. (Citation2008) used a different method to assess the value of wetlands for hurricane protection. They created a regression using data from 34 hurricanes in the United States with damage per unit of gross domestic product as the dependent variable and the natural logs of wind speed and wetland area (herbaceous wetlands only, not including forested wetlands such as mangroves) as independent variables. The independent variables were able to explain 60% of the variation in damage. They were then able to derive estimates of the annual value of wetlands for storm protection resulting in a mean value of US$ 8236 ha−1 year−1. The authors were surprised to find that forested wetlands did not improve the statistical model and attributed this to coastal mangroves not being distinguished from inland riparian forested wetlands which likely had little protection value and offset the protection provided by mangroves (Costanza et al. Citation2008). They noted that in future studies, better data should be obtained to allow for the differentiation between mangroves and riparian forested wetlands. Costanza et al. (Citation2014) revisited the calculations of the highly cited Costanza et al. (Citation1997) paper, which calculated the economic value of global ecosystems, using some revised unit values determined by de Groot et al. (Citation2012) and others from a new United Nations-sponsored post-Millennium Ecosystem Assessment. Inland swamps/floodplain unit values stayed approximately the same, whereas the tidal marsh/mangroves unit value increased 14-fold (Mitsch & Gosselink Citation2015), ‘largely due to new studies of the storm protection, erosion protection, and waste treatment values’ of these tidal wetlands (Costanza et al. Citation2014). These valuations provide important information for decision-makers when weighing the costs and benefits of mangrove conservation and restoration for the purpose of coastal protection.
8. Conclusions
Governments around the world have begun to invest in mangrove restoration and conservation for the ecosystem services they can provide. Mangrove establishment, conservation, and restoration can be an expensive and difficult venture with many opportunity costs; therefore, it is important to assess the potential results and benefits to both the environment and nearby communities. Empirical studies of storm surge and tsunami protection by mangroves have historically been difficult, but the development of remote-sensing technologies and GIS is making it easier to assess damage after natural disasters. Computer simulations using numerical models have also made it much simpler to test theories of protection mechanisms, and to predict risks, but many assumptions must be made in such studies. There is still much to be learned concerning just how much protection mangroves can provide and what factors control this protection.
Observational studies have not provided conclusive results on the extent of the protection that mangroves can provide for coastal areas from tsunamis and cyclones. Further studies utilizing multivariate statistical analyses based on robust datasets will provide stronger conclusions that decision-makers can utilize. These studies should aim to compare the coastal protection capabilities across a variety of topographies, land covers, and wave types with the goal of reducing confounding variables. Also, direct measures of attenuation within mangrove forests such as wave height and velocity reduction should be considered in addition to post-event damage analyses to further strengthen conclusions and enable comparisons with numerical hydrodynamic models.
The results of recent numerical models and laboratory experiments tend to support the potential of mangroves to reduce the water height and velocity of both cyclone storm surges and smaller tsunamis. However, there have been only a few numerical models published thus far that have been validated using high-quality datasets. Running these numerical models using data from different locations and various tsunamis and cyclones, or creating new models that are more generally applicable, will continue to provide more insight into the potential protection provided by mangroves. Additionally, a better method for estimating the Manning’s roughness coefficient for a mangrove forest could greatly aid in the accuracy of these models when used for risk assessment or to quantify the protective capabilities of mangroves.
Studies on the economic value of mangroves have estimated their potential to provide coastal protection to be a significant portion of their total estimated value. Mangrove conservation, restoration, and establishment can come with significant opportunity costs, but this is likely outweighed by the valuable ecosystem services they provide. Future studies that can provide detailed economic analyses of the costs and benefits of a coastal protection plan that utilizes mangroves will prove valuable as more countries begin considering this option. Regardless of the extent of the coastal protection provided by mangroves, comprehensive coastal protection programs should not rely on just mangroves for protection, but should also invest in early warning systems and education to better protect human life. This combination of strategies would allow for livelihoods dependent on mangroves to be maintained while also potentially providing improved protection from coastal natural disasters.
Acknowledgements
This manuscript was developed at Florida Gulf Coast University’s Everglades Wetland Research Park (EWRP) in Naples, Florida, USA. The School of Environment and Natural Resources and the Ohio Agricultural Research and Development Center (OARDC) at The Ohio State University providing salary support for the first author during this study. We appreciate the detailed reviews by two anonymous reviewers; the article is much stronger in our view because of these reviews.
Additional information
Funding
References
- Alongi DM. 2002. Present state and future of the world’s mangrove forests. Environ Conserv. 29:331–349.
- Alongi DM. 2008. Mangrove forests: resilience, protection from tsunamis, and responses to global climate change. Estuar Coast Shelf Sci. 76:1–13.
- Baird AH, Bhalla RS, Kerr AM, Pelkey NW, Srinivas V. 2009. Do mangroves provide an effective barrier to storm surges? Proc Natl Acad Sci U S A. 106:E111.
- Baird AH, Kerr AM. 2008. Landscape analysis and tsunami damage in Aceh: comment on Iverson and Prasad (2007). Landsc Ecol. 23:3–5.
- Balmford A, Bruner A, Cooper P, Costanza R, Farber S, Green RE, Jenkins M, Jefferiss P, Jessamy V, Madden J, et al. 2002. Ecology - Economic reasons for conserving wild nature. Science. 297:950–953.
- Barbier EB. 2007. Valuing ecosystem services as productive inputs. Econ Policy. 49:177–229.
- Barbier EB, Hacker SD, Kennedy C, Koch EW, Stier AC, Silliman BR. 2011. The value of estuarine and coastal ecosystem services. Ecol Monogr. 81:169–193.
- Cahoon DR, Hensel P, Rybczyk J, McKee KL, Proffitt CE, Perez BC. 2003. Mass tree mortality leads to mangrove peat collapse at Bay Islands, Honduras after Hurricane Mitch. J Ecol. 91:1093–1105.
- [CIESIN] Center for International Earth Science Information Network. 2007. National aggregates of geospatial data collection: population, landscape, and climate estimates, Version 2 (PLACE II). Palisades (NY): NASA Socioeconomic Data and Applications Center (SEDAC).
- Chatenoux B, Peduzzi P. 2007. Impacts from the 2004 Indian Ocean tsunami: analysing the potential protecting role of environmental features. Nat Hazards. 40:289–304.
- Check E. 2005. Natural disasters: roots of recovery. Nature. 438:910–911.
- Choi BH, Hong SJ, Pelinovsky E. 2006. Distribution of runup heights of the December 26, 2004 tsunami in the Indian Ocean. Geophys Res Lett. 33:L13601.
- Choi BH, Pelinovsky E, Ryabov I, Hong SJ. 2002. Distribution functions of tsunami wave heights. Nat Hazards. 25:1–21.
- Cochard R. 2013. Natural hazards mitigation services of carbon-rich ecosystems. In: Lal R, Lorenz K, Huttl RF, Schneider BU, von Braun J, editors. Ecosystem services and carbon sequestration in the biosphere. New York (NY): Springer; p. 222–293.
- Cochard R, Ranamukhaarachchi SL, Shivakoti GP, Shipin OV, Edwards PJ, Seeland KT. 2008. The 2004 tsunami in Aceh and Southern Thailand: a review on coastal ecosystems, wave hazards and vulnerability. Perspect Plant Ecol. 10:3–40.
- Costanza R, d’Arge R, Degroot R, Farber S, Grasso M, Hannon B, Limburg K, Naeem S, O’Neill RV, Paruelo J, et al. 1997. The value of the world’s ecosystem services and natural capital. Nature. 387:253–260.
- Costanza R, de Groot R, Sutton P, van der Ploeg S, Anderson J, Kubiszewski I, Farber S, Turner RK. 2014. Changes in the global value of ecosystem services. Global Environ Chang 26: 152–158.
- Costanza R, Farley J. 2007. Ecological economics of coastal disasters: introduction to the special issue. Ecol Econ. 63:249–253.
- Costanza R, Mitsch WJ, Day JW. 2006. A new vision for New Orleans and the Mississippi delta: applying ecological economics and ecological engineering. Front Ecol Environ. 4: 465–472.
- Costanza R, Pérez-Maqueo O, Martinez ML, Sutton P, Anderson SJ, Mulder K. 2008. The value of coastal wetlands for hurricane protection. Ambio. 37:241–248.
- Dahdouh-Guebas F, Koedam N. 2006. Coastal vegetation and the Asian tsunami. Science. 311:37–38.
- Danielsen F, Sorensen MK, Olwig MF, Selvam V, Parish F, Burgess ND, Hiraishi T, Karunagaran VM, Rasmussen MS, Hansen LB, et al. 2005. The Asian tsunami: a protective role for coastal vegetation. Science. 310:643–643.
- Danielsen F, Sørensen MK, Olwig MF, Selvam V, Parish F, Burgess ND, Topp-Jorgensen E, Hiraishi T, Karunagaran VM, Rasmussen MS, Hansen LB, et al. 2006. Response to “Coastal vegetation and the Asian tsunami”. Science. 311:37–38.
- Das S, Crépin A-S. 2013. Mangroves can provide protection against wind damage during storms. Estuar Coast Shelf Sci. 134:98–107.
- Das S, Vincent JR. 2009. Mangroves protected villages and reduced death toll during Indian super cyclone. Proc Natl Acad Sci USA 106:7357–7360.
- Davis SE, Cable JE, Childers DL, Coronado-Molina C, Day JW, Hittle CD, Madden CJ, Reyes E, Rudnick D, Sklar F. 2004. Importance of storm events in controlling ecosystem structure and function in a Florida gulf coast estuary. J Coast Res. 20:1198–1208.
- Day Jr JW, Boesch DF, Clairain EJ, Kemp GP, Laska SB, Mitsch WJ, Orth K, Mashriqui H, Reed DJ, Shabman L, et al. 2007. Restoration of the Mississippi Delta: lessons from Hurricanes Katrina and Rita. Science. 315:1679–1684.
- de Groot R, Brander L, van der Ploeg S, Costanza R, Bernard F, Braat L, Christie M, Crossman N, Ghermandi A, Hein L, et al. 2012. Global estimates of the value of ecosystems and their services in monetary units. Ecosystem Serv 1:50–61.
- [DOE] Bangladesh Department of Environment. 2006. Bangladesh: national programme of action for protection of the coastal and marine environment from land-based activities [Internet]. Ministry of Environment and Forests, Government of the People’s Republic of Bangladesh. [cited 2013 Dec 15]. Available from: www.doe.gov.bd/publication_images/8_npa_draft.pdf
- Duke NC, Ball MC, Ellison JC. 1998. Factors influencing biodiversity and distributional gradients in mangroves. Global Ecol Biogeogr. 7:27–48.
- Duke NC, Meynecke J-O, Dittmann S, Ellison AM, Anger K, Berger U, Cannicci S, Diele K, Ewel KC, Field CD, et al. 2007. A world without mangroves? Science. 317:41–42.
- Ewel KC, Twilley RR, Ong JE. 1998. Different kinds of mangrove forests provide different goods and services. Global Ecol Biogeogr. 7:83–94.
- [FDEP] Florida Department of Environmental Protection. 1996. Mangrove Trimming & Preservation Act [Internet]. [cited 2014 Jan 5]. Available from: http://www.dep.state.fl.us/water/wetlands/mangroves/docs/mtpa96.pdf
- [FDEP] Florida Department of Environmental Protection. 2012. Mangroves “Walking Trees” [Internet]. [cited 2014 Jan 10]. Available from: http://www.dep.state.fl.us/coastal/habitats/mangroves.htm.
- [FAO] Food and Agricultural Organization of the United Nations. 2007. The world’s mangroves 1980–2005. FAO Forestry Paper 153. Rome: FAO.
- Feagin RA, Mukherjee N, Shanker K, Baird AH, Cinner J, Kerr AM, Koedam N, Sridhar A, Arthur R, Jayatissa LP, et al. 2010. Shelter from the storm? Use and misuse of coastal vegetation bioshields for managing natural disasters. Conserv Lett. 3:1–11.
- Furukawa K, Wolanski E, Mueller H. 1997. Currents and sediment transport in mangrove forests. Estuar Coast Shelf Sci. 44:301–310.
- Gedan KB, Kirwan ML, Wolanski E, Barbier EB, Silliman BR. 2011. The present and future role of coastal wetland vegetation in protecting shorelines: answering recent challenges to the paradigm. Clim Change. 106:7–29.
- Gelfenbaum G, Apotsos A, Stevens AW, Jaffe B. 2011. Effects of fringing reefs on tsunami inundation: American Samoa. Earth-Sci Rev. 107:12–22.
- Gilman E, Ellison J, Coleman R. 2007. Assessment of mangrove response to projected relative sea-level rise and recent historical reconstruction of shoreline position. Environ Monit Assess. 124:105–130.
- Giri C, Ochieng E, Tieszen LL, Zhu Z, Singh A, Loveland T, Masek J, Duke N. 2011. Status and distribution of mangrove forests of the world using earth observation satellite data. Global Ecol Biogeogr. 20:154–159.
- Granek EF, Ruttenberg BI. 2007. Protective capacity of mangroves during tropical storms: a case study from ‘Wilma’ and ‘Gamma’ in Belize. Mar Ecol-Prog Ser. 343:101–105.
- Harada K, Imamura F, Hiraishi T. 2002. Experimental study on the effect in reducing tsunami by the coastal permeable structures. In: Chung JS, Koterayama W, Ch K, Li Y, editors. Proceedings of the Twelfth (2002) International Offshore and Polar Engineering Conference, Vol 3. Cupertino (CA): International Society Offshore& Polar Engineers; p. 652–658.
- Hossain MS, Lin CK, Hussain MZ. 2001. Goodbye Chakaria Sundarban: the oldest mangrove forest. Wetland Sci Pract. 18:19–22.
- Husrin S, Strusinska A, Oumeraci H. 2012. Experimental study on tsunami attenuation by mangrove forest. Earth Planets Space. 64:973–989.
- Iverson LR, Prasad AM. 2007. Using landscape analysis to assess and model tsunami damage in Aceh province, Sumatra. Landscape Ecol. 22:323–331.
- Iverson LR, Prasad AM. 2008. Modeling tsunami damage in Aceh: a reply. Landsc Ecol. 23:7–10.
- Kaiser G, Scheele L, Kortenhaus A, Løvholt F, Römer H, Leschka S. 2011. The influence of land cover roughness on the results of high resolution tsunami inundation modeling. Nat Hazards Earth Syst Sci. 11:2521–2540.
- Kamthonkiat D, Rodfai C, Saiwanrungkul A, Koshimura S, Matsuoka M. 2011. Geoinformatics in mangrove monitoring: damage and recovery after the 2004 Indian Ocean tsunami in Phang Nga, Thailand. Nat Hazards Earth Syst Sci. 11:1851–1862.
- Kathiresan K. 2003. How do mangrove forests induce sedimentation? Revista de biologia tropical. 51:355–359.
- Kathiresan K, Rajendran N. 2005. Coastal mangrove forests mitigated tsunami. Estuar Coast Shelf Sci. 65:601–606.
- Kathiresan K, Rajendran N. 2006. Reply to ’Comments of Kerr et al. on “Coastal mangrove forests mitigated tsunami” [Estuar. Coast. Shelf Sci. 65 (2005) 601–606]. Estuar Coast Shelf Sci. 67:542–542.
- Kerr AM, Baird AH. 2007. Natural barriers to natural disasters. Bioscience. 57:102–103.
- Kerr AM, Baird AH, Bhalla RS, Srinivas V. 2009. Reply to “Using remote sensing to assess the protective role of coastal woody vegetation against tsunami waves.” Int J Remote Sens. 30:3817–3820.
- Kerr AM, Baird AH, Campbell SJ. 2006. Comments on “Coastal mangrove forests mitigated tsunami” by K. Kathiresan and N. Rajendran [Estuar. Coast. Shelf Sci. 65 (2005) 601–606]. Estuar Coast Shelf Sci. 67:539–541.
- Knutson TR, McBride JL, Chan J, Emanuel K, Holland G, Landsea C, Held I, Kossin JP, Srivastava AK, Sugi M. 2010. Tropical cyclones and climate change. Nat Geosci. 3:157–163.
- Komar PD. 1998. Beach processes and sedimentation. 2nd ed. Upper Saddle River (NJ): Prentice Hall.
- Krauss KW, Doyle TW, Doyle TJ, Swarzenski CM, From AS, Day RH, Conner WH. 2009. Water level observations in mangrove swamps during two hurricanes in Florida. Wetlands. 29:142–149.
- Laso Bayas JC, Marohn C, Dercon G, Dewi S, Piepho HP, Joshi L, van Noordwijk M, Cadisch G. 2011. Influence of coastal vegetation on the 2004 tsunami wave impact in west Aceh. P Natl Acad Sci USA. 108:18612–18617.
- Liu H, Zhang K, Li Y, Xie L. 2013. Numerical study of the sensitivity of mangroves in reducing storm surge and flooding to hurricane characteristics in southern Florida. Cont Shelf Res. 64:51–65.
- Massel SR, Furukawa K, Brinkman RM. 1999. Surface wave propagation in mangrove forests. Fluid Dyn Res. 24:219–249.
- Mattocks C, Forbes C. 2008. A real-time, event-triggered storm surge forecasting system for the state of North Carolina. Ocean Model. 25:95–119.
- Mazda Y, Magi M, Ikeda Y, Kurokawa T, Asano T. 2006. Wave reduction in a mangrove forest dominated by Sonneratia sp. Wetl Ecol Manag. 14:365–378.
- McIvor AL, Spencer T, Moller I, Spalding M. 2012. Storm surge reduction by mangroves [Internet]. Natural Coastal Protection Series: Report 2. Cambridge Coastal Research Unit Working Paper 41. The Nature Conservancy and Wetlands International. [cited 2013 Dec 10]. Available from: http://coastalresilience.org/sites/default/files/resources/storm-surge-reduction-by-mangroves-report.pdf
- McKee KL, Vervaeke WC. 2009. Impacts of human disturbance on soil erosion potential and habitat stability of mangrove-dominated islands in the Pelican Cays and Twin Cays ranges, Belize. Smithson Contrib Mar Sci. 38:415–427.
- [MEA] Millenium Ecosystem Assessment. 2005. Ecosystems and human well-being: current state and trends. Washington (DC): Island Press.
- Mirchina N, Pelinovsky E. 1981. The Dependence of Tsunami Wave Period on the Source Dimensions. Mar Geod. 5:201–208.
- Mitsch WJ, Gosselink JG. 2015. Wetlands. 5th ed. Hoboken (NJ): Wiley.
- Mitsch WJ, Gosselink JG, Anderson CJ, Zhang L. 2009. Wetland ecosystems. Hoboken (NJ): Wiley.
- [NCDC] National Climactic Data Center. 2005. Hurricane Katrina. National Oceanic and Atmospheric Administration [Internet]. [cited 2013 Nov 13]. Available from: http://www.ncdc.noaa.gov/extremeevents/specialreports/Hurricane-Katrina.pdf
- [NHC] National Hurricane Center. 2013. Saffir-Simpson hurricane wind scale. National Oceanic and Atmospheric Administration [Internet]. [cited 2013 Nov 20]. Available from: http://www.nhc.noaa.gov/aboutsshws.php
- [NOAA] National Oceanic and Atmospheric Administration. 2012. Historical hurricane tracks [Internet]. [cited 2013 Nov 9]. Available from: http://csc.noaa.gov/hurricanes.
- [NOAA] National Oceanic and Atmospheric Administration. 2013. Natural hazards viewer [Internet]. [cited 2013 Nov 9]. Available from: http://maps.ngdc.noaa.gov/viewers/hazards.
- Nicholls RJ, Cazenave A. 2010. Sea-level rise and its impact on coastal zones. Science. 328:1517–1520.
- Olwig MF, Sørensen MK, Rasmussen MS, Danielsen F, Selvam V, Hansen LB, Nyborg L, Vestergaard KB, Parish F, Karunagaran VM. 2007. Using remote sensing to assess the protective role of coastal woody vegetation against tsunami waves. Int J Remote Sens. 28:3153–3169.
- Ostling JL, Butler DR, Dixon RW. 2009. The biogeomorphology of mangroves and their role in natural hazards mitigation. Geogr Compass. 3:1607–1624.
- Porwal MC, Padalia H, Roy PS. 2012. Impact of tsunami on the forest and biodiversity richness in Nicobar Islands (Andaman and Nicobar Islands), India. Biodivers Conserv. 21:1267–1287.
- Rabinovich A, Thomson R. 2007. The 26 December 2004 Sumatra tsunami: analysis of tide gauge data from the world ocean Part 1. Indian Ocean and South Africa. Pure Appl Geophys. 164:261–308.
- Rasmussen MS, Selvam V, Sørensen MK, Danielsen F, Olwig MF, Parish F. 2009. Response to Kerr et al. Int J Remote Sens. 30:3821–3825.
- Roy AKD, Alam K, Gow J. 2012. A review of the role of property rights and forest policies in the management of the Sundarbans Mangrove Forest in Bangladesh. Forest Policy Econ. 15:46–53.
- Saenger P, Siddiqi N. 1993. Land from the sea: the mangrove afforestation program of Bangladesh. Ocean Coast Manage. 20:23–39.
- Samson MS, Rollon RN. 2008. Growth performance of planted mangroves in the Philippines: revisiting forest management strategies. Ambio. 37:234–240.
- Sathirathai S. 1998. Economic valuation of mangroves and the roles of local communities in the conservation of natural resources: case study of Surat Thani, south of Thailand [Internet]. EEPSEA Research Report RR1998061, Economy and Environment Program for Southeast Asia (EEPSEA), Singapore. [cited 2014 May 10]. Available from: http://www.eepsea.net/pub/rr/10536137110ACF9E.pdf
- Smith TJ, Anderson GH, Balentine K, Tiling G, Ward GA, Whelan KRT. 2009. Cumulative impacts of hurricanes on Florida mangrove ecosystems: sediment deposition, storm surges and vegetation. Wetlands. 29:24–34.
- Spalding M, Kainuma M, Collins L. 2010. World atlas of mangroves. London: Earthscan.
- Storch H, Woth K. 2008. Storm surges: perspectives and options. Sustain Sci. 3:33–43.
- Straits Times. 2013. Typhoon Haiyan: Philippines to plant more mangroves [Internet]. [cited 2013 Dec 4]. Available from: http://www.straitstimes.com/breaking-news/se-asia/story/typhoon-haiyan-philippines-plant-more-mangroves-20131124
- Strusińska-Correia A, Husrin S, Oumeraci H. 2013. Tsunami damping by mangrove forest: a laboratory study using parameterized trees. Nat Hazards Earth Syst Sci. 13:483–503.
- Tanaka N. 2009. Vegetation bioshields for tsunami mitigation: review of effectiveness, limitations, construction, and sustainable management. Landsc Ecol Eng. 5:71–79.
- Thampanya U, Vermaat JE, Sinsakul S, Panapitukkul N. 2006. Coastal erosion and mangrove progradation of Southern Thailand. Estuar Coast Shelf Sci. 68:75–85.
- Turner RK, Subak S, Adger WN. 1996. Pressures, trends, and impacts in coastal zones: interactions between socioeconomic and natural systems. Environ Manage. 20:159–173.
- U.S. Congress. 2000. Estuaries and clean water act of 2000. 106th Congress of America. Washington (DC): U.S. Government Printing Office.
- Valiela I, Bowen JL, York JK. 2001. Mangrove forests: one of the world’s threatened major tropical environments. Bioscience. 51:807–815.
- Vincent JR, Das S. 2009. Reply to Baird et al.: mangroves and storm protection: getting the numbers right. P Natl Acad Sci USA. 106:E112–E112.
- Walters BB. 2003. People and mangroves in the Philippines: fifty years of coastal environmental change. Environ Conserv. 30:293–303.
- Wang J, Gao W, Xu S, Yu L. 2012. Evaluation of the combined risk of sea level rise, land subsidence, and storm surges on the coastal areas of Shanghai, China. Clim Chang. 115:537–558.
- Webster PJ. 2005. Changes in tropical cyclone number, duration, and intensity in a warming environment. Science. 309:1844–1846.
- Xu H, Zhang K, Shen J, Li Y. 2010. Storm surge simulation along the U.S. East and Gulf Coasts using a multi-scale numerical model approach. Ocean Dyn. 60:1597–1619.
- Yanagisawa H, Koshimura S, Goto K, Miyagi T, Imamura F, Ruangrassamee A, Tanavud C. 2009. The reduction effects of mangrove forest on a tsunami based on field surveys at Pakarang Cape, Thailand and numerical analysis. Estuar Coast Shelf Sci. 81:27–37.
- Yanagisawa H, Koshimura S, Miyagi T, Imamura F. 2010. Tsunami damage reduction performance of a mangrove forest in Banda Aceh, Indonesia inferred from field data and a numerical model. J Geophys Res-Oceans. 115:C06032.
- Zhang K, Liu H, Li Y, Xu H, Shen J, Rhome J, Smith TJ. 2012. The role of mangroves in attenuating storm surges. Estuar Coast Shelf Sci. 102–103:11–23.