Abstract
Urbanization changes water balance, degrades water quality and disrupts habitats. Wetlands offer storm water volume and flow control, water pollution mitigation, and rich land–water interphase habitats. In the present case study, urban wetlands were designed and implemented to provide multiple functions, including water quality improvement and the establishment of critically endangered clay stream habitat, along a revived urban stream within the Baltic Sea watershed in Southern Finland. The primary water quality concern in the recipient lake is algal bloom controlling and clay particle-carried phosphorus.
Wetlands were monitored for functioning over five calendar years. At a wetland monitored for 5 years, herbaceous vegetation was well self-established in the second year, and reached 102 species, of which 97% were native, in the fifth growing season. Successful breeding of amphibians and water birds occurred right after construction. Continuous water quality monitoring over the fourth year at this wetland, with 0.1% area of its watershed, revealed seasonal and event-based differences: for total phosphorus, an annual 10% average with lower removal rates outside, and up to 71% event reductions during the growing season, while highest load reductions occurred during heavy rain and snowmelt events outside the growing season. The created wetlands provided critical habitat and beneficial functions and thus compensated partly for urbanization.
Introduction
Urbanization and associated imperviousness change water balance, increasing the severity of flooding and draught periods. Run-off from rain and melting snow moves quickly over sealed urban surfaces into impervious conveyance structures designed to quickly deposit storm water in a receiving stream or a larger water body. Climate change is expected to increase rainfall intensity and duration in Southern Finland, as well as intensify spells of heat and drought during warm periods (Jylhä et al. Citation2004; Kovats et al. Citation2014). Elevated flow volumes and energies reaching urban streams during run-off events cause flooding and channel erosion. Conventional responses to these problems include further sealing of natural waterways into culverts or clearing, straightening and stabilizing for augmented conveyance and erosion control. Added habitat degradation is brought about by pollution due to harmful substances washed from the urban surfaces and resulting from erosion. While sealing and altering stream corridors, the water purification and flood management services by natural wetlands and waterways, the rich and connected habitats in the water–land interphase and areas of high recreational potential are lost, and urban dwellers distanced from local nature.
Wetlands that are created to mitigate the many water environment challenges within an urban watershed, contrary to waste water treatment wetlands receiving a steady inflow, are subjected to great fluctuations in the inflow volume, flow patterns and water quality resulting from natural and built landscape factors as well as weather and climate impacts. In the urbanizing world, a management shift in solving storm water-related problems is both necessary and possible and thus, e.g., the conflicting demands on ecosystem services can be alleviated (cf. Mitsch, Zhang, Anderson, et al. Citation2005; Mitsch, Zhang, Lane Citation2005; Hansson et al. Citation2005; Vohla et al. Citation2007; Mitsch et al. Citation2014). Here, we present a case study in which storm water is treated as an asset and wetlands are established in a residential area to provide storm water management services, critically endangered habitats and recreational amenities.
Two urban wetlands, the Nummela Gateway and the Nummela Niittu, have been designed and implemented as water quality improvement urban park areas (cf. Mitsch, Zhang, Anderson, et al. Citation2005). The total park sizes are 6 ha and 7 ha, respectively. The Gateway wetland was constructed in 2010 at the mouth of a stream between the recipient lake and a commuter highway. The Niittu wetland was constructed in 2012–2014 within a residential area 0.5 km upstream from the Gateway wetland. Excavation works were carried out carefully in winter time on frozen clay soils, preventing both erosion and compaction of soils.
In this paper, we show some results from the ongoing studies on vegetation establishment, water quality improvement and on how fast different animals colonize newly established urban wetlands within boreal climate with four distinct seasons and an average annual temperature of ca. 5°C, as well as comment on how the wetlands can serve as an environmentally sound and accepted park landscape alternative to urban dwellers. Most of the results shown are from the earlier established Nummela Gateway wetland.
The hypotheses of this study included that created wetlands will provide multiple beneficial ecosystem services in an urban setting and that these services can be demonstrated through monitoring in four seasons. It was proposed that such wetland sites can be allowed to self-establish for vegetation. We hypothesized that in addition to sustainable storm water management, such landscapes would provide for a higher biodiversity with increased resilience, lower maintenance and more valuable habitats, as well as higher carbon binding than, e.g., a lawn landscape would. We proposed that we would be able to draw definite conclusions on the impact of the created wetlands on water quality in reference to their size and specific design over four seasons, if we would carry out state of the art continuous monitoring. To test for these hypotheses, we established two adjacent wetlands with slightly different design. The two created wetlands with their adjacent subsites are in different phases of establishment allowing for comparison in wildlife establishment. Traditional discrete water sampling and continuous measurements carried out at 10 min intervals were compared to elucidate year-round functioning of the wetlands in water pollution mitigation.
Materials and methods
Site description
Our case wetlands, , are located within a 550 ha urbanized and urbanizing Kilsoi stream watershed, in the catchment of Lake Enäjärvi, in the Nummela community, Municipality of Vihti, Southern Finland. Lake Enäjärvi has poor water quality, resulting in algal blooms and fish kills, due to both internal phosphorus load from past human activities and run-off from its catchments (e.g., Varis et al. Citation1989; Salonen & Varjo Citation2000). Stream Kilsoi is an inland clay-soil stream located at the headwaters of River Siuntio draining into the Baltic Sea. The inland habitat type clay-dominated stream is red-listed in the Red-list Assessment of Finnish habitat types (Raunio et al. Citation2008) as a critically endangered habitat in Southern Finland. While the major branch of the Kilsoi stream was already sealed in a 260 ha closed sewershed with a large connection culvert released into the stream at one eroding location, a change in the stream sealing practice was made in early twenty-first century. The remaining 2 km straightened channel was given the tasks of providing water environment protection to the recipient lake and the urban and urbanizing watershed, creating clay-stream habitat and establishing sustainable and local environmental protection awareness-raising parks to urban dwellers. Naming the bare conveyance ditch with its old name Kilsoi was the first step in reviving the forgotten stream.
Figure 1. Wetlands within the Kilsoi stream: (1) Lake Enäjärvi shoreline wetland, (2) Gateway wetland, (3) Niittu wetland, (4) flood meadow, (5) pocket wetland and (6) braided wetland. Continuous water monitoring points Stream weir, Gateway wetland inflow and Gateway wetland outflow are shown.
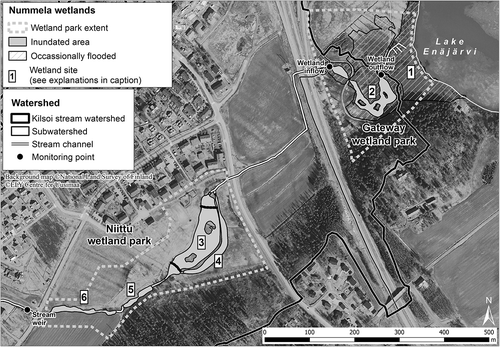
In 2008, the first sustainable storm water management effort within the Kilsoi watershed addressed a 200 m long strongly eroding straightened and cleared stream section adjacent to the largest sewershed, 260 ha, outlet. Mitigation included slight widening and stabilization with rocks, biodegradable seedmats and willow bundles. Wide wetland creation was not possible due to two constraints: the stream bank was privately owned at one side, and underground structures such as wastewater pipelines were buried inside the municipality-owned bank. To meet the goal for urban wetland creation, zoning plans with a minimum of 60 m wide park corridor with no above or below ground hardscape were made along the stream Kilsoi (Salminen et al. Citation2012).
The Nummela Kilsoi stream watershed area contributing to the mitigation wetlands’ inflow consists of a distinctively urban area and an area with agricultural fields undergoing urbanization. Based on land-use assessment, the 260 ha urban subwatershed area is 30% impervious and largely built on a sand and moraine ridge, with a high level of natural infiltration where the soils are not sealed under the urban fabric. The major aquifer beneath the sand and moraine ridge does not flow towards Lake Enäjärvi. The 174 ha agricultural subwatershed currently consists of 30% crop fields. Major run-off contribution within the agricultural subwatershed results from 50 ha of crop fields located along the straightened and cleared stream on clay soil, while the 80 ha of impervious surfaces connected to the sewer network is the main contributor to the urban run-off. The two subwatersheds merge 100 m upstream from the Niittu wetland park and the Stream weir monitoring point.
The Gateway wetland mean water surface area is 0.4 ha, and the Niittu extended wetland area 1.5 ha, including a 0.4 ha flood meadow area and a section of wide braided clay stream. The Gateway wetland main pool was designed to accommodate lake fish, while fish passage to the Niittu wetland has been restricted. The upstream Niittu wetland is intended fishless, as wetland habitats without fish are very rare in Finland. Many wetland species, especially ducks, common newt (Triturus vulgaris), frogs and many aquatic invertebrates, survive much better in fishless habitats, increasing biodiversity in our wetland parks (see Nummi et al. Citation2012). Both wetland parks have been designed to include ample wetland space for amphibian habitat. To provide habitat for waterfowl and to attract people, wider open water areas were designed for both wetland parks than would have been required as stilling pond areas created for water pollution mitigation purposes. Islands were constructed within the open water areas for habitat, for more wetland shoreline, and to direct flow.
Vegetation monitoring
The Nummela wetlands have been excavated on abandoned crop fields undergoing urbanization and herbaceous vegetation has been allowed to fully self-establish at the Gateway wetland. Annual monitoring for species and vegetation coverage (%) in summers 2010, 2011 and 2012 at the Gateway wetland was carried out at 94 plots each of 0.5 m2 organized in a 20 m × 20 m grid. In 2013 and 2014, plant species were counted overall, and the zoning of species to regions relevant to hydrology was evaluated. Species from distinct patches were identified for triplicate 0.5 m2 plots which were harvested for biomass analyses. Presented above-ground samples were dried at 60°C and weighed.
Wildlife observations
Wetlands were monitored for wildlife over monthly visits since 2010. Waterfowl were monitored using the standardized waterfowl ‘round count’ method described by Kauppinen et al. (Citation1991). During waterfowl monitoring other water birds and amphibians were also monitored. In 2014, nektonic and benthic invertebrates were sampled in Niittu, Gateway and Lake Enäjärvi with activity traps, which give an index of abundance and biomass (e.g., Elmberg et al. Citation1992). Activity traps are largely used in studies related with water birds and aquatic invertebrates (e.g., Elmberg et al. Citation1993; Nummi et al. Citation2012). We compared the invertebrate fauna between our study wetlands and the nearby Lake Enäjärvi.
Hydrology and water quality monitoring
Hydrology and water quality was monitored over the time period 1 November 2012 to 31 December 2013, both continuously and with discrete sampling, at the inflow and outflow of the Gateway wetland. The Niittu wetland was disconnected with standing water to prevent erosion from major construction into the stream network and to allow vegetation establishment without disturbance during peak flows till January 2014, and water monitoring at Niittu was thus not feasible during the presented study period.
Wetland hydrological conditions and water quality were monitored with 10 min intervals with automated monitoring stations established at the inflow (60.328293°N, 24.335635°E) and at the outflow (60.328145°N, 24.337756°E) of the Gateway wetland, and . Distance of the two monitoring stations is 250 m across the centre of the flow path during normal flow conditions. Water quality and flows from the two subwatershed areas (urban and agricultural) contributing to the wetlands’ inflow were also monitored continuously. The measuring frequency was selected as it did not have any averaging impact on data in the field conditions specific to the site. The recorded data were transmitted to a data server over a GSM network and visualized in online data service.
Inflow to the wetland was monitored with an acoustic flow meter (StarFLow, Unidata Pty Ltd, O’Connor, ACT, Australia) measuring flow velocity and water level in the stream. A concrete culvert cut in half was placed in the stream to give an even and stable yet open channel shape for anchoring the flow meter in. Discharge was calculated as a function of flow velocity and water level in a defined cross section of the stream. Water level at the outlet was measured with a pressure gauge (STS Sensor Technik Sirnach AG, Sirnach, Switzerland).
Water quality measurements were carried out with four different sensors. Turbidity, oxygen concentration, conductivity, pH and temperature were measured with the YSI-6000 (YSI Incorporated, Yellow Springs, OH, USA) series multiparameter sonde. Nitrogen, dissolved (DOC) and total (TOC) organic carbon concentrations were measured with s::can spectro::lyser™ spectrometer. Both the inflow and the outflow monitoring stations were equipped with YSI and s::can sensors (scan Messtechnik GmbH, Vienna, Austria).
In addition to continuous hydrological and water quality monitoring, local weather conditions were recorded with a Vaisala WXT weather transmitter (Vaisala Oyj, Helsinki, Finland) at the Gateway inflow monitoring station. Rainfall, wind speed and direction, temperature and relative humidity were recorded continuously with 10 min measurement intervals.
The collected hydrological, water quality and meteorological data were used in analyses of wetland processes. In order to ensure long-term autonomous monitoring with high data quality, sensors were equipped with an automatic cleaning system. In addition to automatic cleaning, a manual service routine was also carried out to eliminate the impact of biofouling and pollution. Data from the manual water samples analysed in laboratory were used as a reference and for the calibration of sensor data.
Water samples from incoming and outgoing water of wetland were collected using a 2 l Limnos sampler (Limnos Oyj, Turku, Finland). Altogether 38 sampling sets were taken at different flow circumstances during the continuous monitoring period of 14 months, but sampling was especially focused on spring and autumn flood periods. Sensor data transferred from the measurement stations were used to determine the correct timing. Samples were taken on the measuring depth of sensors exactly at the same time that the sensor measured the water quality.
All water samples were analysed according to European or Finnish standard methods in an accredited laboratory in Helsinki. Suspended solid (SS) concentrations were measured by means of filtration through a 0.45 µm membrane filter (SFS-EN 872). Also, turbidity (SFS-EN ISO 7027) and total phosphorus (TP) (SFS 3026) concentrations were analysed in laboratory.
Sensor turbidity was used as a surrogate measure to concentration of TP and SS concentrations. Turbidity and TP (TP = 1.13Tur + 36.19, n = 19, R2 = 0.93) and turbidity and SS (SS = 0.83Tur + 0.47, n = 19, R2 = 0.99) were related to calculate SS and TP loads every 10 min by multiplying concentration and discharge Q (l s−1). Turbidity and SS and turbidity and TP relationships were established using data collected from both input and output water of the Gateway wetland. Monthly and annual loads were computed as the total sum of these 10 min loads according to Equation (1):
where Ls is the annual load, Qi is discharge at sensor measuring time, Ci is concomitant concentration of SS, TP or NO3–N and n is the total number of measurements during a year.
Theoretical hydraulic retention time was calculated for the Gateway wetland based on the time required for the entire water volume replacement. The volume used in this calculation was based on field measurements in 2010, excluding biomass. Realized hydraulic retention time was calculated based on monitored turbidity peak travel time across the wetland (travel between the inflow monitoring station and outflow monitoring station).
The impact of wetland maturity on water quality improvement was assessed by comparing SS retention in the Gateway wetland during snowmelt over the first 5 years.
Results
Seasonal changes in hydrology
Typical of an urbanized watershed, flow in the Kilsoi stream greatly changes following rain and snowmelt events (). Observed changes in flow from circa 1 l s−1 during low flow to over 1000 l s−1 during heavy rains and spring snowmelt pose a challenge in maintaining clay stream structures. When designing the Nummela water environment park areas, establishing connected clay stream habitats was one central goal. Clay is easily eroding and the fast and high flows from urban watersheds are more erosive than the receiving landscape is adapted to. The installed structures of rock lining and stilling ponds dissipate flow energy during high flow events. Rock bottom dams installed within the stream also maintain water elevation and intended detention during flood events, which were observed for the Gateway wetland: . Even low frequency of inundation () was observed to impact vegetation, and .
Figure 2. Rainfall and temperature in Nummela, and water level at the Gateway wetland during the hydrological year 2012–2013 (1 November 2012 to 31 October 2013) and the calendar year 2013. Growing season in 2013 ranged 29 April to 13 October. An unusual snowmelt event occurred 1–2 January 2013; the end of the year 2013 was unusually warm with no ice formation.
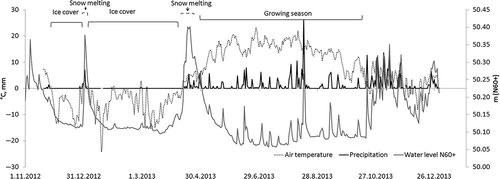
Figure 3. Monitored water level fluctuation within the Gateway wetland impacts vegetation distribution. Background photograph from April 2010 shows the site right after excavation. Construction road, which was strictly used to avoid area compaction, shows in the centre. Continuous water monitoring stations are shown at the inflow and outflow of the wetland.
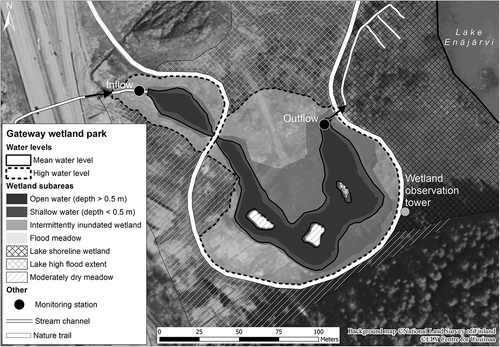
Table 1. Inundation levels reached at the Gateway wetland based on continuous water level monitoring at the outflow monitoring station from 1 November 2012 to 31 December 2013.
Table 2. Distribution of identifiable distinct vegetation patches at the Gateway wetland in 2013.
Development of vegetation
Vegetation self-establishment at the Gateway wetland was rapid, and the vegetation was rich in taxa supporting the hypotheses, and dominated by native species. Vegetation was arranged in dense patches of distinct dominating species. The 20 m × 20 m plot grid division met with definable patches at random in terms of light conditions, while certain patches were found at specific inundation levels ( and and ). In July 2010, already 57 herbaceous species were identified, while many unidentified young shoots were listed. The following July, 80 herbaceous species were identified. The number of non-vegetated plots decreased from 11 to 1, and mean coverage increased from 74% to 117% over the first two growing seasons. Over a dozen species were added the consequent years and a total of 102 mostly native (97%) herbaceous species were identified at the Gateway wetland on the fifth growing season in July 2014, i.e., the number of species had almost doubled.
Definable vegetation patches at the Gateway wetland that were found frequently inundated at water levels below 50.35 m (N60 elevation system; meters from the Baltic Sea level, cf. ) included Typha-type, or areas dominated by Typha latifolia; Iris-type, or areas dominated by Iris pseudacorus, Carex-type, or areas dominated by Carex spp.; and Juncus-type, or areas dominated by Juncus effusus. None of the species formed monocultures, but were accompanied by other, such as Potentilla palustris was commonly found within the Carex-type and Caltha palustris and Calla palustris was found within the Typha-type. The less frequently inundated level, 50.35–50.45 m, was found to be occupied by Filipendula–Lysimachia–Lythrum–type in which the three species, Filipendula ulmaria, Lysimachia vulgaris and Lythrum salicaria, coexist in 1:1:1 ratio. At the edge of the permanently inundated area (around 50.00 m) where the shoreline was formed by excavation in early winter 2010, the plant species in 2013, the fourth growing season, represented a random mixture of the species found in patch types at the levels 50.45 m and below. This establishing wetland-type patch was framed at the water’s edge by primary species Calla palustris and Alisma plantago-aquatica. At the elevations of 50.45–50.60 m, vegetation represents moderately dry meadow species with most areas covered by grasses (such as Poa spp. and Calamagrostis spp.). Besides grass-dominated areas, the drier meadow areas included patches dominated by Cirsium species.
The Nummela Gateway wetland shown in consists of a deep (1.5 m) inlet stilling pool, a meandering open water area with three habitat islands and a deep (1.5 m) outlet pool area. A nature trail embraces the wetland and a wetland observation tower facilitates wildlife observation. Watershed reaching the inflow is 550 ha in size; outflow is released to Lake Enäjärvi through shoreline wetland. Bottom dam at the wetland outflow sets low water level within the Gateway wetland to 50.06 m above the Baltic Sea level (N60 elevation system). Wetland vegetation was observed to reach the 50.35 m level, whereas levels 50.35–50.45 m were occupied by flood meadow species. The area north of the stream-impacted Gateway wetland is flooded by the lake approximately once in 5 years. This wet meadow includes blocked former drainage ditches with an overflow connection to the lake north of the Gateway outflow.
Among the species three (3%) non-native herbaceous plants were found: Elodea canadensis in open water areas, and Epilobium adenocaulon and Epilobium hirsutum in moderately wet to dry meadow areas. The non-native willowherbs (Epilobium) compete with native ones yet they offer similar ecosystem services. E. canadensis offers shelter to many insects, fish and amphibian young. Furthermore, E. canadensis slows down water flow, increasing detention and sediment trapping by the wetland. At the Gateway wetland open water areas, the quantity of E. canadensis is still increasing. Within the Gateway wetland, no native or non-native plant has formed distinctive monoculture areas or is outcompeting other species. Phragmites australis was first found at this wetland in 2014 as few individual plants. While P. australis is not found within the Kilsoi stream watershed today, remains of it are found at depths 30–50 cm below the current Gateway wetland, indicating that during early agricultural era, the mouth of the Kilsoi stream was a prominently reed-covered wetland.
Directly downstream from the storm water collection culvert release to the Kilsoi stream, the invasive species Himalayan balsam (Impatiens glandulifera) has been found. In Southern Finland, this garden escaping annual plant outcompetes native perennial wetland and stream corridor plants such as those present in the Filipendula–Lysimachia–Lythrum-type which is critical for year round stream bank stabilization and for habitat as well as view. Himalayan balsam is of little use to native fauna, providing food only to pollinating insects in late summer when the outcompeted native plants bloom as well. In terms of water environment ecosystem services, as an annual plant, Himalayan balsam does not stabilize clay stream banks in the Southern Finland climate as its soft shoots and roots are withered during the high flows of fall rain and spring snowmelt. Thus, the Himalayan balsam must be controlled within the Kilsoi watershed for maintaining the intended wetland environment vegetation. Locals have been engaged into controlling this plant and efforts are ongoing to remove it from the entire watershed.
Vegetation in an urban landscape may serve the functions of primary production and carbon sink. Measuring above-ground biomass bound at various landscape types demonstrates levels at which vegetation types may provide these services. At the Gateway wetland, the major dry meadow types Cirsium and Poa-Calamagrostis show similar levels of biomass, which is at a level between the lowest landscape type measured, the lawn, and the highest, the Typha-type intermittently inundated wetland (). Mixed vegetation found at the 2010-constructed new shoreline showed the second highest biomass level, followed by the flood meadow Filipendula–Lysimachia–Lythrum-type. While the Juncus-type provided more biomass than the dry meadow landscapes, the Carex- and Iris-type wetlands were lower in average biomass content than the observed dry meadows. The overall highest plants present at the Gateway wetland were individuals of Typha latifolia and Cirsium spp., correlating with observed high biomass values. While the invasive Impatiens glandulifera was not found at the Gateway wetland, it is present upstream. The patches close to monoculture, found downstream from the storm water sewershed release culvert, were used to collect I. glandulifera for biomass. This annual invasive plant was shown to hold the lowest biomass of wetland and flood meadow plants measured, yet it successfully outcompetes native species in areas from the shoreline to flood meadows. The lawn studied was located on fill next to the storm water 260 ha sewershed outlet culvert. In terms of primary production and carbon sink, the Gateway native wetland and landscapes occupied by flood meadow species outcompeted lawn and non-native species patches.
Establishment of wildlife
Activity trap sampling of aquatic invertebrates revealed fast colonization in both wetlands following excavation and landforming works. From Niittu and Gateway, 12 invertebrates groups were found, whereas from the nearby Lake Enäjärvi only 10 groups of aquatic invertebrates were found. In constructed wetlands, the most common groups were Cladocera, Copepoda, Hydracarina and Dytiscidae, whereas in Lake Enäjärvi the most common groups were Cladocera, Copepoda, Hydracarina and Isopoda.
At the time of ice melt in early spring, pikes come to spawn at the Gateway wetland and insects begin to feed on willow flowers. Spawning amphibians as well as nesting birds quickly follow. In summertime, the wetland is bursting with sights and sounds of wildlife. When phase 1 of the Niittu wetland excavation was completed in April 2013, both common frogs, Rana temporaria, and common newts, Triturus vulgaris, were found spawning in the constructed ponds in May with successful adults spotted in June. In June, newts were also found in one of the invertebrate traps. Amphibians were similarly found at the Gateway wetland following construction in 2010. The Gateway wetland, however, was connected to existing lakeside shore wetlands, while phase 1 of the Niittu construction included isolated low ponds in a dry field next to a residential area in 2013.
Six wetland sites were monitored for frog spawning in spring 2014. The areas in the order from upstream to downstream were: braided wetland, pocket wetland, Niittu wetland and flood meadow, Gateway wetland and Lake Enäjärvi shoreline wetland, . At the Gateway wetland, the spawn clusters were found at pockets isolated from the main pool with vegetation which protects the frogs from strong flows and predatory fish. The pocket wetland had well-established vegetation while the 2013–2014-constructed areas were still largely lacking vegetation cover in 2014.
Spawning of frogs spanned over the period 15 April to 15 May in 2014, . It was first observed at the small in-stream 2011-constructed wetland under the conditions of mean air and water temperatures of 5°C, maximum water temperature 10°C and average flow 20 l s−1. Spawning at this site was richest in numbers of observed eggs and spanned over an earlier, shorter and more intensive time than observed at the larger wetland areas. During high flows following rain events in early May, spawning was observed at the Gateway wetland and at the Lake shoreline wetland within areas protected from high flows and fish by dense wetland vegetation.
Figure 5. Weather in Nummela, as well as flow and water temperature at the Stream weir monitoring station during frog spawning in 2014.
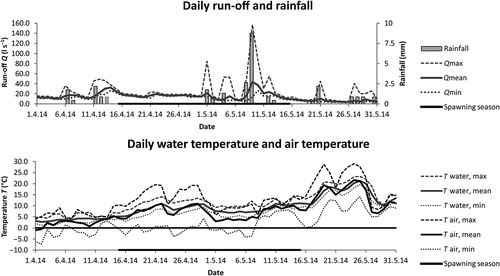
The pocket wetland with its lush vegetation and absence of fish was by far the most preferred spawning location for frogs within the monitored area, . This table also shows the sighting locations of individual newts and toads as their spawn cannot be counted in the same manner as frogspawn. A forested hillside follows the wetlands along the entire length of the parks, providing sites for amphibian hibernation.
Table 3. Results from frogspawn cluster count conducted in 2014 at the Nummela wetlands.
Waterbirds bred at both wetlands already during the first summer following excavation. Green sandpiper (Tringa ochropus) bred at the Gateway wetland already in 2010 and black-headed gull (Larus ridibundus) at the Niittu wetland in 2013. There were eight breeding duck pairs at the Gateway wetland, with only 0.4 ha of open water area in 2012, with one Eurasian teal (Anas crecca), two common goldeneye (Bucephala clangula) and five mallard (Anas platyrhynchos) pairs. The Niittu wetland also attracted one pair of nesting northern lapwing (Vanellus vanellus) in 2013 and two pairs in 2014. Three pairs of little ringed plover Charadrius dubius bred in Niittu in 2014. In addition, one pair of common moorhen Gallinula chloropus bred successfully in Gateway wetland.
Hydrology and water quality
Water quality and flows from the two subwatershed areas, urban and urbanizing agricultural, contributing to the wetlands’ inflow, were monitored continuously over a 14-month period with following observations: flows and water quality fluctuate over the four seasons with rain and snowmelt events as well as construction works contributing to the highest levels of water turbidity. Soils within the subwatersheds had a significant impact on infiltration, resulting in a lower volume/ha run-off observed from the larger urban watershed of highly pervious soils than from the agricultural watershed of naturally low-in-infiltration clay soils. The highest monthly inflow loads of SS to the wetlands were observed during snowmelt in April 2013 (21 kg) and the fall rainy season in November 2013 (36 kg), .
Table 4. Monthly averages of load reductions by the Gateway wetland during the hydrological year from November 2012 to October 2013 and the calendar year 2013.
There was a clear difference in outflow conductivity from the two subwatersheds: mean conductivity in urban run-off during November 2012 and April 2013 was 228 µS cm−1 and in agricultural run-off, 131 µS cm−1. In urban run-off, 96% of variation (n = 19) of conductivity was explained by SO4 and Cl− concentrations. Highest Cl− concentration (69 mg l−1) was detected in the end of March 2013, concomitant with slight conductivity peak (319 µS cm−1). The source of the Cl− was suggested to be in de-icing substances used especially in urban area during winter time. No clear impact on conductivity was detected by the wetlands.
Continuous water monitoring over four seasons from 1 November 2012 to 31 December 2013 at the Gateway wetland inflow and outflow demonstrated that the wetland does reduce the loading of pollutants, such as phosphorus (10% over the hydrological year 2012–2013), into Lake Enäjärvi. If monitoring had been conducted by intermittent manual sampling only, the impact would not have been detected (). Observed pollutant reductions varied and depended on season, inflow concentration, characteristics of the preceding hydrological events (both recent and over the ongoing hydrological year), as well as design and maturity of the constructed wetland. The highest observed reduction of phosphorus was 71%, following a rain event late in the 2013 growing season ( and and ). The highest observed inflow phosphorus loads were related to the melting of snow (April 2013) and heavy rain late in the fall (November 2013). In both months, reduction reached 8% which, however, referred to 4 kg load reductions. The reduction rates were highest between July and September, when loads were low. The annual average reduction, 10%, is one-third of the highest observed monthly average of 31% (July 2013), which, however, referred to only 0.5 kg, and one-seventh of the highest observed event reduction of 71%. Seasonal and event-based variation was observed for NO3–N and DOC as well, however, the annual average reduction for both was slightly lower than that observed for phosphorus. Best NO3–N reductions were reached at low loads in winter and summer. In the end of the growing season, NO3–N was released from the wetland. Due to susceptibility of Lake Enäjärvi to eutrophication, and the tangible impact of algal blooms on urban dwellers, the reduction of phosphorus-rich clay particles in water remains of particular concern in Nummela.
Figure 6. Small urban streams have strongly fluctuating flows and changing pollutant concentrations, which necessitates the employment of continuous sensor-based monitoring as compared to manual sampling to detect water pollution mitigation needs and services provided by wetlands. SS concentration monitored at the Gateway wetland outflow station from 1 November 2012 to 25 May 2013 is shown as an example.
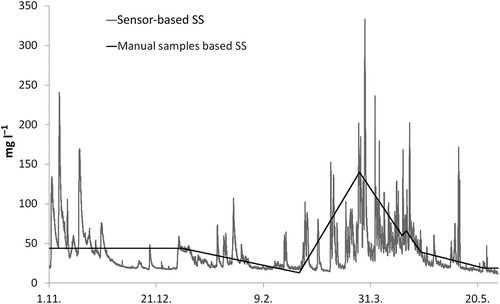
Figure 7. Continuous water monitoring results from 1 November 2012 to 31 December 2013 for TP for the Nummela Gateway wetland inflow and outflow. The TP values have been calculated with a sensor-based turbidity – manual sample-based TP correlation from turbidity values measured at 10 min intervals. The highest phosphorus loads relate to heavy rain events outside the growing season and the melting of snow, while the highest relative reductions occurred during growing season low loads.
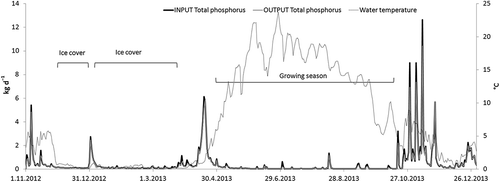
Figure 8. An event-based detail for continuous water monitoring results from 11 to14 September 2013 for TP at the Nummela Gateway wetland inflow and outflow. Reduction of TP during this late growing season rain event was 71%. Even with an event like the high reduction one shown, monitoring solely by manual sampling, e.g., late in the day on 12 September could have resulted in misinterpretation of the wetland’s water pollution mitigation abilities.
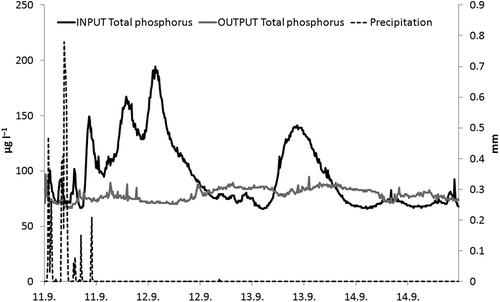
Monitored dissolved oxygen concentration in winter was lower in effluent water, indicating oxygen to be consumed in the wetland under ice cover (). Mean concentration in the effluent water from January to March was 11.5 mg l−1 and in the influent water, 12.6 mg l−1. Lowest O2 concentration (0.2 mg l−1) was measured in the wetland effluent after a rain event on 9 August 2013. Difference between O2 concentration in influent and effluent was at its highest on 21 September 2013, when the daily average of O2 was 5.0 mg l−1 lower in the wetland outflow water than was observed in the inflow.
Figure 9. Monitored dissolved oxygen levels at the inflow and outflow of the Gateway wetland in 2012–2013 showed seasonal variation relating to changes in water temperature, growing season and decomposition of organic matter; and diurnal variation during growing season.
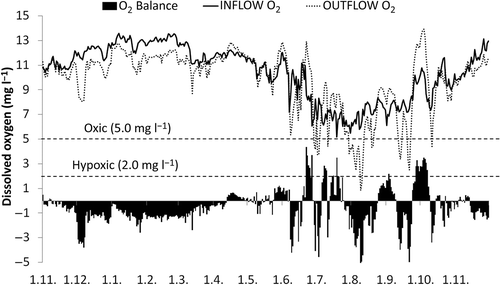
Theoretical hydraulic retention time at the Gateway wetland exceeded the realized monitoring time throughout the year, . The realized retention time remains below 24 h, which means that water finds fast routes through the wetland. Periods of low water flow velocities and high hydraulic retention time during the growing season contribute to higher observed SS reductions than during the high flow, and thus low hydraulic retention time periods during the fall rainy season and spring snowmelt season. Vegetation as well as filamentous algae contribute to trapping SS and nutrients during growing season. Elodea canadensis along with dormant wetland plants contributes to turbidity reduction throughout the year.
The Gateway wetland showed well-established herbaceous vegetation by the second growing season, yet the number of species and biomass continued to increase through the monitored 5 years. Continuous water quality monitoring during snowmelt in 2010–2014, , showed that the monthly average reduction of SS increased through the first three growing seasons.
Discussion
The wetlands Gateway and Niittu were designed to provide multiple ecosystem services to an urban community (Zedler & Leach Citation1998). The acceptance and appreciation of constructed wetlands by urban dwellers is important. The many beneficial functions of wetlands need to be demonstrated to and recognized by decision-makers. A holistic approach in both designing and monitoring urban wetlands was attempted in Nummela to meet these tasks. In our hypotheses, we envisioned to bring state-of-the-art monitoring-based insight into wetland science applicable to watershed-scale urban planning, wetland location and allowed area definition by zoning, and wetland hydraulic dimensioning, as they relate to the establishment of diverse vegetation and wildlife, threatened habitats and the mitigation of water environment challenges. Water quality and water provision are the most referred to and studied ecosystem services after carbon storage and sequestration and food production (Martínez-Harms & Balvanera Citation2012). Whereas the changes in hydrology resulting from land-use change and climate change pose threats to water environments beyond borders, through proper planning and design, these changes can, and should, be compensated for within the urban fabric.
Water treatment by wetlands relies on the work of plants and their associated microbes (Stottmeister et al. Citation2003). Since storm water acts as the carrier for potentially any pollutants in use and reaching an urban surface, high diversity of plants and microbes is needed for treatment (Tanner Citation1996; Read et al. Citation2008). Besides vegetation diversity, the species native origin was also found to be important to protect urban streams from the erosive storm and snowmelt flows year round (cf. Bonilla-Warford & Zedler Citation2002). As a headwater stream, the Nummela Kilsoi offers an example for controlling the invasive annual Himalayan balsam, which is found and weeded upstream from the Gateway and Niittu wetlands, at a watershed scale (c.f. Dawson & Holland Citation1999).
The Gateway wetland herbaceous plant self-establishment in 2010 was rapid and diverse with mainly native species. Construction included only defining the presence of water through changes in microtopography and wintertime excavation during low flow conditions and vegetation dormancy. Compaction of soils during construction was avoided. Nearby shoreline and old drainage ditches provided a seedbank, and by the fifth growing season, with no maintenance 6 ha of mainly native plants are increasingly supporting diverse native food webs. We expect the larger Niittu wetland complex, established in 2012–2014 further away from the lake and including a test site for planting, to reveal us more about the benefits and possible challenges of wetland self-vegetation.
While the wetlands were designed as amphibian and waterfowl habitats, the way how the excavated wetland sites became successful breeding grounds for both amphibians and birds the month after wintertime excavation was completed was very rapid and not foreseen. Only two of the European Commission (EC) habitats directive species are verifiably found and greatly supported by the Nummela wetlands: the moor frog, Rana arvalis, and the common frog, Rana temporaria. These species have been found to be rapid also in their colonization of beaver ponds (Vehkaoja & Nummi Forthcoming Citation2015). The Gateway and Niittu wetlands were successful in creating high biodiversity at the clay–stream habitat scale in the centre of a Southern Finland residential community. Relying on abundant invertebrates and shallow water (Nummi & Hahtola Citation2008), waterbirds colonized the newly formed wetlands rapidly; this is especially typical of the Eurasian teal and green sandpiper, but mallards were also numerous (Kantrud & Stewart Citation1977; Nummi & Holopainen Citation2014). An especially valuable waterbird species was the moorhen, which is a very rare breeding bird in Finland. Likewise, bats used the created wetlands intensively, as they do with beaver ponds (Nummi et al. Citation2011). In addition, aquatic invertebrates, especially dytiscids, were numerous in the created wetlands. We found no dytiscids in Lake Enäjärvi where predatory fish are abundant, whereas they are common both in Gateway wetland and Niittu wetland. Especially large dytiscids avoid predatory fish (Nummi et al. Citation2012). It should be noted that even though the patch-level diversity of organisms in flooded areas may not be much higher than in permanent waters, the landscape-level diversity may become higher if there are different kinds of organisms in flowages (Wright et al. Citation2002).
While the Gateway wetland mean inundated area is only 0.1% of its 550 ha watershed area, and the monitored year 2013 only the fourth growing season since wetland excavation, an annual average of 10% for TP reduction was reached. In peatland forestry, long-restored peatland buffers between managed areas and recipient watercourses with the size of over 1% of the watershed area have been noted to be effective in SS and nutrient trapping (Nieminen et al. Citation2014).
While the Gateway wetland has a meandering open water route, which allows for realized low hydraulic retention times, the Niittu wetland is but triple in size to the Gateway wetland; it also includes shallow areas within the mean flow path where water has to pass through completely vegetated areas. It remains to be seen how pollution removal improves with the chain of Nummela-created wetlands continuing. Both the presented urban wetland parks were designed to accommodate open water areas for habitat and recreation at the cost of densely vegetated areas for more efficient pollution removal. We felt that this compromise was essential both for wildlife conservation and environmental awareness-raising and acceptance in urban areas. It remains to be noted that wetlands created to mitigate run-off volume and pollution in urban areas do not remove the need for volume and pollutant source control: attention needs to be given to dimensioning imperviousness and the overall use of harmful substances. The better the source storm water pollution control, the more productive urban wetlands’ habitat and recreational services may become.
Majority of the nitrogen load to the wetland was in the form of NO3–N, and thus denitrification would be the main process in nitrogen removal (Poe at al. Citation2003). However, it is known that rates of denitrification are, in general, lower in Finnish lakes and their littoral zones than in temperate zone due to lower temperatures and NO3–N concentrations (Rissanen et al. Citation2011, Citation2013). Even if the relative reduction rate was higher in March and September (48% and 61%, respectively), the residence time in the Gateway wetland was probably too short to effectively remove nitrate from run-off water in annual perspective (Arheimer & Wittgren Citation2002).
Unlike nitrogen, phosphorus input to the wetland was mainly observed in particulate form, and thus the TP removal was mainly resulting from sedimentation. Reduction rate of SS has been suggested to be strongly related to the wetland area to watershed area ratio, and the Gateway wetland did achieve annual relative TP and SS reduction rates as expected on the basis of previous reported studies (Koskiaho Citation2006; Puustinen et al. Citation2007). However, previously reported studies have been mostly based on discrete water sampling or composite sampling rather than continuous monitoring (Braskerud Citation2002; Vohla et al. Citation2007; Lu et al. Citation2009). In our study, the high frequency-automated monitoring was observed to give much more reliable nutrient load estimations than discrete sampling. Very accurate information on the TP, NO3–N and SS retention in the small constructed wetland monitored was acquired during highly varying water quality and quantity conditions.
Unlike in warmer climates, at the latitudes of Southern Finland, tree canopies do not provide the extent of shade that they would fast or completely shade and remove wetland vegetation. At the Nummela wetland parks, there is a plan for controlling woody vegetation; however, in Nummela, the threshold for open space maintenance is brought up by fauna rather than light-requiring wetland vegetation: in order to support, e.g., songbirds, both open areas and shrubbery, as well as all the habitat types from open water to forest, are needed. While excessive moisture in itself controls the establishment of woody plants, the parks are monitored for the need of open space maintenance within the designated meadow and wetland areas. According to the current estimate, meadow maintenance cutting will be necessary every 5 to 10 years.
The local public interviewed in Nummela has found the established wetland parks very appealing due to the diversity of plants and animals which they encountered, and because these native landscapes ‘changed every visit’, providing ‘endless surprises’ and ‘pride’ of own residential neighbourhood. While diversity of vegetation was attempted in the design for the purposes of water pollution mitigation and habitat enhancement, it was found that locals valued such amenities as sounds of songbirds and water movement at the wetlands more than they appreciated the wetlands’ storm water management services. It appears that wetlands provide a far richer palette of ecosystem services to the urban setting than mere storm water management. Promoting wetlands as an integral part of urban areas, which is necessary to improve water quality in receiving waters, is made possible by acknowledging and designing for the many services that they provide.
Conclusion
In overall conclusion, monitoring of the Nummela wetlands demonstrated that the establishment of these landscapes did successfully compensate for various challenges brought about by urbanization; the extent of compensation related to each monitored wetland size, design, and maturity. The hypotheses on allowing herbaceous vegetation to self-establish, and achieving high species diversity and higher than a typical lawn landscaping carbon binding were supported. Diverse perennial native vegetation provided for high resilience towards erosive flows and required no maintenance during the first 5 years of observation. The proposed state-of-the-art continuous monitoring allowed for drawing definite conclusions on the impact of the created wetlands on water pollution mitigation as well as helped in defining connections on vegetation self-establishment with flooding and amphibian spawning with flow patterns. The number of vegetation species increased each year. Frog spawning was most numerous in the most mature created wetland subsite with best established vegetation. Continuous monitoring of hydrology and water quality demonstrated that the created wetlands were able to provide water purification services year-round; the extent of water quality mitigation observed related to the wetland’s size, design, and maturity, as well as ongoing season and weather patterns. Water quality mitigation was demonstrated with continuous monitoring, and would not have been detected with discrete water sampling. The observed 5 years showed an increase in phosphorus reduction following the first three growing seasons, yet further monitoring is needed to elucidate the impact of wetland maturity on water pollution mitigation. The wetlands were found to provide a landscape-scale laboratory for observing the establishment of a critically endangered habitat in an urban site.
Disclosure statement
No potential conflict of interest was reported by the authors.
Acknowledgements
The Gateway Wetland Park was a Vihti Municipality contribution to the UN International Year of Biodiversity (Salminen et al. Citation2012). The presented demonstration of the Nummela wetland’s ecosystem services, as well as the planning and implementation of Niittu Wetland Park belong to the ongoing 2012–2017 EC Life+11 ENV/FI/911 Urban Oases project which promotes water environment mitigation by urban landscapes designed and implemented as multifunctional oases.
Continuous monitoring for water quality was conducted by the skilled staff of the Luode Consulting Oy; we owe special acknowledgements to Mikko Kiirikki and Joose Mykkänen for assistance on data analyses and interpretation. Sincere thanks go to Eeva Vaahtera, Kukka Koskinen, Sari Juutinen and Egle Köster from the University of Helsinki Department of Forest Sciences (UH) for vegetation field work, as well as to Timo Lehto, Tuukka Jussila and Ville Turunen from the Environment and Natural Resources, Centre for Economic Development, Transport and the Environment for Uusimaa (UUDELY) for assistance in defining the watershed and wetland landscapes in the field and by geographic information systems. Mika Rekola from UH is kindly acknowledged for organizing interviews on wetland ecosystem services in Nummela. Mikko Yli-Rosti from the Vihti Lake Enäjärvi Water Protection Association is acknowledged for assistance in various tasks in the field and for maintaining vivid collaboration on environmental protection at the local level.
References
- Arheimer B, Wittgren HB. 2002. Modelling nitrogen removal in potential wetlands at the catchment scale. Ecol Eng. 19:63–80. doi:10.1016/S0925-8574(02)00034-4
- Bonilla-Warford CM, Zedler JB. 2002. Potential for using native plant species in stormwater wetlands. Environ Manage. 29:385–394. doi:10.1007/s00267-001-0032-0
- Braskerud B. 2002. Factors affecting phosphorus retention in small constructed wetlands treating agricultural non-point source pollution. Ecol Eng. 19:41–61. doi:10.1016/S0925-8574(02)00014-9
- Dawson FH, Holland D. 1999. The distribution in bankside habitats of three alien invasive plants in the U.K. in relation to the development of control strategies. Hydrobiologia. 415:193–201. doi:10.1023/A:1003872325274
- Elmberg J, Nummi P, Poysa H, Sjoberg K. 1993. Factors affecting species number and density of dabbling duck guilds in North Europe. Ecography. 16:251–260. doi:10.1111/j.1600-0587.1993.tb00214.x
- Elmberg J, Nummi P, Pöysä H, Sjöberg K. 1992. Do intruding predators and trap position affect the reliability of catches in activity traps? Hydrobiologia. 239:187–193. doi:10.1007/BF00007676
- Hansson L-A, Brönmark C, Anders Nilsson P, Åbjörnsson K. 2005. Conflicting demands on wetland ecosystem services: nutrient retention, biodiversity or both? Freswat Biol. 50:705–714. doi:10.1111/j.1365-2427.2005.01352.x
- Jylhä K, Tuomenvirta H, Ruosteenoja K. 2004. Climate change projections for Finland during the 21st century. Bor Env Res. 9:127–152.
- Kantrud HA, Stewart RE. 1977. Use of natural basin wetlands by breeding waterfowl in North Dakota. J Wildl Manage. 41:243–253. doi:10.2307/3800601
- Kauppinen J, Koskimies P, Väisänen RA. 1991. Waterfowl round count. In: Koskimies P, Väisänen RA, editors. Monitoring bird populations. Helsinki (Finland): Zoological Museum, Finnish Museum of Natural History; p. 45–53.
- Koskiaho J 2006. Retention performance and hydraulic design of constructed wetlands treating runoff waters from arable land [ dissertation]. Acta Univ Oulu Ser C Technica 252. Oulu (Finland): University of Oulu.
- Kovats RS, Valentini R, Bouwer LM, Georgopoulou E, Jacob D, Martin E, Rounsevell M, Soussana JF. 2014. Climate change 2014: impacts, adaptation, and vulnerability. Part B: regional aspects. In: Barros VR, Field CB, Dokken DJ, Mastrandrea MD, Mach KJ, Bili TE, Chatterjee M, Ebi KL, Estrada YO, Genova RC, et al., editors. Contribution of working group ii to the fifth assessment report of the intergovernmental panel on climate change. Cambridge (UK): Cambridge University Press; p. 1267–1326.
- Lu S, Wu F, Lu Y, Xiang C, Zhang P, Jin C. 2009. Phosphorus removal from agricultural runoff by constructed wetland. Ecol Eng. 35:402–409. doi:10.1016/j.ecoleng.2008.10.002
- Martínez-Harms MJ, Balvanera P. 2012. Methods for mapping ecosystem service supply: a review. Int J Biodivers Sci, Ecosyst Serv Manag. 8:17–25. doi:10.1080/21513732.2012.663792
- Mitsch WJ, Zhang L, Anderson CJ, Altor AE, Hernández ME. 2005. Creating riverine wetlands: ecological succession, nutrient retention, and pulsing effects. Ecol Eng. 25:510–527. doi:10.1016/j.ecoleng.2005.04.014
- Mitsch WJ, Zhang L, Lane RR. 2005. Nitrate-nitrogen retention in wetlands in the Mississippi river basin. Ecol Eng. 24:267–278. doi:10.1016/j.ecoleng.2005.02.005
- Mitsch WJ, Zhang L, Marois D, Song K. 2014. Protecting the Florida Everglades wetlands with wetlands: can stormwater phosphorus be reduced to oligotrophic conditions? Ecol Eng. Available from: http://dx.doi.org/10.1016/j.ecoleng.2014.10.006
- Nieminen M, Kaila A, Koskinen M, Sarkkola S, Fritze H, Tuittila E-S, Nousiainen H, Koivusalo H, Laurén A, Ilvesniemi H, et al. 2014. Natural and restored wetland buffers in reducing sediment and nutrient export from forested catchments: Finnish experiences. In: Vymazal J, editor. The role of natural and constructed wetlands in nutrient cycling and retention on the landscape. Switzerland: Springer International Publishing; p. 57–72.
- Nummi P, Hahtola A. 2008. The beaver as an ecosystem engineer facilitates teal breeding. Ecography. 31:519–524. doi:10.1111/j.0906-7590.2008.05477.x
- Nummi P, Holopainen S. 2014. Whole-community facilitation by beaver: ecosystem engineer increases waterbird diversity. Aquat Conserv: Mar and Freshwater Ecosyst. 24:623–633. doi:10.1002/aqc.2437
- Nummi P, Kattainen S, Ulander P, Hahtola A. 2011. Bats benefit from beavers: a facilitative link between aquatic and terrestrial food webs. Biodivers Conserv. 20:851–859. doi:10.1007/s10531-010-9986-7
- Nummi P, Väänänen V-M, Rask M, Nyberg K, Taskinen K. 2012. Competitive effects of fish in structurally simple habitats: perch, invertebrates, and goldeneye in small boreal lakes. Aquat Sci. 74:343–350. doi:10.1007/s00027-011-0225-4
- Poe A, Piehler M, Thompson S, Paerl H. 2003. Denitrification in a constructed wetland receiving agricultural runoff. Wetlands. 23:817–826. doi:10.1672/0277-5212(2003)023[0817:DIACWR]2.0.CO;2
- Puustinen M, Koskiaho J, Jormola J, Järvenpää J, Karhunen A, Mikkola-Roos M, Pitkänen J, Riihimäki J, Svensberg M, Vikberg P. 2007. Maatalouden monivaikutteisten kosteikkojen suunnittelu ja mitoitus [Multipurpose wetlands for agricultural water protection – guidelines of wetland planning and dimensioning]. The Finnish Environment 21/2007. Helsinki (Finland): Finnish Environment Institute.
- Raunio A, Schulman A, Kontula T, editors. 2008. Suomen luontotyyppien uhanalaisuus – Osa 1: tulokset ja arvioinnin perusteet [Assessment of threatened habitat types in Finland – Part 1: results and basis for assessment]. Suomen ympäristö 8/2008. Helsinki (Finland): Suomen ympäristökeskus. 264 s.
- Read J, Wevill T, Fletcher T, Deletic A. 2008. Variation among plant species in pollutant removal from stormwater in biofiltration systems. Water Res. 42:893–902. doi:10.1016/j.watres.2007.08.036
- Rissanen AJ, Tiirola M, Hietanen S, Ojala A. 2013. Interlake variation and environmental controls of denitrification across different geographical scales. Aquat Microb Ecol. 69:1–16. doi:10.3354/ame01619.
- Rissanen AJ, Tiirola M, Ojala A. 2011. Spatial and temporal variation in denitrification and in the denitrifier community in a boreal lake. Aquat Microb Ecol. 64:27–40. doi:10.3354/ame01506
- Salminen O, Ahponen H, Valkama P, Vessman T, Rantakokko K, Vaahtera E, Taylor A, Vasander H, Nikinmaa E. 2012. Benefits of green infrastructure – socioeconomic importance of constructed urban wetlands (Nummela, Finland). In: Kettunen M, Vihervaara P, Kinnunen S, D’Amato D, Badura T, Argimon M, Ten Brink P, editors. Socio-economic importance of ecosystem services in the Nordic Countries – Synthesis in the context of The Economics of Ecosystems and Biodiversity (TEEB). Copenhagen: Nordic Council of Ministers; p. 247–254.
- Salonen V-P, Varjo E. 2000. Vihdin Enäjärven kunnostuksen vaikutus pohjasedimentin ominaisuuksiin [The effects of restoration actions at the Lake Enäjärvi in Vihti, Finland on bottom sediment characteristics]. Geologi. 52:159–163. Finnish.
- Stottmeister U, Wießner A, Kuschk P, Kappelmeyer U, Kästner M, Bederski O, Müller RA, Moormann H. 2003. Effects of plants and microorganisms in constructed wetlands for wastewater treatment. Biotechnol Adv. 22:93–117. doi:10.1016/j.biotechadv.2003.08.010
- Tanner CC. 1996. Plants for constructed wetland treatment systems — A comparison of the growth and nutrient uptake of eight emergent species. Ecol Eng. 7:59–83. doi:10.1016/0925-8574(95)00066-6
- Varis O, Sirvio H, Kettunen J. 1989. Multivariate analysis of lake phytoplankton and environmental factors. Arch Hydrobiol. 117:163–175.
- Vehkaoja M, Nummi P. Forthcoming 2015. Beaver facilitation in the conservation of boreal anuran communities. Herpetozoa. 28.
- Vohla C, Alas R, Nurk K, Baatz S, Mander Ü. 2007. Dynamics of phosphorus, nitrogen and carbon removal in a horizontal subsurface flow constructed wetland. Sci Total Environ. 380:66–74. doi:10.1016/j.scitotenv.2006.09.012
- Wright JP, Jones CG, Flecker AS. 2002. An ecosystem engineer, the beaver, increases species richness at the landscape scale. Oecologia. 132:96–101. doi:10.1007/s00442-002-0929-1
- Zedler JB, Leach MK. 1998. Managing urban wetlands for multiple use: research, restoration, and recreation. Urban Ecosyst. 2:189–204. doi:10.1023/A:1009528505009