ABSTRACT
The production of sufficient food for an increasing global population while conserving natural capital is a major challenge to humanity. Tree-mediated ecosystem services are recognized as key features of more sustainable agroecosystems but the strategic management of tree attributes for ecosystem service provision is poorly understood. Six agroforestry and tree cover transition studies, spanning tropical/subtropical forest zones in three continents, were synthesized to assess the contribution of tree cover to the conservation of biodiversity and ecosystem services. Loss of native earthworm populations resulted in 76% lower soil macroporosity when shade trees were absent in coffee agriculture. Increased tree cover contributed to 53% increase in tea crop yield, maintained 93% of crop pollinators found in the natural forest and, in combination with nearby forest fragments, contributed to as much as 86% lower incidence for coffee berry borer. In certain contexts, shade trees contributed to negative effects resulting from increases in abundance of white stem borer and lacebugs and resulted in 60% reduction of endangered tree species compared to forest. Managing trees for ecosystem services requires understanding which tree species to include and how to manage them for different socio-ecological contexts. This knowledge needs to be shared and translated into viable options with farming communities.
Edited by Christian Albert
1. Introduction
Agriculture is the most prevalent of the human–environment interaction and consumes more natural resources than any other human activity (FAO Citation2007). This has raised concerns about natural resource management trajectories as they relate to planetary boundaries and land degradation tipping points (Rockström et al. Citation2009). Conventional agriculture in the last century led to a dramatic increase in crop yields largely as a result of the introduction of new high-yielding crop varieties, the use of mineral fertilizers, chemical pesticides, mechanization and the expansion of irrigation infrastructure (Foley et al. Citation2005). While agriculture that overcomes production constraints through external inputs has been one way to increase yields per hectare, these agricultural systems have often resulted in negative economic and environmental side effects in part due to their low resource-use efficiency and negative impacts on biodiversity (Matson et al. Citation1997). Therefore, in recent years, increasing attention on productive agricultural systems that are resource-use efficient has placed biodiversity at the center-stage of discussions on agricultural intensification (Brussaard et al. Citation2010; Snapp et al. Citation2010; Barrios et al. Citation2015; Prabhu et al. Citation2015).
The valuable contribution of trees to more ecological forms of agricultural intensification has been increasingly highlighted in the literature (Schroth et al. Citation2004; Pretty et al. Citation2006; Tscharntke et al. Citation2012a). Ecological intensification can be defined as generating more output from the same area of land while reducing the negative environmental impacts by decreasing the reliance on anthropogenic inputs through the management of regulating and supporting ecosystem services in agricultural practices (Bommarco et al. Citation2013). Further, it also recognizes the valuable contribution of farmer’s knowledge to the generation of technologies better adapted to context variation (Tittonell Citation2014). Agroforestry, broadly defined as the integration of trees and agriculture, has been increasingly recognized as a multifunctional land management option that can simultaneously contribute to income, food security and conservation of biodiversity and ecosystem services (Bhagwat et al. Citation2008; Tscharntke et al. Citation2011; Kuyah et al. Citation2016). Trade-offs among these potential benefits to rural communities, however, are common as it is well recognized that agroforestry trees can negatively influence crop production and income if not properly selected and managed due to competitive effects for light, water and nutrients (Beer et al. Citation1998; Ong et al. Citation2004; Steffan-Dewenter et al. Citation2007; Kuyah et al. Citation2016). Nonetheless, if we consider tree cover on agricultural land greater than 10% as agroforestry, this land use would represent over 1 billion ha of land inhabited by 900 million people (Zomer et al. Citation2016) and hence of significant importance to the agriculture sector. Recent concern, however, has been raised about the global decline in shade tree cover particularly in coffee agroecosystems and the potential implications for biodiversity, ecosystem services and livelihoods (Jha et al. Citation2014).
Biodiversity responsible for key ecological functions that provide benefits to society (i.e. ecosystem services) is a key component of agricultural sustainability strategies (Kremen Citation2005; Balvanera et al. Citation2006; Barrios Citation2007). Biodiversity in agricultural landscapes can be broadly separated into the better studied, visible aboveground biodiversity, which may be directly or indirectly managed by farmers like plants (e.g. shade trees) and insects with bio-control and pollination functions (e.g. parasitoid wasps, bees), and the less-studied, largely invisible and mostly unmanaged soil biodiversity contributing to soil health (Swift et al. Citation2004; Wall et al. Citation2010). Soil health is considered here as an integrative property that expresses the capacity of soil to respond to agricultural management where both agricultural production and the provision of other soil-mediated ecosystem services are maintained (Kibblewhite et al. Citation2008). The complexity of interactions between soil biodiversity and ecological functions underpinning soil health requires a focused approach on sets of soil organisms playing major roles (Beare et al. Citation1995; Barrios Citation2007). Efforts in this direction show that soil organisms can be grouped into four key functional assemblages: (1) decomposers, (2) nutrient transformers, (3) ecosystem engineers and (4) biocontrollers, each composed of several functional groups which contribute to four key aggregated ecosystem functions, namely carbon transformations, nutrient cycling, soil structure maintenance and population regulation, respectively, which, through a variety of soil-based delivery processes, generate and sustain soil health (Kibblewhite et al. Citation2008; Barrios et al. Citation2012). Trees in agricultural landscapes can provide more favorable habitats for soil biodiversity, through microclimate buffering and continuous supply of organic matter inputs, thus fostering ‘hot spots’ of biological activity responsible for many ecological functions underpinning soil health (Pauli et al. Citation2010; Ushio et al. Citation2010; Diedhiou-Sall et al. Citation2013; Kamau et al. Citation2017). Increased tree cover often, but not always, also reduces pest pressures and improves pollination services (Ricketts et al. Citation2004; Pumariño et al. Citation2015).
Agricultural landscapes, where agroforestry cover is significant, represent intermediate levels of complexity presumably most effective for the conservation of biodiversity and ecosystem services, yet also contributing to more resilient rural livelihoods (Schroth et al. Citation2004; Tscharntke et al. Citation2012b; Prabhu et al. Citation2015; Balvanera et al. Citation2016). In this paper, the contribution of trees to the conservation of biodiversity and ecological functions underpinning selected ecosystem services was evaluated across dominant land uses in agricultural landscapes representing different levels of tree cover ().
Figure 1. Approximate tree cover of dominant land uses in agricultural landscapes studied where each symbol represents a study site.
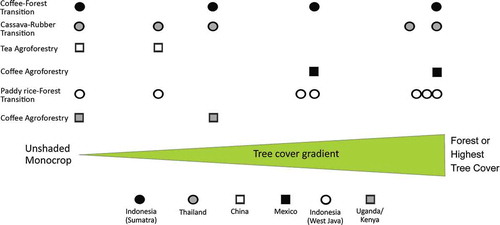
The objectives were to (1) assess the effects of changes in tree cover (e.g. presence/absence, density, diversity and functional traits) on belowground and aboveground biodiversity; (2) assess the effect of increasing tree cover on ecological functions of belowground organisms underpinning soil health and crop yield; (3) assess the effect of increasing tree cover on ecological functions of aboveground organisms underpinning habitat provision, pollination and biological control services and (4) examine the value of agroforestry as a biodiversity-based intervention contributing to more sustainable trajectories of agricultural intensification.
2. Approach and methods
We examined six study sites that investigated the impact of changing tree cover on biodiversity and ecosystem services in different agricultural landscapes (). We selected study sites occurring in areas described as biodiversity ‘hotspots’ (Myers et al. Citation2000) where agricultural expansion is threatening natural capital.
Table 1. General characteristics of study sites.
Agroecosystems studied included coffeeof differences in soil nutritional agroforestry, tea agroforestry and three agricultural landscapes in transition that resulted in tree presence/absence or tree cover gradients which presumably influence the belowground or aboveground biodiversity and associated ecosystem functions and services. Shade coffee agroforestry constitutes an intermediate intensification stage, and hence of tree cover, between the native forest and coffee monoculture that was a common feature in Mexico and Kenya–Uganda study sites (). The tea agroforestry included the N-fixing tree, Alnus nepalensis D. Don, as companion tree to the otherwise monoculture tea plantations in southwestern China. The transitions from forest to unshaded coffee monocrop in Indonesia (Sumatra), from cassava monocrop to tree-based agroecosystems involving rubber in Thailand and from forest to paddy rice agriculture in Indonesia (West Java) generate decreasing and increasing tree cover gradients scenarios (). All case studies provide different socio-ecological contexts in which to examine the effect of tree cover on biodiversity trends and selected ecosystem services aiming to contribute to generalizations on the role of agroforestry as a biodiversity-based intervention for ecological intensification of agriculture. Nevertheless, we do not address socioeconomic dimensions in any detail. While farmers and the number of factors that influence their decisions are inherent to any agricultural intervention, we recognize that any principles proposed for agroecosystem management in addition to scientific validity must also be relevant and feasible. In order to compare different agroecosystems studied on a common basis, percent change in mean biodiversity measure or ecological process was calculated using the following formulae: [(monocrop − forest)/forest] × 100 and [(agroforestry − forest)/forest)] × 100. The forest was considered the baseline as it is the natural system and other agroecosystems were considered relative to it. In case forest was not part of land uses studied in a particular agricultural landscape, the land use of highest tree cover was used as a reference.
3. Results
3.1. Case studies
Land uses evaluated included dominant land-cover scenarios useful to comparatively assess the effects of trees on both belowground and aboveground biodiversity (). While unshaded monocrop/shaded agroforestry allowed the assessment of shade-tree absence/presence scenarios, respectively, the monocrop to forest/mature plantation transitions allowed examining a more gradual variation in tree cover. When considering natural forest as the baseline, and other systems relative to it, negative percent values are expected resulting from a loss in ecological function relative to forest when converted to agriculture (). Biodiversity-based interventions such as agroforestry would presumably cause less negative effects than monoculture because they can generate agricultural production while maintaining more biodiversity and ecological functions. Further, positive values would highlight additional virtues of agroforestry.
Table 2. Effects of changes in tree cover on biodiversity and biological processes studied in dominant land uses across six agricultural landscapes (designs with sample sizes are summarized in ).
3.1.1. Linking trees, belowground biodiversity and ecosystem services
The perennial nature of trees in agroforestry systems profoundly impacts microclimate and soil properties. By positively influencing the abundance, diversity and activity of soil biota, trees in agroforestry systems contribute to soil health and functional resilience (Barrios et al. Citation2012). In this section, we examine the impact of tree cover in coffee monocrop – forest transition on native earthworm populations influencing soil physical properties and water dynamics; the influence of canopy closure on the abundance and diversity of soil macrofauna in a cassava monocrop/agroforestry/rubber plantation transition; and the contribution of N-fixing trees to soil microbial biomass, diversity and crop yield in tea agroforestry.
3.1.1.1. Coffee agroforestry transition in Indonesia (Sumatra)
Forest conversion to monoculture coffee systems in Sumatra (Indonesia) significantly affected the abundance and diversity of native earthworm populations and influenced soil physical properties (Hairiah et al. Citation2006). Four relatively large-bodied native earthworms were lost upon forest conversion to coffee agriculture (Figure S1, ): (1) Metaphire sp. 1 (epigeic = feed on and live in the litter layer), (2) Metaphire sp. 2 (epigeic), (3) Metaphire javanica group (anecic = feed on litter layer and mix it with soil, and make vertical burrows) and (4) Megascolex sp. (endogeic = feed on and live in the soil) were completely replaced by six exotic and smaller bodied earthworms, dominated by the invasive exotic Pontoscolex corethrurus (endogeic) (Dewi et al. Citation2006; Dewi and Senge Citation2015).
The lower mean body size, and dominance of endogeic earthworms, in agroecosystems compared to natural forest resulted in 71–76% lower soil macroporosity (). The conversion of forest to agriculture also resulted in 71% and 43% reduction in annual litterfall and standing litter respectively in coffee monocrops which compared to lower reductions in coffee agroforestry (e.g. 41% and 29%) (). These observations suggest that encouraging land uses and management that maintain litter inputs and conserve native earthworm vertical burrowing activity could contribute to positive changes in soil macroporosity that can increase infiltration and reduce run-off and soil erosion (Hairiah et al. Citation2006). Nevertheless, there was some evidence that organic inputs from Gliricidia sepium shade trees may generate negative effects on some soil organisms and their contribution to soil function. Hence, further attention should be devoted to negative interactions resulting from agroforestry trees and future studies should explore how common G. sepium litter toxicity is to earthworms.
3.1.1.2. Cassava monocrop transition to rubber plantation in Thailand
The shift from cassava to young rubber plantations in Thailand resulted in an initial sharp decrease in the density and biomass of the soil macrofauna. However, after canopy closure, when the habitat functions provided by trees become more prevalent, important changes were observed in the soil macrofauna community structure with an overall increase in biomass accompanied by a decrease in ant and increase in earthworms and termite populations (Figure S2, ). The species richness of soil macrofauna was highest in the older rubber plantations. There was an important turnover of soil macrofauna functional groups along the rubber chronosequence caused by increased dominance of earthworms and presence of predators in the older plantations (8–12 → 23–25 years).
This study highlights that the conversion of annual monocrops to perennial monocrops generates system changes contributing to higher diversity and evenness (but lower abundance and higher biomass) in the soil macrofauna community, with a significantly greater earthworm population (p < 0.05) which is often associated with improved soil health (Pauli et al. Citation2011; Legname et al. Citation2012). Therefore, in this ecological context characterized by an adverse edaphic soil condition and a long dry season (Chambon et al. Citation2016), the shift from an annual cash crop such as cassava to rubber could bring positive impacts on soil biodiversity and associated ecological functions through continuity of organic inputs, reduced tillage, lower input of mineral fertilizers and maintenance of soil moisture.
3.1.1.3. Tea agroforestry in China
Tea production in China increased by close to 50% when the tea was grown with A. nepalensis (Figure S3, ). This finding is in line with past work relating to the positive effects of A. nepalensis on crop production (Vanlalhluna and Sahoo Citation2009; Das et al. Citation2010). These changes in crop production came about despite no significant changes in soil chemical characteristics (Mortimer et al.Citation2015).
Although no significant change was detected in the overall soil microbial diversity between tea monoculture and agroforestry systems, significant increases of 21–23% were recorded in the microbial biomass of various functional groups in the soils associated with A. nepalensis (). The Actinomycetes biomass was significantly greater in the agroforestry soils, which concurs with the findings of Golinska and Dahm (Citation2011) who reported an increase in the biomass of Actinomycetes in the soils associated with Alnus glutinosa. Furthermore, ectomycorrhizal biomass, as well as that of gram positive and negative bacteria, was found to be greater in the soils surrounding A. nepalensis. These findings are consistent with past studies showing the same trend of increased fungal and bacterial biomass in soils surrounding Alder trees of various species (Selmants et al. Citation2005; Prescott and Grayston Citation2013; Šnajdr et al. Citation2013).
This study clearly showed that the incorporation of A. nepalensis into monoculture tea plantations resulted in the improved productivity of the tea plantation (Mortimer et al. Citation2015). The lack of differences in soil nutritional status under both monoculture and agroforestry plots suggests that differences observed in tea production may be biologically driven by increased microbial biomass in the soils under agroforestry. A clear increase in the presence of certain functional groups known to aid plant growth, nutrient cycling and disease resistance provide evidence for this.
3.1.2. Linking trees, aboveground biodiversity and ecosystem services
Agroforestry systems conserve plants, arthropods and vertebrates that have been linked to the delivery of habitat as well as important regulating services, such as pollination and pest control (Perfecto et al. Citation2004; Klein et al. Citation2008; Tscharntke et al. Citation2011). In this section, we examine the effect of shade-coffee agroforestry on tree species diversity and community composition; the effect of a tree cover gradient on insect pollinator species richness and abundance; and the effects of shade, landscape level tree cover and elevation on various pests in coffee agroforestry systems.
3.1.2.1. Coffee agroforestry in Mexico
While at the farm level (i.e. alpha diversity), coffee agroforests conserve lower tree diversity than forests, at the landscape level (i.e. gamma diversity) when the entire ensemble of coffee agroforestry farms is considered, forests and agroforests conserve comparable levels of tree richness (Valencia et al. Citation2014). However, stark differences were found between agroforests and surrounding forests when tree community composition considered trees of conservation concern status (i.e. listed as critically endangered, endangered or vulnerable in the IUCN Red List of Threatened Species in IUCN Citation2013; or The Red List of Mexican Cloud Forest Trees in Gonzalez-Espinosa et al. Citation2011) or succession traits (e.g. pioneer, intermediate and late stages of succession). The relative abundance of trees of conservation concern decreased by 60% in coffee agroforestry compared to the native forest (Figure S4, ). Furthermore, agroforests showed close to 150% increase in relative abundance of pioneer trees, in particular native Inga spp. trees that increased by 390% ().
The uncovered differences in floristic patterns between agroforests and surrounding forests were explained primarily by farmers’ tree preferences and selection criteria rather than as an unintended by-product of management (Valencia et al. Citation2015). Farmers believe that agroforests dominated by Inga spp. enhance ecosystem services such as soil fertility and production of higher yields; however, some studies have shown that this is not always the case (Romero-Alvarado et al. Citation2002; Peeters et al. Citation2003). Recent findings show that increasing the proportion of Inga spp. trees has a significant negative impact on the proportions of trees of late-successional stage and threatened species status that may be conserved in agroforestry systems (Valencia et al. Citation2016).
3.1.2.2. Forest–agriculture transition in Indonesia (West Java)
The transition from forests to habitats with lower tree cover, as a result of increasing human modification, significantly affected the species richness, abundance and functionality of insect pollinators (Figure S5). A total of 453 insect pollinator individuals from 21 families were collected in pan traps that included eight species of bees, seven of wasps, seven of beetles, four of moths/butterflies and four of flies (Muhamad Citation2013). Of them, all eight bee species, three wasps, five beetles, one butterfly and all four flies are known as efficient insect crop pollinators. Species richness and abundance were significantly different among landscape element types, except those of less efficient insect crop pollinators.
Richness of total insect pollinators declined in monocrops (rice paddy fields) by 14% compared to natural forests, while it remained unchanged under mixed-tree (MT) agroforestry (). A decrease in richness of efficient insect pollinators close to 40% was observed under monocrops, but this decrease was only about 15% under MT agroforestry. Richness of less efficient pollinators, on the other hand, did not change under monocrops but slightly increased under MT agroforestry. Richness values for other land uses were intermediate between rice paddy fields and natural forests.
The abundance of total insect pollinators declined in monocrops by 20% compared to natural forests, and that of efficient pollinators by 23%, while increasing the abundance of less-efficient insect pollinators by 100% (). In contrast, MT agroforestry was colonized by 93% of crop pollinators found in the remnant forest and supported the highest richness of insect pollinators together with the natural forest. While MT agroforestry maintained 85% of the efficient insect pollinators found in the natural forest, an increase in abundance of less-efficient pollinators was also observed (). The abundance values for other land uses were intermediate between bamboo-dominated agroforestry and MT agroforestry. The lowest abundance values found in bamboo-dominated agroforestry could be related to bamboo species being largely wind pollinated and usually flowering at very long intervals of many years and decades (Janzen Citation1976).
Variations in pollinator richness and abundance observed across this forest–agriculture transition study are consistent with patterns reported by Ricketts et al. (Citation2008) in their review of landscape effects on crop pollination services. Increased vegetation cover has been identified as an important factor fostering greater species diversity of insect pollinators in tropical landscapes with fragmented remnant forest (Donaldson et al. Citation2002; Potts et al. Citation2010). Furthermore, differences in vegetation structure could also affect the abundance and diversity of insect pollinators by influencing the abundance and distribution of flower resources, availability of nesting sites and light intensity levels (Klein et al. Citation2007, Citation2008). These findings emphasize the importance of human-modified landscapes, particularly MT agroforestry, in maintaining a high diversity and abundance of efficient insect crop pollinators. However, the protection of remnant forests as a source of crop pollinator diversity needs to be a conservation priority. The enhancement of agroforestry buffer zones around forest fragments stands out as a key feature of appropriate landscape design and habitat management. This is largely because this strategy would maintain a diversity of plant species of different life forms and phenology that can provide an abundant source of floral resources expected to maintain and attract more insect pollinators.
3.1.2.3. Coffee agroforestry in east Africa (eastern Uganda and western Kenya)
The effect of shade differed between pest species (Figure S6). In Uganda, the coffee berry borer was more common in sun-exposed plantations, whereas the white stem borer was more common at shaded sites (Jonsson et al. Citation2015). The infestation of the coffee berry borer was on average about 45% higher in sun-exposed plantations compared to shaded ones (). The effect of shade on the white stem borer depended on altitude, with the differences between shade levels being most pronounced in plantations at lower altitudes. Stem borer infestations were on average 69% lower in plantations with full or moderate shade at low altitudes, compared to sun-exposed plantations (). The negative effect of agroforestry on coffee berry borer infestations may be due to both top-down and bottom-up factors. Natural enemies of coffee berry borers such as ants, parasitoids (Perfecto et al. Citation1996) and birds (Karp et al. Citation2013) are often more common in agroforestry systems and therefore biological control may be more effective there. The coffee berry borer populations could also have developed at a slower rate in shaded plantations due to lower temperatures (Jaramillo et al. Citation2009) and females may have had difficulties locating suitable berries due to altered biochemical composition (Jaramillo et al. Citation2013). Less is known about variables influencing white stem borers, but it appears likely that the positive effects of shade on this pest were due to improved microhabitat conditions rather than to predator attack rates as predators are usually more common under agroforestry practices.
In Kenya, results showed that the effect of shade on lacebugs depended on the amount of trees present in the surrounding landscape. More lacebugs were present in shaded plantations, but only in landscapes with a low tree-cover. The abundance of lacebugs was reduced by 86% in high shade sites compared to those with low shade and low tree cover (). This work shows that the impact of agroforestry on pest regulation in coffee is highly context dependent; it depends on the identity of the most important pests, altitude and landscape composition ().
Figure 2. Diagram showing whether including shade trees into coffee plantations is likely to reduce pest problems (Yes), if it is likely to have no effect (Doesn’t matter) or if it is likely to increase pest problems (No) depending on pest identity, altitude and tree cover in the surrounding landscape.
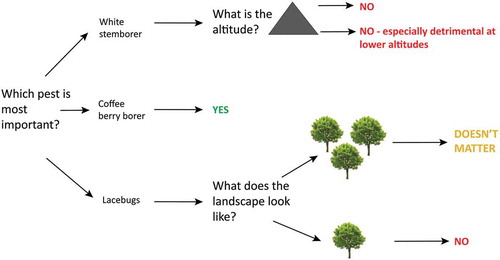
Coffee berry borers, white stem borers and lacebugs do co-occur in some areas of Africa, but often one of the species is the one causing most damage in a particular plantation. Coffee berry borers and white stem borers are currently primarily a concern below about 1700 m a.s.l. (Rutherford and Phiri Citation2006), whereas lacebugs thrive also at higher elevations. White stem borers are restricted to sub-Saharan Africa, whereas the coffee berry borer has spread through trade and is common in most coffee-growing regions of the world. White stem borers are especially serious in plantations established on poor soils (Rutherford and Phiri Citation2006). If coffee berry borers are the primary concern, then adding shade trees to a coffee plantation is likely to be an effective way of reducing pest problems. In contrast, if white stem borers are the key concern, then adding shade trees is likely to be detrimental, especially at lower altitudes. Finally, if lacebugs are the most destructive pests, then shade trees may also be detrimental, but only when coffee-growing areas are located in simplified agricultural landscapes with few trees.
4. Discussion
4.1. Agroforestry as a promising biodiversity-based agricultural intervention
Case studies highlighted in this paper provide examples of agricultural landscapes where tree-based land management practices contribute to the conservation of biodiversity and ecosystem services including agricultural production. Given the contrasting tree-based agricultural systems, organisms and ecosystem services considered, comparisons and general conclusions will be difficult to make and should be taken with caution. Coffee agroforestry landscapes were most common and the focus of three of the six study sites. The socio-ecological contexts in which they occurred, however, were contrasting and included an agricultural expansion frontier in Indonesia (Sumatra), smallholder agriculture inside a biosphere reserve in Mexico and smallholder farmer settlements in Kenya and Uganda. The cassava–agroforestry–rubber plantation transition in Thailand is an increasingly common agricultural practice where cassava, usually representing an attractive cash crop for bioethanol production, is replaced by rubber with higher market prices and hence greater profit. The introduction of N-fixing trees in tea agroforestry landscapes in China represents an increasingly common practice aiming at reducing fertilizer inputs and their contribution to high production costs and environmental pollution. The forest–agriculture transition in Indonesia (West Java) provides a good example of the impact of land-use intensification gradients on insect pollinators and the potential of agroforestry to conserve efficient insect crop pollinator species richness and abundance.
Protecting refuges and reproduction habitats for wild plants and animals in agroecosystems contributes to the in situ conservation of biological and genetic diversity that underpins regulation and production ecosystem functions (De Groot et al. Citation2002). Case studies, however, highlight the challenge of conserving native forest biodiversity in agroforestry systems (agroforestry-habitat hypothesis – Schroth et al. Citation2004). The Indonesia (Sumatra) study found the loss of native forest earthworms after conversion of natural forest to coffee monocrops or agroforestry practices which encouraged G. sepium as shade trees. Similarly, the Mexico case study found lower tree species diversity than the native forest when the agroforestry practice encouraged a single species of shade tree, Inga spp., in coffee agroforestry systems. These case studies highlight that changes in native biodiversity are a result of both the intended and unintended consequences of farmers’ management. For example, in the Mexico study site, farmers’ tree preferences and selection criteria intentionally modified tree species community composition in agroforests causing it to diverge from that found in natural forests. In Indonesia (Sumatra), the unintended results of removing tree cover (e.g. coffee monocrops) and of lower tree abundance and diversity in coffee agroforestry on the soil condition caused a reduction in the diversity and body size of earthworms. Significant replacement of native earthworm species by the invasive earthworm P. corethrurus has also been reported in the Colombian Andes in soils under Indigofera constricta L. tree fallows which, like G. sepium, produce fast-decomposing organic resources of high N content (Barrios et al. Citation2005). More recently, Marichal et al. (Citation2010) showed that P. corethrurus density co-varied with soil N content in a study of agricultural landscapes in the Amazon. These results call attention to the need of identifying agroforestry systems which encourage tree cover diversity to reduce negative effects on biodiversity conservation and prevent creating environmental conditions that may favor invasion by exotic species.
Although the extent to which some agroforestry systems may conserve species richness and community composition similar to that in forests is debatable (Philpott and Dietsch Citation2003; Rappole et al. Citation2003; Valencia et al. Citation2016), an extensive body of literature has shown that increased tree cover improves the quality of the agricultural matrix, thus supporting metapopulations at the landscape level (Perfecto and Vandermeer Citation2002, Citation2008; Vandermeer and Perfecto Citation2007) and the ecosystem services that they provide. The Indonesia (West Java) case study is an example of the role that agroforestry systems play as buffer zones around forest fragments thus supporting the agroforestry-matrix hypothesis (Schroth et al. Citation2004). This case study highlights the importance of tree cover in agricultural plots, particularly MT agroforests, in supporting remnant forests in maintaining a high abundance and diversity of bees and other crop pollinators. Furthermore, a global study by Maestre et al. (Citation2012) showed that preservation of plant biodiversity is crucial to maintaining multiple ecosystem functions and also to buffer negative effects of climate change. More recently, Jing et al. (Citation2015) reported that the combined effects of above- and belowground biodiversity contributed to 45% of the variation in ecosystem multifunctionality.
Case studies also highlighted the ecologically restorative role of including trees in agricultural plots compared to tree-less monocultures. The Thailand case study shows that the transition from annual crop fields to tree plantations improves the capacity for restoring soil macrofauna diversity over time, and presumably their contribution to soil-mediated ecological functions. Similarly, rubber plantations in the Orinoco river basin also harbored more abundant and diverse soil macrofauna communities than those in annual crops (Lavelle et al. Citation2014). The China case study, on the other hand, showed that the inclusion of N-fixing trees in tea plantations, as compared to tea monocultures, was associated with increases in tea production, possibly related with increases in microbial biomass of various functional groups rather than increases in microbial diversity. Nevertheless, it is important to note that the effects of agroforestry practices are not always positive and their effects on biodiversity and ecosystem services can be highly context dependent. While the Indonesia (Sumatra) case study showed that organic input from certain agroforestry tree species may have negative effects on soil animals and functions, agroforestry practices used in Thailand resulted in early reduction in soil macrofauna abundance and biomass, and the Uganda–Kenya case study showed that the effects of shade trees on pest problems can be negative depending on landscape context, altitude and the pest species that are of strongest concern in an area. All these issues need to be considered when designing agroforestry systems that minimize trade-offs and maximize synergies and complementarities that aim at biodiversity conservation and the delivery of multiple ecosystem services.
4.2. Challenges and opportunities to an ecological intensification of agriculture
The high levels of biodiversity and inherent ecological complexity of terrestrial ecosystems, particularly in the tropics, make the study of linkages between biodiversity and ecosystem functions/services supporting biodiversity-based agriculture difficult (Swift et al. Citation2004; Barrios Citation2007). Recent methodological reviews highlight the importance of using gradients of agricultural intensification as living laboratories at which biodiversity and ecosystem services are manifested at multiple scales (Balvanera et al. Citation2016). Furthermore, emphasis on agricultural landscapes where dominant practices maintain relatively high levels of aboveground and belowground biodiversity (e.g. agroforestry) has provided simpler but realistic scenarios to study linkages between biodiversity and the provision of ecosystem services (Perfecto et al. Citation1996; Steffan-Dewenter et al. Citation2007; Fonte et al. Citation2010; Tscharntke et al. Citation2011; Rousseau et al. Citation2013; Balvanera et al. Citation2016; Kamau et al. Citation2017).
The strong linkage between aboveground biodiversity (vegetation/crops) and belowground biodiversity (soil organisms) highlights the potential of tree cover management to influence the provision of soil-based ecosystem services in agricultural landscapes (Wardle et al. Citation2004; Diaz et al. Citation2007; De Deyn et al. Citation2008; De Bello et al. Citation2010). Research addressing key questions on the link between plant–soil feedbacks and the provision of ecosystem services (van der Putten et al. Citation2013; Bardgett and van der Putten Citation2014) should be conducted at scales relevant to management and this can be facilitated by integrating local and scientific spatial variability information on ‘hot spots’ of soil biological activity (Barrios Citation2007; Pauli et al. Citation2010, Citation2012; Barrios et al. Citation2012; Kamau et al. Citation2017). Furthermore, focusing on functions that are relatively specific, such as the roles of ecosystem engineers or specific nutrient transformations, would help with achieving more reliable assessments of the linkages between biodiversity and ecosystem services (Giller et al. Citation2005; Balvanera et al. Citation2016). Nevertheless, this focused approach should be integrated as part of an interdisciplinary framework where the continuous interaction between functional diversity components and priorities of social actors define land-use decisions and consequently the provision of ecosystem services (Díaz et al. Citation2011; Jackson et al. Citation2012).
After the conversion of natural ecosystems to agriculture and subsequent intensification, there is gradual replacement of ecological functions (e.g. nutrient mineralization, biological control of pests) by external inputs such as agrochemicals. This trend leads to a reduction in the capacity of agroecosystems for self-regulation, greater reliance on external inputs and thus greater vulnerability to environmental and market changes (Barrios et al. Citation2015). There is growing interest in agroecosystems characterized by greater internal resource-use efficiency, less reliance on external inputs, and that can sustain a suitable balance between productivity and the provisioning of other ecosystem services (Brussaard et al. Citation2010; Dore et al. Citation2011). The positive impact of agroforestry, compared to conventional agriculture, on biodiversity belowground and aboveground (e.g. N-fixing organisms, ecosystem engineers, pollinators, biological control agents) contributes to sustaining a greater diversity of ecological functions. Furthermore, agricultural practices that foster enhanced functional diversity increase the potential for multiple benefits but it is important to recognize the need to understand the interactions among multiple ecosystem services for greater resilience benefits and adaptation capacity to climate change.
The adaptation of ecological concepts and principles to the design and management of agroecosystems and food systems, defined as agroecology, is a key strategy that can contribute to addressing agricultural sustainability concerns (Altieri Citation1995; Gliessman Citation2007). Agroforestry is a good example of ‘agroecology in practice’ that has great potential to support a biodiversity-based and multifunctional agriculture that successfully addresses the challenge of optimizing crop productivity while conserving biodiversity and maintaining the provision of other ecosystem services (Prabhu et al. Citation2015). In order to realize this potential at scale, however, Coe et al. (Citation2014) highlight that we need to better understand (1) how fine-scale variation in social, economic and ecological context creates a need for local adaptation of practices; (2) how to develop effective service delivery mechanisms, markets and institutional contexts in addition to technologies and (3) how to foster adaptive innovation processes that supports co-learning amongst research, development and private sector actors. Moreover, Tomich et al. (Citation2011) highlight the prospective role of agroecology in fostering the technological and institutional innovations, as well as the adaptive management and social learning, required to face global change challenges in the twenty-first century such as increasing global food production and resilience of agriculture and food systems in the context of increased environmental variability and socioeconomic vulnerability.
5. Conclusions
Increasing tree cover in agricultural landscapes can support plant and invertebrate biodiversity and significantly improve ecosystem functions that underpin ecosystem services based on the species/taxa studied here. While the results highlight a general pattern of trees promoting an increase in beneficial organisms, negative effects were also identified. This was particularly the case in agroforestry systems where single shade tree species were encouraged to replace a diverse tree cover or when different pests respond differently to tree shade level and altitude. The restricted number of studies examined which related increases in abundance and diversity with functional attributes, however, limited the wider attribution of functional benefits that trees may nurture. Agroforestry practices show great potential as biodiversity-based interventions contributing to an ecological intensification of agriculture. Nevertheless, the importance of matching tree-based management options to context for minimizing trade-offs, and maximizing synergies and complementarities, cannot be overstated. Developing understanding through knowledge sharing that encourages the blending of local and scientific knowledge could significantly facilitate this process by fostering the relevance, credibility and legitimacy required for the wide adoption of promising management options aiming at the sustainable management of lands for multiple ecosystem services and livelihood benefits.
Barrios_et_al_2017_SupplementaryData.pdf
Download PDF (1 MB)Acknowledgments
This paper provides a synthesis of the session entitled ‘Biodiversity and agroforested habitats’ held at the 3rd World Congress of Agroforestry in New Delhi, India from 10 to 14 February 2014. Two additional contributions were invited (PM, SO) given they had been originally selected but were later unable to participate in the session. Helpful comments provided by anonymous reviewers and statistical advice by Richard Coe contributed to improve the paper and are highly appreciated.
Disclosure statement
No potential conflict of interest was reported by the authors.
Supplemental data
Supplemental data for this article can be accessed here.
Additional information
Funding
References
- Altieri MA. 1995. Agroecology: the science of sustainable agriculture. 2nd ed. London (UK): Intermediate Technology Publications (ITP).
- Anderson JM, Ingram JSI. 1993. Tropical soil biology and fertility: a handbook of methods. 2nd ed. Wallingford: CAB International.
- Balvanera P, Pfisterer AB, Buchmann N, He J-S, Nakashizuka T, Raffaelli D, Schmid B. 2006. Quantifying the evidence for biodiversity effects on ecosystem functioning and services. Ecol Lett. 9:1146–1156.
- Balvanera P, Quijas S, Martín-López B, Barrios E, Dee L, Isbell F, Durance I, White P, Blanchard R, De Groot R. 2016. The links between biodiversity and ecosystem services. In: Potschin M, Haines-Young R, Fish R, Turner RK, editors. Routledge handbook of ecosystem services. London (UK): Routledge, Taylor & Francis Group; p. 45–49.
- Bardgett RD, van der Putten WH. 2014. Belowground biodiversity and ecosystem functioning. Nature. 515:505–511.
- Barrios E. 2007. Soil biota, ecosystem services and land productivity. Ecol Econ. 64:269–285.
- Barrios E, Cobo JG, Rao IM, Thomas RJ, Amezquita E, Jimenez JJ, Rondon MA. 2005. Fallow management for soil fertility recovery in tropical Andean agroecosystems in Colombia. Agric Ecosyst Envir. 110:29–42.
- Barrios E, Shepherd K, Sinclair F. 2015. Soil health and agricultural sustainability: the role of soil biota. In: FAO, editor. Agroecology for food security and nutrition. Rome (Italy): Proceedings of the FAO International Symposium; p. 104–122.
- Barrios E, Sileshi GW, Shepherd KD, Sinclair F. 2012. Agroforestry and soil health: linking trees, soil biota and ecosystem services. In: Wall DH, Bardgett RD, Behan- Pelletier V, Herrick JE, Jones TH, Ritz K, Six J, Strong DR, Van der Putten WH, editors. Soil ecology and ecosystem services. Oxford (UK): Oxford University Press; p. 315–330.
- Beare MH, Coleman DC, Crossley DA Jr, Hendrix PF, Odum EP. 1995. A hierarchical approach to evaluating the significance of soil biodiversity to biogeochemical cycling. Plant Soil. 170:5–22.
- Beer J, Muschler R, Kass D, Somarriba E. 1998. Shade management in coffee and cacao plantations. Agroforestry Syst. 38:139–164.
- Bhagwat S, Willis KJ, Birks HJB, Whittaker RJ. 2008. Agroforestry: a refuge for tropical biodiversity? Trends in Ecol Evol. 23:261–267.
- Bligh EG, Dyer WJ. 1959. A rapid method of total lipid extraction and purification. Can J Biochem Physiol. 37:911–917.
- Bommarco R, Kleijn D, Potts SG. 2013. Ecological intensification: harnessing ecosystem services for food security. Trends Ecol Evol. 28:230–238.
- Brussaard L, Caron P, Campbell B, Lipper L, Mainka S, Rabbinge R, Babin D, Pulleman M. 2010. Reconciling biodiversity conservation and food security: scientific challenges for a new agriculture. Curr Opin Environ Sustain. 2:34–42.
- Chambon B, Ruf F, Kongmanee C, Angthong S. 2016. Can the cocoa cycle model explain the continuous growth of the rubber (Hevea brasiliensis) sector for more than a century in Thailand? J Rural Stud. 44:87–197.
- Coe R, Sinclair FL, Barrios E. 2014. Scaling up agroforestry requires research ‘in’ rather than ‘for’ development. Curr Opin Environ Sustain. 6:73–77.
- Dafni A, Kevan PG, Husband BC, editors. 2005. Practical pollination ecology. Cambridge (Canada): Enviroquest.
- Das A, Tomar J, Ramesh T, Munda G, Ghosh P, Patel D. 2010. Productivity and economics of lowland rice as influenced by incorporation of N-fixing tree biomass in mid-altitude subtropical Meghalaya, North East India. Nutr Cycl Agroecosyst. 87:9–19.
- De Bello F, Lavorel S, Díaz S, Harrington R, Cornelissen JHC, Bardgett RD, Berg MP, Cipriotti P, Feld CK, Hering D, et al. 2010. Towards an assessment of multiple ecosystem processes and services via functional traits. Biodiv Conserv. 19:2873–2893.
- De Deyn GB, Cornelissen JHC, Bardgett RD. 2008. Plant functional traits and soil carbon sequestration in contrasting biomes. Ecol Lett. 11:516–531.
- De Groot RS, Wilson MA, Boumans RMJ. 2002. A typology for the classification, description and valuation of ecosystem functions, goods and services. Ecol Econ. 41:393–408.
- Dewi WS, Hairiah K, Yanuwiyadi B, Suprayogo D. 2006. Can agroforestry systems maintain earthworm diversity after conversion of forest to agricultural land? Agrivita. 28:198–220.
- Dewi WS, Senge M. 2015. Earthworm diversity and ecosystem services under threat. Rev Agric Sci. 3:25–35.
- Diaz S, Lavorel S, De Bello F, Quétier F, Grigulis K, Robson TM. 2007. Incorporating plant functional diversity effects in ecosystem service assessments. Pnas. 104:20684–20689.
- Díaz S, Quétier F, Cáceres DM, Trainor SF, Pérez-Harguindeguy N, Bret-Harte MS, Finegan B, Peña-Claros M, Poorter L. 2011. Linking functional diversity and social actor strategies in a framework for interdisciplinary analysis of nature’s benefits to society. Pnas. 108:895–902.
- Diedhiou-Sall S, Dossa EL, Diedhiou I, Badiane AN, Assigbetsé KB, Ndiaye Samba SA, Khouma M, Sène M, Dick R. 2013. Microbiology and macrofaunal activity in soil beneath shrub canopies during residue decomposition in agroecosystems of the Sahel. Soil Sci Soc Am J. 77:501–511.
- Donaldson J, Nänni I, Zachariades C, Kemper J. 2002. Effects of habitat fragmentation on pollinator diversity and plant reproductive success in Renosterveld shrublands of South Africa. Conserv Biol. 5:1267–1276.
- Dore T, Makowski D, Malezieux E, Munier-Jolain N, Tchamitchian M, Tittonell P. 2011. Facing up to the paradigm of ecological intensification in agronomy: revisiting methods, concepts and knowledge. Eur J Agron. 34:197–210.
- Foley JA, DeFries R, Asner GP, Barford C, Bonan G, Carpenter SR, Chapin FS, Coe MT, Daily GC, Gibbs HK, et al. 2005. Global consequences of land use. Science. 305:570–574.
- Fonte SJ, Barrios E, Six J. 2010. Earthworms, soil fertility and aggregate-associated soil organic matter dynamics in the Quesungual agroforestry system. Geoderma. 155:320–328.
- Food and Agriculture Organization (FAO). 2007. The state of food and agriculture 2007. Paying farmers for environmental services. Rome: FAO.
- Frostegåd Å, BåÅh E. 1996. The use of phospholipid fatty acid analysis to estimate bacterial and fungal biomass in soil. Biol Fertil Soils. 22:59–65.
- Giller KE, Bignell DE, Lavelle P, Swift MJ, Barrios E, Moreira F, Van Noordwijk M, Barois I, Karanja N, Huising J. 2005. Soil biodiversity in rapidly changing tropical landscapes: scaling down and scaling up. In: Bardgett RD, Usher MB, Hopkins DW, editors. Biological diversity and function in soils. Cambridge (UK): Cambridge University Press; p. 295–318.
- Gliessman SR. 2007. Agroecology: the ecology of sustainable food systems. Boca Raton (FL): CRC Press; p. 384.
- Golinska P, Dahm H. 2011. Occurrence of actinomycetes in forest soil. Dendrobiology. 66:3–13.
- Gonzalez-Espinosa M, Meave J, Lorea-Hernandez F, Ibarra-Manriquez G, Newton A, editors. 2011. The red list of Mexican cloud forest trees. Fauna & Flora International. [accessed 2012 Apr 24]. http://www.globaltrees.org/rl_mexican_cloudforest.htm.
- Hairiah K, Sitompul SM, Van Noordwijk M, Palm CA. 2001. Methods for sampling carbon stocks above and below ground. ASB_LN 4B. In: VanNoordwijk M, Williams SE, Verbist B, editors. Towards integrated natural resource management in forest margins of the humid tropics: local action and global concerns. ASB-Lecture Notes 1–12. Bogor (Indonesia): International Centre for Research in Agroforestry (ICRAF). http://www.icraf.cgiar.org/sea/Training/Materials/ASB-TM/ASB-ICRAFSEA-LN.htm.
- Hairiah K, Sulistyani H, Suprayogo D, Widianto, Purnomosidhi P, Widodo RH, Van Noordwijk M. 2006. Litter layer residence time in forest and coffee agroforestry systems in Sumberjaya, West Lampung. Forest Ecol Mgmt. 224:45–57.
- IUCN. 2013. IUCN red list of threatened species. Version 2013.1. http://www.iucnredlist.org/search.
- Jackson LE, Pulleman MM, Brussaard L, Bawa KW, Brown GG, Cardoso IM, De Ruiter PC, Garcia-Barrios L, Hollander AD, Lavelle P, et al. 2012. Social-ecological and regional adaptation of agrobiodiversity management across a global set of research regions. Global Environ Change. 22:623–639.
- Janzen DH. 1976. Why bamboos wait so long to flower. Ann Rev Ecol Syst. 7:347–391.
- Jaramillo J, Chabi-Olaye A, Kamonjo C, Jaramillo A, Vega FE, Poehling H-M, Borgemeister C. 2009. Thermal tolerance of the Coffee berry borer Hypothenemus hampei: predictions of climate change impact on a tropical insect pest. PloS One. 4:e6487.
- Jaramillo J, Setamou M, Muchugu E, Chabi-Olaye A, Jaramillo A, Mukabana J, Maina J, Gatharan S, Borgemeister C. 2013. Climate change or urbanization? Impacts on a traditional coffee production system in East Africa over the last 80 years. PloS One. 8:e51815.
- Jha S, Bacon CM, Philpott SM, Ernesto Méndez VE, Läderach P, Rice RA. 2014. Shade coffee: update on a disappearing refuge for biodiversity. BioScience. 64:416–428.
- Jing X, Sanders NJ, Shi Y, Chu H, Classen AT, Zhao K, Chen L, Shi Y, Jiang Y, He J-S. 2015. The links between ecosystem multifunctionality and above- and belowground biodiversity are mediated by climate. Nat Commun. 6:8159.
- Jonsson M, Raphael IA, Ekbom B, Kyamanywa S, Karungi-Tumutegyereize J. 2015. Contrasting effects of shade level and altitude on two important coffee pests. J Pest Sci. 88:281–287.
- Kamau S, Barrios E, Karanja N, Ayuke F, Lehmann J. 2017. Soil macrofauna abundance under dominant tree species increases along a soil degradation gradient. Soil Biol Biochem. 112:35–46.
- Karp DS, Mendenhall CD, Sandi RF, Chaumont N, Ehrlich PR, Hadly EA, Daily GC. 2013. Forest bolsters bird abundance, pest control and coffee yield. Ecol Lett. 16:1339–1347.
- Kearns CA, Inouye DW. 1993. Techniques for pollination biologists. Niwot (CO): University Press of Colorado.
- Kibblewhite MG, Ritz K, Swift MJ. 2008. Soil health in agricultural systems. Philos Trans Royal Soc B Biol Sci. 363:685–701.
- Klein A-M, Cunningham SA, Bos M, Steffan-Dewenter I. 2008. Advances in pollination ecology from tropical plantation crops. Ecology. 89:935–943.
- Klein A-M, Vaissiere BE, Cane JH, Steffan-Dewenter I, Cunningham SA, Kremen C, Tscharntke T. 2007. Importance of pollinators in changing landscapes for world crops. Proc Royal Soc B Biol Sci. 274:303–313.
- Kremen C. 2005. Managing ecosystem services: what do we need to know about their ecology. Ecol Lett. 8:468–479.
- Kutywayo D, Chemura A, Kusena W, Chidoko P, Mahoya C. 2013. The impact of climate change on the potential distribution of agricultural pests: the case of the Coffee white stem borer (Monochamus leuconotus P.) in Zimbabwe. PLoS One. 8:e73432.
- Kuyah S, Oborn I, Jonsson M, Dahlin AS, Barrios E, Muthuri C, Malmer A, Nyaga J, Magaju C, Namirembe S, et al. 2016. Trees in agricultural landscapes enhance provision of ecosystem services in Sub-Saharan Africa. Int J Biodiv Sci Eco Serv Mgmt. 12:255–273.
- Lavelle P, Rodriguez N, Arguello O, Bernal J, Botero C, Chaparro P, Gomez Y, Gurierrez A, Hurtado MP, Loaiza S, et al. 2014. Soil ecosystem services and land use in the rapidly changing Orinoco River Basin of Colombia. Agric Ecosyst Environ. 185:107–116.
- Legname E, Brauman A, Poulain J, Razafimbelo T, Pablo A-L, Brüls T, O’Donohue M, Razafindrakoto M, Blanchart E, Chapuis-Lardy L, et al. 2012. Endogeic earthworms shape bacterial functional communities and affect organic matter mineralization in a tropical soil. ISME J. 6:213–222.
- Maestre FT, Quero JL, Gotelli NJ, Escudero A, Ochoa V, Delgado-Baquerizo M, García-Gómez M, Bowker MA, Soliveres S, Escolar C, et al. 2012. Plant species richness and ecosystem multifunctionality in global drylands. Science. 335:214–218.
- Marichal E, Feijoo Martinez A, Praxedes C, Ruiz D, Carvajal AF, Oszwald J, Hurtado MP, Brown GG, Grimaldi M, Desjandins T. 2010. Invasion of Pontoscolex corethrurus (Glossoscolecidae, Oligochaeta) in landscapes of the Amazonian deforestation arc. Appl Soil Ecol. 46(3):443–449.
- Matson PA, Parton WJ, Power AG, Swift MJ. 1997. Agricultural intensification and ecosystem properties. Science. 277:504–509.
- McGregor SE. 1976. Insect pollination of cultivated crop plants. United States Department of Agriculture. https://www.ars.usda.gov/SP2UserFiles/Place/20220500/OnlinePollinationHandbook.pdf.
- Mortimer PE, Gui H, Xu J, Zhang C, Barrios E, Hyde KD. 2015. Alder trees enhance crop productivity and soil microbial biomass in tea plantations. Appl Soil Ecol. 96:25–32.
- Muhamad D. 2013. Socio-ecological assessment of ecosystem services in relation to biodiversity along a gradient from forest to agricultural landscape in West Java, Indonesia [ PhD dissertation]. Department of Ecosystem Studies, Graduate School of Agriculture and Life Sciences, The University of Tokyo, Japan.
- Myers N, Mittermeler RA, Mittermeler CG, Gab DF, Kent J. 2000. Biodiversity hotspots for conservation priorities. Nature. 403(6772):853–858.
- Ong CK, Kho RM, Radersma S. 2004. Ecological interactions in multispecies agroecosystems: concepts and rules. In: Van Noordwijk M, Cadisch G, Ong CK, editors. Below-ground interactions in tropical agroecosystems – concepts and models with multiple plant components. Wallingford (UK): CAB International; p. 1–15.
- Pauli N, Barrios E, Conacher AJ, Oberthur T. 2011. Soil macrofauna in agricultural landscapes dominated by the Quesungual Slash-and-Mulch Agroforestry System, western Honduras. Appl Soil Ecol. 47:119–132.
- Pauli N, Barrios E, Conacher AJ, Oberthur T. 2012. Farmer knowledge of the relationships among soil macrofauna, soil quality, and tree species in a small holder agroforestry system of western Honduras. Geoderma. 189–190:186–198.
- Pauli N, Oberthur T, Barrios E, Conacher AJ. 2010. Fine-scale spatial and temporal variation in earthworm surface casting activity in agroforestry fields, western Honduras. Pedobiologia. 53:127–139.
- Peacock AD, Mullen MD, Ringelberg DB, Tyler DD, Hedrick DB, Gale PM, White DC. 2001. Soil microbial community responses to dairy manure or ammonium nitrate applications. Soil Biol Biochem. 33:1011–1019.
- Peeters LYK, Soto-Pinto L, Perales H, Montoya G, Ishiki M. 2003. Coffee production, timber, and firewood in traditional and Inga-shaded plantations in Southern Mexico. Agric Ecosyst Environ. 95:481–493.
- Perfecto I, Rice RA, Greenberg R, van der Voort ME. 1996. Shade coffee: a disappearing refuge for biodiversity. Bioscience. 46:598–608.
- Perfecto I, Vandermeer J. 2002. Quality of agroecological matrix in a tropical montane landscape: ants in coffee plantations in southern Mexico. Conserv Biol. 16:174–182.
- Perfecto I, Vandermeer J. 2008. Biodiversity conservation in tropical agroecosystems: a new conservation paradigm. Annals NY Acad Sci. 1134:173–200.
- Perfecto I, Vandermeer J, Lopez Bautista G, Nuñez G, Greenberg R, Bichier P, Langridge S. 2004. Greater predation in shaded coffee farms: the role of resident neotropical birds. Ecology. 85:2677–2681.
- Philpott SM, Dietsch T. 2003. Coffee and conservation: a global context and the value of farmer involvement. Conserv Biol. 17:1844–1846.
- Potts SG, Biesmeijer JC, Kremen C, Neumann P, Schweiger O. 2010. Global pollinator declines: trends, impacts and drivers. Trends Ecol Evol. 25:345–353.
- Prabhu R, Barrios E, Bayala J, Diby L, Donovan J, Gyau A, Graudal L, Jamnadass R, Kahia J, Kehlenbeck K, et al. 2015. Agroforestry: realizing the promise of an agroecological approach. In: FAO, editor. Agroecology for food security and nutrition. Rome (Italy): Proceedings of the FAO International Symposium; p. 201–224.
- Prescott CE, Grayston SJ. 2013. Tree species influence on microbial communities in litter and soil: current knowledge and research needs. Forest Ecol Mgmt. 309:19–27.
- Pretty JN, Noble AD, Bossio D, Dixon J, Hine RE, Penning de Vries FWT, Morison JIL. 2006. Resource-conserving agriculture increases yields in developing countries. Environ Sci Technol. 40:1114–1119.
- Pumariño L, Sileshi GW, Gripenberg S, Kaartinen R, Barrios E, Muchane MN, Midega C, Jonsson M. 2015. Effects of agroforestry on pest, disease and weed control: a meta-analysis. Basic Appl Ecol. 16:573–582.
- Rappole JH, King DI, Rivera JHV. 2003. Coffee and conservation. Conserv Biol. 17:334–336.
- Ricketts TH, Daily GC, Ehrlich PR, Michener CD. 2004. Economic value of tropical forest to coffee production. Pnas. 18:12579–12582.
- Ricketts TH, Regetz J, Steffan-Dewenter I, Cunningham SA, Kremen C, Bogdanski A, Gemmill-Herren B, Greenleaf SS, Klein AM, Mayfield MM, et al. 2008. Landscape effects on crop pollination services: are there general patterns? Ecol Lett. 11:499–515.
- Rockström J, Steffen W, Noone K, Persson Å, Chapin-III FS, Lambin E, Lenton TM, Scheffer M, Folke C, Schellnhuber H, et al. 2009. Planetary boundaries: exploring the safe operating space for humanity. Ecol Soc. 14(2):32. [online]. http://www.ecologyandsociety.org/vol14/iss2/art32/.
- Romero-Alvarado Y, Soto-Pinto L, Garcıa-Barrios L. 2002. Coffee yields and soil nutrients under the shades of Inga sp. vs. multiple species in Chiapas, Mexico. Agroforestry Syst. 54:215–224.
- Roubik DW. 1995. Pollination of cultivated plants in the tropics. FAO Agricultural Services Bulletin 118. Rome: FAO.
- Rousseau L, Fonte SJ, Tellez O, van der Hoek R, Lavelle P. 2013. Soil macrofauna as indicators of soil quality and land use impacts in smallholder agroecosystems of western Nicaragua. Ecol Indic. 27:71–82.
- Rutherford MA, Phiri N, editors. 2006. Pests and diseases of coffee in Eastern Africa: a technical and advisory manual. Wallingford (UK): CAB International.
- Schroth G, Da Fonseca GAB, Harvey CA, Gascon C, Vasconcelos HL, Izac AM, editors. 2004. Agroforestry and biodiversity conservation in tropical landscapes. Washington (DC): Island Press. http://publications.cirad.fr/une_notice.php?dk=518308.
- Selmants PC, Hart SC, Boyle SI, Stark JM. 2005. Red alder (Alnus rubra) alters community-level soil microbial function in conifer forests of the Pacific Northwest, USA. Soil Biol Biochem. 37:1860–1868.
- Šnajdr J, Dobiášová P, Urbanová M, Petránková M, Cajthaml T, Frouz J, Baldrian P. 2013. Dominant trees affect microbial community composition and activity in post-mining afforested soils. Soil Biol Biochem. 56:105–115.
- Snapp SS, Blackie M, Gilbert RA, Bezner-Kerr R, Kanyama-Phiri GY. 2010. Biodiversity can support a greener revolution in Africa. PNAS. 107:20840–20845.
- Steffan-Dewenter I, Kessler M, Barkmann J, Bos MM, Buchori D, Erasmi S, Faust H, Gerold G, Glenk K, Gradstein SR, et al. 2007. Trade-offs between income, biodiversity, and ecosystem functioning during tropical rainforest conversion and agroforestry intensification. PNAS. 104:4973–4978.
- Swift MJ, Izac AM, Van Noordwijk M. 2004. Biodiversity and ecosystems services in agricultural landscapes - are we asking the right questions? Agric Ecosyst Environ. 104:113–134.
- Teodoro A, Klein AM, Reis PR, Tscharntke T. 2009. Agroforestry management affects coffee pests contingent on season and developmental stage. Agric Forest Entomol. 11:295–300.
- Tittonell P. 2014. Ecological intensification of agriculture - sustainable by nature. Curr Opin Environ Sustain. 8:53–61.
- Tomich TP, Brodt S, Ferris H, Galt R, Horwarth WR, Kebreab E, Leveau JHJ, Liptzin D, Lubell M, Merel P, et al. 2011. Agroecology: a review from a global-change perspective. Annu Rev Environ Resour. 36:193–222.
- Tscharntke T, Clough Y, Bhagwat SA, Buchori D, Faust H, Hertel D, Hölscher D, Juhrbandt J, Kessler M, Perfecto I, et al. 2011. Multifunctional shade-tree management in tropical agroforestry landscapes - a review. J Appl Ecol. 48:619–629.
- Tscharntke T, Clough Y, Wanger TC, Jackson L, Motzke I, Perfecto I, Vandermeer J, Whitbread A. 2012a. Global food security, biodiversity conservation and the future of agricultural intensification. Biol Conserv. 151:53–59.
- Tscharntke T, Tylianakis JM, Rand TA, Didham RK, Fahrig L, Batáry P, Bengtsson J, Clough Y, Crist TO, Dormann CF, et al. 2012b. Landscape moderation of biodiversity patterns and processes - eight hypotheses. Biol Rev. 87:661–685.
- Ushio M, Kitayama K, Balser T. 2010. Tree species-mediated spatial patchiness of the composition of microbial community and physicochemical properties in the topsoils of a tropical montane forest. Soil Biol Biochem. 42:1588–1595.
- Valencia V, García-Barrios L, West P, Sterling EJ, Naeem S. 2014. The role of coffee agroforestry in the conservation of tree diversity and community composition of native forests in a Biosphere Reserve. Agric Ecosyst Environ. 189:154–163.
- Valencia V, Naeem S, Garcia-Barrios L, West P, Sterling EJ. 2016. Conservation of tree species of late succession and conservation concern in coffee agroforestry systems. Agric Ecosyst Environ. 219:32–41.
- Valencia V, West P, Sterling EJ, García-Barrios L, Naeem S. 2015. The use of farmers’ knowledge in coffee agroforestry management: implications for the conservation of tree biodiversity. Ecosphere. 6:art122.
- Van der Putten WH, Bardgett R, Bever JD, Bezemer TM, Casper BB, Fukami T, Kardol P, Kliromonos JN, Kulmatski A, Schweitzer JA, et al. 2013. Plant-soil feedbacks: the past, the present and future challenges. J Ecology. 101:265–276.
- Vandermeer J, Perfecto I. 2007. The agricultural matrix and a future paradigm for conservation. Conserv Biol. 21:274–277.
- Vanlalhluna PC, Sahoo UK. 2009. Performance of multi-purpose trees and associated crops in agroforestry system of Mizoram. Indian J Forest. 32:191–194.
- Wall DH, Bardgett RD, Kelly EF. 2010. Biodiversity in the dark. Nat Geosci. 3:297–298.
- Wardle DA, Bardgett RD, Kliromonos JN, Setala H, Van der Putten W, Wall DH. 2004. Ecological linkages between aboveground and belowground biota. Science. 304:1629–1633.
- Zomer RJ, Neufeldt H, Xu J, Ahrends A, Bossio D, Trabucco A, van Noordwijk M, Wan M. 2016. Global tree cover and biomass carbon on agricultural land: the contribution of agroforestry to global and national carbon budgets. Sci Rep. 6:29987.