ABSTRACT
The YEATS domains of AF9 and Taf14 have recently been found to recognize the histone H3K9ac modification. In this commentary, we discuss the mechanistic and biological implications of this interaction. We compare structures of the YEATS-H3K9ac complexes, highlighting a novel mechanism for the acetyllysine recognition through the aromatic cage. We also summarize the latest findings underscoring a critical role of the acetyllysine binding function of AF9 and Taf14 in transcriptional regulation and DNA repair.
Introduction
The YEATS (Yaf9, ENL, AF9, Taf14, and Sas5) domain is an evolutionarily conserved module present in at least 4 human proteins (ENL, AF9, GAS41, YEATS2) and 3 yeast proteins (Sas5, Taf14, Yaf9) (reviewed inCitation1). The YEATS domain proteins are found in major chromatin-remodeling and histone acetyltransferase (HAT) complexes and are implicated in the regulation of chromatin structure, histone acetylation and deposition, gene transcription and DNA damage response. Although the YEATS domain was identified over a decade ago,Citation2 its functional role has not been understood until recently.Citation3,4 In 2009, based on genetic analysis, the YEATS domain was proposed to bind acetylated lysine residues in histones, which would facilitate the recruitment of the host proteins and complexes to specific genomic sites.Citation1,5 However, the experimentally established function of the YEATS domain surprisingly remained undetermined for 5 more years.
Recently, the YEATS domain of AF9, a component of the super elongation complex (SEC), was found to recognize acetyllysine modifications in histone H3, preferring H3K9ac.Citation3 In a subsequent study, the YEATS domain of Taf14, a member of at least 6 chromatin-associated complexes in yeast, has been shown to target H3K9ac.Citation4 The YEATS domain has expanded the family of the acetyllysine readers, which currently comprises bromodomain, the double PHD finger (DPF) and the double pleckstrin homology (PH) domain.Citation6-9 While some acetyllysine readers are known to interact with acetyl marks broadly associated with active transcription, none had been shown to select for the H3K9ac mark commonly enriched at transcriptional start sites.Citation10,11
Role of the YEATS domain proteins in transcription
The human YEATS domain-encoding AF9, ENL and GAS41 genes are common fusion partners of the mixed lineage leukemia (MLL) gene, with the AF9-MLL genetic rearrangement occurring in approximately 20% of acute leukemias.Citation12 Early studies in mice implicated AF9 in normal embryonic development possibly through regulation of HOX gene expression,Citation13 and recent ChIP-seq analysis revealed that AF9 is recruited to H3K9ac-enriched loci near the HOX gene cluster in cancer cells.Citation3 AF9 is found to associate with several nuclear complexes, most notably the SEC,Citation14-16 and separately with the histone H3K79-specific methyltransferase DOT1L.Citation3,17,Citation18 DOT1L-mediated H3K79 methylation is strongly linked to active gene expression, suggesting that interaction of AF9 with DOT1L may play a role in DOT1L recruitment to gene promoters and transcriptional activation. Significantly, Li et al. showed that the YEATS domain of AF9 is required for targeting of DOT1L to H3K9ac-enriched chromatin and subsequent H3K79 methylation and for transcription of target genes such as MYC.Citation3 These results provide new insight into how H3K9 acetylation links AF9 to DOT1L-mediated methylation and transcription. Like DOT1L, SEC binds to the C-terminus of AF9;Citation17 however, it remains unclear if H3K9ac has a role in AF9-dependent SEC recruitment and function. Moreover, as SEC associates with the bromodomain-containing protein BRD4,Citation19 it is also conceivable that SEC recruitment and function may depend on the combinatorial acetyllysine-binding activities of both BRD4 and AF9.
Taf14 is a core component of the general transcription factor complexes TFIID and TFIIF that promote the assembly of the RNA polymerase II pre-initiation complex,Citation20 and is also found in at least 3 chromatin-remodeling complexes: INO80, SWI/SNF and RSC.Citation20-22 In addition, Taf14 is an integral member of the histone acetyltransferase complex NuA3.Citation23 Because Taf14 is present in such a diverse set of chromatin-associated complexes, its biological role has been difficult to discern. Initial studies demonstrated that the YEATS domain of Taf14 negatively impacts cell growth,Citation24,25 and more recently, this domain was shown to recognize H3K9ac.Citation4 Genetic assays using Taf14 impaired in H3K9ac binding revealed a critical role of this interaction in mediating transcription of a subset of target genes in yeast.Citation4 Furthermore, the acetyllysine binding activity of Taf14 was found to be essential in the response to DNA damage induced by the alkylating agent methyl methanesulfonate.Citation4 Given the fact that Taf14 is a subunit of various distinct transcription and chromatin-remodeling complexes, future studies are necessary to establish the precise contribution of this protein to the specific activities of each Taf14-containing complex.
Unique mechanism for the acetyllysine recognition via an aromatic cage
The crystal structures of the YEATS domains of AF9 and Taf14 in complex with H3K9ac peptides have recently been determined and show high degree similarities in overall architecture and the acetyllysine binding mechanism (). Both YEATS domains adopt an extended immunoglobin fold, composed of 8 antiparallel β strands (β1-β8) connected by 8 loops (L1-L8).Citation3,4 Comparison of the H3K9ac-binding sites reveals a unique acetyllysine-recognition mechanism that is conserved in the 2 YEATS domains but differs substantially from the binding mechanisms used by other acetyllysine readers, such as the bromodomain. Unlike bromodomains, which anchor acetyllysine in the binding pocket primarily through the formation of a hydrogen bond between the acetyl carbonyl group and an invariable asparagine residue of the bromodomain, the YEATS domains lock K9ac in an aromatic cage through a network of hydrophobic, π-π stacking, and polar interactions. The aromatic cage in AF9 consists of 5 aromatic residues (F28, H56, F59, Y78 and F81) and a serine (S58), whereas the aromatic cage in Taf14 has 4 aromatic residues (H59, F62, W81, F84) and a threonine (T61) ().
Figure 1. A comparative structural analysis of the AF9-H3K9ac and Taf14-H3K9ac complexes. (A) An overlay of ribbon diagrams of the AF9 YEATS domain (cyan) in complex with the H3K9ac peptide (salmon) and the Taf14 YEATS domain (yellow) in complex with the H3K9ac peptide (gray). (B) The Taf14 (yellow) and AF9 (cyan) aromatic cages highlighting the interactions with K9ac. Red spheres represent water molecules, and apparent hydrogen bonds are indicated by blue dashes. For clarity backbone atoms were removed, unless involved in hydrogen bonds. (C) Superimposition of the H3K9ac peptides bound by Taf14 (gray) and AF9 (salmon) reveals that the RKac motif adopts a very similar conformation in both complexes. PDB IDs 4TMP and 5D7E.
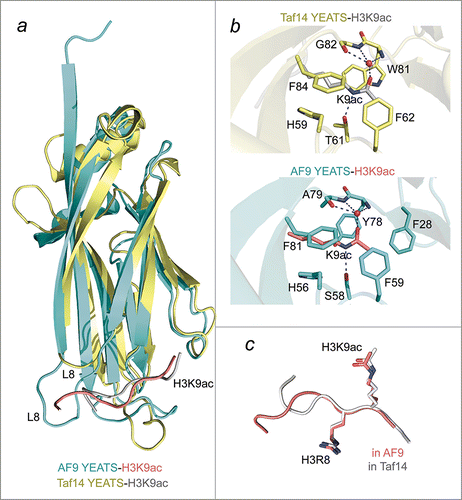
Arguably, one of the most striking characteristics of the acetyllysine binding mechanism of the YEATS domain is that the acetyl group of K9ac is intercalated between 2 aromatic residues, F59/F62 and Y78/W81 in AF9/Taf14, respectively. Additionally, the side chain amide of K9ac is restrained by hydrogen bonds formed with the hydroxyl group of S58/T61 and the backbone amide of Y78/W81 of AF9/Taf14 and through water-mediated hydrogen bonds with A79/G82. Substitution of any of the aromatic cage residues with an alanine disrupts or diminishes binding of the YEATS domain to H3K9ac, underscoring the imperative role of these residues in the recognition and retention of the histone mark.
AF9 and Taf14 YEATS domains select for the RKac motif
The YEATS domains of AF9 and Taf14 are selective readers of the H3K9ac mark, however both proteins are also capable of recognizing the H3K18ac and H3K27ac modifications with slightly reduced affinities.Citation3,4 In contrast, binding of the YEATS domains to H3K14ac and to various acetylated histone H4 peptides is considerably weaker or undetectable. Unlike the H3K14ac sequence, the H3K9ac, H3K18ac, and H3K27ac sequences all possess an arginine in the -1 position and a small hydrophobic residue in the -2 position relative to the acetyllysine mark. In the YEATS-H3K9ac complexes, the guanidino group of R8 of the histone peptide forms a salt bridge with a conserved aspartic acid of the YEATS domain that provides additional stabilization of the complex. In support of the important role of R8 for the interaction, the AF9 YEATS domain has been shown to associate with the H3R8AK9ac peptide ~200 fold weaker than it associates with the H3K9ac peptide.Citation3 It is likely that methylation of R8, which is linked to transcriptional repression and is antagonistic to K9ac, also blocks recognition of H3K9ac by the YEATS domain.Citation26 An overlay of the bound H3K9ac peptides in the AF9 and Taf14 complexes demonstrates that the R8 and K9ac residues can be superimposed entirely, whereas the residues preceding R8 adopt different conformations (). This structural analysis suggests that the YEATS domains of AF9 and Taf14 recognize the signature RKac motif, which may explain the ability of these proteins to bind not only H3K9ac but also H3K18ac and H3K27ac, albeit ~3-4 times weaker.
Multiple contacts increase affinity
While the AF9 and Taf14 YEATS domains demonstrate selectivity toward H3K9ac, there are substantial differences in their binding affinities.Citation3,4 The AF9 YEATS domain associates with H3K9ac with a 3.7 μM affinity, whereas binding affinity of the Taf14 YEATS domain for H3K9ac is 150 μM. The difference is most likely due to additional intermolecular polar and hydrophobic interactions observed in the AF9 YEATS-H3K9ac complex. These include water-mediated hydrogen bonds between the hydroxyl group of T6 and the side chain of Q5 and the main chain amide of L106 (in the Taf14 structure, the hydroxyl group of T6 is pointed away from the interface). The side chain of Q5 is hydrogen bonded to the side chain of R8 and forms an additional water-mediated hydrogen bond with the side chain of D103. A number of van der Waals interactions are seen between the 11-residue (L8) loop of AF9 and aliphatic portion of K4. However this loop is much shorter in Taf14, spanning only 6 residues, and is unlikely capable of providing the number of interactions with K4, as observed in the AF9 structure. Collectively, the increased contacts of Q5, T6, and K4 may account for a tighter binding of AF9.
A possible role of polyacetylation?
Peptide microarray containing multiply modified histone peptides and fluorescence polarization binding assays demonstrate that polyacetylated histone H3 substrates enhance binding activity of the Taf14 YEATS domain ( and refCitation4), and this general trend appears to be conserved for the YEATS domain of AF9 (). These results suggest additional potential mode for the acetyllysine recognition. Specifically, we have shown that the Taf14 YEATS domain associates with the H3K9acK14acK18ac peptide with a Kd of 50 μM.Citation4 This corresponds to a 3-fold increase in binding activity compared to binding of this domain to the mono-acetylated H3K9ac peptide. Analysis of the surface of the Taf14 YEATS domain in the context of electrostatic potential and the presence of either an asparagine residue or an additional aromatic cage reveals putative binding sites that can potentially accommodate extra acetyllysine residues in proximity of the K9ac-binding aromatic cage; however, further studies are needed to validate this possibility.
Figure 2. H3K9ac-YEATS interaction is modulated by neighboring histone PTMs. (A) Analysis of peptide microarray data from ref.Citation4 showing interactions between the YEATS domain of Taf14 and the indicated H3K9ac-containing peptides. Binding intensity is shown relative to H3K9ac without neighboring modifications (white). (B) Peptide pulldown and western blot analysis of the interaction between AF9-YEATS and the indicated peptides performed as previously described.Citation4 (C) The binding site of the Taf14 YEATS domain (yellow) for the H3K9ac peptide (gray).
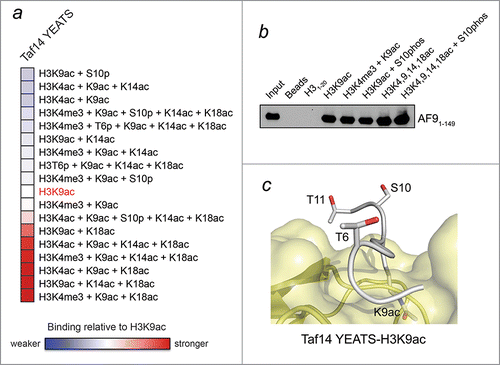
Effect of other neighboring PTMs on the ability of Taf14 to bind H3K9ac
Histone H3K9 is a vital residue that can be methylated (H3K9me3) to mediate heterochromatin formation, or acetylated to stimulate active transcription. H3K9me3 recruits chromodomain-containing proteins, such as HP1, to condense chromatin and silence gene expression.Citation27 As H3K9me3 recruitment is negatively regulated, in part, through phosphorylation of neighboring serine 10 (H3S10ph), this finding has given rise to the concept of a ‘phospho-methyl’ switch.Citation28,29 A similar switch has been shown to eject the H3K4me3-interacting PHD fingers when neighboring H3T3 or H3T6 are phosphorylated.Citation30-33 To assess whether ‘phospho-acetyl’ switches can regulate the function of Taf14, we evaluated binding activity of the Taf14 YEATS domain toward histone peptides harboring S10ph and T6ph modifications along with H3K9ac ( and refCitation4). We found that unlike chromodomain and the PHD fingers, the Taf14 YEATS domain can accommodate adjacent phosphorylation marks, T6ph and S10ph, when it binds to H3K9ac. In the structure of the Taf14-H3K9ac complex, the hydroxyl groups of T6 and S10 are both solvent exposed and orientated away from the binding interface, making phosphorylation tolerable (). This analysis suggests that Taf14, unlike HP1 and PHD finger-containing proteins, might remain on mitotic chromatin throughout M-phase and act similar to BRD4 as a mitotic bookmark for transcription.Citation34,35
Methylation of H3R8 and acetylation of H3K9 mediate opposite transcriptional outcomes, and the prior H3K9ac mark is known to prevent methylation of H3R8.Citation26,36 It remains unclear whether the H3K8me(1/2)K9ac modifications co-exist on the same histone tail, but if they do, methylation of R8 should likely have a negative impact on the recognition of K9ac by the YEATS domain, revealing a 'methylarginine-acetyllysine' epigenetic switch.
Concluding remarks
In the past several years, we have witnessed a rapid expansion of our knowledge about writing, reading and erasing histone modifications. Not only have a large number of PTMs, and combinations thereof, been identified, but novel writers, readers and erasers of these marks are still continuing to be discovered – arguing that we may have reached only the tip of the iceberg in terms of our understanding of epigenetic phenomenon. The YEATS domain represents an exciting example in this regard, revealing a previously unknown mechanism for reading the acetyllysine PTM. This advance further suggests that many more chromatin regulators are expected to be uncovered in the future.
Disclosure of potential conflicts of interest
No potential conflicts of interest were disclosed.
Funding
Research in the T.G.K. laboratory is supported by grants from the NIH, GM101664, GM106416, and GM100907. Research in the B.D.S. laboratory is supported by a grant from the NIH, GM110058. F.H.A is supported by the NIH grant T32AA007464. E.K.S. is supported by the NIGMS IRACDA grant K12-GM000678.
References
- Schulze JM, Wang AY, Kobor MS. Reading chromatin: insights from yeast into YEATS domain structure and function. Epigenetics 2010; 5:573-7; PMID:20657183; http://dx.doi.org/10.4161/epi.5.7.12856
- Le Masson I, Yu DY, Jensen K, Chevalier A, Courbeyrette R, Boulard Y, Smith MM, Mann C. Yaf9, a novel NuA4 histone acetyltransferase subunit, is required for the cellular response to spindle stress in yeast. Mol Cell Biol 2003; 23:6086-102; PMID:12917332; http://dx.doi.org/10.1128/MCB.23.17.6086-6102.2003
- Li Y, Wen H, Xi Y, Tanaka K, Wang H, Peng D, Ren Y, Jin Q, Dent SY, Li W, et al. AF9 YEATS domain links histone acetylation to DOT1L-mediated H3K79 methylation. Cell 2014; 159:558-71; PMID:25417107; http://dx.doi.org/10.1016/j.cell.2014.09.049
- Shanle EK, Andrews FH, Meriesh H, McDaniel SL, Dronamraju R, DiFiore JV, Jha D, Wozniak GG, Bridgers JB, Kerschner JL, et al. Association of Taf14 with acetylated histone H3 directs gene transcription and the DNA damage response. Genes Dev 2015; 29:1795-800; PMID:26341557; http://dx.doi.org/10.1101/gad.269977.115
- Schulze JM, Wang AY, Kobor MS. YEATS domain proteins: a diverse family with many links to chromatin modification and transcription. Biochem Cell Biol 2009; 87:65-75; PMID:19234524; http://dx.doi.org/10.1139/O08-111
- Dhalluin C, Carlson JE, Zeng L, He C, Aggarwal AK, Zhou MM. Structure and ligand of a histone acetyltransferase bromodomain. Nature 1999; 399:491-6; PMID:10365964; http://dx.doi.org/10.1038/20974
- Lange M, Kaynak B, Forster UB, Tonjes M, Fischer JJ, Grimm C, Schlesinger J, Just S, Dunkel I, Krueger T, et al. Regulation of muscle development by DPF3, a novel histone acetylation and methylation reader of the BAF chromatin remodeling complex. Genes Dev 2008; 22:2370-84; PMID:18765789; http://dx.doi.org/10.1101/gad.471408
- Zeng L, Zhang Q, Li S, Plotnikov AN, Walsh MJ, Zhou MM. Mechanism and regulation of acetylated histone binding by the tandem PHD finger of DPF3b. Nature 2010; 466:258-62; PMID:20613843; http://dx.doi.org/10.1038/nature09139
- Su D, Hu Q, Li Q, Thompson JR, Cui G, Fazly A, Davies BA, Botuyan MV, Zhang Z, Mer G. Structural basis for recognition of H3K56-acetylated histone H3-H4 by the chaperone Rtt106. Nature 2012; 483:104-7; PMID:22307274; http://dx.doi.org/10.1038/nature10861
- Filippakopoulos P, Picaud S, Mangos M, Keates T, Lambert JP, Barsyte-Lovejoy D, Felletar I, Volkmer R, Muller S, Pawson T, et al. Histone recognition and large-scale structural analysis of the human bromodomain family. Cell 2012; 149:214-31; PMID:22464331; http://dx.doi.org/10.1016/j.cell.2012.02.013
- Bernstein BE, Kamal M, Lindblad-Toh K, Bekiranov S, Bailey DK, Huebert DJ, McMahon S, Karlsson EK, Kulbokas EJ, 3rd, Gingeras TR, et al. Genomic maps and comparative analysis of histone modifications in human and mouse. Cell 2005; 120:169-81; PMID:15680324; http://dx.doi.org/10.1016/j.cell.2005.01.001
- Meyer C, Hofmann J, Burmeister T, Groger D, Park TS, Emerenciano M, Pombo de Oliveira M, Renneville A, Villarese P, Macintyre E, et al. The MLL recombinome of acute leukemias in 2013. Leukemia 2013; 27:2165-76; PMID:23628958; http://dx.doi.org/10.1038/leu.2013.135
- Collins EC, Appert A, Ariza-McNaughton L, Pannell R, Yamada Y, Rabbitts TH. Mouse Af9 is a controller of embryo patterning, like Mll, whose human homologue fuses with Af9 after chromosomal translocation in leukemia. Mol Cell Biol 2002; 22:7313-24; PMID:12242306; http://dx.doi.org/10.1128/MCB.22.20.7313-7324.2002
- Lin C, Smith ER, Takahashi H, Lai KC, Martin-Brown S, Florens L, Washburn MP, Conaway JW, Conaway RC, Shilatifard A. AFF4, a component of the ELL/P-TEFb elongation complex and a shared subunit of MLL chimeras, can link transcription elongation to leukemia. Mol Cell 2010; 37:429-37; PMID:20159561; http://dx.doi.org/10.1016/j.molcel.2010.01.026
- Sobhian B, Laguette N, Yatim A, Nakamura M, Levy Y, Kiernan R, Benkirane M. HIV-1 Tat assembles a multifunctional transcription elongation complex and stably associates with the 7SK snRNP. Mol Cell 2010; 38:439-51; PMID:20471949; http://dx.doi.org/10.1016/j.molcel.2010.04.012
- Yokoyama A, Lin M, Naresh A, Kitabayashi I, Cleary ML. A higher-order complex containing AF4 and ENL family proteins with P-TEFb facilitates oncogenic and physiologic MLL-dependent transcription. Cancer Cell 2010; 17:198-212; PMID:20153263; http://dx.doi.org/10.1016/j.ccr.2009.12.040
- He N, Chan CK, Sobhian B, Chou S, Xue Y, Liu M, Alber T, Benkirane M, Zhou Q. Human Polymerase-Associated Factor complex (PAFc) connects the Super Elongation Complex (SEC) to RNA polymerase II on chromatin. Proc Natl Acad Sci U S A 2011; 108:E636-45; PMID:21873227; http://dx.doi.org/10.1073/pnas.1107107108
- Zhang W, Xia X, Reisenauer MR, Hemenway CS, Kone BC. Dot1a-AF9 complex mediates histone H3 Lys-79 hypermethylation and repression of ENaCalpha in an aldosterone-sensitive manner. J Biol Chem 2006; 281:18059-68; PMID:16636056; http://dx.doi.org/10.1074/jbc.M601903200
- Luo Z, Lin C, Shilatifard A. The super elongation complex (SEC) family in transcriptional control. Nature reviews. Mol Cell Biol 2012; 13:543-7; PMID:22895430
- Kabani M, Michot K, Boschiero C, Werner M. Anc1 interacts with the catalytic subunits of the general transcription factors TFIID and TFIIF, the chromatin remodeling complexes RSC and INO80, and the histone acetyltransferase complex NuA3. Biochem Biophys Res Commun 2005; 332:398-403; PMID:15896708; http://dx.doi.org/10.1016/j.bbrc.2005.04.158
- Shen X. Preparation and analysis of the INO80 complex. Methods Enzymol 2004; 377:401-12; PMID:14979041; http://dx.doi.org/10.1016/S0076-6879(03)77026-8
- Cairns BR, Henry NL, Kornberg RD. TFG/TAF30/ANC1, a component of the yeast SWI/SNF complex that is similar to the leukemogenic proteins ENL and AF-9. Mol Cell Biol 1996; 16:3308-16; PMID:8668146; http://dx.doi.org/10.1128/MCB.16.7.3308
- John S, Howe L, Tafrov ST, Grant PA, Sternglanz R, Workman JL. The something about silencing protein, Sas3, is the catalytic subunit of NuA3, a yTAF(II)30-containing HAT complex that interacts with the Spt16 subunit of the yeast CP (Cdc68/Pob3)-FACT complex. Genes Dev 2000; 14:1196-208; PMID:10817755
- Schulze JM, Kane CM, Ruiz-Manzano A. The YEATS domain of Taf14 in Saccharomyces cerevisiae has a negative impact on cell growth. Mol Genet Genomics 2010; 283:365-80; PMID:20179968; http://dx.doi.org/10.1007/s00438-010-0523-x
- Zhang W, Zhang J, Zhang X, Xu C, Tu X. Solution structure of the Taf14 YEATS domain and its roles in cell growth of Saccharomyces cerevisiae. Biochem J 2011; 436:83-90; PMID:21355849; http://dx.doi.org/10.1042/BJ20110004
- Gayatri S, Bedford MT. Readers of histone methylarginine marks. Biochim Biophys Acta 2014; 1839:702-10; PMID:24583552; http://dx.doi.org/10.1016/j.bbagrm.2014.02.015
- Blus BJ, Wiggins K, Khorasanizadeh S. Epigenetic virtues of chromodomains. Crit Rev Biochem Mol Biol 2011; 46:507-26; PMID:22023491
- Fischle W, Tseng BS, Dormann HL, Ueberheide BM, Garcia BA, Shabanowitz J, Hunt DF, Funabiki H, Allis CD. Regulation of HP1-chromatin binding by histone H3 methylation and phosphorylation. Nature 2005; 438:1116-22; PMID:16222246; http://dx.doi.org/10.1038/nature04219
- Hirota T, Lipp JJ, Toh BH, Peters JM. Histone H3 serine 10 phosphorylation by Aurora B causes HP1 dissociation from heterochromatin. Nature 2005; 438:1176-80; PMID:16222244; http://dx.doi.org/10.1038/nature04254
- Garske AL, Oliver SS, Wagner EK, Musselman CA, LeRoy G, Garcia BA, Kutateladze TG, Denu JM. Combinatorial profiling of chromatin binding modules reveals multisite discrimination. Nat Chem Biol 2010; 6:283-90; PMID:20190764; http://dx.doi.org/10.1038/nchembio.319
- Varier RA, Outchkourov NS, de Graaf P, van Schaik FM, Ensing HJ, Wang F, Higgins JM, Kops GJ, Timmers HT. A phospho/methyl switch at histone H3 regulates TFIID association with mitotic chromosomes. EMBO J 2010; 29:3967-78; PMID:20953165; http://dx.doi.org/10.1038/emboj.2010.261
- Gatchalian J, Futterer A, Rothbart SB, Tong Q, Rincon-Arano H, Sanchez de Diego A, Groudine M, Strahl BD, Martinez AC, van Wely KH, et al. Dido3 PHD modulates cell differentiation and division. Cell Rep 2013; 4:148-58; PMID:23831028; http://dx.doi.org/10.1016/j.celrep.2013.06.014
- Ali M, Rincon-Arano H, Zhao W, Rothbart SB, Tong Q, Parkhurst SM, Strahl BD, Deng LW, Groudine M, Kutateladze TG. Molecular basis for chromatin binding and regulation of MLL5. Proc Natl Acad Sci U S A 2013; 110:11296-301; PMID:23798402; http://dx.doi.org/10.1073/pnas.1310156110
- Voigt P, Reinberg D. BRD4 jump-starts transcription after mitotic silencing. Genome Biol 2011; 12:133; PMID:22126464; http://dx.doi.org/10.1186/gb-2011-12-11-133
- Zhao R, Nakamura T, Fu Y, Lazar Z, Spector DL. Gene bookmarking accelerates the kinetics of post-mitotic transcriptional re-activation. Nat Cell Biol 2011; 13:1295-304; PMID:21983563; http://dx.doi.org/10.1038/ncb2341
- Pal S, Vishwanath SN, Erdjument-Bromage H, Tempst P, Sif S. Human SWI/SNF-associated PRMT5 methylates histone H3 arginine 8 and negatively regulates expression of ST7 and NM23 tumor suppressor genes. Mol Cell Biol 2004; 24:9630-45; PMID:15485929; http://dx.doi.org/10.1128/MCB.24.21.9630-9645.2004