ABSTRACT
Epigenetic marks determine cell fate via numerous reader proteins. H3K36 methylation is a common epigenetic mark that is thought to be associated with the activities of the RNA polymerase 2 C-terminal domain. We discuss a novel silencing mechanism regulated by Set2-dependent H3K36 methylation that involves exosome-dependent RNA processing.
Introduction
Post-translational modification of histone proteins plays a critical role in the alteration of chromatin structure, which determines epigenetic inheritance of cellular traits. Three groups of proteins define the state of chromatin. “Writer” proteins are required for adding modifications to specific histone residues, the “reader” recognizes modifications and recruits effector proteins that organize chromatin, and the “eraser” removes unnecessary modifications from histones. These three factors act co-operatively during cell proliferation, differentiation, and environmental adaptation to regulate the state of chromatin and maintain cellular homeostasis. In this point of view, we describe what is known about the distinct physiological roles of H3K36 methylation (H3K36me) states, which are regulated in vivo by Set2 and RNA polymerase 2 (Pol2).
Histone H3K36me writer
Histone H3K36me is highly conserved in eukaryotesCitation1 and is important for chromatin organization. A recent study showed that H3K36me is required for the formation of the highly condensed “knob” chromatin structure adjacent to subtelomeric regions in fission yeast.Citation2 H3K36me comprises three states—i.e., mono-, di-, and tri-methylation of lysine side chain amino groups. Several “writer” proteins for H3K36me have shown enzymatic activity in vitro and in vivo. Only SETD2 has trimethylation activity for H3K36, although eight other H3K36 methyltransferases [nuclear receptor-binding SET domain protein (NSD)1, NSD2, NSD3, MES-4, absent, small, or homeotic-like-1L, SET domain and mariner transposase fusion gene, SET and MYND domain-containing 2, and SETD3] have H3K36 mono- or di-methylation activity in metazoans,Citation1 suggesting that each H3K36me state has a distinct role. The basic mechanisms of Set2—the most highly conserved H3K36 methyltransferase (SETD2 and SETD3 are members of the Set2 family in metazoans)—have been established from studies of budding yeast. Set2 was shown to modify histone H3K36 by mono-, di-, and tri-methylation via its catalytic SET domain.Citation3 Moreover, the Set2-Rpb1-interacting (SRI) domain of Set2 interacts with RNA Pol2 when the Pol2 C-terminal domain (CTD) is phosphorylated at S2/S5 during transcriptional elongation.Citation4 This indicates that Pol2 also functions as a “writer,” which is supported by the overlapping genome-wide chromatin immunoprecipitation (ChIP) patterns of S2/S5-phosphorylated Pol2, H3K36me2, and H3K36me3 along gene bodies. Meanwhile, H3K36me is also detected in promoter regions and double-strand break (DSB) sites.Citation5-8 Other factors may associate with Set2 for H3K36me in untranscribed regions. Clarifying the mechanisms of Pol2 CTD-independent Set2 recruitment to promoters and DSBs and identifying H3K36me-associated factors are important issues in future studies.
H3K36me reader
H3K36me is recognized by the plant homeodomain (PHD) finger, as well as the chromo, tudor, and PWWP domains.Citation1 Additional domains may recognize different states of H3K36me. In budding yeast, the Rpd3S histone deacetylase complex (HDAC) associates with methylated H3K36, which requires a combination of the PHD finger (Rco1) and the chromodomain (Eaf3) to repress cryptic transcription from gene bodies.Citation9,10 In mammals, a similar transcriptional repression phenomena in gene bodies have been reported. Dnmt3a, a Dnmt3 family DNA methyltransferase protein, directly binds to H3K36 trimethylation (H3K36me3) via PWWP domain to methylate nucleosomal DNA in vitro.Citation11 Dnmt3b, another Dnmt3 family protein, is also suggested to correlate with H3K36me3 in ZNF gene bodies located on human chromosome 19 for transcriptional repression.Citation12 H3K36me3 is specifically bound by PHF1 and PHF19 via the tuder domain. PHF1 and PHF19 are accessory components of polycomb repressive complex 2 (PRC2), and Hoxb5/Hoxb6 gene locus and differentiation associated genes (Otx2, Hoxa5, Fgf15, and Meis1) are selectively H3K27me3 enriched by PRC2 via the tuder domain of PHF1 and PHF15, respectively.Citation13,14 H3K36me3 is also related to alternative splicing. MRG15 interacts with H3K36me3 marks via the chromodomain to regulate alternative splicing of FGFR2 pre-mRNA in mesenchymal cells. H3K36me3-bound MRG15 recruits polypyrimidine-tract binding protein to chromatin for pre-mRNA alternative splicing.Citation15-17 There is another factor that connects H3K36me3 and alternative splicing. Psip1 also selectively interacts with H3K36me3 via PWWP domain, and recruits Srsf1 and other splicing factors to regulate alternative splicing of 95 exons in MEFs.Citation18 These results are important examples of epigenetic-mark-dependent RNA processing. In budding yeast, Ecm5 and Nto1 bind to H3K36me3 via PHD fingers, although their biological functions as “readers” have yet to be established.Citation19
H3K36me eraser
Histone lysine demethylase (KDM) is required to demethylate histone H3/H4. KDM contains the JmjC catalytic site, which requires α-ketoglutarate and Fe2+ for activity.Citation20,21 In yeast, Jhd1 (KDM2) and Rph1 (KDM4)—both of which contain a JmjC domain for demethylation activity—are known “erasers” of H3K36me.Citation22,23 Phenotypic analyses of mutants of these two demethylases have revealed that Rph1 is more critical than Jhd1, although the dynamics and functional redundancies of these enzymes as well as their associated factors and relationship to methylation states of H3K36 remain to be elucidated.
Set2-dependent H3K36me
Histone H3K36 is highly methylated along the transcriptional unit in chromatin. Set2—which was initially identified as a nucleosomal histone methyltransferase from budding yeast whole cell extractCitation24—contains a SET domain, as well as an SRI domain that interacts with phosphorylated Ser2 and Ser5 of the Pol2 CTD. Hence, this post-translational modification of H3K36 is a mark left by the passage of Pol2. Truncating the SRI domain abolishes both H3K36me2 and H3K36me3 in budding yeast;Citation4 consequently, interaction between Set2 and Pol2 is necessary for proper distribution of H3K36me along the gene body. Our previous reports showed that in fission yeast, Set2 is the sole enzyme for H3K36meCitation25. Fission yeast Set2 also has an SRI domain, which we have shown to be essential for the interaction between Set2 and the Pol2 CTD.Citation25,26 Surprisingly, Set2 lacking the SRI domain (set2SRI) shows loss of overall H3K36me3, while H3K36me2 level is comparable to the wild type.Citation25 This indicates that in fission yeast, interaction between Set2 and the Pol2 CTD is essential for trimethylation but not dimethylation of H3K36 (). The different findings in fission and budding yeast imply that distinct mechanisms of H3K36me by Set2 exist in this organism.
Figure 1. Model of Set2-dependent gene silencing. At the transcriptional initiation step, Set2 is recruited to chromatin independently of Pol2 CTD, and methylates H3K36 up to dimethylation. At the elongation step, Pol2 CTD S2 and S5 are phosphorylated, and Set2 interacts with Pol2 CTD to co-transcriptionally methylate H3K36 up to trimethylation along the gene body. This leads to post-transcriptional gene silencing in heterochromatin and contributes to transcriptional gene silencing in the subtelomeric region.
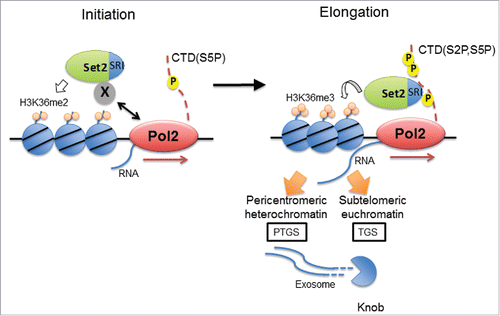
ChIP-sequencing (ChIP-seq) analyses of H3K36me2 and H3K36me3 have been carried out to clarify H3K36me patterns of in set2SRI cells in fission yeast. H3K36me2 signals were found to be highly enriched and widely distributed within the gene body, similar to the pattern of H3K36me3 observed in Set2 wild-type cells. Meanwhile, ChIP-seq analyses of Set2 and Set2SRI have revealed that both proteins are enriched at the gene body. These data suggest that Set2 is recruited to chromatin by proteins other than Pol2 and modifies H3K36 up to dimethylation, since H3K36me2 remained enriched along the gene body in the absence of Pol2 CTD interaction in set2SRI cells. Thus, Set2—independent of the SRI domain—associates with Pol2 via an unknown protein for H3K36 dimethylation at the beginning of transcription; during transcriptional elongation, Set2 interacts with phosphorylated Pol2 CTD via the SRI domain to induce switching from H3K36me2 to H3K36me3 ().
Higher eukaryotes have multiple H3K36 methyltransferases with distinct H3K36 mono-, di-, and tri-methylation activities, although their physiological roles are not fully known. But a recent report exhibited that transcriptional interference of the sense gene of a sense-antisense convergent gene reporter was coupled with H3K36me3, which was enriched by the antisense promoter activation.Citation27 The maintain system of this transcriptional interference of the sense gene changes depending on the state of the cell. H3K36me3 is promptly removed by the inactivation of the antisense promoter in ES cells, but it is maintained even after the inactivation of the antisense promoter to repress the transcription from the sense strand as a transcriptional memory in differentiating cells.Citation27 In fission yeast, Set2 is the sole methyltransferase that produces all methylation states of H3K36. We found that in set2SRI cells, H3K36me3 was abolished while H3K36me2 was unaffected.Citation25 Based on this observation, we examined functional differences between H3K36me3 and H3K36me2 in terms of transcriptional repression. A transcriptome analysis revealed that in set2 cells, many genes were upregulated while small number were downregulated, indicating that H3K36me mainly functions to silence genes. We also found that derepressed genes in set2SRI cells substantially overlapped (79.4%) with those in set2 cells. For example, two typical upregulated genes—SPBPB2B2.06c and SPBPB2B2.08—were located in subtelomeric regions, where the “knob” chromatin structure is formed in an H3K36me-dependent manner.Citation2 Pol2 occupancy at these loci was increased by loss of H3K36me3, indicating that transcriptional gene silencing is the pre-dominant mechanism for repression. In budding yeast, Set2 prevents the synthesis of cryptic transcripts from intragenic regions, H3K36me2 was found to be sufficient for the recruitment of Rpd3S HDAC to repress cryptic transcription.Citation28 These results suggest that in fission yeast there are other gene silencing mechanisms besides the one involving HDAC (which specifically recognizes H3K36me2 and H3K36me3 as in metazoans), we speculate that they include novel reader proteins that preferentially recognize H3K36me3 over H3K36me2.
3′ →5′ exosome pathway and H3K36me3
Chromosomes contain many highly repetitive sequences. For example, satellite repeats at the pericentromere, terminal repeats at the telomere, and interspersed repeats at transposons are prevalent in eukaryotes. In many cases, these loci exist as heterochromatin to maintain chromosome homeostasis. In heterochromatic regions, transcription is repressed by heterochromatin protein 1 (HP1) and its effectors, which involves H3K9 methylation (H3K9me) as an epigenetic silencing mark.Citation29 After establishing de novo heterochromatin, the RNA interference (RNAi) machinery generates short interfering (si)RNA from heterochromatic non-coding RNA transcribed by Pol2 in fission yeast.Citation30 The RNA-induced transcriptional silencing (RITS) complex captures siRNAs, which are used to recruit Clr4 H3K9 methyltransferase complex to heterochromatin to spread and/or maintain H3K9me during cell proliferation, differentiation, and adaptation.Citation31 In addition to the RNAi machinery, the exosome has been implicated in heterochromatic non-coding RNA processing.Citation32
Set2 functions in the heterochromatic region to repress transcription.Citation33 We also found that H3K36me3 was present in heterochromatin ().Citation25 However, H3K9 methylation levels were unchanged in set2 cells, indicating that heterochromatin structure was also unaffected. Moreover, deleting dcr1+ gene, an RNAi factor, in set2 cells induced additive heterochromatin silencing defectsCitation25, indicating that the RNAi and Set2 pathways act independently but possibly in parallel in this process. Previous studies have also implicated Clr6 HDAC—a paralog of budding yeast Rpd3S HDAC—in heterochromatic silencing;Citation34 Clr6 HDAC mutant cells had higher levels of acetylated histone H3K9 and H3K14 in heterochromatin regions. However, this was not the case in set2 cells, indicating that Clr6 HDAC does not associate with Set2 for heterochromatin silencing.
We also demonstrated that Set2-dependent heterochromatic silencing is related to the exosome pathway, which eliminates unnecessary RNA in cells.Citation25 Rrp6 is a 3′→5′ exoribonuclease—i.e., a catalytic subunit in the exosome system that is required for heterochromatin formation.Citation35 rrp6 set2 double-mutant cells exhibited the same heterochromatic silencing defect as single mutants, this suggests that Set2 is genetically linked to the exosome pathway, although the molecular basis for this relationship remains obscure.
We previously reported that at the transcription initiation step, Set2 associates with an unknown factor and is thereby recruited to the transcription start site to methylate H3K36 up to dimethylation (). During the elongation phase, the Pol2 CTD is phosphorylated at S2 and S5, Set2 interacts with these phosphorylated residues via its SRI domain and methylates H3K36 up to trimethylation in gene bodies. Set2 represses numerous antisense and coding RNAs transcribed from subtelomeric euchromatin, where “knobs” are formed. “Knobs” are defined as chromosome regions that have condensed and silent chromatin structure but distinct from heterochromatin and Set2 contributes, at least in part, to the formation of “knob.”Citation2 Moreover, Set2 cooperates with the exosome pathway for heterochromatic silencing to exert transcriptional repression of subtelomeric genes. Further analysis is required in order to clarify the mechanisms linking H3K36me3, “knob” formation, and the exosome pathway for gene silencing in fission yeast.
Disclosure of potential conflicts of interest
No potential conflicts of interest were disclosed.
Funding
This work was supported by a Grant-in-Aid for Scientific Research (A) from the Japan Society for the Promotion of Science (JSPS; no. 25250022 to Y.M.), a Grant-in-Aid for Scientific Research (C) from JSPS (no. 26440001 to S.T.), and a Grant-in-Aid for Scientific Research on Innovative Areas (Transcription Cycle) from the Ministry of Education, Culture, Sports, Science, and Technology of Japan (no. 15H01339 to S.T.).
References
- Wagner EJ, Carpenter PB. Understanding the language of Lys36 methylation at histone H3. Nat Rev Mol Cell Biol 2012; 13:115-126; PMID:22266761; http://dx.doi.org/10.1038/nrm3274
- Matsuda A, Chikashige Y, Ding DQ, Ohtsuki C, Mori C, Asakawa H, Kimura H, Haraguchi T, Hiraoka Y. Highly condensed chromatins are formed adjacent to subtelomeric and decondensed silent chromatin in fission yeast. Nat Commun 2015; 6:7753; PMID:26205977; http://dx.doi.org/10.1038/ncomms8753
- Kizer KO, Phatnani HP, Shibata Y, Hall H, Greenleaf AL, Strahl BD. A novel domain in Set2 mediates RNA polymerase II interaction and couples histone H3 K36 methylation with transcript elongation. Mol Cell Biol 2005; 25:3305-3316; PMID:15798214; http://dx.doi.org/10.1128/MCB.25.8.3305-3316.2005
- Youdell ML, Kizer KO, Kisseleva-Romanova E, Fuchs SM, Duro E, Strahl BD, Mellor J. Roles for Ctk1 and Spt6 in regulating the different methylation states of histone H3 lysine 36. Mol Cell Biol 2008; 28:4915-4926; PMID:18541663; http://dx.doi.org/10.1128/MCB.00001-08
- Biswas D, Takahata S, Stillman DJ. Different genetic functions for the Rpd3(L) and Rpd3(S) complexes suggest competition between NuA4 and Rpd3(S). Mol Cell Biol 2008; 28:4445-4458; PMID:18490440; http://dx.doi.org/10.1128/MCB.00164-08
- Jha DK, Strahl BD. An RNA polymerase II-coupled function for histone H3K36 methylation in checkpoint activation and DSB repair. Nat Commun 2014; 5:3965; PMID:24910128; http://dx.doi.org/10.1038/ncomms4965
- Pai CC, Deegan RS, Subramanian L, Gal C, Sarkar S, Blaikley EJ, Walker C, Hulme L, Bernhard E, Codlin S et al. A histone H3K36 chromatin switch coordinates DNA double-strand break repair pathway choice. Nat Commun 2014; 5:4091; PMID:24909977; http://dx.doi.org/10.1038/ncomms5091
- Zhang Y, Xie S, Zhou Y, Xie Y, Liu P, Sun M, Xiao H, Jin Y, Sun X, Chen Z et al. H3K36 histone methyltransferase Setd2 is required for murine embryonic stem cell differentiation toward endoderm. Cell Rep 2014; 8:1989-2002; PMID:25242323; http://dx.doi.org/10.1016/j.celrep.2014.08.031
- Carrozza MJ, Li B, Florens L, Suganuma T, Swanson SK, Lee KK, Shia WJ, Anderson S, Yates J, Washburn MP et al. Histone H3 methylation by Set2 directs deacetylation of coding regions by Rpd3S to suppress spurious intragenic transcription. Cell 2005; 123:581-592; PMID:16286007; http://dx.doi.org/10.1016/j.cell.2005.10.023
- Lickwar CR, Rao B, Shabalin AA, Nobel AB, Strahl BD, Lieb JD. The Set2/Rpd3S pathway suppresses cryptic transcription without regard to gene length or transcription frequency. PLoS One 2009; 4:e4886; PMID:19295910; http://dx.doi.org/10.1371/journal.pone.0004886
- Dhayalan A, Rajavelu A, Rathert P, Tamas R, Jurkowska RZ, Ragozin S, Jeltsch A. The Dnmt3a PWWP domain reads histone 3 lysine 36 trimethylation and guides DNA methylation. J Biol Chem 2010; 285:26114-26120; PMID:20547484; http://dx.doi.org/10.1074/jbc.M109.089433
- Hahn MA, Wu X, Li AX, Hahn T, Pfeifer GP. Relationship between gene body DNA methylation and intragenic H3K9me3 and H3K36me3 chromatin marks. PLoS One 2011; 6:e18844; PMID:21526191; http://dx.doi.org/10.1371/journal.pone.0018844
- Brien GL, Gambero G, O'Connell DJ, Jerman E, Turner SA, Egan CM, Dunne EJ, Jurgens MC, Wynne K, Piao L et al. Polycomb PHF19 binds H3K36me3 and recruits PRC2 and demethylase NO66 to embryonic stem cell genes during differentiation. Nat Struct Mol Biol 2012; 19:1273-1281; PMID:23160351; http://dx.doi.org/10.1038/nsmb.2449
- Cai L, Rothbart SB, Lu R, Xu B, Chen WY, Tripathy A, Rockowitz S, Zheng D, Patel DJ, Allis CD et al. An H3K36 methylation-engaging Tudor motif of polycomb-like proteins mediates PRC2 complex targeting. Mol Cell 2013; 49:571-582; PMID:23273982; http://dx.doi.org/10.1016/j.molcel.2012.11.026
- Zhang P, Du J, Sun B, Dong X, Xu G, Zhou J, Huang Q, Liu Q, Hao Q, Ding J. Structure of human MRG15 chromo domain and its binding to Lys36-methylated histone H3. Nucleic Acids Res 2006; 34:6621-6628; PMID:17135209; http://dx.doi.org/10.1093/nar/gkl989
- Li Q, Lee JA, Black DL. Neuronal regulation of alternative pre-mRNA splicing. Nat Rev Neurosci 2007; 8:819-831; PMID:17895907; http://dx.doi.org/10.1038/nrn2237
- Luco RF, Pan Q, Tominaga K, Blencowe BJ, Pereira-Smith OM, Misteli T. Regulation of alternative splicing by histone modifications. Science 2010; 327:996-1000; PMID:20133523; http://dx.doi.org/10.1126/science.1184208
- Pradeepa MM, Sutherland HG, Ule J, Grimes GR, Bickmore WA. Psip1/Ledgf p52 binds methylated histone H3K36 and splicing factors and contributes to the regulation of alternative splicing. PLoS Genet 2012; 8:e1002717; PMID:22615581; http://dx.doi.org/10.1371/journal.pgen.1002717
- Shi X, Kachirskaia I, Walter KL, Kuo JH, Lake A, Davrazou F, Chan SM, Martin DG, Fingerman IM, Briggs SD et al. Proteome-wide analysis in Saccharomyces cerevisiae identifies several PHD fingers as novel direct and selective binding modules of histone H3 methylated at either lysine 4 or lysine 36. J Biol Chem 2007; 282:2450-2455; PMID:17142463; http://dx.doi.org/10.1074/jbc.C600286200
- Tsukada Y, Fang J, Erdjument-Bromage H, Warren ME, Borchers CH, Tempst P, Zhang Y. Histone demethylation by a family of JmjC domain-containing proteins. Nature 2006; 439:811-816; PMID:16362057; http://dx.doi.org/10.1038/nature04433
- Whetstine JR, Nottke A, Lan F, Huarte M, Smolikov S, Chen Z, Spooner E, Li E, Zhang G, Colaiacovo M et al. Reversal of histone lysine trimethylation by the JMJD2 family of histone demethylases. Cell 2006; 125:467-481; PMID:16603238; http://dx.doi.org/10.1016/j.cell.2006.03.028
- He J, Kallin EM, Tsukada Y, Zhang Y. The H3K36 demethylase Jhdm1b/Kdm2b regulates cell proliferation and senescence through p15(Ink4b). Nat Struct Mol Biol 2008; 15:1169-1175; PMID:18836456; http://dx.doi.org/10.1038/nsmb.1499
- Crona F, Dahlberg O, Lundberg LE, Larsson J, Mannervik M. Gene regulation by the lysine demethylase KDM4A in Drosophila. Dev Biol 2013; 373:453-463; PMID:23195220; http://dx.doi.org/10.1016/j.ydbio.2012.11.011
- Strahl BD, Grant PA, Briggs SD, Sun ZW, Bone JR, Caldwell JA, Mollah S, Cook RG, Shabanowitz J, Hunt DF et al. Set2 is a nucleosomal histone H3-selective methyltransferase that mediates transcriptional repression. Mol Cell Biol 2002; 22:1298-1306; PMID:11839797; http://dx.doi.org/10.1128/MCB.22.5.1298-1306.2002
- Suzuki S, Kato H, Suzuki Y, Chikashige Y, Hiraoka Y, Kimura H, Nagao K, Obuse C, Takahata S, Murakami Y. Histone H3K36 trimethylation is essential for multiple silencing mechanisms in fission yeast. Nucleic Acids Res 2016; 44:4147-4162; PMID:26792892; http://dx.doi.org/10.1093/nar/gkw008
- Suzuki S, Nagao K, Obuse C, Murakami Y, Takahata S. A novel method for purification of the endogenously expressed fission yeast Set2 complex. Protein Expr Purif 2014; 97:44-49; PMID:24583182; http://dx.doi.org/10.1016/j.pep.2014.02.005
- Loos F, Loda A, van Wijk L, Grootegoed JA, Gribnau J. Chromatin-mediated reversible silencing of sense-antisense gene pairs in embryonic stem cells is consolidated upon differentiation. Mol Cell Biol 2015; 35:2436-2447; PMID:25963662; http://dx.doi.org/10.1128/MCB.00029-15
- Li B, Gogol M, Carey M, Lee D, Seidel C, Workman JL. Combined action of PHD and chromo domains directs the Rpd3S HDAC to transcribed chromatin. Science 2007; 316:1050-1054; PMID:7510366; http://dx.doi.org/10.1126/science.1139004
- Nishibuchi G, Nakayama J. Biochemical and structural properties of heterochromatin protein 1: understanding its role in chromatin assembly. J Biochem 2014; 156:11-20; PMID:24825911; http://dx.doi.org/10.1093/jb/mvu032
- Kato H, Goto DB, Martienssen RA, Urano T, Furukawa K, Murakami Y. RNA polymerase II is required for RNAi-dependent heterochromatin assembly. Science 2005; 309:467-469; PMID:15947136; http://dx.doi.org/10.1126/science.1114955
- Bayne EH, White SA, Kagansky A, Bijos DA, Sanchez-Pulido L, Hoe KL, Kim DU, Park HO, Ponting CP, Rappsilber J et al. Stc1: a critical link between RNAi and chromatin modification required for heterochromatin integrity. Cell 2010; 140:666-677; PMID:20211136; http://dx.doi.org/10.1016/j.cell.2010.01.038
- Zofall M, Yamanaka S, Reyes-Turcu FE, Zhang K, Rubin C, Grewal SI. RNA elimination machinery targeting meiotic mRNAs promotes facultative heterochromatin formation. Science 2012; 335:96-100; PMID:22144463; http://dx.doi.org/10.1126/science.1211651
- Chen ES, Zhang K, Nicolas E, Cam HP, Zofall M, Grewal SI. Cell cycle control of centromeric repeat transcription and heterochromatin assembly. Nature 2008; 451:734-737; PMID:18216783; http://dx.doi.org/10.1038/nature06561
- Nicolas E, Yamada T, Cam HP, Fitzgerald PC, Kobayashi R, Grewal SI. Distinct roles of HDAC complexes in promoter silencing, antisense suppression and DNA damage protection. Nat Struct Mol Biol 2007; 14:372-380; PMID:17450151; http://dx.doi.org/10.1038/nsmb1239
- Oya E, Kato H, Chikashige Y, Tsutsumi C, Hiraoka Y, Murakami Y. Mediator directs co-transcriptional heterochromatin assembly by RNA interference-dependent and -independent pathways. PLoS Genet 2013; 9:e1003677; PMID:23966866; http://dx.doi.org/10.1371/journal.pgen.1003677