ABSTRACT
In Saccharomyces cerevisiae, a group of more than 200 co-regulated genes (Ribi genes) is involved in ribosome biogenesis. This regulon has recently been shown to rely on a small set of transcriptional regulators (mainly Abf1, but also Reb1, Tbf1 and Rap1) previously referred to as general regulatory factors (GRFs) because of their widespread binding and action at many promoters and other specialized genomic regions. Intriguingly, Abf1 binding to Ribi genes is differentially modulated in response to distinct nutrition signaling pathways. Such a dynamic promoter association has the potential to orchestrate both activation and repression of Ribi genes in synergy with neighboring regulatory sites and through the functional interplay of histone acetyltransferases and deacetylases.
Introduction
Ribosome biogenesis, a core process in cell growth, is subjected to strict regulation. In Saccharomyces cerevisiae this occurs mainly through rigorous transcriptional control. Indeed, various nutrient and stress signaling pathways regulate the transcription of 138 ribosomal protein (RP) genes, coding for structural protein components of cytoplasmic ribosomes, and of 200–250 genes whose products are required for the biogenesis of ribosomes without becoming a structural part of them (Ribi genes).Citation1 RP and Ribi genes have long been recognized as among the largest regulons in yeast cells.Citation2-5
RP gene promoter architecture and transcription regulatory mechanisms have been investigated in great detail, and shown to involve either the Rap1 (for most promoters) or Abf1 general regulatory factors (GRFs). Both Rap1 and Abf1 play the role of sequence-specific DNA binding activators. Their action at RP promoters is exerted in cooperation with several other positively (Fhl1, Ihf1, Hmo1, Sfp1) or negatively (Crf1, Stb3) acting transcription proteins, whose mechanisms have especially been addressed and clarified at Rap1-dependent RP promoters. These additional regulators generally require promoter-bound GRF for recruitment and, with the exception of Fhl1, are modulated in their promoter association and activity by the two major nutrient-sensing kinase pathways, target of rapamycin complex 1 (TORC1) and protein kinase A (PKA), but also by casein kinase 2.Citation1,6-11 According to a well-established paradigm, Rap1 is necessary for Fhl1 recruitment. Under rich growth conditions, Fhl1 in turn recruits the transcriptional activator Ifh1 to drive gene expression. Nutrient starvation affects Ifh1 recruitment to RP promoters, thus causing transcription downregulation without any effect on Rap1 and Fhl1 promoter occupancy.Citation1 In contrast, at Abf1-dependent RP promoters nutritional perturbation may lead to increased Abf1 occupancy (see below).Citation11
Until recently, our knowledge about Ribi gene regulatory mechanisms was somewhat less complete than the one of RP regulon, because it exclusively focused on the well established presence of two negatively acting promoter elements, PAC (for polymerase A and C) and RRPE (for rRNA processing element), at positions ranging from −50 to −125 with respect to the transcription start sites of Ribi genes.Citation3,Citation12 Several studies in the last 10 y have shown that these elements act as recognition sites for transcriptional repressor proteins: Stb3, binding to the RRPE,Citation13,Citation14 and the two Myb-like HTH transcription factors Dot6 and Tod6, recognizing sequence-specifically the PAC element (reviewed in ref.Citation1). Promoter-bound Stb3 and Dot6/Tod6 are thought to recruit the histone deacetylase Rpd3L thereby leading to transcriptional downregulation under nutritional stress conditions. Citation1,Citation9
This relatively simple picture has been recently expanded by a study showing that most Ribi gene promoters are demarcated, at positions ranging from 250 to 50 bp upstream of PAC and RRPE, by one or more binding sites for the GRFs Abf1, Reb1, Tbf1 and Rap1.Citation15 All of these proteins possess a Myb-like DNA-binding domain, bind to several hundreds of promoter regions in the S. cerevisiae genome and are multifunctional, being involved in processes other than transcription initiation, such as DNA repair,Citation16,Citation17 telomere regulation,Citation18,Citation19 and transcription termination.Citation20 The observation that all the yeast regulons involved in rRNA maturation and ribosome production (including snoRNA genes in addition to RP and Ribi genesCitation21) share GRF-associated promoters raises questions about the physiologic significance of these transcription factors in regulon modulation according to cell growth conditions. Here, we provide an account of recent experimental observations and we suggest a speculative scenario hopefully helping to re-evaluate the key role of GRFs, especially Abf1, in yeast regulatory responses involving global reprogramming of transcription and chromatin structure.
Modulation of GRF association with RP and Ribi gene promoters by nutrient signaling pathways
Among the GRFs, Abf1 has the potential for playing a key role in the transcriptional regulation of yeast ribosome biogenesis, in that it binds not only to the 40–50% of Ribi gene promotersCitation15 but also to a small group of RP gene promoters.Citation11 In addition, there is evidence for Abf1 association with a significant fraction of mitochondrial ribosomal protein gene promoters.Citation22 In contrast with a common idea of Abf1 as permanently bound at promoters, where it would counteract nucleosome deposition and favor the action of other more tunable transcription factors, the study of its behavior at RP and Ribi gene promoters has revealed that Abf1-promoter association changes dramatically in response to certain nutritional signals. In particular, inhibition of the nitrogen-sensitive TORC1 pathway by rapamycin, which causes a quick and dramatic decrease in both RP and Ribi gene transcription, was found to produce a parallel, large increase in Abf1 association with both types of promotersCitation11,Citation15 (see , upper panel). Such an unpredicted behavior makes Abf1 to resemble the large number of tunable transcriptional regulators whose association with promoters is modulated in response to environmental stimuli.Citation23 As the expression levels of Abf1 do not change in response to rapamycin,Citation11 nor does its subcellular localization, which tends to remain constantly nuclear,Citation24 the regulated binding of Abf1 to RP and Ribi gene promoters is likely to depend on a change in its DNA binding properties, or in its interaction with DNA binding partners, possibly due to a change in its covalent modification (e.g., phosphorylation) status. According to such hypothesis, Abf1 has long been known to be a phosphoprotein, with at least four different phosphorylation states whose balance varies in response to nutrient availability.Citation25 Moreover, a recent phosphoproteomic study identified Abf1 as a candidate proximal target of TORC1.Citation26 Based on biochemical evidence, casein kinase 2 (CK2) has been implicated in Abf1 phosphorylation.Citation27 It is thus possible that TORC1 influences the phosphorylation state of Abf1 through CK2, whose association with some of its substrates has recently been proposed to be modulated by TORC1 via its effector kinase Kns1.Citation28
Figure 1. Abf1-dependent changes at Ribi gene promoters in response to nutrient signaling. Partially speculative scheme illustrating the changes in transcription factor interactions, histone acetylation and transcription activity of Abf1-dependent Ribi genes in response to TORC1 inactivation (upper panel) or glucose starvation (lower panel). The different shapes and colors of Abf1 refer to its different phosphorylation states that may predominate under certain conditions. SAGA could support gene activation in the absence of promoter-bound Abf1 (see text). The possibility of an Rpd3L-independent pathway of repression, which might involve Stb3 and Dot6 only, in the absence of bound Abf1, is labeled with an interrogation mark to indicate its purely speculative character. Such a pathway would help to explain how full repression could be attained even at those promoters that do not carry bound Abf1 and would thus be incapable of Rpd3L recruitment. Nucleosomes located upstream and downstream of the nucleosome-free region are indicated (−1, +1). For simplicity of representation, the cis-acting elements recognized by Abf1, Stb3 (RRPE element) and Dot6/Tod6 (PAC element) are not represented on the DNA; their relative positions are approximately indicated by the positions of the corresponding DNA-binding proteins.
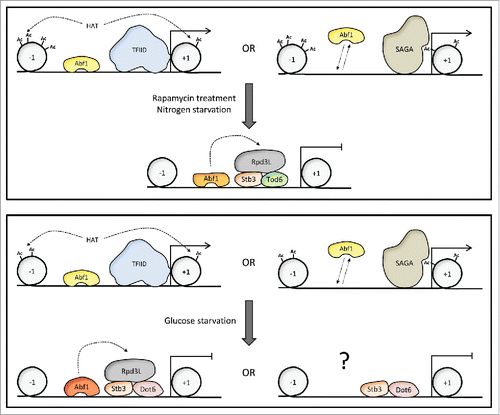
Modulation of the association of Abf1 with a target RP gene promoter (RPS28B) has also been observed in correlation with the different phases of yeast cell culture growth. Increased Abf1 occupancy of this promoter was observed in going from exponential, to post-diauxic to stationary phase, concomitant with a progressive reduction in mRNA expression. Upon dilution into fresh medium the expected rescue of transcription rate was accompanied by a progressive decrease in Abf1–promoter association.Citation11
Given the role of Abf1 as a transcriptional activator of both RP and Ribi genes under rich medium conditions,Citation11,Citation15 why should repressive nutritional stimuli (TORC1 inactivation, stationary phase) lead to increased Abf1 recruitment? Is Abf1 acting both as an activator and as a repressor, with either of the two roles predominating under certain growth conditions? While the possibility remains that Abf1 participates in transcription repression mechanisms in response to nutrient starvation (see below), experiments with an rps28b promoter mutant unable to bind Abf1 suggested that increased Abf1 occupancy under restrictive conditions allows for more prompt recovery of transcription once more permissive nutritional conditions are re-established.Citation11 The overall increase of Abf1 occupancy at RP and Ribi genes upon TORC1 inactivation is likely to be related to the peculiar behavior of Abf1 at some of its target promoters, where this GRF needs not to be constantly bound in order for the activated state to be maintained.Citation29 It can be speculated that, during exponential growth in rich media, Abf1-dependent RP and Ribi gene promoters are to some extent unoccupied by this transcriptional regulator, yet fully active, thus leaving room for an increase in Abf1 occupancy in response to starvation.
It must be pointed out that, based on current literature, the other GRFs binding to RP and/or Ribi gene promoters have not been shown to undergo nutrient-regulated changes in their promoter occupancy. While this possibility has been experimentally addressed and excluded for Rap1, at least under some conditions,Citation30,Citation31 it was not explored in the case of Tbf1 and Reb1. Moreover, interestingly, Abf1 association with Ribi gene promoters proved to be modulated to variable extents in response to different types of nutritional stimuli. In particular, glucose starvation, known to induce a rapid drop followed by slow recovery in Ribi gene expression, did not result in any significant change in Abf1 occupancy at their promotersCitation15 (, lower panel). The response of Ribi genes to glucose starvation is likely to depend on the cAMP-protein kinase A signaling pathway, with a specific involvement of the PAC-binding repressor Dot6, while the response to rapamycin, more representative of nitrogen starvation conditions, relies more on inactivation of TORC1 and appears to involve preferentially the Tod6 repressor.Citation32 Again, differences in Abf1–promoter association dynamics in response to different types of nutritional stress might be accounted for by distinct changes in Abf1 phosphorylation states induced by different types of nutritional cues.
Roles of GRFs in nutrient-regulated changes of histone acetylation at RP and Ribi gene promoters
It has previously been established that activation of S. cerevisiae RP genes entails the recruitment of the histone acetyl-transferase (HAT) complex NuA4, targeting histones H4 and H2A, and that NuA4 recruitment requires promoter-bound GRFCitation33 and is dependent on TOR signaling.Citation34 In response to rapamycin treatment or nitrogen starvation, RP genes were shown to undergo a repression process that involves the Rpd3 histone deacetylase (HDAC).Citation34 This particular HDAC only was found to mediate rapamycin-dependent downregulation of RP and other genes, through a mechanism implicating rapamycin-induced recruitment of Rpd3 to target genes.Citation35 A subsequent study greatly extended these observations by showing that the involved HDAC complex is Rpd3L (one of the two functionally distinct Rpd3 complexes), and that Rpd3L is recruited to both RP and Ribi genes in response to TORC1-controlled Sch9 kinase inhibition.Citation9 As shown by the same study, Rpd3L recruitment is operated through the cooperation of Tod6/Dot6 and Stb3 for Ribi genes, and predominantly through Stb3 for RP genes, with these repressive transcription factors being hypo-phosphorylated following Sch9 inhibition.Citation9 More recently, Rpd3 has been shown to be the key mediator of global transcriptional shutoff during quiescence entry. In quiescent budding yeast, this HDAC is targeted via repressors including Stb3 to half of gene promoters, where it mediates repressive chromatin changes.Citation36
Of relevance to the mechanistic understanding of nutrient-dependent Rpd3 recruitment to promoters, we have recently observed that promoter-bound Abf1 is required for Rpd3L recruitment at the Ribi gene LTV1 in response to glucose depletion.Citation15 In this case, thus, a GRF acting as an activator under rich medium conditions is also required for recruitment of the main chromatin-modifying complex mediating transcriptional repression (). Such a dual function of a transcriptional regulator as an activator and as a repressor within the same promoter context (certainly not unprecedented; see ref.Citation37 as a recent example), and the widespread presence of GRFs at the majority of yeast promoters, lead to speculate that GRFs might play two major roles at their target promoters genome wide. This is exemplified in for the case of Ribi genes recognized by Abf1. On the one hand, in the presence of abundant resources, GRFs would allow promoters (especially those of growth-related genes) to be fully active and thus, as observed for RP and Ribi gene promoters, fully repressible upon resource starvation.Citation11,Citation15 On the other hand, promoter-bound GRFs would predispose growth-related promoters to global targeting by Rpd3 HDAC, a process occurring in response to unfavorable growth conditions with the participation of more specialized repressor proteins (such as Stb3 and Tod6/Dot6). As exemplified by Abf1-dependent Ribi genes, the GRF would thus play a pivotal role in the reversible switching between alternative histone acetylation states accompanying the transcriptional response of growth-related genes to changing nutritional conditions (). Under nutrient abundance, the GRF would promote histone acetylation through direct or indirect recruitment of histone acetyltransferase complexes such as NuA433 or SAGA. Even though RP genes and Ribi genes are mostly TATA-less and thus considered to be TFIID-dominated and predominantly acetylated by NuA4 HAT,Citation38 there is evidence that SAGA recruitment can also occur at these promoters.Citation39 One could speculate that, in the absence of promoter-bound Abf1 (a situation that might occur under favorable growth conditions, see above), SAGA could be recruited, via its bromodomain, to nucleosomes that have been previously acetylated by NuA4,Citation40 thus maintaining a hyper-acetylated state even in the absence of Abf1. Upon nutrient starvation, residual promoter-bound Abf1 would help the transition to hypo-acetylated chromatin by favoring Rpd3L recruitment to Ribi (and a few RP) genes; in the particular case of nitrogen starvation (mimicked by TORC1 inactivation), such a chromatin transition would further be forced by increased Abf1 occupancy. Relevant to this point, genetic interactions have recently been reported between ABF1 (but also RAP1, REB1) and genes coding for Rpd3 components.Citation41 Upon nutrient replenishment, promoter-bound Abf1 would support the transcriptional rescue of Abf1-dependent RP and Ribi promoters, perhaps by switching from a form favoring the recruitment of Rpd3 to a form more favorable to HAT recruitment/activity. It is worth noting that the idea of promoter-bound GRF switching between a form favoring histone acetylation and a form facilitating the recruitment of histone deacetylase is also consistent with the reversible recruitment of chromatin modifying factors observed at Rap1-dependent RP promoters,Citation9,Citation34 even though the requirement of promoter-bound Rap1 for Rpd3L recruitment has still to be demonstrated.
Perspective
Although the above speculation mainly derives from studies focused on the subset of RP and Ribi genes regulated by Abf1, some of its aspects might also be relevant for Rap1-dependent RP genes and for Ribi genes whose promoters are bound by Reb1 or Tbf1. It will thus be interesting to systematically explore the response of yeast cells exposed to different nutritional perturbations and other types of stress, as well as to exit from stress, in terms of GRF-promoter association and HAT/HDAC reversible recruitment at RP and Ribi gene promoters. The related issue of changes in Abf1 phosphorylation state under different conditions clearly also awaits further investigations, as do similar issues concerning the other GRFs mentioned in this article, all reported to be phosphoproteins.Citation22 Other questions that are still largely unanswered concern the mechanisms of co-regulation and possible cross-talk at different levels: (i) Within the Ribi regulon: are there functional differences between Ribi promoters demarcated by different GRFs? Does altered expression of one Ribi gene also influence the expression of other members of the same regulon? (ii) Between the Ribi regulon and other regulons involved in ribosome biogenesis: are there specific regulatory features that are shared by Abf1-bound Ribi genes and the small group of Abf1-bound RP genes? And are there other regulatory features shared by Tbf1-bound Ribi genes and the majority of snoRNA genes, whose promoters are demarcated by the same transcription factor21? The recent discovery of the elegant molecular titration system coordinating RP gene transcription with rRNA synthesisCitation42 clearly points to the existence of sophisticated cross-talk mechanisms controlling under each growth condition the balanced supply of all components required for ribosome assembly and function.
Disclosure of potential conflicts of interest
No potential conflicts of interest were disclosed.
Acknowledgments
We thank Rodolfo Negri (Sapienza University of Rome) and Gloria Spagnoli and Elisabetta Levati (University of Parma) for discussions and support.
Funding
The work in authors' laboratory is supported by Italian Association for Cancer Research (AIRC, Grant IG16877 to G.D.).
References
- Broach JR. Nutritional control of growth and development in yeast. Genetics 2012; 192:73-105; PMID:22964838; https://doi.org/10.1534/genetics.111.135731
- Warner JR. The economics of ribosome biosynthesis in yeast. Trends Biochem Sci 1999; 24:437-440; PMID:10542411; https://doi.org/10.1016/S0968-0004(99)01460-7
- Wade C, Shea KA, Jensen RV, McAlear MA. EBP2 is a member of the yeast RRB regulon, a transcriptionally coregulated set of genes that are required for ribosome and rRNA biosynthesis. Mol Cell Biol 2001; 21:8638-8650; PMID:11713296; https://doi.org/10.1128/MCB.21.24.8638-8650.2001
- Jorgensen P, Rupes I, Sharom JR, Schneper L, Broach JR, Tyers M. A dynamic transcriptional network communicates growth potential to ribosome synthesis and critical cell size. Genes Dev 2004; 18:2491-2505; PMID:15466158; https://doi.org/10.1101/gad.1228804
- Wade CH, Umbarger, MA, McAlear, MA. The budding yeast rRNA and ribosome biosynthesis (RRB) regulon contains over 200 genes. Yeast 2006; 23:293-306; PMID:16544271; https://doi.org/10.1002/yea.1353
- Knight B, Kubik S, Ghosh B, Bruzzone MJ, Geertz M, Martin V, Denervaud N, Jacquet P, Ozkan B, Rougemont J et al. Two distinct promoter architectures centered on dynamic nucleosomes control ribosomal protein gene transcription. Genes Dev 2014; 28:1695-1709; PMID:25085421; https://doi.org/10.1101/gad.244434.114
- Reja R, Vinayachandran V, Ghosh S, Pugh BF. Molecular mechanisms of ribosomal protein gene coregulation. Genes Dev 2015; 29:1942-1954; PMID:26385964; https://doi.org/10.1101/gad.268896.115
- Kim MS, Hahn JS. Role of CK2-dependent phosphorylation of Ifh1 and Crf1 in transcriptional regulation of ribosomal protein genes in Saccharomyces cerevisiae. Biochim Biophys Acta 2016; 1859:1004-1013; PMID:27321754; https://doi.org/10.1016/j.bbagrm.2016.06.003
- Huber A, French SL, Tekotte H, Yerlikaya S, Stahl M, Perepelkina MP, Tyers M, Rougemont J, Beyer AL, Loewith R. Sch 9 regulates ribosome biogenesis via Stb3, Dot6 and Tod6 and the histone deacetylase complex RPD3L. EMBO J 2011; 30:3052-3064; PMID:21730963; https://doi.org/10.1038/emboj.2011.221
- Bosio MC, Negri R, Dieci G. Promoter architectures in the yeast ribosomal expression program. Transcription 2011; 2:71-77; PMID:21468232; https://doi.org/10.4161/trns.2.2.14486
- Fermi B, Bosio MC, Dieci G. Promoter architecture and transcriptional regulation of Abf1-dependent ribosomal protein genes in Saccharomyces cerevisiae. Nucleic Acids Res 2016; 44:6113-6126; PMID:27016735; https://doi.org/10.1093/nar/gkw194
- Hughes JD, Estep PW, Tavazoie S, Church GM. Computational identification of cis-regulatory elements associated with groups of functionally related genes in Saccharomyces cerevisiae. J Mol Biol 2000; 296:1205-1214; PMID:10698627; https://doi.org/10.1006/jmbi.2000.3519
- Liko D, Slattery MG, Heideman W. Stb3 binds to ribosomal RNA processing element motifs that control transcriptional responses to growth in Saccharomyces cerevisiae. J Biol Chem 2007; 282:26623-26628; PMID:17616518; https://doi.org/10.1074/jbc.M704762200
- Liko D, Conway MK, Grunwald DS, Heideman W. Stb3 plays a role in the glucose-induced transition from quiescence to growth in Saccharomyces cerevisiae. Genetics 2010; 185:797-810; PMID:20385783; https://doi.org/10.1534/genetics.110.116665
- Bosio MC, Fermi B, Spagnoli G, Levati E, Rubbi L, Ferrari R, Pellegrini M, Dieci G. Abf1 and other general regulatory factors control ribosome biogenesis gene expression in budding yeast. Nucleic Acids Res 2017; PMID:28158860; https://doi.org/10.1093/nar/gkx058
- Yu S, Smirnova JB, Friedberg EC, Stillman B, Akiyama M, Owen-Hughes T, Waters R, Reed SH. ABF1-binding sites promote efficient global genome nucleotide excision repair. J Biol Chem 2009; 284:966-973; https://doi.org/10.1074/jbc.M806830200
- Bonetti D, Anbalagan S, Lucchini G, Clerici M, Longhese MP. Tbf1 and Vid22 promote resection and non-homologous end joining of DNA double-strand break ends. EMBO J 2013; 32:275-289; PMID:23222485; https://doi.org/10.1038/emboj.2012.327
- Ribaud V, Ribeyre C, Damay P, Shore D. DNA-end capping by the budding yeast transcription factor and subtelomeric binding protein Tbf1. EMBO J 2012; 31:138-149; PMID:21952045; https://doi.org/10.1038/emboj.2011.349
- Fukunaga K, Hirano Y, Sugimoto K. Subtelomere-binding protein Tbf1 and telomere-binding protein Rap1 collaborate to inhibit localization of the Mre11 complex to DNA ends in budding yeast. Mol Biol Cell 2012; 23:347-359; PMID:22130795; https://doi.org/10.1091/mbc.E11-06-0568
- Colin J, Candelli T, Porrua O, Boulay J, Zhu C, Lacroute F, Steinmetz LM, Libri D. Roadblock termination by reb1p restricts cryptic and readthrough transcription. Mol Cell 2014; 56:667-680; PMID:25479637; https://doi.org/10.1016/j.molcel.2014.10.026
- Preti M, Ribeyre C, Pascali C, Bosio MC, Cortelazzi B, Rougemont J, Guarnera E, Naef F, Shore D, Dieci G. The telomere-binding protein Tbf1 demarcates snoRNA gene promoters in Saccharomyces cerevisiae. Mol Cell 2010; 38:614-620; PMID:20513435; https://doi.org/10.1016/j.molcel.2010.04.016
- Fermi B, Bosio MC, Dieci G. Multiple roles of the general regulatory factor Abf1 in yeast ribosome biogenesis. Curr Genet 2017; 63:65-68; PMID:27262581; https://doi.org/10.1007/s00294-016-0621-3
- Hahn S, Young ET. Transcriptional regulation in Saccharomyces cerevisiae: transcription factor regulation and function, mechanisms of initiation, and roles of activators and coactivators. Genetics 2011; 189:705-736; PMID:22084422; https://doi.org/10.1534/genetics.111.127019
- Chong YT, Koh JL, Friesen H, Duffy SK, Cox MJ, Moses A, Moffat J, Boone C, Andrews BJ. Yeast proteome dynamics from single cell imaging and automated analysis. Cell 2015; 161:1413-1424; PMID:26046442; https://doi.org/10.1016/j.cell.2015.04.051
- Silve S, Rhode PR, Coll B, Campbell J, Poyton RO. ABF1 is a phosphoprotein and plays a role in carbon source control of COX6 transcription in Saccharomyces cerevisiae. Mol Cell Biol 1992; 12:4197-4208; PMID:1324416; https://doi.org/10.1128/MCB.12.9.4197
- Oliveira AP, Ludwig C, Zampieri M, Weisser H, Aebersold R, Sauer U. Dynamic phosphoproteomics reveals TORC1-dependent regulation of yeast nucleotide and amino acid biosynthesis. Sci Signal 2015; 8:rs4; PMID:25921291; https://doi.org/10.1126/scisignal.2005768
- Upton T, Wiltshire S, Francesconi S, Eisenberg S. ABF1 Ser-720 is a predominant phosphorylation site for casein kinase II of Saccharomyces cerevisiae. J Biol Chem 1995; 270:16153-16159; PMID:7608180; https://doi.org/10.1074/jbc.270.27.16153
- Sanchez-Casalongue ME, Lee J, Diamond A, Shuldiner S, Moir RD, Willis IM. Differential phosphorylation of a regulatory subunit of protein kinase CK2 by target of rapamycin complex 1 signaling and the Cdc-like kinase Kns1. J Biol Chem 2015; 290:7221-7233; PMID:25631054; https://doi.org/10.1074/jbc.M114.626523
- Paul E, Tirosh I, Lai W, Buck MJ, Palumbo MJ, Morse RH. Chromatin mediation of a transcriptional memory effect in yeast. G3 (Bethesda) 2015; 5:829-838; PMID:25748434; https://doi.org/10.1534/g3.115.017418
- Wade JT, Hall DB, Struhl K. The transcription factor Ifh1 is a key regulator of yeast ribosomal protein genes. Nature 2004; 432:1054-1058; PMID:15616568; https://doi.org/10.1038/nature03175
- Schawalder SB, Kabani M, Howald I, Choudhury U, Werner M, Shore D. Growth-regulated recruitment of the essential yeast ribosomal protein gene activator Ifh1. Nature 2004; 432:1058-1061; PMID:15616569; https://doi.org/10.1038/nature03200
- Lippman SI, Broach JR. Protein kinase A and TORC1 activate genes for ribosomal biogenesis by inactivating repressors encoded by Dot6 and its homolog Tod6. Proc Natl Acad Sci USA 2009; 106:19928-19933; PMID:19901341; https://doi.org/10.1073/pnas.0907027106
- Reid JL, Iyer VR, Brown PO, Struhl K. Coordinate regulation of yeast ribosomal protein genes is associated with targeted recruitment of Esa1 histone acetylase. Mol Cell 2000; 6:1297-1307; PMID:11163204; https://doi.org/10.1016/S1097-2765(00)00128-3
- Rohde JR, Cardenas ME. The tor pathway regulates gene expression by linking nutrient sensing to histone acetylation. Mol Cell Biol 2003; 23:629-635; PMID:12509460; https://doi.org/10.1128/MCB.23.2.629-635.2003
- Humphrey EL, Shamji AF, Bernstein BE, Schreiber SL. Rpd3p relocation mediates a transcriptional response to rapamycin in yeast. Chem Biol 2004; 11:295-299; PMID:15123258; https://doi.org/10.1016/j.chembiol.2004.03.001
- McKnight JN, Boerma JW, Breeden LL, Tsukiyama T. Global promoter targeting of a conserved lysine deacetylase for transcriptional shutoff during quiescence entry. Mol Cell 2015; 59:732-743; PMID:26300265; https://doi.org/10.1016/j.molcel.2015.07.014
- Nacht AS, Beato M, Vicent GP. Steroid hormone receptors silence genes by a chromatin-targeted mechanism similar to those used for gene activation. Transcription 2017; 8:15-20; PMID:27700223; https://doi.org/10.1080/21541264.2016.1242456
- Huisinga KL, Pugh BF. A genome-wide housekeeping role for TFIID and a highly regulated stress-related role for SAGA in Saccharomyces cerevisiae. Mol Cell 2004; 13:573-885; PMID:14992726; https://doi.org/10.1016/S1097-2765(04)00087-5
- Uprety B, Sen R, Bhaumik SR. Eaf1p is required for recruitment of NuA4 in targeting TFIID to the promoters of the ribosomal protein genes for transcriptional initiation in vivo. Mol Cell Biol 2015; 35:2947-2964; PMID:26100014; https://doi.org/10.1128/MCB.01524-14
- Hassan AH, Prochasson P, Neely KE, Galasinski SC, Chandy M, Carrozza MJ, Workman JL. Function and selectivity of bromodomains in anchoring chromatin-modifying complexes to promoter nucleosomes. Cell 2002; 111:369-379; PMID:12419247; https://doi.org/10.1016/S0092-8674(02)01005-X
- Costanzo M, VanderSluis B, Koch EN, Baryshnikova A, Pons C, Tan G, Wang W, Usaj M, Hanchard J, Lee SD et al. A global genetic interaction network maps a wiring diagram of cellular function. Science 2016; 353:aaf1420; https://doi.org/10.1126/science.aaf1420
- Albert B, Knight B., Merwin J, Martin V, Ottoz D, Gloor Y, Bruzzone MJ, Rudner A, Shore D. A Molecular titration system coordinates ribosomal protein gene transcription with ribosomal RNA synthesis. Mol Cell 2016; 64:720-733; PMID:27818142; https://doi.org/10.1016/j.molcel.2016.10.003