Abstract
Oxidative stress caused by reactive oxygen species and free radicals generated in living cells may cause various chronic, non-communicable diseases. Current research is directed towards finding naturally occurring antioxidants of plant origin. This study investigated the phytochemistry, antioxidant and free radical scavenging potential of ethanolic root extract of Coleus forskohlii (ERECF) using different antioxidant models. The phytochemical analysis of ERECF revealed the presence of alkaloids, glycosides, terpenoids, saponins, phenols and steroids. ERECF showed antioxidant capacity with the inhibition of 1,1-diphenyl-2-picrylhydrazyl (DPPH) radical by 71.21%. ERECF also had a potent inhibitory effect on scavenging nitric oxide, superoxide and hydroxyl radicals, by 66.23%, 61.22% and 61.68%, respectively. Moreover, ERECF displayed a concentration-dependent reducing power ability and a remarkable ferric ion-induced lipid peroxidation inhibitory effect (69.24%) in bovine brain extract. These findings confirm the biological efficacy of C. forskohlii as a potential source of natural antioxidants.
Introduction
Oxidation processes are intrinsic to the management of energy in all living organisms and are, therefore, kept under strict control by various cellular mechanisms (Halliwell & Gutteridge Citation2007). However, an imbalance between the production of excessive reactive oxygen species (ROS) and the antioxidant protection mechanisms can result in the onset of numerous diseases such as cancer, arteriosclerosis, diabetes and cardiovascular diseases, as well as accelerating ageing (Alma et al. Citation2003). Antioxidant molecules are considered to be possible protective agents, which reduce oxidative damage in the human body when the internal enzymatic mechanisms fail or are inefficient (Halliwell Citation1995). In the intracellular system, ROS are produced in cytoplasmic molecules, cytoplasmic proteins, membrane enzymes, peroxisomes, and mitochondrial and microsomic electron transport systems (Martinez Citation1995; Gulcin Citation2006). These ROS produced by a number of exogenous sources are potentially damaging transient chemical species (Alma et al. Citation2003). The importance of dietary antioxidant components for the prevention of chronic diseases and improvement of health quality has attracted huge research attention during the past few decades (Alvarado et al. Citation2006; Skotti et al. Citation2014).
Oxidation caused by ROS may result in the rancidity of processed or unprocessed food products rich in unsaturated fatty acids, and natural antioxidants are suggested to be superior to synthetic antioxidants including butylated hydroxyanisole (BHA) and butylated hydroxytoluene (BHT) (Li et al. Citation2008). Nowadays, a variety of synthetic antioxidants is commonly used in the food industry for food safety purposes. The use of these compounds has been restricted by the regulatory authorities owing to their long-term toxicity and carcinogenicity (Gulcin et al. Citation2010). Hence, interest has greatly increased in finding naturally occurring antioxidants to replace synthetic antioxidants for use in food and for medical purposes.
Medicinal plants are important sources of natural products which differ widely in terms of their structures, biological properties and mechanisms of action. Various phytochemical components, especially polyphenols such as tannins, flavonoids, phenyl propanoids and phenolic acids, are known to be have antioxidant and free radical scavenging efficacy. Polyphenols have various biological effects, which are mainly attributed to their antioxidant activities in scavenging free radicals, inhibition of lipid peroxidation and metal chelation. Polyphenols share the same general chemical pattern, with one or more phenolic groups which react as hydrogen donors and thus neutralize free radicals (Amari et al. Citation2014). Polyphenols are natural biologically active components found in every part of the plant including the leaves, flowers, shoot, stem and root, which work as a defence mechanism against diseases or, more accurately, protect the plant from disease (Amari et al. Citation2014).
Coleus forskohlii is a medicinal plant of the mint family, Lamiaceae, with well-known efficacy in the Ayurveda and Indian indigenous system of medicine for the treatment of asthma, heart ailments, insomnia, bronchitis, epilepsy, anaemia and inflammation (Sunitha et al. Citation2013). It is widely grown throughout India, and is also found in Thailand and other parts of South-East Asia. The roots of C. forskohlii are the natural source of forskolin and are amoebicidal. In addition, the roots of C. forskohlii are used in the activation and degranulation of platelets, leading to an increase in the force of contraction of the heart muscle, relaxation and vasodilatation of arteries, and increased insulin secretion (Upadhyaya & Prajapati Citation2014). A natural compound, rosmarinic acid, isolated from C. forskohlii, has a multitude of biological activities including anti-inflammatory, antioxidative, antimicrobial and antiviral effects (Sunitha et al. Citation2013). The presence of rosmarinic acid in medicinal plants, herbs and spices has beneficial and health-promoting effects in humans (Sunitha et al. Citation2013).
With regard to scientific surveys, there is no systematic report available in the literature on the antioxidant efficacy of ethanolic root extract of Coleus forskohlii (ERECF). Therefore, the aim of this research is to determine the potential efficacy of ERECF in various antioxidant and radical scavenging models, along with its lipid peroxidation inhibitory potential and phytochemical analysis.
Materials and methods
Chemicals and instruments
The chemicals and reagents used in this study, including 1,1-diphenyl-2-picrylhydrazyl (DPPH), sodium nitroprusside (SNP), Griess reagent, trichloroacetic acid (TCA), bovine brain extract, nitroblue tetrazolium (NBT), ferric chloride, potassium ferricyanide and gallic acid, as well as standard antioxidant compounds ascorbic acid and BHA, were purchased from Sigma-Aldrich (St Louis, MO, USA) and were of analytical grade. Spectrophotometric measurements were taken using a 96-well microplate enzyme-linked immunosorbent assay (ELISA) reader (Infinite M200; Tecan, Männedorf, Switzerland).
Plant material and extraction
Dried root powder of C. forskohlii was a gift from Jeevan Herbal Products (Sagar, MP, India). The dried root powder (100 g) was extracted by drenching in ethanol (95%) in a conical flask for 7 days at room temperature. The solvent was filtered followed by distillation under reduced pressure using a rotary evaporator (Eyela, China) until the solvent was completely dry. Finally, the extract, with a yield of 7.8 mg/100 g, was preserved in a sealed vial at 4°C until tested and analysed.
Preliminary phytochemical screening
A qualitative phytochemical screening of ERECF to detect the presence of essential phytoconstituents, such as alkaloids, tannins, saponins, flavonoids, anthraquinone glycoside, steroids, terpenes, glycosides, proteins, amino acids, reducing sugars and phenol, was carried out using standard biochemical procedures as described previously (Edeoga et al. Citation2005; Patra et al. Citation2009).
Determination of DPPH radical scavenging activity
The antioxidant activity, based on scavenging of stable DPPH free radical, was determined as described previously (Bajpai et al. Citation2013). Different concentrations of ERECF (25–150 µg/ml) were added to 0.004% methanolic solution of DPPH in a 1:1 ratio in a 96-well microplate. The mixture was incubated at 37°C in the dark for 30 min with shaking (150 rpm). Absorbance was recorded at 517 nm using the Tecan ELISA reader against a blank sample. All the tests were run in triplicate. Ascorbic acid was used as the reference compound in the concentration range of 25–150 µg/ml. The per cent inhibition activity was calculated using the following formula:
where Acontrol is the absorbance of the control reaction and Atest represents the absorbance of a test reaction.
Determination of nitric oxide radical scavenging activity
In this assay, a solution of SNP (10 mM) in phosphate-buffered saline (PBS, pH 7.4) was mixed with different concentrations of ERECF (20–100 µg/ml). The mixture was incubated at 37°C for 60 min in the light. Half the quantity of the aliquots was taken and mixed with an equal quantity of Griess reagent, and the mixture was incubated at 25°C for 30 min in the dark. The absorbance of pink chromophore generated during diazotization of nitrite ions with sulfanilamide and subsequent coupling with naphthyl ethylene diamine dihydrochloride (NED) was read at 546 nm against a blank (Bajpai et al. Citation2013). All tests were performed in triplicate. Ascorbic acid was used as the standard reference compound in the concentration range of 20–100 µg/ml. The per cent inhibition activity was calculated by the same formula as used for determination of DPPH radical scavenging activity (see above).
Determination of superoxide radical scavenging activity
Superoxide radical scavenging activity of ERECF was measured by the reduction of NBT according to the previously reported method (Bajpai et al. Citation2013). In this method, superoxide radical reduces the yellow dye (NBT2+) to produce the blue formazan, which is measured spectrophotometrically at 560 nm. Antioxidants inhibit the formation of blue NBT. The decrease in absorbance at 560 nm with antioxidants indicates the consumption of superoxide anion radical in the reaction mixture. The non-enzymatic phenazine methosulfate–nicotinamide adenine dinucleotide (PMS/NADH) system generates superoxide radicals, which reduce NBT to a purple formazan. In this assay, the reaction mixture (150 µl) contained phosphate buffer (0.2 M, pH 7.4), NADH (73 µM), NBT (50 µM), PMS (15 µM) and various concentrations (50–250 µg/ml) of the ERECF and standard compound. After incubation for 60 min at room temperature, the absorbance of the reaction mixture was measured at 560 nm against an appropriate blank to determine the quantity of formazan generated. All tests were performed three times. Ascorbic acid was used as the standard. The per cent inhibition activity was calculated by the same formula as used for determination of DPPH radical scavenging activity (see above).
Determination of hydroxyl radical scavenging activity
To determine the hydroxyl radical scavenging activity of ERECF, a previously described method was adopted (Bajpai et al. Citation2013). The assay is based on quantification of the degradation product of 2-deoxy-2-ribose sugar by condensation with 2-thiobarbituric acid (TBA). In this assay, hydroxyl radicals were generated by Fenton's reaction using the Fe3+–ascorbate–EDTA–H2O2 system. The reaction mixture in a total volume of 240 µl contained 2-deoxy-2-ribose (3 mM), KH2PO4-KOH buffer (20 mM, pH 7.4), FeCl3 (0.1 mM), EDTA (0.1 mM), H2O2 (2 mM), ascorbic acid (0.1 mM) and various concentrations (100–500 µg/ml) of ERECF or standard compound. After incubation for 45 min at 37°C, 40 µl of 2.8% TCA and 40 µl of TBA (0.5% in 0.025 M NaOH solution containing 0.02% BHA) were added to the reaction mixture, and the mixture was incubated at 95°C for 15 min to develop the pink colour. After cooling, the absorbance was measured at 532 nm against an appropriate blank solution. All tests were performed three times. BHA was used as the reference standard. The per cent inhibition activity was calculated by the same formula as used for determination of DPPH radical scavenging activity (see above).
Determination of antilipid peroxidation activity
A previously reported method was adopted to determine the Fe3+/ascorbic acid-dependent non-enzymatic lipid peroxidation activity of ERECF in bovine brain extract (Bajpai et al. Citation2013). The reaction mixture, in the absence and presence of ERECF (50–250 µg/ml) or reference compound, containing 50 µl of bovine brain phospholipids (5 mg/ml), 1 mM FeCl3 and 1 mM ascorbic acid in 20 mM phosphate buffer with a final volume of 330 µl, was incubated at 37°C for 1 h. The hydroxyl radicals generated in the reaction initiated the lipid peroxidation, resulting in malondialdehyde (MDA) production that was measured by the TBA reaction. All tests in this assay were performed three times. BHA was used as the reference compound. The per cent inhibition activity was calculated by the same formula as used for determination of DPPH radical scavenging activity (see above).
Determination of reducing power activity
The ferric ion (Fe3+) reducing power of ERECF was determined by the previously reported method (Bajpai et al. Citation2013). Aliquots (50 µl) of different concentrations of ERECF (5–25 µg/ml) and/or the positive control, ascorbic acid, were mixed with 50 µl phosphate buffer (0.2 M, pH 6.6) and 50 µl potassium ferricyanide (1% w/v in H2O), followed by incubation at 50°C for 20 min in the dark. After incubation, 50 µl of TCA (10% w/v in H2O) was added to terminate the reaction and the mixture was subjected to centrifugation at 3000 rpm for 10 min. For the final reaction mixture, the supernatant (50 µl) was mixed with 50 µl distilled water and 10 µl FeCl3 solution (0.1% w/v in H2O). The reaction mixture was incubated for 10 min at room temperature and the absorbance was measured at 700 nm against an appropriate blank solution. A higher absorbance of the reaction mixture indicated greater reducing power ability. All tests were run in triplicate. Ascorbic acid was used as the standard drug.
Statistical analysis
All data are expressed as the mean ± SD. One-way analysis of variance (ANOVA) was performed to test the significance of differences between means at the 5% level of significance using SAS statistical analysis software (SAS version 9.1, NC, USA).
Results and discussion
Phytochemical analysis
Several researchers have reported that phytochemicals including alkaloids, glycosides, terpenoids, saponins, phenols and steroids possess enormous antioxidant and free radical scavenging activities (Farhan et al. Citation2012; Amari et al. Citation2014). Plant extracts rich in polyphenols and essential phytoconstituents have been shown to exert potent antioxidant and free radical scavenging activities in various antioxidant models (Farhan et al. Citation2012; Amari et al. Citation2014). As shown in , ERECF was found to contain various phytochemicals or polyphenols including flavonoids, tannins, phenolics, steroids, glycosides, terpenoids and alkaloids, which are known to have potent antioxidant or free radical scavenging activities. In general, the antioxidant compounds of essential oils are terpenoids, which are phenolic in nature, and it would seem rational that their antioxidant mode of action could be related to that of other compounds. Although several different methods have been developed to evaluate the antioxidant activity of biological samples, it is relatively difficult to measure each antioxidant component separately. Phytochemical analysis of a mint family member, Perilla frutescens, also revealed the presence of polyphenolic compounds which have been found to display potent biological efficacy, including antioxidant activity (Asif Citation2012). In addition, various leaf extracts of Mentha piperita which contained terpenoids, steroids, phenols, flavonoids and tannins also showed remarkable antioxidant potential (Singh et al. Citation2011). Organic extracts of another member of the mint family, Nepeta nepetella, which also contained essential phytoconstituents such as tannins, saponins, phenols and flavonoids, displayed a significant amount of antioxidant activity in different scavenging models (Seladji et al. Citation2014).
Table 1. Phytochemical analysis of ethanolic root extract of Coleus forskohlii (ERECF).
DPPH scavenging activity
The results of this assay showed significant a decrease in the concentration of DPPH radicals due to the scavenging ability of ERECF and the standard compound, ascorbic acid (). The scavenging effects on the DPPH radical of ERECF and the standard compound at the concentration of 100 µg/ml were 59.26% and 72.91%, respectively. However, 150 µg ml−1 of ERECF resulted in 71.21% inhibition of DPPH radicals. The results were thus concentration dependent and statistically significant (p < 0.05). Similar findings on the DPPH radical scavenging activities of various plant extracts have been observed previously (Farhan et al. Citation2012; Amari et al. Citation2014). Kaur and Mondal (Citation2014) also reported on the DPPH radical scavenging activity of ethanolic extracts of selected medicinal plants, with a maximum inhibitory effect on inhibiting DPPH radicals by 81.80%.
Nitric oxide scavenging activity
As a cell signalling molecule, nitric oxide has been associated with a variety of physiological processes in the human body. It transmits signals from vascular endothelial cells to vascular smooth muscle cells and causes vasodilatation (Aliev et al. Citation2009). It plays a vital role in physiological functions in the respiratory, immune, neuromuscular and other systems. Nitric oxide also regulates the release of neurotransmitters, neuronal excitability, learning and memory processes, as well as inflammatory bowel syndrome, sepsis, septic shock, cephalalgia, dementia, multiple sclerosis and stroke (Aliev et al. Citation2009). Moreover, it has been shown that nitric oxide modulates neurotoxin-induced cellular damage and is involved in neuronal cell death in Parkinson's disease and other neurodegenerative disorders such as Alzheimer's disease (Aliev et al. Citation2009). Several studies have suggested that nitric oxide may modulate iron-catalysed oxidation reactions such as the superoxide-anion driven Fenton's reaction, which produces powerful oxidants such as the hydroxyl radical and organometallic complexes (Dadashpour et al. Citation2011).
Although the mechanisms by which nitric oxide may inhibit lipid peroxidation are not clearly understood, one possible mechanism relates to its ability to terminate the propagation of lipid peroxidation reactions. shows the scavenging effects of ERECF and the standard compound on the nitric oxide radicals at the concentration of 80 µg/ml, which were 54.22% and 63.57%, respectively, whereas ERECF at 100 µg/ml inhibited 66.23% of nitric oxide radicals. The results were statistically significant (p < 0.05) and concentration dependent. The nitric oxide radical scavenging activities of various plant-based products and extracts have been reported previously (Farhan et al. Citation2012; Amari et al. Citation2014). An ethanolic extract of Ixora coccinea was found to exhibit potential effects on scavenging nitric oxide radicals in vitro (Banerjee et al. Citation2011). Organic extracts from selected plants showed remarkable nitrite radical scavenging ability (Agrawal et al. Citation2013). In addition, methanol extracts of Bangladeshi medicinal plants significantly inhibited the formation of nitric oxide radicals in vitro (Parul et al. Citation2008).
Superoxide scavenging activity
The body generates the highly toxic superoxide radical (O2−) species through various biological and metabolic reactions. Although the relatively weak superoxide oxidants have only limited chemical reactivity, they are potential precursors of highly reactive species including hydrogen peroxide, hydroxyl radicals and singlet oxygen, causing lipid peroxidation (Kanatt et al. Citation2007). Therefore, superoxide radical scavenging capacity is the first line of defence against oxidative stress in humans. The superoxide anion is an oxygen-centred radical with a selective reactivity (Kanatt et al. Citation2007). It has been reported that the antioxidant properties of some plant products are effective mainly via scavenging of superoxide anion radicals (Dadashpour et al. Citation2011). In this assay, superoxide anions derived from dissolved oxygen by the PMS/NADH system reduce NBT.
shows the significant (p < 0.05) and concentration-dependent inhibition of superoxide radical generation by all concentrations of ERECF and the standard compound tested. At the concentration of 250 µg/ml, the superoxide radical inhibitory effects of ERECF and ascorbic acid were found to be 61.22% and 73.01%, respectively. Various plant-based products and extracts have previously been shown to exert superoxide scavenging efficacy (Hazra et al. Citation2010). Similarly, leaf and stem alcoholic extracts of Drymaria diandra were found to exhibit potent superoxide radical scavenging activity (Mandal et al. Citation2009).
Hydroxyl scavenging activity
A highly damaging species, the hydroxyl radical is an extremely reactive free radical formed in biological systems. It has long been implicated in free radical pathology and is capable of damaging almost every molecule found in living cells (Halliwell Citation1991). The best characterized biological damage caused by the hydroxyl radical is its capacity to stimulate lipid peroxidation, which occurs when the hydroxyl radical is generated close to membranes and attacks the fatty acid side-chains of the membrane phospholipids (Halliwell Citation1991). The hydroxyl radical is the most reactive free radical and can be formed from superoxide anion and hydrogen peroxide in the presence of metal ions, such as Cu2+ and Fe2+. The hydroxyl radical has the capacity to join nucleotides in DNA and cause strand breakage, which contributes to carcinogenicity, mutagenicity and cytotoxicity (Valko et al. Citation2007). The hydroxyl radical scavenging capacity of a drug is directly related to its antioxidant activity. The highly reactive hydroxyl radicals can cause oxidative damage to DNA, lipids and proteins (Valko et al. Citation2007). The effect of ERECF on the inhibition of free radical-mediated deoxyribose damage was assessed by means of the iron (II)-dependent DNA damage assay. Fenton's reaction generates hydroxyl radicals which degrade DNA deoxyribose sugar, using Fe2+ salts as an important catalytic component (Valko et al. Citation2007). Oxygen radicals may attack DNA either at the sugar or at the base, giving rise to a large number of products. ERECF was capable of reducing DNA damage at all concentrations used.
In this assay, ERECF was analysed for its hydroxyl radical scavenging activity to better examine its antioxidant capacity. As shown in , ERECF displayed the potential for hydroxyl radical scavenging activity in a concentration-dependent manner. The hydroxyl radical scavenging activities of ERECF and BHA at the concentration of 500 µg/ml were 61.68% and 70.02%, respectively. The ability of ERECF to quench hydroxyl radicals seems to be directly related to the prevention of propagation of the process of lipid peroxidation and seems to be a good scavenger of ROS, thus reducing the rate of the chain reaction. BHA was used as a reference standard. The hydroxyl radical scavenging activity of various plant extracts has been reported previously (Hazra et al. Citation2010).
Lipid peroxidation inhibition
Lipid peroxidation is a major cause of food deterioration, affecting the colour, flavour, texture and nutritional value of food and food products (Balu et al. Citation2005). During the process of lipid peroxidation, free radicals steal electrons from the lipids in cell membranes, which may result in a loss of membrane fluidity, an increase in membrane permeability and a decrease in physiological performance, thus endangering cell viability (Balu et al. Citation2005). The chemical structure of iron, and its capacity to drive one-electron reactions, makes iron a key factor in the formation of free radicals. In biological systems, lipid peroxidation generates many aldehyde products, among which MDA is considered to be the most important derivative (Barrera et al. Citation2008). Lipid peroxidation begins by a free radical chain reaction mechanism. It mostly affects polyunsaturated fatty acids, and is a major cause of cell membrane disruption and cell damage (Barrera et al. Citation2008). This process is initiated by hydroxyl and superoxide radicals leading to the formation of peroxy radicals that eventually propagate the chain reaction in lipids. Therefore, antioxidants capable of scavenging peroxy radicals could prevent lipid peroxidation.
In this study, the potential efficacy of ERECF in inhibiting lipid peroxidation induced via the Fe3+/ascorbate system was measured in bovine brain extract. The inhibitory effect of ERECF on Fe3+-induced lipid peroxidation in bovine brain homogenates is presented in . ERECF and BHA at the concentration of 250 µg/ml showed 69.24% and 76.59% inhibitory effects on lipid peroxides, respectively. ERECF protected considerably against lipid peroxidation induced by Fe3+ , by reducing lipid peroxidation in a concentration-dependent manner, and the results were statistically significant (). Other plant extracts have also shown protective effects against Fe3+-induced lipid peroxidation (Geetha & Vasudevan Citation2004). The inhibition of Fe3+-induced lipid peroxidation by ERECF may be because of Fe3+ chelation and its hydroxyl radical scavenging abilities. This capacity could be important because Fe3+ can stimulate free radical formation; thus, when complexes are formed between ERECF and Fe3+, lipid peroxidation could be prevented or reduced. ERECF was able to scavenge the hydroxyl radical produced during Fe3+-catalysed decomposition of hydrogen peroxide (Fenton's reaction); the highly reactive hydroxyl radical initiates a process of membrane lipid peroxidation that could lead to alterations in cell structure and function (Barrera et al. Citation2008).
Reducing power ability
Recent reports have shown that the electron donation capacity reflects the reducing power of biologically active compounds in relation to their antioxidant activity (Gulcin et al. Citation2010). Antioxidants are reducing agents, and inactivation of oxidants by reductants can be described as a reduction–oxidation (redox) reaction, in which one reaction species is reduced at the expense of the oxidation of the other (Gulcin et al. Citation2010). The reduction of Fe3+ is often used as an indicator of electron donating ability, which is an important mechanism of phenolic antioxidant action. In the reducing power assay, the presence of antioxidants in the sample would result in the reduction of Fe3+ to Fe2+ by the donation of an electron. The amount of Fe2+ complex can be then be monitored by measuring the formation of Perl's Prussian blue ferric ferrocyanide (Fe4[Fe(CN)6]3) at 700 nm. Increasing absorbance at 700 nm indicates an increase in reductive ability.
In this assay, shows the reducing power of ERECF as a function of its concentration. It was found that the reducing power of ERECF and ascorbic acid increased with the increase in their concentrations. The reducing power efficacy of ERECF and ascorbic acid at the concentration of 25 µg/ml was found to be 0.89 and 1.21, respectively, and the results were statistically significant. Previously, various plant extracts containing different phytochemicals have been shown to exhibit antioxidant activity through their reductive capacity in an Fe3+–Fe2+ system (Hazra et al. Citation2010). The results obtained in this study indicate that the marked reducing power of ERECF may be attributable to its antioxidant activity.
Figure 6. Reducing power activity of ethanolic root extract of Coleus forskohlii (ERECF) and standard compound, ascorbic acid.
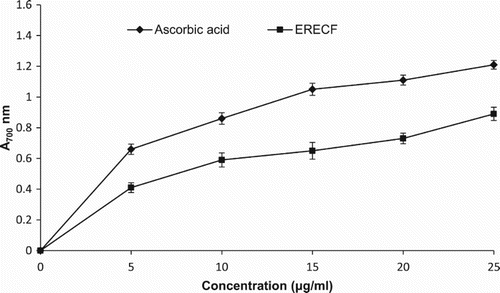
The antioxidant activity of plants comes mainly from the active compounds or essential phytoconstituents that they contain (Wojdylo et al. Citation2007). Although the activity of synthetic antioxidants is often reported to be higher than that of natural antioxidants, their higher toxicity and carcinogenicity prohibit their use at certain levels (Gulcin et al. Citation2010). At certain concentrations, various herbal products and/or extracts rich in polyphenols noticeably slowed down the formation of conjugated diolefin. There has been increasing interest in polyphenolic compounds in the food industry because of their inhibitory effects on lipid peroxidation and on the formation of off-flavours and other objectionable compounds, which could lead to improvements in the quality and nutritional value of fresh and processed foods (Wojdylo et al. Citation2007).
On the basis of the results obtained in this study, it can be concluded that ERECF, containing alkaloids, glycosides, terpenoids, saponins, phenols and steroids as essential phytochemicals, exhibited significant antioxidant and free radical scavenging activities. ERECF also exerted potent antioxidant activity through its reducing power ability. Moreover, ERECF had a potent inhibitory effect on ferric ion-induced lipid peroxidation. The antioxidant activity of herbal extracts is of considerable interest to those in the food industry who are looking for such compounds with significant biological potential to be used as alternatives to synthetic and conventional food preservation systems. These findings indicate that ERECF could be a source of natural antioxidants for use in the food industry against oxidative deterioration, as well as a useful therapeutic agent in the prevention of oxidative stress-related degenerative diseases. Further studies are planned to isolate individual biologically active constituents from ERECF and establish their precise in vivo antioxidant mode of action.
Disclosure statement
The authors declare that there is no conflict of interest.
References
- Agarwal R, Gupta SK, Agarwal P, Srivastava S, Alyayutdin R. 2013. Anticholinesterase, antioxidant, and nitric oxide scavenging activity of the aqueous extract of some medicinal plants. Br J Pharma Res. 3(4):807–816. doi: 10.9734/BJPR/2013/4833
- Aliev G, Palacios HH, Lipsitt AE, Fischbach K, Lamb BT, Obrenovich ME, Morales L, Gasimov E, Bragin V. 2009. Nitric oxide as an initiator of brain lesions during the development of Alzheimer disease. Neurotox Res. 16:293–305. doi: 10.1007/s12640-009-9066-5
- Alma MH, Mavi A, Yildirim A, Digrak M, Hirata T. 2003. Screening, chemical composition and in vitro antioxidant and antimicrobial activities of the essential oils from Origanum syriacum L. growing in Turkey. Biol Pharm Bull. 26:1725–1729. doi: 10.1248/bpb.26.1725
- Alvarado C, Álvarez P, Puerto M, Gausserès N, Jiménez L, De la Fuente M. 2006. Dietary supplementation with antioxidants improves functions and decreases oxidative stress of leukocytes from prematurely aging mice. Nutrition. 22:767–777. doi: 10.1016/j.nut.2006.05.007
- Amari NO, Bouzouina M, Berkani A, Lotmani B. 2014. Phytochemical screening and antioxidant capacity of the aerial parts of Thymelaea hirsuta L. Asian Pac J Trop Dis. 4:104–109. doi: 10.1016/S2222-1808(14)60324-8
- Asif M. 2012. Phytochemical study of polyphenols in Perilla frutescensas an antioxidant. Avicenna J Phytomed. 2:169–178.
- Bajpai VK, Sharma A, Kim SH, Baek KH. 2013. Phenolic content and antioxidant capacity of essential oil obtained from sawdust of Chamaecyparis obtusa by microwave-assisted hydrodistillation. Food Technol Biotechnol. 51:360–369.
- Balu M, Sangeetha P, Haripriya D, Panneerselvam C. 2005. Rejuvenation of antioxidant system in central nervous system of aged rats by grape seed extract. Neurosci Lett. 383:295–300. doi: 10.1016/j.neulet.2005.04.042
- Banerjee S, Chanda A, Ghoshal A, Debnath R, Chakraborty S, Saha R, Das A. 2011. Nitric oxide scavenging activity study of ethanolic extracts of Ixora coccinea from two different areas of Kolkata. Asian J Exp Biol Sci. 2:595–599.
- Barrera G, Pizzimenti S, Dianzani MU. 2008. Lipid peroxidation, control of cell proliferation, cell differentiation and cell death. Mol Asp Med. 29:1–8. doi: 10.1016/j.mam.2007.09.012
- Dadashpour M, Rasooli I, Sefidkon F, Rezaei MB, Alipour D, Astaneh S. 2011. Lipid peroxidation inhibition, superoxide anion and nitric oxide radical scavenging properties of Thymus daenensis and Anethum graveolens essential oils. J Med Plants. 10:109–120.
- Edeoga HO, Okwa DE, Mbaebie BO. 2005. Phytochemical constituents of some Nigerian medicinal plants. Afr J Biotechnol. 4:685–688. doi: 10.5897/AJB2005.000-3127
- Farhan H, Malli F, Rammal H, Hijazi A, Bassal A, Ajouz N, Badran B. 2012. Phytochemical screening and antioxidant activity of Lebanese Eryngium creticum L. Asian Pac J Trop Biomed. 12:S1217–S1220. doi: 10.1016/S2221-1691(12)60388-8
- Geetha RK, Vasudevan DM. 2004. Inhibition of lipid peroxidation by botanical extracts of Ocimum sanctum, in vivo and in vitro studies. Life Sci. 19:21–28. doi: 10.1016/j.lfs.2004.05.036
- Gulcin I. 2006. Antioxidant and antiradical activities of L-carnitine. Life Sci. 78:803–811. doi: 10.1016/j.lfs.2005.05.103
- Gulcin I, Elias R, Gepdiremen A, Chea A, Topal F. 2010. Antioxidant activity of bisbenzylisoquinoline alkaloids from Stephania rotunda, cepharanthine and fangchinoline. J Enz Inh Med Chem. 25:44–53. doi: 10.3109/14756360902932792
- Halliwell B. 1991. Reactive oxygen species in living systems: source, biochemistry and role in human disease. Am J Med. 91:14–22. doi: 10.1016/0002-9343(91)90279-7
- Halliwell B. 1995. Antioxidant characterization methodology and mechanism. Biochem Pharmacol. 49:1341–1348. doi: 10.1016/0006-2952(95)00088-H
- Halliwell B, Gutteridge JMC. 2007. Free radicals in biology and medicine. Oxford University Press, Oxford.
- Hazra B, Sarkar R, Biswas S, Mandal N. 2010. Comparative study of the antioxidant and reactive oxygen species scavenging properties in the extracts of the fruits of Terminalia chebula, Terminalia belerica and Emblica officinalis. BMC Comp Alt Med. 10:1–15. doi: 10.1186/1472-6882-10-20
- Kanatt KR, Chander R, Sharma A. 2007. Antioxidant potential of mint (Mentha spicata L.) in radiation processed lamb meat. Food Chem. 100:451–458. doi: 10.1016/j.foodchem.2005.09.066
- Kaur S, Mondal P. 2014. Study of total phenolic and flavonoid content, antioxidant activity and antimicrobial properties of medicinal plants. J Microbiol Exp. 1:5–10.
- Li HB, Wong CC, Cheng KW, Chen F. 2008. Antioxidant properties in vitro and total phenolic contents in methanol extracts from medicinal plants. LWT - Food Sci Technol. 41:385–390. doi: 10.1016/j.lwt.2007.03.011
- Mandal P, Misra TK, Ghosal M. 2009. Free-radical scavenging activity and phytochemical analysis in the leaf and stem of Drymaria diandra Blume. Int J Integr Biol. 7:80–85.
- Martinez CM. 1995. Review: oxygen free radicals and human disease. Biochimie. 77:147–161. doi: 10.1016/0300-9084(96)88119-3
- Parul R, Kundu SK, Saha P. 2008. In vitro nitric oxide scavenging activity of methanol extract of three Bangladeshi medicinal plants. Pharma Innov J. 1(12):83–88.
- Patra JK, Panigrahi TK, Rath SK, Dhal NK, Thatoi HN. 2009. Phytochemical screening and antimicrobial assessment of leaf extracts of Excoecaria agallocha L., a mangal species of Bhitarkanika, Orissa, India. Adv Nat Appl Sci. 3:241–246.
- Seladji M, Bekhechi C, Beddou F, Dib H, Bendimerad N. 2014. Antioxidant activity and phytochemical screening of Nepeta nepetella aqueous and methanolic extracts from Algeria. J Appl Pharma Sci. 4(2):012–016.
- Singh R, Shushni MAM, Belkheir A. 2011. Antibacterial and antioxidant activities of Mentha piperita L. Arabian J Chem. DOI:10.1016/j.arabjc.2011.01.019.
- Skotti E, Anastasaki E, Kanellou G, Polissiou M, Tarantilis PA. 2014. Total phenolic content, antioxidant activity and toxicity of aqueous extracts from selected Greek medicinal and aromatic plants. Ind Crops Products. 53:46–54. doi: 10.1016/j.indcrop.2013.12.013
- Sunita K, Chary KB, Nimgulkar CC, Kumar BD, Rao DM. 2013. Identification, quantification, and antioxidant activity of secondary metabolites in leaf and callus extracts of Coleus forskohlii. Int J Pharma BioSci. 4:1139–1149.
- Upadhyaya M, Prajapati G. 2014. Chlorophyl, antioxidant and phenolic contents in Coleus forskohlii. Int J Food Diary Technol. 1:13–15.
- Valko M, Leibfritz D, Moncola J, Cronin MTD, Mazura M, Telser J. 2007. Free radicals and antioxidants in normal physiological functions and human disease. Int J Biochem Cell Biol. 39:44–84. doi: 10.1016/j.biocel.2006.07.001
- Wojdylo A, Oszmianski J, Czemerys R. 2007. Antioxidant activity and phenolic compounds in 32 selected herbs. Food Chem. 105:940–949. doi: 10.1016/j.foodchem.2007.04.038