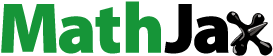
Abstract
Citric acid has high economic potential owing to its numerous applications. It is mostly produced by microbial fermentation using Aspergillus niger. In view of surges in demand and growing markets, there is always a need for the discovery and development of better production techniques and solutions to improve production yields and the efficiency of product recovery. To support the enormous scale of production, it is necessary and important for the production process to be environmentally friendly by utilizing readily available and inexpensive agro-industrial waste products, while maintaining high production yields. This article reviews the biochemistry of citric acid formation, choices of citric-acid producing microorganisms and raw materials, fermentation strategies, the effects of various fermentation conditions, citric acid recovery options and the numerous applications of citric acid, based on information drawn from the literature over the past 10 years.
Introduction
Citric acid, or 2-hydroxy-propane-1,2,3-tricarboxylic acid (C6H8O7.H2O) (), is a naturally occurring weak organic acid found in all citrus fruits. The name of this organic acid is derived from Latin word citrus, which refers to trees of the genus Citrus, including lemon trees. Citric acid in its pure form is readily soluble in water and colourless (Angumeenal & Venkappayya Citation2013). It is solid at room temperature. Citric acid has a melting point of 153°C and it decomposes at higher temperatures. Citric acid has a molecular weight of 210.14 g/mol and possesses three different pKa values, at pH 3.1, 4.7 and 6.4, owing to the presence of three functional groups of carboxylic acid in its structure (Papagianni Citation2007).
Citric acid can be derived from natural sources (e.g. lemon, lime and orange) or synthetic sources (e.g. chemical reaction and microbial fermentation). The method of extracting citric acid from lemon juice was pioneered by a Swedish chemist, Karl Wilhelm Scheele (1742–1786), in 1784. This method was adopted in England around 1826 for the commercial production of citric acid using lemons imported from Italy. The method maintained its monopoly as the only commercial source for citric acid production until the late nineteenth century, when a German botanist, Wehmer, in 1893, first observed the feasibility of obtaining citric acid through the fermentation of a sugar medium containing inorganic salts with Penicillium glaucum (Soccol et al. Citation2006). Two years after this discovery, Wehmer successfully isolated two strains which were able to produce citric acid. These strains were later named Citromyces spp. (Penicillium). However, the production of citric acid using Citromyces spp. did not gain much popularity in industrial practice because of contamination problems and the long fermentation process time (Bauweleers et al. Citation2014).
In 1916, a study conducted by James Currie made a breakthrough for successful economic industrial production of citric acid from Aspergillus niger. He discovered that significant amounts of citric acid could be obtained from various strains of A. niger. The most important findings were the ability of A. niger to grow at a pH of around 2.5–3.5, which curbed the formation of gluconic and oxalic acid, and the increase in citric acid production with increasing sugar concentration. This single piece of research laid the foundation for present-day industrial citric acid production, which was established in the USA by the pharmaceutical company Pfizer in 1923 (Dashen et al. Citation2014).
Over the years, a great variety of microorganisms has been studied, ranging from fungi and bacteria to yeasts. However, the microbial conversion of organic materials to citric acid is a complex biochemical reaction which requires careful control and specifically tailored operating conditions. Factors affecting the fermentation process include the concentration and type of carbon source, the pH of the fermentation medium, phosphate and nitrogen limitations, aeration, the morphology of the citric-acid producing microorganism and the concentrations of trace elements. Some of the nutrients, such as trace metals like manganese, phosphate and nitrogen, must be below defined limits to have a positive effect on the fermentation process. However, other elements, such as oxygen and sugar, are required in excess (Soccol et al. Citation2006; Max et al. Citation2010).
Aspergillus niger is superior to other microorganisms for the commercial synthesis of citric acid because of its better production yield. It is easy to handle, can ferment various cheap raw materials and delivers high yields. As such, strains of this microorganism can be improved to create industrial strains for use in commercial production, and mutagenesis and strain selection have been carried out for such improvement. Different mutagens, including radiation, such as ultraviolet, X-rays and gamma-rays, and chemicals, such as ethyl methane sulphonate and diethyl sulphonate, have been used to induce the mutation of A. niger.
Citric acid can also be produced by purely chemical reactions. Citric acid was first synthesized chemically in Citation1880 by Grimaux and Adam, using glycerol as a starting material, but this method was not economically competitive enough compared to other production routes such as fermentation. Therefore, microbial fermentation became the choice for commercial citric acid fermentation as it was more successful than chemical means. Citric acid is multifunctional and vital in many industries. Its vast and extensive range of versatile applications in various industries since the beginning of the twentieth century has created a continuous high demand. The worldwide production of citric acid in 2007 was 1.6 million tonnes, with an annual increase in demand and consumption of 3.5–4.0% (Anastasssiadis et al. Citation2008). Citric acid is commonly used in food and beverages, detergents, pharmaceuticals, cosmetics, toiletries and other industries (Majumder et al. Citation2010). The beverage and food industries account for about 75% of the world's citric acid consumption, mainly as an ingredient in carbonated drinks and an acidulant. Industrially, metal finishing and cleaning accounts for the largest use of citric acid, followed by lubricants, chelating agents, animal feeds and plasticizers (Bauweleers et al. Citation2014).
Consumption of citric acid is expected to multiply owing to its low prices and numerous applications. The competitive pricing of citric acid is mainly caused by suppliers from China being willing to sell it at the lowest price possible, and this phenomenon has led to extremely tough competition for European suppliers (Gruben et al. Citation2014). ADM and Tate & Lyle have had to shut down their citric acid plants in Ireland and the UK owing to fierce competition from China (Weyda et al. Citation2014). In 2012, China was responsible for 59% of the global production of citric acid (Angumeenal & Venkappayya Citation2013). According to estimations, the market value of citric acid will continue to grow and will soon exceed $2 billion (van der Straat et al. Citation2014).
Based on market trends, it is apparent that there will be a surge in the global citric acid demand. Hence, it is crucial to optimize citric acid production by looking for alternatives that are more economical, more environmentally friendly and have a higher production yield than current methods. In light of this, this review discusses the biochemistry of citric acid production, raw material choices, selection of citric-acid producing microorganisms, production methods and strategies, the effects of various fermentation conditions and recovery options. The aim is to provide a thorough and comprehensive review, compared to other reviews that focus on specific areas (Pazouki & Panda Citation1998; Anastassiadis et al. Citation2008; Dhillonet al. Citation2011a).
Biochemistry of citric acid production by Aspergillus niger
Formation of citric acid
The biochemistry of citric acid formation is complex. The production of citric acid is caused by the collective effect of different nutritional conditions in the medium (Kristiansen & Sinclair Citation1979). Nutritional conditions synergistically affect the production yield of citric acid, including carbon source concentration, dissolved oxygen, hydrogen ions, and suboptimal concentrations of phosphate and trace metals. Some studies report that limitation of the nitrogen source and deficiency of manganese or phosphate in the fermentation medium will inhibit the anabolism of A. niger, resulting in protein degradation which, in turn, leads to a higher concentration of ammonium ions (Rohr & Kubicek Citation1981).
Excessive citrate can be produced by A. niger through an active glycolytic pathway (). Citrate is a well-known inhibitor of glycolysis, thus making it an area of research interest. Under specific conditions, citrate inhibition can be curbed as a result of build-up of different positive effectors of the phosphofructokinase gene (PFK-1) (Käppeli et al. Citation1978; Kristiansen & Sinclair Citation1979). Protein breakdown occurs as a result of manganese deficiency and this leads to an increase in intracellular NH4+ concentration (Habison et al. Citation1983). The phenomenon is known as the ‘ammonium pool’ and it inhibits the enzyme phosphofructokinase, which is vital for the conversion of fructose and glucose to pyruvate. As a result, there is a flux through glycolysis and citric acid formation. High glucose and NH4+ concentrations will strongly limit 2-oxoglutarate dehydrogenase formation and eventually inhibit the catabolism of the citric acid present in the tricarboxylic acid (TCA) cycle (Rohr & Kubicek Citation1981).
Figure 2. Schematic representation of the main metabolic reactions involved in the production of citric acid by Aspergillus niger (Soccol et al. Citation2006).
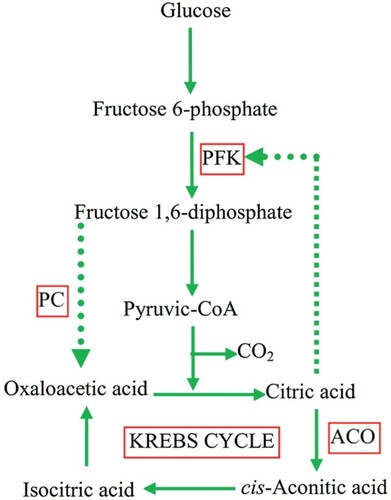
Results from a study by Papagianni et al. (Citation2005) on the early stages of accumulation of citric acid by A. niger contradicted those from the studies by Rohr and Kubicek (Citation1981) and Habison et al. (Citation1983), who reported that the presence of an intracellular ammonium pool does not account for the inhibition of phosphofructokinase. Ammonium ions enter cells to form glucosamine by combining with glucose, rather than just being simply deposited inside the cell (Boddy et al. Citation1993).
Thus, inhibition of the phosphofructokinase enzyme was found not to be a result of the ammonium pool. This is caused by several factors such as the release of synthesized glucosamine into the fermentation broth, the very low concentration of intracellular ammonium ions (about one-hundredth of the external pH) and the intracellular pH. Papagianni et al. (Citation2005) recommend further investigation into the relationship of the enzymes phosphofructokinase, 2-oxoglutarate dehydrogenase and glucosamine synthase with the high levels of glucose and ammonium ions within the TCA cycle. The enzymes of A. niger act as the driving force in citric acid formation. These enzymes include invertase (a membrane-bound enzyme), which hydrolyses sucrose into glucose and fructose, which are transported into the cell as the reaction pathway from sucrose to citric acid begins outside the cell (Rubio & Maldonado Citation1995). Hexokinase is more abundant than other enzymes in A. niger, and it has an affinity for glucose over fructose in a ratio of 1000:1. Citrate inhibits glucokinase non-competitively (Steinböck et al. Citation1994). Schreferl-Kunar et al. (Citation1989) showed that mutation can be used to produce an A. niger strain with higher affinity for sucrose growth .
Glucose oxidase stimulates A. niger to oxidize glucose to gluconic acid (Hayashi & Nakamura Citation1981). The enzyme is most potent under a high concentration of glucose, with strong aeration and low concentrations of other nutrients (typical conditions for citric acid fermentation) (Mischak et al. Citation1985; Dronawat et al. Citation1995). The effect of glucose oxidase is limited, however, as it is deactivated at pH lower than 3.5. The pH is reduced as a result of the accumulation of protons in the fermentation broth (Roukas & Harvey Citation1988). It is not known whether the gluconic acid will be used later in the citric acid formation during fermentation. Phosphofructokinases (PFK1 and PFK2) are responsible for the phosphorylation of fructose-6-phosphate. PFK1 phosphorylates at the C1 position to produce fructose-1:6-bisphosphate. High concentrations of ATP, citrate and manganese inhibit PFK1, but it can be activated by the products of the PFK2 reaction, such as Zn2+, Mg2+, NH4+ and fructose-2,6-bisphosphate (Kubicek-Pranz et al. Citation1990).
Well-known studies by Martin and Wilson (Citation1951) and Cleland and Johnson (Citation1954) revealed that citric acid was formed via the glycolytic reactions pathway. The glycolytic and pentose phosphate reaction pathways act as a channel for Aspergillus species to utilize glucose and other carbohydrates for biosynthesis and maintenance. Pyruvate kinase was previously found to be an essential regulatory stage in citric acid synthesis, but a study showed that the enzyme in its pure form was only marginally affected by the metabolic levels of inhibitors (Meixner-Monori et al. Citation1984). Citrate synthase is a catalyst for the reversible condensation reaction between acetyl coenzyme A (acetyl CoA) and oxaloacetate. This reaction favours citrate production and, as a result, thioester hydrolysis occurs during the reaction (Mischak et al. Citation1985). The equation is shown below:
Figure 3. Schematic representation of the metabolic reactions involved in citric acid production, the enzymes (italics), the known feedback loops (dashed lines) and their locations within the cellular structure of Aspergillus niger (Papagianni Citation2007).
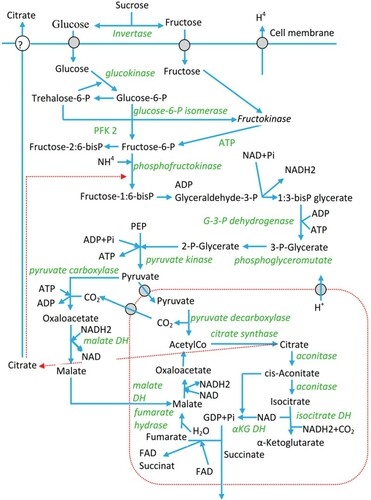
Three different isocitrate dehydrogenase isoenzymes exist in A. niger, which can be differentiated from each other by the recipient that they utilize for the removal of protons from isocitrate (). These enzymes are known as NADP+-dependent forms (one exists in mitochondria and another in the cytoplasm) and NAD+-dependent isocitrate dehydrogenases (which exists only in mitochondria). The NAD+-dependent enzymes are found in lower quantities than the NADP+-dependent enzymes (La Nauze Citation1966; Jernejc et al. Citation1992).
Accumulation of citric acid
In general, the build-up of citric acid involves deactivation of aconitase and/or isocitrate dehydrogenase. Activity in the Krebs cycle produces intermediates necessary for biomass formation during the formation of citric acid (Jernejc et al. Citation1992; Aghdam & Taherzadeh Citation2008). The Krebs cycle is a series of eight reactions that take place in the mitochondrion. These reactions take a two-carbon molecule (acetate) and completely oxidize it to carbon dioxide. The cycle is summarized in the following chemical equation:
Therefore, the accumulation of citric acid probably results from enhanced (deregulated) biosynthesis instead of inhibited degradation (Punekar et al. Citation1984).
Kubicek-Pranz et al. (Citation1990) explained the accumulation of citric acid by associating it with the tricarboxylate transporter competing for citric acid with aconitase. Under conditions where the tricarboxylate transporter has a greater affinity for citric acid than for aconitase, the enzyme removes citric acid from the mitochondria of the cell without inhibiting the enzymes present in the cycle.
Glutamine synthase is linked with the effects of pH, ammonia and manganese ions on the accumulation of citric acid (Punekar et al. Citation1984). The low pH value during the production phase (pH < 2) reduces the risk of contamination by other microorganisms and inhibits the production of unwanted organic acids (gluconic and oxalic acids), which facilitates recovery of the product. The presence of Mn2+ or Mg2+ ions in the enzyme structure determines its form. The isozymes of isocitrate dehydrogenase in A. niger, which are present in the mitochondria as well as the cytoplasm, require Mg2+ or Mn2+ for reaction, and their inhibition by α-ketoglutaric and citric acid ensures citric acid accumulation (Ratledge & Kristiansen Citation2001).
Isocitrate undergoes a two-phase conversion process to become α-ketoglutarate. First, protons are detached to form oxalosuccinate. Secondly, carbon dioxide is formed and released, completing the conversion. The metal ions Mg2+ and Mn2+ are essential for the reaction, as they are attached to the active sites by these enzymes. They are responsible for the stabilization of oxalosuccinate (intermediate reaction product) and the substrate–enzyme binding. Either Mg2+ or Mn2+ can be utilized by the enzymes without adverse effects on their activity. Citric acid accumulation is made possible as citrate and α-ketoglutarate inhibit the NADP+-dependent form of the enzyme (Mattey Citation1977).
Microbial production of citric acid via fermentation
Microorganisms
Species of Aspergillus such as A. wentii, A. foetidus, A. aculeatus, A. awamori, A. fonsecaeus, A. phoenicis and A. carbonarius, as well as Trichoderma viride and Mucor piriformis, have been found to produce significant amounts of citric acid (Berovic & Legisa Citation2007).
Besides fungi and bacteria, yeast species such as Candida tropicalis (Legiša & Mattey Citation1986), Candida oleophila (Käppeli et al. Citation1978), Candida guilermondii (Angumeenal et al. Citation2003), Yarrowia lipolytica (Angumeenal & Venkappayya Citation2013), Torulopsis, Hansenula, Dabaryomyces, Torula, Pichia, Kloeckera, Saccharomyces and Zygosaccharomyces are capable of producing citric acid from n-alkanes and carbohydrates (Weyda et al. Citation2014). The drawback of using yeast is that it produces large quantities of isocitric acid, which is an undesirable by-product; therefore, mutant strains that have low aconitase activity are required. In addition, the increasing cost of oil makes it less feasible economically as oils are now used as the principal carbon source, in a manner analogous to the previous use of alkanes (Mazinanian et al. Citation2015).
Aspergillus niger has so far maintained its place in citric acid production as it has advantages over other bacterial microorganisms such as Arthrobacter paraffineus, Bacillus licheniformis, Bacillus subtilis, Brevibacterium flavum, Corynebacterium spp. and Penicillium janthinellum (Ikram-ul et al. Citation2004). It is easy to handle, can ferment a broad range of low-cost raw materials and provides high yields (Themelis & Tzanavaras Citation2001). Mutagenesis has been used in recent years to improve the citric-acid producing strains so that they can be used in industrial applications. The most common methods include the use of mutagens to induce mutations on the parental strains. The mutagens utilized for improvements are gamma radiation, ultraviolet radiation and often chemical mutagens. For hyperproducer strains, a hybrid method that combines ultraviolet and chemical mutagens is used (Ratledge & Kristiansen Citation2001). The selection methods are the passage and single-spore techniques. The passage method is preferred, as the single-spore method has a disadvantage in that organic acids (oxalic and gluconic acids) and mineral acids simulate the presence of citric acid (Soccol et al. Citation2006).
The fermentation technique also affects the citric acid yield. As an example, a strain may have a good yield in the submerged fermentation process but a poor yield in the solid-state fermentation process. Therefore, the producer strains need to be tested in each of the fermentation methods as well as the industrial substrates to ascertain the best fermentation method (Chen et al. Citation2014). Microorganisms for citric production have to be inoculated by spores, which are transferred to the fermentation medium. The various transfer media include air, and can be in the form of a suspension which is then introduced into bottles containing the substrate. Ideally, for high yields, an incubation time of 7 days is required for A. niger. However, after the 7 days of incubation, the capacity for germination tends to reduce with time (Vergano et al. Citation1996).
Substrates
A wide range of substrates is utilized in the fermentation process of the microorganisms. Materials such as hydrocarbons, molasses and starchy materials are commonly used. The review by Soccol et al. (Citation2006) mentioned examples such as beet molasses, black strap molasses, cane molasses, carob pod extract, n-paraffin, glycerol, corn starch, hydrolysate starch, yam bean starch, wood hemicellulose, olive oil, rapeseed oil, palm oil and soya bean oil.
Owing to the need to use less expensive substrates with the aim of reducing the production costs of citric acid and making it more environmentally sustainable, the non-crystallizable effluents (molasses) after sucrose isolation from sugar refineries may be used. Molasses offers reduced cost and a high sugar content of 40–55% in the form of fructose, glucose and sucrose (Dronawat et al. Citation1995). The quality of molasses varies according to its source. Therefore, it requires pretreatment [e.g. mixing with K4Fe(CN)6 at pH 4.5, 90°C for 15 min and then removal of the precipitate by filtration] to make it suitable for fermentation. This variation in the molasses is usually a result of the different varieties of sugar cane/beet, cultivation methods and storage conditions (Li et al. Citation2014).
Studies have shown that beet molasses produces a higher yield than cane molasses; this can be attributed to the relatively high content of trace metals such as magnesium, zinc, manganese, calcium and iron in cane molasses, which are all detrimental to the synthesis of citric acid (Chen et al. Citation2014). Attempts have also been made to improve the citric acid yield of molasses by the addition of yield-increasing materials. Lu et al. (Citation1998) used a plant constituent (phytate) to supplement the molasses at the beginning of incubation, thereby tripling the citric acid accumulation (Themelis & Tzanavaras Citation2001). Ambati and Ayyanna (Citation2001) used a palmyra jiggery, which is sugar syrup extracted from the palmyra palm. Wojtatowicz et al. (Citation1993) supplemented beet molasses with natural oil with a high unsaturated fatty acid content, and observed an increased yield. In recent years, as a result of increasing demand for citric acid and increasing environmental concerns, there has been more interest in feasible novel substrates for citric acid production. Various residues and by-products from the agricultural industry have been used as substrates for different fermentation systems, as shown in .
Table 1. Raw materials used for citric acid fermentation.
Some substrates lack necessary ingredients such as nitrogen and phosphate that are required to be at optimum levels for optimum citrate formation. In situations where these nutrients are unavailable, nitrogen and phosphate are supplied by the addition of KNO3 or NH4NO3 and K2HPO4 or KH2PO4, respectively (Angumeenal & Venkappayya Citation2013).
Fermentation strategies in citric acid production
Citric acid production by fermentation has become established as the most widely used and economical process to obtain citric acid. Over 90% of the citric acid used around the globe today is produced from fermentation. This method offers advantages such as having simple, stable and less complicated operations; requiring less complex control systems and lower technical skill; consuming less energy; and not being critically affected by frequent plant power failure (Soccol et al. Citation2006). In general, all fermentation processes, irrespective of the type of fermentation, have three phases: preparation and inoculation, fermentation, and recovery of the citric acid.
Over the years, citric acid fermentation has undergone a series of developments. In the 1910s, production was limited to species of Penicillium and Aspergillus utilizing surface or stationary culture conditions. In the 1940s, Shu and Johnson (Citation1948a) developed submerged fermentation from Aspergillus. This research provided the basis for submerged fermentation.
Various types of fermenter have been used for the fermentation to produce citric acid. These include trays, glass incubators, Erlenmeyer conical flasks, rotating and horizontal drum bioreactors, and packed-bed single-layer packed-bed and multi-layer packed-bed column bioreactors.
Surface fermentation
Surface fermentation, also known as liquid surface culture, was the original citric acid industrial production technique. Even though in recent years submerged fermentation has gained popularity, there are still small- and medium-scale industries that make use of this method (Bauweleers et al. Citation2014). Surface fermentation offers advantages such as lower installation and energy costs (as it does not require energy for aeration and agitation), and is also foam free. However, it is labour intensive (Drysdale & McKay Citation1995) and sensitive to changes in composition of the media (Benghazi et al. Citation2014). This method consists of two phases, both of which are characterized by a rapid uptake of carbohydrates. The first phase is the development of the fungus as mycelial mat on the surface of the medium and the second phase utilizes carbohydrates by converting them to citric acid (Kiel et al. Citation1981). The process is conventionally performed in fermentation chambers, using trays made from materials such as special-grade steel, high-purity aluminium or polyethylene. However, stainless steel trays are preferred, as they are resistant to deformation with prolonged use (Bauweleers et al. Citation2014).
Since the chamber needs to be effectively ventilated, sterile air is aseptically passed continuously over the medium surface through a bacteriological filter. The air supplies the required oxygen demand of the microorganisms due to the highly aerobic nature of the process; it also controls the humidity and temperature (via evaporative cooling). The air also serves to remove carbon dioxide, which is an inhibitor of citric acid production at concentrations higher than 10% (Soccol et al. Citation2006). Contamination is also an important issue in surface cultures. Yeasts, penicillia, lactic bacteria and species of Aspergillus are the most common sources of contamination (Soccol et al. Citation2006). After completion of the second phase, the citric acid is recovered by washing the mycelial mats and the impregnated citric acid is extracted (Max et al. Citation2010). Other research on surface culture includes Sakurai and colleagues’ kinetics study of A. niger citric acid production by surface culture and their research on surface liquid fermentation of banana waste (Sakurai et al. Citation1991).
Submerged fermentation
This is the most widely used fermentation technique in the world today. Eighty per cent of the world's production is estimated to be from the submerged method (Roukas Citation1991). Submerged fermentation was developed after surface fermentation. It requires more sophisticated installation, higher energy cost and rigorous control, and there is formation of foam (which can be resolved using anti-foaming agents), but it provides higher productivity and yields, has reduced capital, maintenance and labour costs, and carries lower contamination risks. In addition, it is less sensitive to change in the medium composition, providing a wider range of substrates and better control of substrates; this advantage makes molasses usable as a medium for citric acid production (Max et al. Citation2010). Submerged fermentation is mostly operated as a batch system (Kishore et al. Citation2008); however, continuous systems are possible and are used in practice. Submerged fermentation also includes the shake flask technique, which is usually used for the optimization of fermentation conditions (Ikram-ul et al. Citation2004). This is basically an Erlenmeyer flask which is placed on a shaker and stirred continuously throughout the fermentation process.
Darouneh et al. (Citation2009) performed a comparative study between surface culture and submerged culture techniques. The outcome from this study was that surface fermentation is superior to submerged fermentation in terms of yield and productivity of citric acid. Ali et al. (Citation2002a) studied citric acid production utilizing cane molasses in a stirred fermenter (Ikram-ul et al. Citation2004). Selected mutants of A. niger producing citric acid from cane molasses were also investigated (Majumder et al. Citation2010). Soccol et al. (Citation2006) studied the influence of metal-complexing agents on the mycelial growth, conidial germination and morphology of A. niger. Roukas (Citation1991) demonstrated the ability of beet molasses to produce citric acid using shake flasks. Anastasssiadis et al. (Citation2008) studied the oxygen requirements for citric acid production using Yarrowia lipolytica. The construction materials (e.g. austenitic stainless steel, type 316L stainless steel) for a submerged fermenter must be able to withstand low pH (acidic) conditions. Both stainless steel and ordinary steel will degrade under acidic conditions and inhibit citric acid formation. A thorough study on molasses and pumpkin as substrates, using A. niger 14/20 and 79/20 strands, showed that both substrates can be used for the production of citric acid, but a mixed substrate gives better overall results (Majumder et al. Citation2010).
Solid-state fermentation
The solid-state process, or ‘Koji’ fermentation, originates from Japan, which has an abundance of agro-industrial residues/wastes (Kareem et al. Citation2010). This process involves the cultivation of microorganisms in the absence of free liquid on moist solid materials (Käppeli et al. Citation1978). The solid materials act as a physical support and source of nutrients for the microorganism. Under optimal conditions, the process should be completed in 4 days (Drysdale & McKay Citation1995). The main advantage of solid-state fermentation is its superior yield and the ability to utilize inexpensive and widely available agro-industrial residues as substrates for bio-production, making it more environmentally friendly than submerged fermentation (Falony et al. Citation2006). It requires less water and has lower operating costs, and does not require complex equipment. There is no need for pretreatment as the system is less sensitive to the presence of trace elements compared to submerged fermentation (Berovic & Legisa Citation2007).
One limitation of this method is that it does not completely utilize available nutrients owing to poor heat and oxygen transfer in the substrate (Sangsurasak & Mitchell Citation1995). There is also a limited pool of viable microorganisms, and strains with large nitrogen and phosphorus requirements cannot be used. Agro-industrial wastes that have been utilized include banana peel (Max et al. Citation2010), cotton waste (Kiel et al. Citation1981), kiwi fruit peel (Hang et al. Citation1987), date pulp (Assadi & Nikkhah Citation2002), apple pomace ultrafiltration sludge and solid waste (Kareem et al. Citation2010), orange peel waste (Torrado et al. Citation2011), pineapple waste (Kareem et al. Citation2010), wheat bran and soya bean meal (Sauer et al. Citation2008), fresh kumara (Ipomoea batatas), potato (Solanum tuberosum) and taro (Colocasia esculenta) (Arshad et al. Citation2014).
Factors affecting citric acid fermentation
Carbon source
Studies over several decades have shown that the carbon source affects the citric acid yield directly. Monosaccharides and disaccharides are the preferred carbon source as they are more rapidly metabolized by the fungus than polysaccharides, thus producing higher yield (Mattey Citation1992). Polysaccharides are not suitable as the raw material as the decomposition process takes too long to meet the rate of sugar catabolism necessary for the production of citric acid. The slow rate of polysaccharide hydrolysis is due to reduced enzymatic activity, which affects the pH in the fermentation medium (Hossain et al. Citation1984; Xu et al. Citation1989; Papagianni et al. Citation2005). Sucrose is superior to glucose, fructose and lactose, in order of decreasing citric acid yield (Angumeenal & Venkappayya Citation2013). The superiority of sucrose has been attributed to the strong extracellular mycelium-bound invertase of A. niger, which rapidly hydrolyses sucrose at low pH (Kubicek-Pranz et al. Citation1990).
Pure sucrose or glucose may not be economically feasible on an industrial scale. Therefore, low-grade carbon sources such as wastes from sugar refineries, for example cane and beet molasses are used. Owing to the various sources from which these raw materials are obtained, there may be a need for pretreatment. Cations are generally the major contaminants, and the commonly used method of pretreatment is by precipitation using potassium ferrocyanide or cation-exchange resins (Angumeenal & Venkappayya Citation2013a). The concentration of the carbon source is as critical to the success of citric acid production as the type of carbon source. Since citric acid production relates directly to sugar concentration, as the concentration increases so does the amount of citric acid produced (Xu et al. Citation1989). However, results showed that the maximum concentration can be obtained with 14-–22% of sugar. A study by Xu et al. (Citation1989) using sucrose, glucose, fructose, mannose and maltose obtained the maximum yield at a sugar concentration of 10% (w/v), except for glucose, where a 7.5% (w/v) maximum yield was obtained (Amenaghawon & Aisien Citation2012).
Nitrogen limitation
The concentration of nitrogen has been found to have a strong effect on the production of citric acid, as nitrogen is not only part of a cell's proteins, but also necessary for cellular metabolism (Ali et al. Citation2002b). Molasses and other industrial media are usually nitrogen rich, whereas laboratory media require additional ammonium salts as supplements. The type of nitrogen source affects the synthesis of citric acid as well as the fungal growth. Ammonium nitrate promotes reduced vegetative growth, while ammonium sulphate promotes a longer period of vegetative growth. Nitrogen limitation is necessary, because at a concentration greater than 0.25%, oxalic acid accumulates and it will decrease the citric acid yield (Gupta et al. Citation1975). A high nitrogen concentration increases the consumption of sugar and fungal growth, while decreasing the amount of citric acid produced (Hang et al. Citation1977). Ammonium sulphate is the preferred choice of salt as it does not produce the unwanted oxalic acid (Nigam Citation2009), while reducing the pH of the medium as the salt is consumed. Kristiansen and Sinclair (Citation1979) also discovered that nitrogen limitation is required for a good yield of citric acid in a continuous system.
The maximum citric acid yield in a laboratory-scale stirred fermenter was achieved with a concentration of ammonium nitrate kept at 0.2% (Ali et al. Citation2002a). Kareem et al. (Citation2010). supplemented the fermentation medium containing pineapple waste with 0.25% (w/v) of methanol and observed a 17.6% increase in the production of citric acid. The biomass increased as well. Further increases or decreases in concentration led to the distortion of fungal growth and lower citric acid production.
Phosphorus source
Along with nitrogen and the carbon source, phosphate has also been shown to be a critical factor. For fungal growth, a phosphorus concentration of 0.5–5 g/l is required for citric acid production (Shu & Johnson Citation1948b). The addition of phosphorus has only a slight effect on the accumulation of citric acid and mycelial growth (Hang et al. Citation1987). Kubicek and Röhr (Citation1977) deduced that citric acid accumulates with limited phosphate, even when nitrogen is not limited.
pH of culture medium
The pH of the medium changes continuously as a result of microbial metabolic activities, largely because of the secretion of organic acids such as citric acid, and the unwanted gluconic and oxalic acid. The metabolic activities of microbes such as Aspergillus, Rhizopus and Penicillium species are able to reduce the pH quickly to below 3, while other fungi such as Sporotrichum and Pleurotus species produce a more stable pH between 4 and 5 (del Campo et al. Citation2006). The pH of the fermentation medium is most important during the sporulation and production phase. In the germination stage, the germinating spores absorb ammonia and release protons, thereby increasing the acidity of the medium and favouring the production of citric acid. At low pH of about less than 2, the formation of unwanted products such as oxalic and gluconic acid is inhibited, and the possibility of contamination by other microorganisms is also reduced, making recovery of citric acid easier (Max et al. Citation2010). The initial pH of a medium must be optimized and defined to suit the microorganism, substrate and production technique. Dashen and colleagues showed that the substrate and production technique influences the pH kinetics, which is the complete pH-rate profile showing the pH changes in difference substrates (Dashen et al. Citation2014).
Concentration of alcohols
Moyer (Citation1953) first reported the effect of alcohols on the production of citric acid. He found out that methanol had a profound positive effect on the formation of citric acid from different carbon sources, such as crude carbohydrate sources as well as commercial glucose (Moyer Citation1953). It was further concluded that the composition of the medium and the strain of microorganism used determine the optimum amount of methanol/ethanol required, which should mostly lie between 1% and 3%.
Moyer (Citation1953) also studied the effect of ethanol on citric acid accumulation, it concluded that the addition of ethanol doubles the citrate synthetase activity, decreases the aconitase activity by 75% and slightly increases the activities of other TCA cycle enzymes. These effects of the alcohol result in increased citric acid accumulation, which is attributed to the slow degradation of the citric acid resulting from reduced aconitase activity. Acting as a carbon source, ethanol also increases the availability of carbon in the citric acid cycle. Roukas and Harvey (Citation1988) obtained maximum citric acid production at a methanol concentration of 2%, whereas a concentration higher than that led to a decrease in citric acid production. It was further concluded that the inductive effect of methanol was a result of its inhibitory effect on metal ions (Kiel et al. Citation1981). This result shows that the amount of methanol has to be limited for optimum citric acid production.
Trace elements
Studies on divalent metal ions including manganese, zinc, copper, magnesium and iron have shown that they have effects on citric acid production (Käppeli et al. Citation1978; Dronawat et al. Citation1995). Tomlinson et al. (Citation1950) concluded that the optimum concentrations of iron and zinc are 1.3 and 0.3 ppm, respectively. These authors further explained the importance of manganese for cell function, sporulation and production of secondary metabolites, and mainly in cell wall synthesis. Manganese deficiency affects the anabolism of A. niger, causing a high intracellular ammonium concentration. A decrease in the accumulation of citric acid with iron has been observed, as well as changes in mycelial growth (Mischak et al. Citation1985). Grewal and Kalra (Citation1995) deduced that at a high zinc concentration, the fungi maintained growth without accumulation of citric acid (Drysdale & McKay Citation1995). Nickel, molybdenum and cobalt are some other trace metals reported to affect the citric acid accumulation of A. niger (Habison et al. Citation1983). The interdependence of medium constituents has to be taken into account as it is crucial to citric acid production; therefore, robust control of these trace elements is required for the optimum production of citric acid.
Aeration
The highly aerobic nature of the bio-production of citric acid makes the amount of oxygen supplied a critical factor. Therefore, varying aeration rates can have adverse effects on the fermentation performance and yield (Grewal & Kalra Citation1995). At high aeration rates, there is reduced partial pressure of the dissolved carbon dioxide in the medium. Carbon dioxide is a substrate for pyruvate carboxylase as it replaces the supply of oxaloacetate for citrate synthase. The reaction catalysed by pyruvate decarboxylase produces carbon dioxide, but extreme aeration can incur some losses. Increased levels of carbon dioxide are damaging to the final biomass and concentrations of citrate (Angumeenal & Venkappayya Citation2013).
However, carbon dioxide has been shown to have a positive effect on the synthesis of citric acid (Vandenberghe et al. Citation1999). The high partial pressure of carbon dioxide impedes the liberation of spores of the filamentous fungi, thereby enhancing citric acid accumulation. However, high aeration rates result in foaming in the medium, mainly during the growth phase, requiring the use of antifoaming agents and/or mechanical defoamers. Economically, it is better to gradually increase the aeration rate, but Li et al. (Citation2014) recommend that aeration should be performed evenly across the medium with similar intensity. This is in line with the study by Kubicek et al. (Citation1980), which revealed that a disruption process (i.e. interruptions in aeration) up to 20 min during idiophase irreversibly destroyed the organism's ability to synthesize and accumulate citric acid, although the viability of the organism was maintained. The role of low aeration is meant to curb the respiratory activity of A. niger, thereby shifting the metabolism away from biomass production to the synthesis of citric acid. It was also concluded that stronger aeration results in increased sporulation and decreased accumulation of citric acid (Soccol et al. Citation2006).
Other factors
Other factors that have effects on citric acid production include lipids, such as groundnut oil (Millis et al. Citation1963; Kumar & Ethiraj Citation1976; Souza et al. Citation2014), and sodium monofluoroacetate (Meixner-Monori et al. Citation1984). Millis et al. (Citation1963) showed that lipid will improve the yield of citric acid with no effect of the dry weight of mycelium. Kareem et al. (Citation2010) showed the effects of calcium fluoride, sodium fluoride and potassium fluoride on the industrial production of citric acid. The main factors that affect citric acid production are shown in .
Table 2. Factors affecting citric acid production.
Table 3. Applications of citric acid.
Recovery of citric acid
Recovery of citric acid can be achieved by three major unit operations, namely precipitation, extraction and adsorption (mainly using ion-exchange resin). It is possible to use crystallization but it carries a risk of contamination as a result of unwanted materials from the raw material and autolysis of the microbial cells (Drysdale & McKay Citation1995). Precipitation has been the most used method for the recovery of citric acid as it is applicable to all processes, but it requires the removal of the micelles from the fungus, fermentation broth and suspended material by filtration (Dhillon et al. Citation2011b). Calcium oxide hydrate (milk lime) is added to the medium and the lime is then precipitated into tricalcium citrate tetrahydrate. Then, the precipitate is filtered and washed with water. Thereafter, it is treated in an acidulator with calcium sulphate, a sulphuric-acid forming gypsum. Next, it is filtered and the mother liquor is treated with active carbon and passed through anion and cation exchangers. It is further concentrated by evaporation in a vacuum at 40°C. Lastly, crystals of citric acid monohydrate are formed in a vacuum crystallizer at a temperature of 20–25°C and anhydrous citric acid is formed at crystallization temperatures above 36.5°C (Kubicek Citation1986; Grewal & Kalra Citation1995). The wastes from this process include calcium sulphate, and microorganism residues containing amino acids, sugar, colloid, pigment and inorganic matter, which could be supplied to cement factories and dried for use in feed factories, respectively (Dhillon et al. Citation2011b).
Solvent extraction is an alternative to the purification and crystallization of citric acid, as it has reduced environmental impacts compared with the precipitation method, by making use of n-octyl alcohol and tridodecylamine for citric acid extraction, thereby eliminating the production of gypsum. This method was also recommended by the US Food and Drug Administration for use in food and drug applications (Grewal & Kalra Citation1995). Electrodialysis makes use of electrically charged membranes, utilizing the electrical potential difference between the ionic and aqueous solution to separate them. This technique has been proven to be economical for clear fermentation media. However, it is 50% more expensive than other recovery processes such as precipitation (Amenaghawon & Aisien Citation2012). In laboratory analyses, the determination and quantification of citric acid are done using spectrophotometry (Themelis & Tzanavaras Citation2001; Jham et al. Citation2002), gas chromatography (Jham et al. Citation2002), high-performance liquid chromatography (Jham et al. Citation2002), high-resolution nuclear magnetic resonance spectroscopy (del Campo et al. Citation2006) and the traditional method of titration (Williams Citation1984).
Applications of citric acid
The versatility and non-toxicity of citric acid are its main positive characteristics. It is accepted globally as ‘generally recognized as safe’ (GRAS) and has been endorsed by the FAO/WHO committee on food additives. It cuts across various industries for various applications. Examples of citric acid applications are shown in (Grewal & Kalra Citation1995).
Conclusions
This review focuses on the details of citric acid production. The economic potential, biochemistry, choices of microorganism employed, raw materials, types of fermentation technique, factors affecting citric acid production, quantification techniques and recovery techniques are discussed. Currently, citric acid is the most produced organic acid in the world. Global production is expected to increase further with increasing demand. Although citric acid production using A. niger provides satisfactory performance at the moment, there is still room for greater improvements in increasing yield and minimizing waste by developing novel fermentation techniques and the optimization of A. niger using genetic manipulation. Microbial strains which are capable of fermenting any substrates have been developed to maximize the utilization of agro-industrial waste and provide a more efficient and environmentally friendly production process.
Disclosure statement
No potential conflict of interest was reported by the author(s).
Additional information
Funding
References
- Aghdam MG, Taherzadeh M. 2008. Production of citric acid by solid state fermentation. J Biotechnol. 136:S464–S495. doi: https://doi.org/10.1016/j.jbiotec.2008.07.1081
- Ali S, Haq I-u, Qadeer M, Iqbal J. 2002a. Production of citric acid by Aspergillus niger using cane molasses in a stirred fermentor. Electron J Biotechnol. 5:19–20.
- Ali S, Ikram Ul H, Qadeer MA, Iqbal J. 2002b. Production of citric acid by Aspergillus niger using cane molasses in a stirred fermentor. Electron J Biotechnol. 5:65–78.
- Ambati P, Ayyanna C. 2001. Optimizing medium constituents and fermentation conditions for citric acid production from palmyra jaggery using response surface method. World J Microbiol Biotechnol. 17:331–335. doi: https://doi.org/10.1023/A:1016613322396
- Amenaghawon NA, Aisien FA. 2012. Modelling and simulation of citric acid production from corn starch hydrolysate using Aspergillus niger. Environment and Natural Resources Research. 2:73–85. doi: https://doi.org/10.5539/enrr.v2n1p73
- Anastassiadis S, Morgunov IG, Kamzolova SV, Finogenova TV. 2008. Citric acid production patent review. Recent Patents on Biotechnology. 2:107–123. doi: https://doi.org/10.2174/187220808784619757
- Angumeenal A, Kamalakannan P, Prabhu HJ, Venkappayya D. 2003. Effect of transition metal cations on the production of citric acid using mixed cultures of Aspergillus niger and Candida gulliermondii. J Indian Chem Soc. 80:903–906.
- Angumeenal AR, Venkappayya D. 2013. An overview of citric acid production. LWT-Food Sci Technol. 50:367–370. doi: https://doi.org/10.1016/j.lwt.2012.05.016
- Arshad Z, Amid A, Yusof F, Jaswir I, Ahmad K, Loke S. 2014. Bromelain: an overview of industrial application and purification strategies. Appl Microbiol Biotechnol. 98:1–15. doi: https://doi.org/10.1007/s00253-014-5889-y
- Assadi MM, Nikkhah M. 2002. Production of citric acid from date pulp by solid state fermentation. J Agric Sci. 4:119–125.
- Bauweleers HMK, Groeseneken DR, Van Peij NNME. 2014. Genes useful for the industrial production of citric acid. In: Google Patents.
- Benghazi L, Record E, Suárez A, Gomez-Vidal JA, Martínez J, de la Rubia T. 2014. Production of the Phanerochaete flavido-alba laccase in Aspergillus niger for synthetic dyes decolorization and biotransformation. World J Microbiol Biotechnol. 30:201–211. doi: https://doi.org/10.1007/s11274-013-1440-z
- Berovic M, Legisa M. 2007. Citric acid production. Biotechnology Annual Review. 13:303–343. doi: https://doi.org/10.1016/S1387-2656(07)13011-8
- Boddy LM, Bergès T, Barreau C, Vainstein MH, Dobson MJ, Ballance DJ, Peberdy JF. 1993. Purification and characterisation of an Aspergillus niger invertase and its DNA sequence. Curr Genet. 24:60–66. doi: https://doi.org/10.1007/BF00324666
- del Campo G, Berregi I, Caracena R, Santos JI. 2006. Quantitative analysis of malic and citric acids in fruit juices using proton nuclear magnetic resonance spectroscopy. Anal Chim Acta. 556:462–468. doi: https://doi.org/10.1016/j.aca.2005.09.039
- Chen H, He X, Geng H, Liu H. 2014. Physiological characterization of ATP-citrate lyase in Aspergillus niger. J Ind Microbiol Biotechnol. 41:1–11. doi: https://doi.org/10.1007/s10295-013-1371-6
- Cleland WW, Johnson MJ. 1954. Tracer experiments on the mechanism of citric acid formation by Aspergillus niger. J Biol Chem. 208:679–689.
- Darouneh E, Alavi A, Vosoughi M, Arjmand M, Sifkordi A, Rabaji R. 2009. Citric acid production: surface culture versus submerged culture. African Journal of Microbiology Research. 3:541–545.
- Dashen M, Ado SA, Ameh J, Amapu T, Zakari H. 2014. Screening and improvement of local isolates of Aspergillus niger for citric acid production. Bayero J Pure Appl Sci. 6:105–111. doi: https://doi.org/10.4314/bajopas.v6i1.22
- Dhillon GS, Brar SK, Verma M, Tyagi RD. 2011a. Recent advances in citric acid bio-production and recovery. Food Bioprocess Technol. 4:505–529. doi: https://doi.org/10.1007/s11947-010-0399-0
- Dhillon GS, Brar SK, Verma M, Tyagi RD. 2011b. Utilization of different agro-industrial wastes for sustainable bioproduction of citric acid by Aspergillus niger. Biochem Eng J. 54:83–92. doi: https://doi.org/10.1016/j.bej.2011.02.002
- Dronawat SN, Svihla CK, Hanley TR. 1995. The effects of agitation and aeration on the production of gluconic acid by Aspergillus niger. Appl Biochem Biotechnol. 51-52:347–354. doi: https://doi.org/10.1007/BF02933438
- Drysdale C, McKay A. 1995. Citric acid production by Aspergillus niger in surface culture on inulin. Lett Appl Microbiol. 20:252–254. doi: https://doi.org/10.1111/j.1472-765X.1995.tb00440.x
- Falony G, Armas JC, Mendoza JCD, Hernández JLM. 2006. Production of extracellular lipase from Aspergillus niger by solid-state fermentation. Food Technol Biotech. 44:235–240.
- Grewal H, Kalra K. 1995. Fungal production of citric acid. Biotechnol Adv. 13:209–234. doi: https://doi.org/10.1016/0734-9750(95)00002-8
- Grimaux E, Adam P. 1880. Synthese der Citronensäure. J Prakt Chem. 22:105–107. doi: https://doi.org/10.1002/prac.18800220105
- Gruben BS, Zhou M, Wiebenga A, Ballering J, Overkamp KM, Punt PJ, de Vries RP. 2014. Aspergillus niger RhaR, a regulator involved in l-rhamnose release and catabolism. Appl Microbiol Biotechnol. 98:1–10. doi: https://doi.org/10.1007/s00253-013-5346-3
- Gupta J, Heding L, Jorgensen O. 1975. Effect of sugars, hydrogen ion concentration and ammonium nitrate on the formation of citric acid by Aspergillus niger. Acta Microbiologica Academiae Scientiarum Hungaricae. 23:63–67.
- Habison A, Kubicek CP, Rohr M. 1983. Partial purification and regulatory properties of phosphofructokinase from Aspergillus niger. Biochem J. 209:669–676.
- Hang Y, Luh B, Woodams E. 1987. Microbial production of citric acid by solid state fermentation of kiwifruit peel. J Food Sci. 52:226–227. doi: https://doi.org/10.1111/j.1365-2621.1987.tb14014.x
- Hang Y, Splittstoesser D, Woodams E, Sherman R. 1977. Citric acid fermentation of brewery waste. J Food Sci. 42:383–384. doi: https://doi.org/10.1111/j.1365-2621.1977.tb01504.x
- Hayashi S, Nakamura S. 1981. Multiple forms of glucose oxidase with different carbohydrate compositions. Biochim Biophys Acta (BBA)-Enzymology. 657:40–51. doi: https://doi.org/10.1016/0005-2744(81)90128-5
- Hossain M, Brooks J, Maddox I. 1984. The effect of the sugar source on citric acid production by Aspergillus niger. Appl Microbiol Biotechnol. 19:393–397. doi: https://doi.org/10.1007/BF00454376
- Ikram-ul H, Ali S, Qadeer M, Iqbal J. 2004. Citric acid production by selected mutants of Aspergillus niger from cane molasses. Bioresour Technol. 93:125–130. doi: https://doi.org/10.1016/j.biortech.2003.10.018
- Jernejc K, Cimerman A, Perdih A. 1992. Composition of Aspergillus niger mycelium during growth on productive and unproductive substrates. J Biotechnol. 25:341–348. doi: https://doi.org/10.1016/0168-1656(92)90166-7
- Jham GN, Fernandes SA, Garcia CF, Silva AAd. 2002. Comparison of GC and HPLC for the quantification of organic acids in coffee. Phytochem Anal. 13:99–104. doi: https://doi.org/10.1002/pca.629
- Käppeli O, Müller M, Fiechter A. 1978. Chemical and structural alterations at the cell surface of Candida tropicalis, induced by hydrocarbon substrate. J Bacteriol. 133:952–958.
- Kareem S, Akpan I, Alebiowu O. 2010. Production of citric acid by Aspergillus niger using pineapple waste. Malaysian Journal of Microbiology. 6:161–165.
- Kiel H, Guvrin R, Henis Y. 1981. Citric acid fermentation by Aspergillus niger on low sugar concentrations and cotton waste. Applied and Environmental Microbiology. 42:1–4.
- Kishore KA, Kumar MP, Krishna VR, Reddy GV. 2008. Optimization of process variables of citric acid production using Aspergillus Niger in a batch fermentor. Engineering Letters. 16:572–577.
- Kristiansen B, Sinclair C. 1979. Production of citric acid in continuous culture. Biotechnol Bioeng. 21:297–315. doi: https://doi.org/10.1002/bit.260210214
- Kubicek C. 1986. The role of the citric acid cycle in fungal organic acid fermentations. Proceedings of the Biochemical Society Symposium.
- Kubicek CP, Röhr M. 1977. Influence of manganese on enzyme synthesis and citric acid accumulation in Aspergillus niger. European Journal of Applied Microbiology and Biotechnology. 4:167–175. doi: https://doi.org/10.1007/BF01390476
- Kubicek CP, Zehentgruber O, El-Kalak H, Röhr M. 1980. Regulation of citric acid production by oxygen: effect of dissolved oxygen tension on adenylate levels and respiration in Aspergillus niger. European Journal of Applied Microbiology and Biotechnology. 9:101–115. doi: https://doi.org/10.1007/BF00503505
- Kubicek-Pranz EM, Mozelt M, Rōhr M, Kubicek CP. 1990. Changes in the concentration of fructose 2,6-bisphosphate in Aspergillus niger during stimulation of acidogenesis by elevated sucrose concentration. Biochim Biophys Acta, Gen Subj. 1033:250–255. doi: https://doi.org/10.1016/0304-4165(90)90128-J
- Kumar K, Ethiraj S. 1976. Influence of methanol and groundnut oil on citric acid production from sugar cane juice by Aspergillus niger. International Sugar Journal. 78:13–15.
- La Nauze JM. 1966. Aconitase and isocitric dehydrogenases of Aspergillus niger in relation to citric acid production. J Gen Microbiol. 44:73–81. doi: https://doi.org/10.1099/00221287-44-1-73
- Legiša M, Mattey M. 1986. Glycerol as an initiator of citric acid accumulation in Aspergillus niger. Enzyme Microb Technol. 8:258–259. doi: https://doi.org/10.1016/0141-0229(86)90018-9
- Li C, Xu D, Zhao M, Sun L, Wang Y. 2014. Production optimization, purification, and characterization of a novel acid protease from a fusant by Aspergillus oryzae and Aspergillus niger. Eur Food Res Technol. 238:1–13. doi: https://doi.org/10.1007/s00217-013-2099-2
- Lu MY, Maddox IS, Brooks JD. 1998. Application of a multi-layer packed-bed reactor to citric acid production in solid-state fermentation using Aspergillus niger. Process Biochem. 33:117–123 . doi: https://doi.org/10.1016/S0032-9592(97)00037-X
- Majumder L, Khalil I, Munshi MK, Alam K, Rashid H, Begum R, Alam N. 2010. Citric acid production by Aspergillus niger using molasses and pumpkin as substrates. European Journal of Biological Sciences. 2:01–08.
- Martin SM, Wilson PW. 1951. Uptake of C14O2 by Aspergillus niger in the formation of citric acid. Arch Biochem Biophys. 32:150–157. doi: https://doi.org/10.1016/0003-9861(51)90248-2
- Mattey M. 1977. Citrate regulation of citric acid production in Aspergillus niger. FEMS Microbiol Lett. 2:71–74. doi: https://doi.org/10.1111/j.1574-6968.1977.tb00910.x
- Mattey M. 1992. The production of organic acids. Crit Rev Biotechnol. 12:87–132. doi: https://doi.org/10.3109/07388559209069189
- Max B, Salgado JM, Rodríguez N, Cortés S, Converti A, Domínguez JM. 2010. Biotechnological production of citric acid. Brazilian Journal of Microbiology. 41:862–875. doi: https://doi.org/10.1590/S1517-83822010000400005
- Mazinanian N, Odnevall Wallinder I, Hedberg Y. 2015. Comparison of the influence of citric acid and acetic acid as simulant for acidic food on the release of alloy constituents from stainless steel AISI 201. J Food Eng. 145:51–63. doi: https://doi.org/10.1016/j.jfoodeng.2014.08.006
- Meixner-Monori B, Kubicek CP, Röhr M. 1984. Pyruvate kinase from Aspergillus niger: a regulatory enzyme in glycolysis? Can J Microbiol. 30:16–22. doi: https://doi.org/10.1139/m84-003
- Millis NF, Trumpy BH, Palmer BM. 1963. The effect of lipids on citric acid production by an Aspergillus niger mutant. J Gen Microbiol. 30:365–379. doi: https://doi.org/10.1099/00221287-30-3-365
- Mischak H, Kubicek CP, Röhr M. 1985. Formation and location of glucose oxidase in citric acid producing mycelia of Aspergillus niger. Appl Microbiol Biotechnol. 21:27–31.
- Moyer AJ. 1953. Effect of alcohols on the mycological production of citric acid in surface and submerged culture. Culture. 8:5–10.
- Nigam PSn. 2009. Production of organic acids from agro-industrial residues. Biotechnology for Agro-Industrial Residues Utilisation. 9:37–60. doi: https://doi.org/10.1007/978-1-4020-9942-7_3
- Papagianni M. 2007. Advances in citric acid fermentation by Aspergillus niger: biochemical aspects, membrane transport and modeling. Biotechnol Adv. 25:244–263. doi: https://doi.org/10.1016/j.biotechadv.2007.01.002
- Papagianni M, Wayman F, Mattey M. 2005. Fate and role of ammonium ions during fermentation of citric acid by Aspergillus niger. Applied and Environmental Microbiology. 71:7178–7186. doi: https://doi.org/10.1128/AEM.71.11.7178-7186.2005
- Pazouki M, Panda T. 1998. Recovery of citric acid–a review. Bioprocess Eng. 19:435–439. doi: https://doi.org/10.1007/PL00009029
- Punekar N, Vaidyanathan C, Appaji Rao N. 1984. Mechanisms of citric acid fermentation by Aspergillus niger. J Sci Ind Res. 43:398–404.
- Ratledge C, Kristiansen B. 2001. Basic biotechnology. Cambridge University Press.
- Rohr M, Kubicek CP. 1981. Regulatory aspects of citric acid fermentation by Aspergillus niger. Process Biochem. 16:34–37.
- Roukas T. 1991. Production of citric acid from beet molasses by immobilized cells of Aspergillus niger. J Food Sci. 56:878–880. doi: https://doi.org/10.1111/j.1365-2621.1991.tb05409.x
- Roukas T, Harvey L. 1988. The effect of pH on production of citric and gluconic acid from beet molasses using continuous culture. Biotechnol Lett. 10:289–294. doi: https://doi.org/10.1007/BF01024422
- Rubio MC, Maldonado MC. 1995. Purification and characterization of invertase from Aspergillus niger. Current Microbiology. 31:80–83. doi: https://doi.org/10.1007/BF00294280
- Sakurai A, Imai H, Ejiri T, Endoh K, Usami S. 1991. Citric acid production by surface culture using Aspergillus niger: kinetics and simulation. J Ferment Bioeng. 72:15–19. doi: https://doi.org/10.1016/0922-338X(91)90139-8
- Sangsurasak P, Mitchell D. 1995. The investigation of transient multidimensional heat transfer in solid state fermentation. Chem Eng J/Biochem Eng J. 60:199–204. doi: https://doi.org/10.1016/0923-0467(95)03016-6
- Sauer M, Porro D, Mattanovich D, Branduardi P. 2008. Microbial production of organic acids: expanding the markets. Trends Biotechnol. 26:100–108. doi: https://doi.org/10.1016/j.tibtech.2007.11.006
- Schreferl-Kunar G, Grotz M, Röhr M, Kubicek CP. 1989. Increased citric acid production by mutants of Aspergillus niger with increased glycolytic capacity. FEMS Microbiol Lett. 59:297–300. doi: https://doi.org/10.1111/j.1574-6968.1989.tb03128.x
- Shu P, Johnson MJ. 1948a. Citric acid. Ind Eng Chem. 40:1202–1205. doi: https://doi.org/10.1021/ie50463a008
- Shu P, Johnson MJ. 1948b. The interdependence of medium constituents in citric acid production by submerged fermentation. J Bacteriol. 56:577–585.
- Soccol CR, Vandenberghe LPS, Rodrigues C, Pandey A. 2006. New perspectives for citric acid production and application. Food Technology & Biotechnology. 44:141–149.
- Souza KST, Schwan RF, Dias DR. 2014. Lipid and citric acid production by wild yeasts grown in glycerol. J Microbiol Biotechnol. 4:497–506. doi: https://doi.org/10.4014/jmb.1310.10084
- Steinböck F, Choojun S, Held I, Roehr M, Kubicek CP. 1994. Characterization and regulatory properties of a single hexokinase from the citric acid accumulating fungus Aspergillus niger. Biochim Biophys Acta, Gen Subj. 1200:215–223. doi: https://doi.org/10.1016/0304-4165(94)90138-4
- van der Straat L, Vernooij M, Lammers M, van den Berg W, Schonewille T, Cordewener J, van der Meer I, Koops A, de Graaff LH. 2014. Expression of the Aspergillus terreus itaconic acid biosynthesis cluster in Aspergillus niger. Microbial Cell Factories. 13:1–9. doi: https://doi.org/10.1186/1475-2859-13-11
- Themelis DG, Tzanavaras PD. 2001. Reagent-injection spectrophotometric determination of citric acid in beverages and pharmaceutical formulations based on its inhibitory effect on the iron (III) catalytic oxidation of 2,4-diaminophenol by hydrogen peroxide. Anal Chim Acta. 428:23–30. doi: https://doi.org/10.1016/S0003-2670(00)01221-6
- Tomlinson N, Campbell JJR, Trussell PC. 1950. The influence of zinc, iron, copper, and manganese on the production of citric acid by Aspergillus niger. J Bacteriol. 59:217–227.
- Torrado AM, Cortés S, Salgado JM, Max B, Rodríguez N, Bibbins BP, Converti A, Domínguez JM. 2011. Citric acid production from orange peel wastes by solid-state fermentation. Brazilian Journal of Microbiology. 42:394–409. doi: https://doi.org/10.1590/S1517-83822011000100049
- Vandenberghe LP, Soccol CR, Pandey A, Lebeault J-M. 1999. Microbial production of citric acid. Brazilian Archives of Biology and Technology. 42:263–276.
- Vergano MF, Soria M, Kerber N. 1996. Short communication: influence of inoculum preparation on citric acid production by Aspergillus niger. World J Microbiol Biotechnol. 12:655–656. doi: https://doi.org/10.1007/BF00327734
- Weyda I, Lübeck M, Ahring BK, Lübeck PS. 2014. Point mutation of the xylose reductase (XR) gene reduces xylitol accumulation and increases citric acid production in Aspergillus carbonarius. J Ind Microbiol Biotechnol. 41:1–7. doi: https://doi.org/10.1007/s10295-014-1415-6
- Williams S. 1984. Official methods of analysis of the Association of Official Analytical Chemists.
- Wojtatowicz M, Marchin G, Erickson L. 1993. Attempts to improve strain A-101 of Yarrowia lipolytica for citric acid production from n-paraffins. Process Biochem. 28:453–460. doi: https://doi.org/10.1016/0032-9592(93)85028-E
- Xu D-B, Madrid CP, Röhr M, Kubicek CP. 1989. The influence of type and concentration of the carbon source on production of citric acid by Aspergillus niger. Appl Microbiol Biotechnol. 30:553–558.