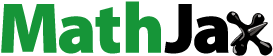
Abstract
This study reports the removal of nickel(II) and copper(II) ions (Ni2+ and Cu2+) from aqueous solution using pure and chemically pretreated biomass from Arachis hypogea (peanut shells), Prunus amygdalus (almond shells), Arundo donax (giant cane) and two clay materials, clay G and clay B. These materials are indigenous, easily available, surpulus by-products for biosorption studies. Batch experiments were carried out to determine the effect of various adsorbent factors such as initial pH, temperature, particle size and contact time on the adsorption process. For adsorption application, up to 99% removal of both metal ions was achieved by biomass and clay materials. Furthermore, chemically modified adsorbents significantly increased the uptake capacity of biomass, suggesting that the affinity between metal and sorbent can be increased after pretreatment. Equilibrium isotherms were analyzed using Langmuir and Freundlich isotherm models, and both models fitted to explain the adsorption behavior of metal ions on to biomass and clay. This shows that the adsorption of metal ions on the adsorbent is a physical adsorption mechanism. In conclusion, owing to its outstanding nickel(II) and copper(II) uptake capacity, the utilized biomass proved to be an excellent biosorbent.
Introduction
Pollution, defined as an undesirable change in the natural functioning of the environment owing to the addition of detrimental particulates, can be categorized into three types: environmental (land), atmospheric (air) and marine (water). Water covers 70% of the earth's surface so in great danger of contamination, which creates major problems. Waste water is a combined term used for spent water released from the community, homes, farms and factories. It usually contains organic compounds, heavy metals, sewage organisms, including bacteria and fungi, and petroleum products, along with dissolved and suspended particles, nutrients and hazardous materials. One of the major sources of waste water pollution is industrial waste water. Many industries, such as textile, pulp and paper, tanning, sugar, fertilizer and rubber industries, release huge volumes of waste water (Haq et al. Citation2011). The use of large volumes of waste water by millions of large-scale farms has increased owing to a lack of any alternative source for irrigation purposes.
‘Heavy metals’ is a general collective term applied to the group of metals and metalloids with an atomic density greater than 6 g cm−3, which is widely recognized and usually applied to elements such as cadmium, chromium, copper (Cu), mercury, nickel (Ni), lead and zinc, that are commonly associated with pollution and toxicity problems (Connell et al. 2008). These toxic chemicals in the waste water have adverse effects on human health and the environment. Extensive use of waste water for irrigation creates an issue of concern to agencies responsible for maintaining public and environmental health (Afkhami et al. Citation2008).
Plants play an important role in cleaning up soil, sediment and water polluted with heavy metals. However, this is a long-term procedure and regeneration of plants, or phytoremediation, is difficult. Plants cannot be used as a cost-effective treatment method for heavy metal removal as they achieve incomplete metal removal (Ahalya et al. Citation2003). Nickel (II) and copper (II) are known environmental pollutants, and their removal from waste water is of great importance. Conventional methods such as precipitation, oxidation/reduction, ion exchange, filtration, membranes and evaporation are extremely expensive or inefficient for metal removal from dilute solutions containing 1–100 mg l–1 of dissolved metal.
The search for new techniques is focusing on biosorption, which is the removal of metal or metalloid species, compounds and particulates from solution by biological material. Adsorption is evolving as a front line of defense. Selective adsorption utilizing biological materials, mineral oxides, activated carbons or polymer resins has generated increasing excitement (Gadd Citation1990). Adsorption is a well-established separation technique, defined as the ability of the adsorbate to attach with the adsorbent. The technique works not only for the removal of dilute pollutants but also for the recovery of useful products from aqueous streams (Chia & Shen Citation2000). Adsorption is divided into two types. One is physiosorption or physical adsorption, and other is chemisorption, which is much stronger than physiosorption as molecules are held to the surface by valence forces. Heat evolved during the chemosorption process ranges from 10 to 100 kcal mol−1, whereas physiosorption evolves less than 5 kcal mol–1 (Motoyuki Citation1990).
Biosorbents show different affinities towards sorbate species (sorbate, e.g. metal ions). The biosorption process involves a solid phase (sorbent or biosorbent, i.e. biological material) and a liquid phase (solvent, normally water). Owing to the higher affinity of the sorbent for the sorbate species, the latter is attracted and bound into the solid (Kratochvil & Velosky Citation1998). The rate of biosorption depends on the type of process. Various mechanisms can operate simultaneously to varying degrees in biosorption (Gadd Citation2009). The origin of the biomass should be kept in mind when designing a metal biosorption experiment (Volesky Citation1987). Considering the number of types of biomass and the metal of interest, all multiplied by the number of experimental parameters, there is very large scope for potential new metal biosorbents (Volesky & Holan Citation1995). Cassia fistula, used as a biosorbent for Ni2+ uptake from aqueous solution, showed excellent removal capacity (Hanif et al. Citation2007; Mushtaq et al. Citation2014). For cadmium, lead and zinc removal, Aspergillus flavus biomass proved excellent in a shaking flask experiment (Kok et al. Citation2001; Tahira et al. Citation2015). Photosynthetic bacteria such as Rhodobacter sphaeroides S and a marine photosynthetic bacterium, Rhodovulum sp. PS88, in a batch culture system achieved a high removal ratio of cadmium from culture medium under anaerobic and aerobic conditions (Watanabe et al. Citation2003). The present research visualizes the use of dead biomass for the removal of heavy metals from aqueous solutions. To account for the problem of heavy metals, different materials have been applied as cheap biosorbents.
High levels of water pollution can destroy aquatic life. Pollutants are taken up by plants and animals and can enter the human body, resulting in many health problems. The situation is worse in countries where people do not have access to potable water, and in many instances polluted water is used as a source of drinking water (Faryal et al. Citation2015; Kousar et al. Citation2015).
In this article, several different adsorbents were tested: shells of almond (Prunus amygdalus), an important stone fruit grown in Pakistan; shells of peanut or groundnut (Arachis hypogaea), a species in the legume or ‘bean' family (Fabaceae), which is an annual herbaceous plant growing 30–50 cm (0.98–1.6 feet) tall; giant cane (Arundo donax), a tall perennial cane that grows in damp, either fresh or moderately saline, soils (Bell Citation1997; Alden et al. Citation1998); and clay material (clay G and clay B) doped with different silicate minerals of heavy metals, which has been shown to be excellent in the removal of heavy metals from aqueous effluents. These adsorbents are inexpensive and easily available throughout the year, and offer a cost-effective alternative to the conventional treatment of waste water from industry (Ashraf et al Citation2013a). The main objective of this study was to study the efficiency of biosorbents in the removal of heavy metals and to assess their potential as a cheap treatment method.
Material and methods
Reagents
For both metals (Ni2+ and Cu2+), stock solutions of their chlorides (1000 mg l–1) were prepared by dissolving molar amounts in volumetric flasks. From the stock solutions, intermediate solutions of different concentration were prepared in double-distilled water by applying the dilution formula:
(1)
(1)
Acidic solutions of 0.1 M HCl, 0.1 M CH3COOH, 0.1 M methanol and 0.1 M acetone were prepared using the following formula:
(2)
(2)
Concentrated acid was diluted using M1V1 = M2V2. All the chemicals used were of analytical grade from the Merck Index.
Pretreatment of biomass
All the adsorbents of plant origin used in the study were obtained from a local market and prepared before being used for treatment. Arachis hypogaea (peanut) shells were washed thoroughly to remove dust and foreign particles, then washed with distilled water, dried in sunlight for 7 days, and ground to a fine powder with a grinder. Washed P. amygdalus (almond) shells were dried in sunlight for 15 days then oven-dried at 150°C. Shells were first ground in a pestle and mortar, then ground to a powder in a grinder. Arundo donax (giant cane) was washed and dried in sunlight for 10 days, then in an oven at 50°C, and finally ground to a powder in a grinder.
Modification of clay
Clay material (clay G and B) doped with different silicate minerals was modified by treatment with nitric acid and kept for 6 hours in sunlight. Once dry, it was cooked at 1500°C for 24 hours continuously. Cooked clay was modified into a granular mass.
Batch biosorption studies
Batch experiments for nickel(II) and copper(II)
Batch experiments were carried out using a series of Erlenmeyer flasks of 100 ml and 250 ml capacity. The effects of pH (2–6), contact time (1.30 min), initial nickel and copper concentrations (2 ppm, 4 ppm and 6 ppm), adsorbent dose (0.05 g, 0.1 g, 0.2 g and 0.3 g) and temperature on adsorption of the material ions from the solutions were investigated during the study. All the adsorption experiments were carried out at room temperature (30 ± 1°C), except where the effect of temperature being investigated. All the flasks were placed on a rotating shaker (PA 250/25 H) with a constant shaking speed of 120 rpm. At the end of the experiment, the flasks were removed from the shaker and the solutions were separated from the biomass by filtration through filter paper (Whatman no. 40, ashless) followed by centrifugation. To adjust the pH of the medium, 0.1 N solutions of NaOH and HCl were used.
Cellulose modification
Adsorbent (almond shells, 100 mesh size) was washed in acetone and dried in an oven at 90°C for 1 hour. Then, using a 100 ml reflux set, 2.2 g of adsorbent, 8.2 g of succinic anhydride and 42 ml of pyridine were added. A condenser was attached and reflux was run for 24 hours. After completion of the reaction, Gooch filtration was performed, followed by washing. The washed modified biomass was dried in an oven at 80°C for 1 hour and left in a desiccator overnight.
Cellulose (peanut shells) preparation chemically modified with succinic anhydride
Adsorbent (peanut shells, 20 mesh size) was washed with acetone and dried in an oven at 80°C for 1 hour. Using a reflux set, 5 g of biomass, 16 g of succinic anhydride and 84 ml of pyridine were added. Reflux was run for 24 hours. The prepared biomass was filtered using Gooch filtration apparatus. The modified product was dried in an oven at 89°C for 1 hour and placed in a desiccator overnight.
Modification in NaOH/urea
Adsorbent was washed with acetone and dried in an oven at 90°C for 40 minutes. A mixture of 6 g NaOH, 4 g urea in 90 ml distilled water and 5 g of adsorbent (almond shells, 100 mesh size) was refluxed for 48 hours. The solution was then filtered and washed with water. The same procedure was repeated for 10 mesh size peanut shells.
Uptake capacity
The quality of sorbent material is judged according to how much sorbate it can attract and retain in an immobilized form. For this, it is customary to determine the metal uptake sorption capacity (q) by the adsorbent as the amount of sorbate bound by the unit of solid phase. The amount of metal bound by the sorbent which disappears from the solution is calculated based on the mass balance for the sorbent in the system, using Equation (Equation3(3)
(3) ):
(3)
(3)
where q is metal ion uptake capacity (mg g−1), Ci is the initial concentration of metal in solution before the sorption analysis (mg l−1), Cf is the final concentration of metal in solution after the sorption analysis (mg l−1), S is the dry weight of adsorbent (g), and V is the solution volume (l).
All the samples were analyzed for nickel and copper concentrations using a Perkin Elmer A Analyst atomic absorption spectrophotometer.
Statistical analysis
Langmuir and Freundlich models were used to characterize the adsorption for single metal component systems. The Langmuir model makes assumptions such as monolayer adsorption and constant adsorption energy, while the Freundlich model deals with heterogeneous adsorption. The Langmuir equation of adsorption is shown in Equation (Equation4(4)
(4) ):
(4)
(4)
It was linearized to the form shown in Equation (Equation5(5)
(5) ):
(5)
(5)
where qmax and b are the Langmuir constants.
The Freundlich equation of the adsorption isotherm is shown in Equation (Equation6(6)
(6) ):
(6)
(6)
Its linearized form is represented by Equation (Equation7(7)
(7) ):
(7)
(7)
where q is the amount adsorbed per unit mass of adsorbent and Cf is the equilibrium concentration. The plot of log q versus log Cf is linear, and constants K and n are evaluated from slopes and intercepts.
Results and discussion
This study tested the efficiency of five non-conventional adsorbents of plant origin and clay material for the removal of metal ions, nickel (Ni2+) and copper (Cu2+), from industrial waste water. The main purpose was to develop cheap and useful metal ion adsorbents that are readily available and economically affordable. Five adsorbents – peanut shells (A. hypogea), almond shells (P. amygdalus), giant cane (A. donax) and two clay materials – were tested for their metal ion removal efficiency in aqueous solution. The results for biosorption of Ni2+ by A. hypogea as a function of initial concentration of control flasks or blank solutions were expressed as Langmuir and Freundlich isotherms. Uptake capacity increased to 0.6314 mg g–1 and removal of Ni2+ from solution was higher at low initial concentration, with a maximum uptake of 98.70% at 1 mg l−1 concentration. Both models describe the experimental data as the value of the coefficient of determination, being 0.9619 and 0.972 for the Langmuir and Freundlich isotherms, respectively. The graph reveals the relationship between initial concentration, percentage removal and metal ion uptake capacity.
The rate of adsorption is a function of the initial concentration of metal ions, which makes it an important factor to consider for effective adsorption (Ahalya et al. Citation2005; Ashraf et al. Citation2010; Ashraf et al. Citation2011). From , in general, the data reveal that the sorption capacity increased with increasing initial metal ion concentration. The table also shows a comparison of uptake capacity (mg g−1) and percentage removal of metal ions by different adsorbents on sorbents. This sorption characteristic shows that surface saturation was dependent on the initial metal ion concentrations. At low concentrations, adsorption sites took up the available metal more quickly. However, at higher concentrations, metal ions need to diffuse to the biomass surface by intraparticle diffusion and greatly hydrolyzed ions will diffuse at a slower rate (Horsefall & Spiff Citation2005). It was found that when the metal ion concentration was low the metal ion adsorption rate increased, whereas when the metal ion concentration was high the metal removal rate decreased. Tables and show individual uptake capacity values for Cu2+ and Ni2+ for all adsorbents. The order of selectivity for metal ions towards the studied adsorbents observed was Ni > Cu.
Table 1. Comparison of Langmuir and Freundlich isotherm models for Ni2+ and Cu2.
Table 2. Adsorption of Cu2+ on chemically modified material.
Table 3. Adsorption of Ni2+ on chemically modified material.
Figures and show the effect of initial pH on the adsorption of metal ions to the adsorbent. Metal ion adsorption by most adsorbents used in the study did not occur below pH 2. Metal adsorption between 2 and 5 was optimal. From , the adsorption pH for Ni2+ was 4 for most adsorbents. Above pH 7, precipitation of Ni2+ was evident (Ashraf et al. Citation2012; Ashraf et al. Citation2013b). Similar behavior was shown by the leached biomass but the percentage of Ni2+ removal was much higher than by the fresh biomass. The increase in percentage removal of metal ions due to increased pH may be explained on the basis of a decrease in competition between protons (H+) and positively charged metal ions at the surface sites, and also by a decrease in positive charge near the surface, which results in a lower repulsion of the adsorbing metal ion. Maximum percentage removal of nickel was observed at pH 4, which could be due to the formation of M(OH) and M(OH)2 as hydrolyzed products. The lower solubility of these hydrolyzed species may be another reason for maximum adsorption. The results are supported by a previous study conducted on Cassia fistula biomass in its natural form, whose uptake capacity for Ni2+ increased at pH 3–6 and decreased at pH 7–8, showing 99–100% removal efficiency (Hanif et al. Citation2007). The results also show that the uptake of free ionic Ni2+ depends on pH. It was observed that the uptake of Ni2+ increased with the increase in pH by 99% over the pH range 2–5. This increase may be due to the presence of negative charge on the surface of the adsorbent, which may be responsible for metal binding. However, as the pH falls, the hydrogen ions compete with the metal ions for the sorption sites in the sorbent; the overall surface charge on the particles becomes positive and hinders the binding of positively charged metal ions. On the other hand, the decrease in adsorption under pH 4 may be due to occupation of the adsorption sites by anionic species, which impedes the approach of such ions towards the sorbent surface (Ashraf et al. Citation2013b; Anjum et al. Citation2015). Copper showed different behavior at different pH values for all adsorbents. Copper adsorption increased as the pH increased.
The results for acid–base-modified peanut shells as adsorbents for metal removal indicate 19–34% higher average uptake values than unmodified shells. Peanut shells modified by 0.6 M citric acid or 0.6 M phosphoric acid have shown promise as metal ion adsorbents. The adsorption of Cu2+ and Ni2+ ions from aqueous solutions on to activated carbon from peanut shells was also studied as a function of the concentration of the ions and the pH value (Luis et al. Citation2009).
Chemical modification of peanut and almond shells
In the first experiment, adsorption of heavy metals from aqueous solution using adsorbents (almond shells) chemically modified with succinic anhydride resulted in the formation of different components, separated by filtration. Separated components were residue, modified product and gel material. Different tests were performed on these components.
Melting point
The melting point for the residue showed that instead of melting, it decomposed at 199°C. The gel became red hot at 180°C and the modified product decomposed at 280°C.
Solubility
Components were tested for their solubility in different solvents, e.g. water, methanol, ethanol, acetone, dimethylglyoxime (DMG), dimethyl sulfoxide (DMSO), carbon tetrachloride (CCl4) and chloroform (CHCl3). The residue was insoluble in all solvents, but soluble in acetone and DMSO on heating. The product was also insoluble in all solvents, except for DMG and DMSO.
Thin-layer chromatography
All three components were tested by thin-layer chromatography. Some differences in the flow rate were seen, suggesting changes in the structure of the adsorbent. Functional groups may show some changes and new sites may be present.
Adsorption
Experiments were also performed for adsorption of Ni2+ and Cu2+ from aqueous solution using material chemically modified with succinic anhydride (almond shells). The modified product was analyzed and the results are shown in and . These tables show the concentration of copper and nickel adsorbed on to the product. The initial concentration of copper was 3 ppm and that of nickel was 5 ppm. Modified product and gel adsorbed about 97% of the metal. Both modified products also show adsorption to nickel of up to 80%. The results were better for copper metal than for nickel. These results suggest that considerable changes have occurred in the modified product.
The same experiment was performed on peanut shells, and the results are shown in . The table shows the concentration of copper and nickel adsorbed on to the peanut product. The initial concentration of copper was 3 ppm and that of nickel was 5 ppm. Unmodified reagent adsorbed 66.31% of Cu2+, modified product adsorbed 81.90% of Cu2+, and other washed materials adsorbed a maximum of 62.53% and 91% of Cu2+.
Table 4. Adsorption of Cu2+ on chemically modified material from peanut shells.
Both modified products also showed adsorption to Ni2+. The results are shown in . Modified product showed maximum adsorption of 98% of Ni2+. The results were better for Ni2+ than for Cu2+.
Table 5. Adsorption of Ni2+ on chemically modified material from peanut shells.
Another experiment was performed on biomass in which the product was chemically modified with NaOH/urea. The results are shown in . shows the adsorption of products formed during experiments on Cu2+. Two adsorbents (peanut shells, almond shells) were tested in the experiment. Peanut shells showed better results than almond shells.
From the above three experiments, it is concluded that there is the possibility of some modification in the structure of adsorbents.
Table 6. Synthesis of adsorbents in NaOH/urea aqueous solution.
Comparison of unmodified and modified adsorbents
Removal percentages of metals in the first two experiments were 94.82% and 66.31% Cu2+ and 65.8% Ni2+ on unmodified adsorbent, and 97.48% and 81.90% Cu2+ and 78.22% and 98.06% Ni2+ on modified adsorbent. In the third experiment, almond shells and peanut shells showed 94.16% and 94.95% Cu2+ removal.
Applications of adsorbents
Five adsorbents were applied on industrial effluent from electroplating and tanning to remove heavy metals such as Cu2+ and Ni2+.
Electroplating effluent contained initial concentrations of 1.5247 ppm Cu2+ and 1.7294 ppm Ni2+. The adsorbents used in this study, P. amygdalus, A. hypogea, A. donax, clay G and clay B, showed metal uptake concentrations of 0.0057 ppm, 0.0093 ppm, 0.0072 ppm, 0.0077 ppm and 0.0275 ppm Cu2+, and 0.0031 ppm, 0.0105 ppm, 0.0142 ppm, 0.01875 ppm and 0.0252 ppm Ni2+, respectively.
Tannery waste contained initial concentrations of 1.6324 ppm Cu2+ and 1.2460 ppm Ni2+. The adsorbents, P. amygdalus, A. hypogea, A. donax, clay G and clay B, showed metal uptake concentrations of 0.8210 ppm, 0.9722 ppm, 0.8124 ppm and 0.5754 ppm Cu2+, and 0.7651 ppm, 0.0523 ppm, 1.0372 ppm, 1.1982 ppm and 1.1324 ppm Ni2+, respectively. These results are in agreement with the study by Ilhan et al. (Citation2004).
Conclusion
All of the adsorbents used in the study proved useful for the removal of metal ions from industrial waste water. Of the adsorbents tested, P. amygdalus showed better results as a biosorbent for Ni2+ ions than for Cu2+, after some chemical modification. Further studies on these adsorbents are recommended.
Disclosure statement
The authors certify that there is no conflict of interest with any financial organization regarding the material discussed in the paper.
Additional information
Funding
References
- Afkhami A, Madrakin T, Karimi Z. 2008. Effect of the impregnation of carbon with ethylenedimine tetra acetic acid on its adsorption capacity for the adsorption of several metals ions. J Hazard Mater. 150:408–412. doi:https://doi.org/10.1016/j.jhazmat.2007.04.123
- Ahalya N, Kanamadi RD, Ramachandra TV. 2005. Biosorption of chromium (vi) from aqueous solutions by the husks of Bengal gram (Cicerarientinum). Electron J Biotechnol. 8(3):258–264. doi:https://doi.org/10.2225/vol8-issue3-fulltext-10
- Alden P, Heath F, Leventer A, Keen R, Zomfler WB. 1998. National Audubon Society field guide to California. New York: Knopf Press.
- Anjum R, Farah F, Uzaira R, Saima N, Ashraf MA. 2015. Analysis of sorption efficiency of activated carbon for removal of anthracene and pyrene for wastewater treatment. Desalin Water Treat. doi:https://doi.org/10.1080/19443994.2015.1015304.
- Ashraf MA, Yusoff I, Yusof I, Alias Y. 2013a. Removal of Cd (II) onto Raphanus sativus peels biomass: Equlibrium, kinetics, and thermodynamics. Desalin Water Treat. 51:4402–4412. doi:https://doi.org/10.1080/19443994.2012.752333.
- Ashraf MA, Yusoff I, Yusof I, Alias Y. 2013b. Removal of acid yellow17 dye from aqueous solution using eco-friendly biosorbent. Desalin Water Treat. 51:4530–4545. doi:https://doi.org/10.1080/19443994.2012.747187.
- Ashraf MA, Maah MJ, Yusoff I. 2012. Removal of lead from synthetic solutions by protonted teleosts biomass. E J Chem. 9(1):345–353. doi:https://doi.org/10.1155/2012/769180
- Ashraf MA, Maah MJ, Yusoff I, Mahmood K, Wajid A. 2011. Study of biosorptive potential in the peel of Citris reticulatae, Punica grantum, Daucus carota and Momordica charantia. African J Biotechnol. 10(68):15364–15371. doi:https://doi.org/10.5897/AJB11.1090
- Ashraf MA, Maah MJ, Yusoff I. 2010. Study of mango biomass (Mangifera Indica L) as cationic bio-sorbent. Int J Environ Sci Technol. 7(3):581–590. doi:https://doi.org/10.1007/BF03326167
- Bell GP. 1997. Ecology and management of Arundo donax, and approaches to riparian habitat restoration in southern California. In Brock JH, Wade M, Pysêk P, Green D. editors. Plant invasions: Studies from North America and Europe. Leiden, the Netherlands: Backhuys; p. 103–113.
- Chia CL, Hwai-shen L. 2000. Adsorption in a centrifugal field: Basic dye adsorption by activated carbon. Ind Eng Chem Res. 39:161–167. doi:https://doi.org/10.1021/ie9902333
- Faryal M, Iqra J, Uzaira R, Noshabah T, Maliha S, Sher ZS, Ismail Y, Ashraf MA. 2015. New method for the adsorption of organic pollutants using natural zeolite incinerator ash (ZIA) and its application as an environmentally friendly and cost-effective adsorbent. Desalin and Water Treat. Online First doi:https://doi.org/10.1080/19443994.2015.1005146
- Gadd GM. 2009. Biosorption: critical review of scientific rationale, environmental importance and significance for pollution treatment, review. J ChemTechnol Biotechnol. 84:13–28. doi:https://doi.org/10.1002/jctb.1999
- Gadd GM. 1990. Heavy metal accumulation by bacteria and other micro organisms. Experientia. 46:834–840. doi:https://doi.org/10.1007/BF01935534
- Hanif MA, Nadeem R, Bhatti HN, Ahmad NR, Ansari TM. 2007. Ni(II) biosorption by Cassia fistula (Golden Shower) biomass. J Hazard Mater. 139(2):345–355. doi:https://doi.org/10.1016/j.jhazmat.2006.06.040
- Haq I, Bhatti HN, Asgher M. 2011. Removal of Solar red BA textile dye from aqueous solution by low cost barley husk: Equilibrium, kinetic and thermodynamic study. Can J Chem Eng. 89:593–600. doi:https://doi.org/10.1002/cjce.20436
- Horsefall MJ, Spiff AI. 2005. Effect of temperature on the sorption of Pb2+ and Cd2+. An inventory of heavy metals inputs to agricultural soils in England and Wales. Sci Total Environ. 311(1–3):205–219.
- Ilhan S, Nourbakhsh MN, Kilicarslan S, Ozdag H. 2004. Removal of chromium, lead and copper ions from industrial wastewaters by Staphylococcus saprophyticus. Turkish J Biotechnol. 2:50–57.
- Kok KH, Karim MIA, Ariff A. 2001. Bioremoval of cadmium, lead and zinc using non-living biomass of Aspergillus flavus. Pak J Biol Sci. 4(7):849–853. doi:https://doi.org/10.3923/pjbs.2001.849.853
- Kousar P, Uzaira R, Sher ZS, Ashraf MA. 2015. A novel method for synthesis of functionalized hybrids and their application for wastewater treatment. Desalin Water Treat. Online First doi:https://doi.org/10.1080/19443994.2015.1006819.
- Kratochvil D, Velosky B. 1998. Biosorption of Cu from ferruginous wastewater by algal biomass. Water Res. 32(9):2760–2768. doi:https://doi.org/10.1016/S0043-1354(98)00015-3
- Luis CR, Antonio B, Elio EG. 2009. Peanut shell activated carbon: Adsorption capacities for copper(II), zinc(II), nickel(II) and chromium(VI) ions from aqueous solutions. Adsorpt Sci Technol. 22(3):237–243.
- Motoyuki S. 1990. Adsorption Engineering. Elsevier Sci. Publishers. pp. 5–61.
- Mushtaq A, Shatirah A, Khaled SB, Samavia B, Nor KAB, Ashraf MA. 2014. Study of modern nanoenhanced techniques for removal of dyes and water. J Nanomater. 864914:1–20. https://doi.org/http://dx.doi.org/10.1155/2014/864914.
- Tahira Q, Najma M, Saima QM, Ashraf MA. 2015. Decontamination of ofloxacin: optimization of removal process onto sawdust using response surface methodology. Desalin Water Treat. Online First. doi: https://doi.org/10.1080/19443994.2015.1006825.
- Volesky B, Holan ZR. 1995. Biosorption of heavy metals. Biotechnol Prog. 11:235–250. doi:https://doi.org/10.1021/bp00033a001
- Volesky B. 1987. Biosorbents for metal recovery. Trends Biotechnol. 5(4):96–101. doi:https://doi.org/10.1016/0167-7799(87)90027-8
- Watanabe M, Kawahara K, Sasaki K, Noparatnaraporn N. 2003. Biosorption of cadmium ions using a photosynthetic bacterium, Rhodobacter sphaeroids S and a marine photosynthetic bacterium, Rhodovulum sp. and their biosorption kinetics. J Biosci Bioengg. 95(4):374–378. doi:https://doi.org/10.1016/S1389-1723(03)80070-1