Abstract
Nitric oxide (NO) plays an important role in the physiology and pathophysiology of disease. Overproduction of NO is associated with chronic inflammatory diseases and cancers. Several species of Solanaceae have been used traditionally to treat inflammatory-related diseases. To analyse the possible anti-inflammatory properties of these species, the Griess assay was used to evaluate the effects of various Solanum melongena and Solanum macrocarpon extracts on NO production in lipopolysaccharide (LPS)-stimulated RAW 264.7 cells. The cytotoxicity of the extracts on the cell line was tested using the 3-(4,5-dimethylthiazol-2-yl)-2,5-diphenyltetrazolium bromide (MTT) assay. Extracts that significantly inhibited NO production were further evaluated for inducible nitric oxide synthase (iNOS) expression by Western blot. Thin-layer chromatography was used to determine the major compounds in the extracts. All extracts significantly inhibited NO production in LPS-stimulated RAW 264.7 cells in a dose-dependent manner. At 200 µg/ml, ethyl acetate extract of S. macrocarpon showed the highest NO inhibition of 81%, with a median inhibitory concentration (IC50) value of 44.78 ± 0.04 µg/ml. The viability of cells treated with the extracts was greater than 80%. Ethanol and ethyl acetate extracts of S. melongena, together with ethanol, hexane and ethyl acetate extracts of S. macrocarpon, reduced iNOS expression significantly. At 200 µg/ml, ethyl acetate extract of S. macrocarpon inhibited iNOS protein expression by 79%. Phytochemical analysis of the extracts showed that fluorescent, double-bond compounds, phenols, flavonoids and terpenoids were mainly present in the extracts. Taken together, the results show the potential of ethanol and ethyl acetate extracts of S. melongena, and hexane and ethyl acetate extracts of S. macrocarpon, as agents for the prevention and treatment of inflammatory-related diseases.
Introduction
Nitric oxide (NO) is a signalling molecule that plays an important role in the physiology and pathophysiology of disease. NO is synthesized from L-arginine by nitric oxide synthase (NOS). Three forms of NOS have been identified. Endothelial nitric oxide synthase (eNOS) and neuronal nitric oxide synthase (nNOS) continuously produce small amounts of NO and are involved in physiological regulation. Inducible nitric oxide synthase (iNOS), in contrast, produces large amounts of NO when induced (Liew et al. Citation2011). NO produced by iNOS has antiviral and antimicrobial effects but overproduction of NO from iNOS is implicated in a wide range of chronic inflammatory diseases (Kleinert et al. Citation2010). In addition, up-regulation of NO production has been observed in many types of cancer. Therefore, the regulation of NO production and iNOS expression could provide possible targets for the treatment of inflammatory-related diseases. Non-steroidal anti-inflammatory drugs (NSAIDs), for example, aspirin, ibuprofen and indomethacin, are used to treat inflammation. However, the use of these synthetic drugs to treat inflammation can cause severe gastrointestinal and cardiovascular complications (Mehta & Bhargava Citation2010; Sostres et al. Citation2010). Thus, there is still a need for the discovery of new anti-inflammatory drugs with fewer or no side-effects.
Plants, including those of the Solanaceae family, have long been used in traditional medicine to treat inflammatory-related diseases, with few side-effects (Fawole et al. Citation2010; Siriwatanametanon et al. Citation2010; Zhang et al. Citation2011; Eddouks et al. Citation2012). Solanum melongena is commonly known as eggplant or aubergine and is a popular vegetable in Asia (Concellón et al. Citation2012; Kaur et al. Citation2014). It is used traditionally to treat asthma and allergic rhinitis (Bello et al. Citation2005; Sharma et al. Citation2011). Studies have shown that phenolic compounds from S. melongena have antioxidant properties (Sadilova et al. Citation2006; Singh et al. Citation2009) and high superoxide scavenging activity (Kaur et al. Citation2014). Anthocyanin from S. melongena fruit peel can also scavenge free radicals that could cause damage to proteins and DNA (Noda et al. Citation2000). Hepatoprotective activity (Akanitapichat et al. Citation2010) and inhibition of the inflammation that can cause atherosclerosis (Han et al. Citation2003) have also been reported.
Solanum macrocarpon is native to Africa and can be found in Southeast Asia (Sanchez-Mata et al. Citation2010). Traditionally, it is used to treat bronchitis, asthma, rheumatism, Parkinson's disease, gout and angina (Oboh et al. Citation2005). Similarly to S. melongena, high concentrations of phenolic compounds have been detected in S. macrocarpon, which correlate with the antioxidant activity (Prohens et al. Citation2007; Plazas et al. Citation2014). Alkaloids from S. macrocarpon have been shown to induce apoptosis in breast cancer cells (Sanchez-Mata et al. Citation2010). Since no scientific study has been carried out to confirm the traditional use of these plants to treat inflammatory-related diseases, this study was undertaken to examine the effects of S. melongena and S. macrocarpon extracts on lipopolysaccharide (LPS)-induced production of NO and iNOS expression in the murine macrophage cell line RAW 264.7.
Materials and methods
Plant materials and extraction
The fruits of S. melongena and S. macrocarpon were washed and dried in an oven at 45°C. For extraction, 50 g of ground fruit was added to 500 ml of 80% ethanol (Thermo Fisher Scientific, Hampton, NH, USA). Incubation was carried out in an environmental shaker at 80°C for 24 h. The extraction process was repeated twice. The extracts obtained were combined, filtered and concentrated using a rotary evaporator to yield an ethanol extract. Crude ethanol extract was successively partitioned with hexane (Thermo Fisher Scientific) and a mixture of ethyl acetate (Thermo Fisher Scientific) and water (1:1) to yield hexane, ethyl acetate and water extracts.
Cell culture
The murine monocyte/macrophage cell line RAW 264.7 (American Type Culture Collection, Manassas, VA, USA) was maintained in Dulbecco's modified Eagle's medium (DMEM) (Sigma Aldrich, St Louis, MO, USA) supplemented with 10% foetal bovine serum (GE Healthcare, Little Chalfont, UK) and 4.5 g/l L-glutamine (Sigma Aldrich). The cells were cultured at 37°C in a humidified atmosphere of 5% carbon dioxide (CO2).
Nitrite determination
RAW 264.7 cells (2 × 106 cells/ml) were seeded into a 96-well plate and incubated for 2 h at 37°C, 5% CO2 to allow cells to attach. Cells were induced with 10 µg/ml bacterial LPS from Escherichia coli strain 55:B5 (Sigma Aldrich) in the presence or absence (negative control) of the plant extracts. The extract concentration used in this study ranged from 3.13 µg/ml to 200 µg/ml. N-Nitro-L-arginine methyl ester hydrochloride (L-NAME, 250 µM) (Sigma Aldrich) was used as a positive control. The cells were incubated for 18 h. At the end of the incubation period, an equal amount of spent medium was mixed with Griess reagent [1% sulfanilamide (Thermo Fisher Scientific) and 0.1% naphthylethylenediamine (Thermo Fisher Scientific) in 2.5% phosphoric acid (Thermo Fisher Scientific)]. The absorbance of the reaction mixture was read at 540 nm. The amount of nitrite was extrapolated from the sodium nitrite (Sigma Aldrich) standard graph. The inhibition rate was calculated as described previously by Tham et al. (Citation2010).
Cell viability test
A cell viability test was carried out to test the cytotoxic effects of the various extracts on RAW 264.7 cells. The viability was measured using the 3-(4,5-dimethylthiazol-2-yl)-2,5-diphenyltetrazolium bromide (MTT) (Sigma Aldrich) assay, as described by Mosmann (Citation1983). After treating the cells with the extracts, the medium was replaced with 20 μl of MTT solution. The cells were incubated for another 4 h. The purple formazan formed was dissolved with 200 μl dimethyl sulfoxide (Sigma Aldrich) and the absorbance was read at 540 nm (Abas et al. Citation2006). The cell viability was calculated as follows: Cell viability (%) = [(OD Sample)/(OD Control)] × 100%, where OD is optical density.
Western blot analysis
Only plant extracts that significantly inhibited NO production were chosen for this assay. First, 2 × 106 cells/ml were seeded into a Petri dish and incubated for 24 h. Cells were then induced with 10 µg/ml LPS in the presence or absence of plant extracts for iNOS protein detection. Cells treated with 10 µg/ml LPS were used as a negative control, while cells treated with 10 µM dexamethasone (Sigma Aldrich) were used as the positive control. After treatment with the selected extracts for 24 h, the cells were washed with ice-cold phosphate-buffered saline (PBS) and lysed with lysis buffer for 15 min on ice. The cells were centrifuged at 14,000 g for 10 min at 4°C. The protein concentration in the cell lysate was determined using the Pierce Bicinchoninic Acid (BCA) Protein Assay kit (Thermo Fisher Scientific) as described by the manufacturer. Sodium dodecyl sulfate–polyacrylamide gel electrophoresis (SDS-PAGE) and Western blot were performed as described by Tham et al. (Citation2010). To detect iNOS and β-actin, the membranes (Pall Corporation, Port Washington, NY, USA) were incubated separately in anti-iNOS rabbit polyclonal primary antibody (1:1000 dilution) (Cayman Chemical Company, Ann Arbor, MI, USA) and anti-β-actin polyclonal antibody (1:1000 dilution) (BioVision Incorporated, Milpitas, CA, USA) overnight. The membranes were washed three times with Tris-buffered saline–1% Tween, followed by incubation with horseradish peroxidase-conjugated donkey anti-rabbit secondary antibody (1:5000 dilution) (Abnova Corporation, Walnut, CA, USA) for 1 h. After the final wash, the bands were developed using 3′3′-diaminobenzidine substrate. The membranes were scanned and the density of the bands was analysed using Image J software (National Institutes of Health, Bethesda, MD, USA).
Phytochemical screening
Thin-layer chromatography was carried out to determine the major compounds present in the plant extracts. Rutin (Sigma Aldrich), vanillic acid (Sigma Aldrich) and catechin (Sigma Aldrich) were used as standards. Chromatograms were inspected under daylight to determine the presence of coloured substances and under short ultraviolet (UV) and long UV light for the presence of fluorescent compounds. Anisaldehyde (Sigma Aldrich), Dragendorff (Sigma), Folin Ciocalteu (Sigma Aldrich), iodine (Sigma Aldrich) and vanillin (Sigma Aldrich) reagents were used to detect terpenoids, alkaloids, phenols, double-bond compounds and flavonoids, respectively (Houghton & Raman Citation1998).
Statistical analysis
All experiments were repeated three times, with each experiment carried out in triplicate. Data are reported as mean ± SD. Statistical analysis was performed by analysis of variance (ANOVA) followed by Dunnet's test. Values with p < 0.05 were considered statistically significant.
Results and discussion
Nitric oxide inhibition and cell viability test
Ethanol, hexane, ethyl acetate and water extracts of S. melongena and S. macrocarpon were evaluated for their NO inhibition activity against LPS-stimulated RAW 264.7 cells using the Griess assay. The cells were incubated with or without LPS in the presence or absence of the test extracts, ranging from 3.13 µg/ml to 200 µg/ml. As expected, RAW 264.7 cells induced with LPS produced large amounts of NO compared with untreated cells, whereas cells treated with L-NAME produced significantly lower levels of NO.
All the tested extracts significantly inhibited NO production in LPS-stimulated RAW 264.7 cells in a dose-dependent manner. Ethyl acetate extract of S. melongena showed the highest percentage of inhibition, with 78% at 200 µg/ml, followed by ethanol extract, 70.16% (c, a). Solanum macrocarpon ethyl acetate extract significantly inhibited NO production, starting at the concentration of 6.25 µg/ml with an inhibition rate of 12% (c). At 200 µg/ml, the inhibition rate was 81%, which was better that that of the control, L-NAME (c). This was followed by ethanol extract, with an inhibition rate of 63.89% (a). The IC50 (concentration needed for 50% of inhibition of NO production) of the plants in increasing order was as follows: ethyl acetate extract of S. macrocarpon, 44.78 ± 0.04 µg/ml < hexane extract of S. macrocarpon, 54.45 ± 0.08 µg/ml < ethanol extract of S. melongena, 59.74 ± 0.14 µg/ml < hexane extract of S. melongena, 59.91 ± 0.08 µg/ml ().
Figure 1. Effect of Solanum melongena extracts on nitric oxide (NO) production: (a) ethanol extract; (b) hexane extract; (c) ethyl acetate extract; (d) water extract. RAW 264.7 cells were stimulated with 10 µg/ml lipopolysaccharide (LPS) alone or in the presence of the indicated concentrations of extract, and the amount of nitrite was evaluated after 24 h. Results are presented as mean ± SD from three independent experiments with three replicates each. *Significantly different value from negative control at 95% confidence level; **significantly different from negative control at 99% confidence level. L-NAME = N-Nitro-L-arginine methyl ester hydrochloride (positive control).
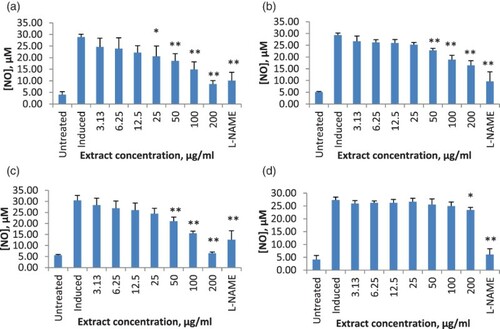
Figure 2. Effects of Solanum macrocarpon extracts on nitric oxide (NO) production: (a) ethanol extract; (b) hexane extract; (c) ethyl acetate extract; (d) water extract. RAW 264.7 cells were stimulated with 10 µg/ml lipopolysaccharide (LPS) alone or in the presence of indicated concentrations of extract and the amount of nitrite was evaluated after 24 h. Results are presented as mean ± SD from three independent experiments with three replicates each. *Significantly different value from negative control at 95% confidence level; **significantly different from negative control at 99% confidence level. L-NAME = N-Nitro-L-arginine methyl ester hydrochloride (positive control).
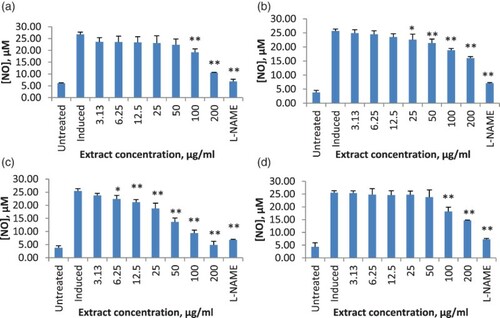
Table 1. Median inhibitory concentration (IC50) (inhibition of nitric oxide production) of various extracts of Solanum melongena and Solanum macrocarpon.
To validate that the decrease in NO production was not due to the death of the cells exposed to the extracts, a cell viability test was carried out. The cytotoxic effect of extracts on RAW 264.7 cells was tested using the MTT assay at concentrations ranging from 3.13 µg/ml to 200 µg/ml. Within this range, none of the extracts showed any cytotoxic effects towards RAW 264.7 cells. At 200 μg/ml, the viability of the cells was more than 80% (). This means that the low NO production in the treated cell was not caused by cell death but was most probably due to NO inhibition by the extracts tested (Rao et al. Citation2007).
Table 2. Viability of RAW 264.7 cells treated with Solanum melongena and Solanum macrocarpon extracts at 200 µg/ml.
Western blot analysis
Western blot analysis was carried out to examine the effects of the S. melongena and S. macrocarpon extracts on iNOS expression. For each extract, only concentrations that had significantly reduced NO production previously were tested. iNOS expression increased significantly following induction by 10 µg/ml LPS, while treating the induced cells with dexamethasone reduced the iNOS expression significantly.
Although all extracts were able to inhibit NO production significantly and dose-dependently in LPS-stimulated RAW 264.7 cells, not all extracts reduced iNOS expression in LPS-stimulated RAW 264.7 cells. Extracts able to reduce iNOS protein expression significantly were ethanol and ethyl acetate extracts of S. melongena (a, c), and ethanol, hexane and ethyl acetate extracts of S. macrocarpon (a, b and c). The ethyl acetate extract of S. macrocarpon was able to reduce iNOS expression significantly when tested at 50 µg/ml, 100 µg/ml and 200 µg/ml of the extract. At 200 µg/ml, densitometric analysis showed that iNOS expression was inhibited by 79%, which was slightly better than that of the positive control drug, dexamethasone, 71% (c). Ethanol and hexane extracts of S. macrocarpon inhibited iNOS expression significantly only when treated at 200 µg/ml, with inhibition rates of 36% and 29%, respectively (a, b).
Figure 3. Immunoblot analysis of the effects of various extracts of Solanum melongena extracts on inducible nitric oxide synthase (iNOS) expression in lipopolysaccharide (LPS)-stimulated RAW 264.7 cells: (a) ethanol extract; (b) hexane extract; (c) ethyl acetate extract; (d) water extract. Results are mean ± SD of three independent experiments with three replicates each. *p < 0.05 and **p < 0.01 indicate significant differences from LPS-induced cells. Dexa = dexamethasone (positive control).
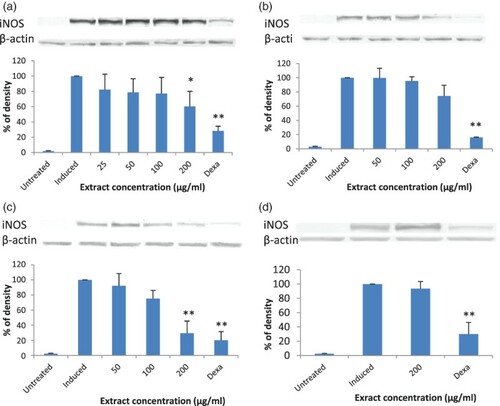
Figure 4. Immunoblot analysis of the effects of various extracts of Solanum macrocarpon extracts on inducible nitric oxide synthase (iNOS) expression in lipopolysaccharide (LPS)stimulated RAW 264.7 cells: (a) ethanol extract; (b) hexane extract; (c) ethyl acetate extract; (d) water extract. Results are mean ± SD of three independent experiments with three replicates each. *p < 0.05 and **p < 0.01 indicate significant differences from LPS-induced cells. Dexa = dexamethasone (positive control).
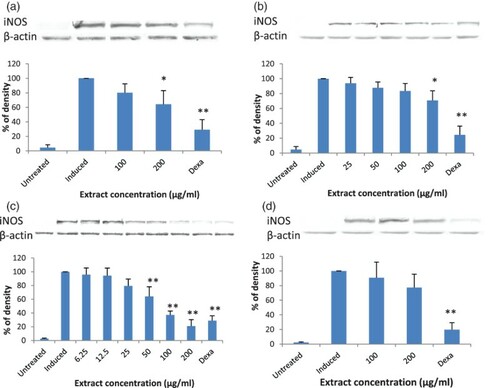
The results indicated that the reduction of iNOS protein expression by these extracts at least in part contributed to the inhibition of NO production in LPS-stimulated RAW 264.7 cells. However, the reduction rate of iNOS protein expression was not correlated with the inhibition of NO production. Since the hexane extract of S. melongena (b) and water extracts of both S. melongena and S. macrocarpon (Figures d and 4d) did not significantly reduce iNOS protein expression after stimulation with LPS, even though they were able to inhibit NO production significantly, it is possible that these extracts interfere with iNOS activity, rather than its expression. This finding is similar to Rao et al. (Citation2007), who reported that chloroform extract from Antrodia camphorate inhibited NO significantly but did not affect iNOS protein expression significantly.
Secondary metabolites and bioactivity
The plant extracts were subjected to thin-layer chromatography to screen for the presence of phytochemicals. Ethanol, hexane and ethyl acetate extracts of S. melongena and S. macrocarpon contained flavonoids and terpenoids. Only the ethanol extracts of both plants were found to contain phenols. Alkaloids were not found in any of the extracts used in this study ().
Table 3. Major compounds detected in Solanum melongena and Solanum macrocarpon extracts by thin-layer chromatography.
Efforts to inhibit NO production have been carried out by other researchers. Solanoflavone, a biflavonol glycoside extracted from the aerial part of white eggplant, was found to have anti-inflammatory activities (Tozer & Everett Citation1997). Plant flavonoids such as wogonin and luteolin from Scutellaria radix were also able to inhibit NO production (Chi et al. Citation2001). This finding is supported by Kim et al. (Citation1999), who showed that flavonoid derivatives, such as apigenin, luteolin and wogonin, which contain a double bond in the C ring and a dihydroxyl group in the A ring strongly inhibit NO production from LPS-stimulated cells. In contrast, flavanone derivatives which do not have a double bond in the C ring do not inhibit NO production from LPS-stimulated cells. These results indicate that the presence of a planar ring system in the flavonoid is crucial in NO inhibition activity (Kim et al. Citation1999). Terpenoids also have NO inhibition properties. For example, genipin, carnosol, ginkgolides and β-carotene could inhibit NO production in LPS-stimulated RAW 264.7 cells by reducing iNOS protein expression (Bredt & Snyder Citation1994).
Since flavonoids are detected through the thin-layer chromatography test, it is also possible that the extracts tested exhibit anti-inflammatory activity through their NO scavenging ability rather than affecting their iNOS protein expression. This may partly explain the Western blot results (Figures b, d and d), which showed that the hexane extract of S. melongena and water extract of both plants had no significant effect on iNOS protein expression. This result is supported by García-Lafuente et al. (Citation2009), whose review showed that flavonoids can exert their anti-inflammatory activities through several mechanisms, including antioxidative and radical scavenging activities.
In this study, phenol compounds were detected in ethanol extracts of both S. melongena and S. macrocarpon (). Both extracts inhibited NO production in LPS-stimulated RAW 264.7 cells significantly, with inhibition rates of 70.16% and 63.89%, respectively, at 200 µg/ml (Figures a and a). Studies have shown that Solanum species have a high content of phenolic acids (Ma et al. Citation2010; Plazas et al. Citation2014). Plazas et al. (Citation2014) showed that S. macrocarpon and S. aethiopicum contain high amounts of phenolic acid, specifically chlorogenic acid, where NO inhibition activity by the extracts correlates with chlorogenic acid content. In their study, methanol was used to extract chlorogenic acid. Similarly to the current study, phenolic compounds were only detected in ethanol extracts of both S. melongena and S. macrocarpon. The presence of phenolic compounds in the ethanol extracts of both plants may partly explain the NO inhibition activity of the extracts, by reducing the iNOS activity.
Conclusion
The results of this study demonstrated that ethanol and ethyl acetate extracts of S. melongena, and hexane and ethyl acetate extracts of S. macrocarpon, can inhibit NO production and iNOS protein expression when tested using RAW 264.7 cells, and therefore have potential as therapeutic agents for inflammatory-related diseases. Further studies are needed to investigate the mechanisms of action.
Disclosure statement
No potential conflict of interest was reported by the authors.
References
- Abas F , Lajis NH , Israf DA , Khozirah S , Umi Kalsom Y . 2006. Antioxidant and nitric oxide inhibition activities of selected Malay traditional vegetables. Food Chem. 95:566–573. Available from: http://www.sciencedirect.com/science/article/pii/S0308814605001226 doi: https://doi.org/10.1016/j.foodchem.2005.01.034
- Akanitapichat P , Phraibung K , Nuchklang K , Prompitakkul S . 2010. Antioxidant and hepatoprotective activities of five eggplant varieties. Food Chem Toxicol. 48:3017–3021. Available from: http://www.sciencedirect.com/science/article/pii/S027869151000503X doi: https://doi.org/10.1016/j.fct.2010.07.045
- Bello SO , Muhammad BY , Gammaniel KS , Abdu-Aguye I , Ahmed H , Njoku CH , Pindiga UH , Salka AM . 2005. Preliminary evaluation of the toxicity and some pharmacological properties of the aqueous crude extract of Solanum melongena . Res J Agric Biol Sci. 1:1–9. Available from: http://www.aensiweb.com/old/rjabs/rjabs/1-9.pdf
- Bredt DS , Snyder SH . 1994. Nitric oxide: a physiologic messenger molecule. Annu Rev Biochem. 63:175–195. Available from: http://www.annualreviews.org/doi/abs/https://doi.org/10.1146/annurev.bi.63.070194.001135
- Chi YS , Cheon BS , Kim HP . 2001. Effect of wogonin, a plant flavone from Scutellaria radix, on the suppression of cyclooxygenase-2 and the induction of inducible nitric oxide synthase in lipopolysaccharide-treated RAW 264.7 cells. Biochem Pharmacol. 61(10):1195–1203. Available from: http://www.sciencedirect.com/science/article/pii/S0006295201005974 doi: https://doi.org/10.1016/S0006-2952(01)00597-4
- Concellón A , Zaro MJ , Chaves AR , Vicente AR . 2012. Changes in quality and phenolic antioxidants in dark purple American eggplant (Solanum melongena L. cv. Lucía) as affected by storage at 0°C and 10°C. Postharvest Biol Technol. 66:35–41. Available from: http://ezproxy.um.edu.my:2095/science/article/pii/S0925521411002900 doi: https://doi.org/10.1016/j.postharvbio.2011.12.003
- Eddouks M , Chattopadhyay D , De Feo V , Cho WC . 2012. Medicinal plants in the prevention and treatment of chronic diseases. Evid-Based Comp Alt. 2012:1–2. Available from: https://doi.org/http://dx.doi.org/10.1155/2012/458274 doi:https://doi.org/10.1155/2012/458274
- Fawole OA , Amoo SO , Ndhlala AR , Light ME , Finnie JF , Van Staden J . 2010. Anti-inflammatory, anticholinesterase, antioxidant and phytochemical properties of medicinal plants used for pain-related ailments in South Africa. J Ethnopharmacol. 127:235–241. Available from: http://ac.els-cdn.com/S0378874109007223/1-s2.0-S0378874109007223-main.pdf?_tid=0d51f502-d241-11e4-a242--00000aab0f6c&acdnat=1427213937_2354debbf48701033637c387a12b0e3e doi: https://doi.org/10.1016/j.jep.2009.11.015
- García-Lafuente A , Guillamón E , Villares A , Rostagno M , Martínez J . 2009. Flavonoids as anti-inflammatory agents: implications in cancer and cardiovascular disease. Inflamm Res. 58:537–552. Available from: https://doi.org/http://dx.doi.org/10.1007/s00011-009-0037-3 doi: https://doi.org/10.1007/s00011-009-0037-3
- Han SW , Tae J , Kim JA , Kim DK , Seo GS , Yun KJ , Choi SC , Kim TH , Nah YH , Lee YM . 2003. The aqueous extract of Solanum melongena inhibits PAR2 agonist-induced inflammation. Clin Chim Acta. 328:39–44. Available from: http://ezproxy.um.edu.my:2095/science/article/pii/S0009898102003777 doi: https://doi.org/10.1016/S0009-8981(02)00377-7
- Houghton PJ , Raman A . 1998. Laboratory handbook for the fractionation of natural extracts. London : Chapman & Hall.
- Kaur C , Nagal S , Nishad J , Kumar R , Sarika S . 2014. Evaluating eggplant (Solanum melongena L) genotypes for bioactive properties: a chemometric approach. Food Res Int. 60:205–211. https://doi.org/http://dx.doi.org/10.1016/j.foodres.2013.09.049 doi: https://doi.org/10.1016/j.foodres.2013.09.049
- Kim HK , Cheon BS , Kim YH , Kim SY , Kim HP . 1999. Effects of naturally occurring flavonoids on nitric oxide production in the macrophage cell line RAW 264.7 and their structure–activity relationships. Biochem Pharmacol. 58:759–765. Available from: http://www.sciencedirect.com/science/article/pii/S0006295299001604 https://doi.org/http://dx.doi.org/10.1016/S0006-2952(99)00160-4 doi: https://doi.org/10.1016/S0006-2952(99)00160-4
- Kleinert H , Art J , Pautz A . 2010. Nitric oxide: biology and pathobiology. San Diego : Academic Press. Chapter 7, Regulation of the Expression of Inducible Nitric Oxide Synthase; p. 211–267.
- Liew CY , Lam KW , Kim MK , Harith HH , Tham CL , Cheah YK , Sulaiman MR , Lajis NH , Israf DA . 2011. Effects of 3-(2-Hydroxyphenyl)-1-(5-methyl-furan-2-y-l) propenone (HMP) upon signalling pathways of lipopoly saccharide-induced iNOS synthesis in RAW 264.7 cells. Int Immunopharmacol. 11:85–95. Available from: http://www.sciencedirect.com/science/article/pii/S1567576910003401 doi: https://doi.org/10.1016/j.intimp.2010.10.011
- Ma C , Whitaker BD , Kennelly EJ . 2010. New 5-O-Caffeoylquinic acid derivatives in fruit of the wild eggplant relative Solanum viarum . J Agric Food Chem. 58:11036–11042. Available from: https://doi.org/http://dx.doi.org/10.1021/jf102963f doi: https://doi.org/10.1021/jf102963f
- Mehta D , Bhargava DK . 2010. Non-steroidal anti inflammatory drugs and gastrointestinal toxicity. Apollo Medicine. 7:251–262. Available from: http://www.sciencedirect.com/science/article/pii/S0976001612600180 doi: https://doi.org/10.1016/S0976-0016(12)60018-0
- Mosmann T . 1983. Rapid colorimetric assay for cellular growth and survival: application to proliferation and cytotoxicity assays. J Immunol Methods. 65:55–63. Available from: http://www.sciencedirect.com/science/article/pii/0022175983903034 https://doi.org/http://dx.doi.org/10.1016/0022-1759(83)90303-4 doi: https://doi.org/10.1016/0022-1759(83)90303-4
- Noda Y , Kneyuki T , Igarashi K , Mori A , Packer L . 2000. Antioxidant activity of nasunin, an anthocyanin in eggplant peels. Toxicology. 148:119–123. Available from: http://ac.els-cdn.com/S0300483X0000202X/1-s2.0-S0300483X0000202X-main.pdf?_tid=a1587f84-d244-11e4-bd51-00000aacb35d&acdnat=1427215473_358d54160308557a82fc7469ef2f5881 doi: https://doi.org/10.1016/S0300-483X(00)00202-X
- Oboh G , Ekperigin MM , Kazeem MI . 2005. Nutritional and haemolytic properties of eggplants (Solanum macrocarpon) leaves. J Food Compos Anal. 18:153–160. Available from: http://www.sciencedirect.com/science/article/pii/S0889157504000134 doi: https://doi.org/10.1016/j.jfca.2003.12.013
- Plazas M , Prohens J , Cunat AN , Vilanova S , Gramazio P , Herraiz FJ , Andujar I . 2014. Reducing capacity, chlorogenic acid content and biological activity in a collection of scarlet (Solanum aethiopicum) and Gboma (S. macrocarpon) eggplants. Int J Mol Sci. 15:17221–17241. Available from: http://www.ncbi.nlm.nih.gov/pmc/articles/PMC4227158/ doi: https://doi.org/10.3390/ijms151017221
- Prohens J , Rodríguez-Burruezo A , Raigón MD , Nuez F . 2007. Total phenolic concentration and browning susceptibility in a collection of different varietal types and hybrids of eggplant: implications for breeding for higher nutritional quality and reduced browning. J Am Soc Hortic Sci. 132:638–646. Available from: http://journal.ashspublications.org/content/132/5/638.full.pdf+html
- Rao YK , Fang S-H , Tzeng Y-M . 2007. Evaluation of the anti-inflammatory and anti-proliferation tumoral cells activities of Antrodia camphorata, Cordyceps sinensis, and Cinnamomum osmophloeum bark extracts. J Ethnopharmacol. 114:78–85. Available from: http://www.sciencedirect.com/science/article/pii/S0378874107003728 https://doi.org/http://dx.doi.org/10.1016/j.jep.2007.07.028 doi: https://doi.org/10.1016/j.jep.2007.07.028
- Sadilova E , Stintzing FC , Carle R . 2006. Anthocyanins, colour and antioxidant properties of eggplant (Solanum melongena L.) and violet pepper (Capsicum annuum L.) peel extracts. Z Naturforsch C. 61:527–535. Available from: http://www.ncbi.nlm.nih.gov/pubmed/16989312 doi: https://doi.org/10.1515/znc-2006-7-810
- Sanchez-Mata MC , Yokoyama WE , Hong YJ , Prohens J . 2010. Alpha-solasonine and alpha-solamargine contents of gboma (Solanum macrocarpon L.) and scarlet (Solanum aethiopicum L.) eggplants. J Agric Food Chem. 58:5502–5508. doi: https://doi.org/10.1021/jf100709g
- Sharma K , Saikia R , Kotoky J , Kalita J , Devi R . 2011. Antifungal activity of Solanum melongena L, Lawsonia inermis L. and Justicia gendarussa B. against dermatophytes. Int J Pharm Tech Res. 3:1635–1640. Available from: http://www.sphinxsai.com/Vol.3No.3/pharm/pdf/PT=64(1635-1640)JS11.pdf
- Singh AP , Luthria D Wilson T , Vorsa N , Singh V , Banuelos GS , Pasakdee S . 2009. Polyphenols content and antioxidant capacity of eggplant pulp. Food Chem. 114:955–961. doi: https://doi.org/10.1016/j.foodchem.2008.10.048
- Siriwatanametanon N , Fiebich BL , Efferth T , Prieto JM , Heinrich M . 2010. Traditionally used Thai medicinal plants: In vitro anti-inflammatory, anticancer and antioxidant activities. J Ethnopharmacol. 130:196–207. Available from: http://www.sciencedirect.com/science/article/pii/S0378874110002680 https://doi.org/http://dx.doi.org/10.1016/j.jep.2010.04.036 doi: https://doi.org/10.1016/j.jep.2010.04.036
- Sostres C , Gargallo CJ , Arroyo MT , Lanas A . 2010. Adverse effects of non-steroidal anti-inflammatory drugs (NSAIDs, aspirin and coxibs) on upper gastrointestinal tract. Best Pract Res Clin Gastroenterol. 24:121–132. Available from: http://www.sciencedirect.com/science/article/pii/S1521691809001589 https://doi.org/http://dx.doi.org/10.1016/j.bpg.2009.11.005 doi: https://doi.org/10.1016/j.bpg.2009.11.005
- Tham CL , Liew CY , Lam KW , Mohamad A-S , Kim MK , Cheah YK , Zakaria Z-A , Sulaiman M-R , Lajis NH , Israf DA . 2010. A synthetic curcuminoid derivative inhibits nitric oxide and proinflammatory cytokine synthesis. Eur J Pharmacol. 628:247–254. Available from: http://www.sciencedirect.com/science/article/pii/S0014299909011054 doi: https://doi.org/10.1016/j.ejphar.2009.11.053
- Tozer GM , Everett SA . 1997. Nitric oxide in tumour biology and cancer therapy. Part 1: physiological aspects. Clin Oncol. 9:282–293. Available from: http://www.sciencedirect.com/science/article/pii/S0936655505800615 https://doi.org/http://dx.doi.org/10.1016/S0936-6555(05)80061-5 doi: https://doi.org/10.1016/S0936-6555(05)80061-5
- Zhang L , Ravipati AS , Koyyalamudi SR , Jeong SC , Reddy N , Smith PT , Bartlett J , Shanmugam K , Münch G , Wu MJ . 2011. Antioxidant and anti-inflammatory activities of selected medicinal plants containing phenolic and flavonoid compounds. J Agric Food Chem. 59:12361–12367. Available from: https://doi.org/http://dx.doi.org/10.1021/jf203146e doi:https://doi.org/10.1021/jf203146e