ABSTRACT
Iron is an essential micro-element for plant growth. In the calcareous soils of Lebanon, immobilization of iron can lead to chlorosis and reduce the yields of agricultural production. Iron fertilization is therefore crucial to ensure good plant growth. The objective of this work was to compare the efficiency of the iron chelate HBED-Fe to that of EDDHA-Fe, on tomato (Solanum lycopersicum L.) and cucumber crops (Cucumis sativus L.). Five modules were studied in two separate greenhouses: one control, one module with fertilization by EDDHA-Fe and three modules with different applications of HBED-Fe. Chlorophyll and Fe contents in leaves were analyzed at different stages of the plants' cycle. Cucumber crops treated with HBED-Fe showed 19.2% higher chlorophyll content than the ones treated with EDDHA-Fe. Tomato crops presented 11.2% higher chlorophyll content when treated with HBED-Fe. For cucumbers, HBED-Fe should be applied in three applications separated by 25 days. For tomatoes, HBED-Fe should be applied in two applications separated by a month. The use of the HBED9% iron chelate was proved to be more effective than that of EDDHA6% because more efficiency was achieved with 40% less application rate. Multiple applications were also more effective than single applications.
KEYWORDS:
Introduction
Iron is an essential micro-element for plant growth. It ranges at the upper limit of the micronutrient category with approximately 2 µmol/g plant dry weight and plays an important role as an activator of many biochemical and enzymatic processes (Hell & Stephan Citation2003). It has an influence on the content of chlorophyll in plants, is associated with protein synthesis and influences the uptake of Mn, Mo and Cu. The availability of Fe for plants varies depending on the nature of the soil and the physicochemical characteristics affecting the form in which the elements exist. In soils with basic pH and high levels of active limestone, the nutritional intake of iron is disrupted (Jana Citation2009; López-Rayo et al. Citation2015). The use of Fe fertilizers ensuring more available forms is, therefore, crucial to meet the nutritional needs of intensive agricultural productions.
In Lebanon, the majority of soils are formed on limestone bedrocks (Darwish & Zurayk Citation1997). Those present on slopes are calcareous and have high levels of total and active limestone (Wakim et al. Citation2010; El Chami & El Moujabber Citation2014). This leads to alkaline soils adsorbing micro-elements and making them unavailable to plants. In fact, in calcareous soils, high carbonate concentrations tend to accumulate nitrates. Hence, plant roots may be exposed to high nitrate and high bicarbonate concentrations, both involved in the induction of Fe chlorosis (Mengel Citation1994; Briat et al. Citation2015).
The aim of this work was to evaluate the efficiency of two different iron chelate products as chlorosis correctors in calcareous Lebanese soils.
The iron chelate EDDHA (4.8% ortho-ortho) is the most commonly used Fe fertilizer in Lebanon. It is known to be an efficient soil fertilizer to treat iron chlorosis in plants growing in calcareous soil. In fact, EDDHA-Fe has stability characteristics, a range of tolerance at high pH (3.5–8.5) and a high solubility. HBED-Fe [N,N′-di-(2-hydroxybenzoyl)-ethylene- diamine-N,N′-diacetic acid] is a close relative of EDDHA-Fe with no chiral carbons in its structure; therefore, it does not present isomers of structure (López-Rayo et al. Citation2015). HBED has a Fe3+-chelate formation or stability constant of 39.68 compared to 33.91 for EDDHA-Fe. This characteristic makes it more stable in soils. It also has a high level of available iron (up to 9%) and is effective at higher pH levels.
The trials presented in this study were done on tomato and cucumber crops which are the most cultivated vegetables in Lebanon, and foliar chlorophyll and Fe content analyses allowed a comparison of the effectiveness of these two products.
Material and methods
Site description
The study took place in the experimental field of the Holy Spirit University-Kaslik (USEK) located at Hboub-Jbeil (34°07′33.6′′N; 35°40′31.1′′E) in Mount Lebanon. Two greenhouses were prepared and planted with cucumber and tomato crops (Greenhouse C for cucumber crops (Cucurbitaceae Cucumis sativus L. ‘Rami’) and Greenhouse T for tomato crops (Solanaceae Solanum lycoperaicum L. ‘Newton’)). Cucumbers were planted in a 640 m2 greenhouse divided into 23 lines with 90 plants each. Tomatoes were planted in a 480 m2 greenhouse divided into 24 lines with 30 plants each.
Applications
The two fertilizers used were HBED-Fe (8%) from Van Iperen and EDDHA-Fe (6%, 4.8 ortho-ortho) from Radicalene. EDDHA-Fe is the most effective and commonly used fertilizer in Lebanese; however, HBED-Fe is known to have higher purity (Nadal et al. Citation2009).
The amount of EDDHA-Fe iron chelate generally applied by Lebanese farmers is 1.5 kg/1000 m2. To test if the HBED-Fe product is effective at concentrations lower than the EDDHA-Fe, following the manufacturer’s guiding, 60% of the previous amount was used, which is equivalent to 0.9 kg/1000 m2 of HBED-Fe. The total amount needed for each greenhouse was then calculated and suitable amounts for each application were weighed and stored away from light and moisture. Fertigation of the required amount was then manually applied to each plant.
In the greenhouses C and T, five modules were studied:
Control module without any application of fertilizers;
EDDHA-Fe fertilizer applied three times during the crop cycle;
HBED-Fe fertilizer applied three times during the crop cycle;
HBED-Fe fertilizer applied two times during the crop cycle;
HBED-Fe fertilizer applied one time during the crop cycle.
shows the distribution of applications and the number of rows in each.
Table 1. Application distribution.
Sampling
Each greenhouse was divided into five sections each representing a processing module from which the samples were taken. Because Fe is not mobile in the plant, its deficiency first appears on new leaves; therefore, only new fully developed leaves were used as samples (Jana Citation2009).
In the cucumber greenhouse, each row was divided into 9 sections of 10 plants. Three sampling campaigns were done (). At each sampling campaign, one leaf sample was randomly picked from each section. In total, 45 samples were analyzed for modules C1, C2, C3 and C4 and 27 samples for C5.
Table 2. Sampling campaigns.
For tomatoes, the same procedure was used. Each row was divided into nine sections of three plants each. For tomato crops, we realized two sampling campaigns for foliar analyses (). In total, 45 samples were analyzed for modules T1, T2, T3 and T4 and 36 samples for T5.
In our study, we were following Lebanese traditional agricultural practices in the greenhouses. The farmers in Lebanon remove the apical part of the tomato plant at the end of the crop cycle in order to reduce its vegetative growth. This procedure limits the appearance of new leaves with iron deficiency. This is why we could not perform our third sampling campaign in the tomato greenhouse.
After sampling, leaves were stored at a temperature of −80°C until they were treated and analyzed.
Soil analyses
Five soil samples were collected from each greenhouse prior to the experiment at a depth of 30 cm (SC = soil sample from the cucumber greenhouse; ST = soil sample from the tomato greenhouse) (Carter Citation1993). Each sample was dried at room temperature, sieved at 2 mm and quartered before being analyzed at USEK, Lebanon.
The characteristics studied were as follows:
pHwater and pHKCl
Cation Exchange Capacity - CEC (Metson's Method)
Total and active limestone (NF ISO 10693)
Granulometry (% clay, silt and sand)
Total Fe content by atomic absorption spectroscopy (AAS) after sample total acidic digestion (HNO3 and H2O2)
Organic matter content (%)
Foliar chlorophyll analysis
Chlorophyll concentration (Stein & De Santis Braga Citation2014) was used as an indicator of the efficiency of the two fertilizers. In fact, the primary response of a higher plant exposed to iron deficiency is the loss of chlorophyll, leading to poorer photosynthetic ability (Pushnik et al. Citation1984). The presence of a linear relationship between the iron content and the chlorophyll levels produced by the leaf (Yoshida Citation1981; Sharma et al. Citation2014) allowed us to use chlorophyll measurements as a comparative parameter to study the difference between the modules.
Two methods were tested for chlorophyll extraction from leaves: acetone extraction (Porra Citation2005) and dimethyl sulfoxide (DMSO) extraction (Stein & De Santis Braga Citation2014). The DMSO method statistically showed more extraction and detection of chlorophyll and was, therefore, chosen for the study.
Hundred milligram of each leaf sample was weighed on an analytical balance and mixed with 7 mL of DMSO in volumetric tubes. These tubes were then incubated at 65°C. Incubation periods depended on the thickness of the leaf. After 24 h, the volume was adjusted to 10 mL and the absorbance of the solution was read using a spectrophotometer JENWAY 6100 (wavelengths: 645 and 663 nm).
Then, the equations established by Arnon (Citation1949) were used to determine chlorophyll concentrations:
(1)
(2)
(3) Equation (3) was used to determine the total concentration of chlorophyll in the leaves.
The results were studied using a one-way ANOVA test on SPSS.
Fe content in leaves
Total dissolution of leaf samples was carried out as follows: a mass of 0.5 g of the sample was placed in 7 mL of HNO3 (65%) and 1 mL of H2O2 (30%) in a microwave. Fe content was determined using AAS.
During the dissolution procedure, blanks and a standard (Estuarine sediment, Certified Reference Material BCR-667) received the same treatment as the samples to evaluate potential external contamination process and valuable efficiency of the chemical procedure. Iron recovery rates of the used standard were satisfactory: 98.7%. Thus, the total dissolution can be considered reliable.
Results and discussion
Soil analyses
Soil factors such as low soil organic matter, high clay content and waterlogged soils can contribute to the low availability of Fe to plants (López-Rayo et al. Citation2015).
Soil analyses’ results () showed slightly alkaline soils with an average conductivity of 0.5 mS/cm (Dahnke Citation1993; Peverill et al. Citation1999).
Table 3. Results of soil analyses.
The soils of Greenhouse C are clay soils, whereas the soils of Greenhouse T are sandy-clay soils. Both soils are sensitive to compaction and present a risk of asphyxiation.
The active limestone content is also low. This is consistent with the pH values. In fact, an excess of limestone in the soil would result in higher pH.
Organic matter content ranges between 1.8% and 3%. This is in agreement with the greenhouses’ means provided by the Lebanese Ministry of Environment (SOER Citation2001).
Total Fe content, determined after total dissolution, is mentioned in and is adequate for crop cultivation. The value corresponds to the new median of soil total concentrations determined by continental-scale geochemical surveys in Europe (De Caritat & Reimann Citation2012). However, in semi-arid regions, although most soils contain adequate total iron, the amount available to the plant is dependent on factors such as iron species and plant genotype (Miller et al. Citation1984).
In the studied soils, we found indexes of chlorosis potential (Equation (4)) between 3.0 and 4.5.
(4)
These indexes characterize soils poorly sensitive to chlorosis, which explains the absence of Fe visual deficiency symptoms in the control modules throughout the study (Dahnke Citation1993; Peverill et al. Citation1999).
Foliar chlorophyll analyses
The average chlorophyll concentration in cucumber leaves in the different modules is shown in . The highest values are found in module C3 where the total dose of HBED-Fe applied was distributed over three applications throughout the cucumber crop cycle.
Figure 1. Average chlorophyll concentration in (a) cucumber leaves and (b) tomato leaves. C is for cucumber modules and T is for tomato modules. 1, 2, 3, 4 and 5 are the five different modules studied. 1, control module without any application; 2, EDDHA-Fe fertilizer applied three times during the crop cycle; 3, HBED-Fe fertilizer applied three times during the crop cycle; 4, HBED-Fe fertilizer applied two times during the crop cycle; 5, HBED-Fe fertilizer applied one time during the crop cycle.*Means with the same letter are not significantly different (p < .05).
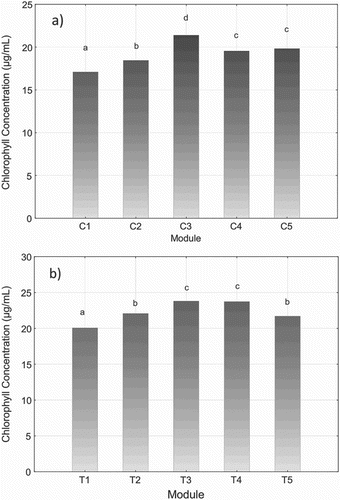
The results in give more details about chlorophyll contents in every module in each sampling campaign. They attest that, at the first sampling, the chlorophyll rate of module C3 is 19.2% higher than that of C2. The fast release of iron by the EDDHA molecule, with lower concentration of total Fe, explains the lower chlorophyll concentration for C2. Also, C2 did not show any significant difference compared to C1, the control module. In fact, the iron released is easily leached away from the root zone or adsorbed onto a soil component with negative charges such as oxides, organic matter or clay minerals. This makes iron less available to plants. The C3 module was applied 10 days before C2, using 40% less amount of chelate than C2, which indicates that HBED-Fe can be applied at lower concentrations and the stability of the product in the soil can be used to reduce the application frequency as mentioned also by Nadal et al. (Citation2012). Previous studies also mentioned that HBED-Fe was stable and that it has low reactivity with soils and soil components (López-Rayo et al. Citation2009).
Table 4. Results of leaf analyses for cucumber crops.
Similarly, C4 and C5 modules have, respectively, higher chlorophyll concentrations of 18.4% and 21.2% than that of the C2 module at the first analysis time.
During the third sampling campaign, only the C3 module had a significant difference compared to other modules with a concentration 18% higher than that of C2. This difference confirms that the chelate HBED has required more time to release the iron in the soil to be assimilated by the plant. The slow release of iron by the HBED molecule makes it more available to the plants, whereas its quick release by the EDDHA molecule does not allow its good assimilation by the plant (Ma et al. Citation1994; López-Rayo et al. Citation2009; Schenkeveld et al. Citation2014).
In the tomato greenhouse () and according to , T3 and T4 modules had higher chlorophyll concentrations than other modules. Since the chlorophyll concentration for both modules is the same and the dose of HBED used for the T3 module is 44% less than that of T4, we can consider the application of two 30 g doses as more efficient than the application of two 45 g of HBED.
Table 5. Results of leaf analyses for tomatoes.
At the first sampling campaign, no significant difference between T3 and T4 was noted even though the application dose was different. This can be due to the fact that the soil is not iron deficient, so the application of two different doses of HBED at the same date gave the same results.
However, at sampling time 2, the chlorophyll concentration of T3 is 18.5% higher than that of T4 and significantly higher than that of all the other modules.
The use of iron EDDHA chelate showed higher chlorophyll production than the control, 7.4% for the cultivation of cucumber and 8.9% for the cultivation of tomato, but its period of availability in soil was more limited than that of HBED-Fe.
Analysis of the T5 module and C5 explained the HBED action period. In fact, the C5 module, which consists of an application of a total dose of 1.52 g/L HBED-Fe 10 days before planting, revealed a concentration 23.3% higher than that of the control unit, the first sample collection taking place 45 days after the application. At time 2, sampling took place 75 days after the application and the concentration is 18.13% higher than the chlorophyll concentration in the control module. At time 3, after 96 days of application, the chlorophyll concentration between the control module and the C5 module showed no significant difference, indicating that the iron released was no longer available to the plants. The T5 module, which consists of an application of a total dose of 4.5 g/L HBED-Fe 10 days before planting, reveals a concentration 11.11% higher than the control, with the first sampling held 50 days after application. At time 2 analysis, sampling took place 98 days after application, and the chlorophyll concentration no longer showed any difference between T1 and T5. In the literature, the application time was extensively studied (Schenkeveld et al. Citation2012). In fact, the rapid biodegradation of chelate-based fertilizers is an important factor that can reduce their efficiency. It is, therefore, always a trade-off between the risk of leaching and the time span the fertilizer remains effective in the soil solution.
shows the availability of HBED-Fe in the soil for cultivations of cucumbers and tomatoes.
Fe contents in leaves
The results of Fe content in cucumber and tomato leaves were similar.
For cucumbers, the results of Fe analyses in leaves showed no significant difference between the different modules (ANOVA test, p < .05). The values averaged between 10.96 ± 0.277 and 13.00 ± 0.279mg/kg.
The Fe content, however, increased with time. The highest Fe content was obtained at sampling 3: 14 ± 0.318 mg/kg, whereas the lowest was obtained during the first sampling campaign: 6.736 ± 0.113 mg/kg. The ANOVA analysis showed a significant difference between Fe contents at sampling 1 and at samplings 2 and 3 (p < .05).
For tomatoes, the results of Fe analyses in leaves showed no significant difference between the different modules (ANOVA test, p < .05). The values averaged between 4.432 ± 0.136 and 9.184 ± 0.313 mg/kg.
The Fe content, however, significantly increased with time (ANOVA test, p < .05). The highest Fe content was obtained at sampling 2: 6.816 ± 0.318 mg/kg.
These results are in accordance with the literature attesting that Fe uptake depends on the growth stage of the plant and that the Fe absorbed by the plant does not necessarily end up in the leaves (Schenkeveld et al. Citation2012).
Recommended applications
We can conclude from our study that the best treatment for growing cucumber is to divide the amount of HBED-Fe into 3 applications separated by 25 days, the first application being during the vegetative growth phase of the plant. The amount of HBED-Fe required for each application for a 640 m2 greenhouse would be 175 g and the total amount required for the entire cycle will be 525 g.
The best HBED-Fe iron application plan for growing tomatoes is to divide the total Fe fertilizer amount into two applications separated by one month. According to our calculations, for a greenhouse having an area of 480 m2, each application must be a dose of 144 g; for both applications the total dose will be 288 g of iron chelate HBED-Fe.
A third application may still be effective for plants, but the third analysis was not performed in our study to confirm this theory.
Comparing the product’s purchase cost to the Lebanese farmer, the reduction in the amount of application from 1.5 kg for EDDHA to 0.9 kg for HBED will reduce the cost up to 12%.
Conclusion
Our study was designed to compare the efficiency of HBED iron chelate on tomato (Solanum lycopersicum L.) and cucumber crops (Cucumis sativus L.) in greenhouses. For this, several processing modules were set up to compare the performance of the plants in response to iron applications. This performance was measured by leaf analysis dosing of the chlorophyll contents.
The soil analysis results showed a high concentration of total iron, which may explain the lack of visual iron deficiency in the control module. In fact, soil chlorosis provoking factor gave values between 3 and 4.5, which is characteristic of low chlorosis soils.
The use of iron chelate HBED 9% was proved to be more effective than that of EDDHA 6% because the application rate can be reduced by 40% while achieving a more interesting efficiency. The HBED-Fe product can be used as a preventive tool for ferric chlorosis soils having iron deficiency. The EDDHA-Fe product can be used as a curative action in response to iron deficiency.
The best identified application plan for cucumber is to divide the amount of HBED-Fe into three applications separated by 25 days, the first application being during the vegetative growth of the plant. The amount of each iron application required for a 640 m2 greenhouse would be 172 g.
For tomato, the best iron treatment plan of HBED-Fe is to divide the amount required for the cultivation into two applications separated by a month. To a greenhouse having an area of 480 m2, each application must be a dose of 131 g.
For further confirmation on our results, future studies could be carried out on soils having higher iron deficiency and on crops less tolerant to this deficiency, while using a more precise experimental design for the study with various parameters such as the production, flowering and fruit set.
To better understand the availability of Fe and predict the efficiency of ferric fertilizers, sequential iron extraction manipulations can also be made to the soil before, during and after an application.
Acknowledgements
This work was undertaken as part of a cooperation program between Van Iperen International B.V represented by its Managing Director Mr Erik van den Bergh domiciled in the Netherlands and the USEK University in Lebanon.
Disclosure statement
No potential conflict of interest was reported by the authors.
References
- Arnon I. 1949. Copper enzymes in isolated chloroplasts in Beta vulgaris. Plant Physiology. 24:1–15. doi: 10.1104/pp.24.1.1
- Briat J-F, Dubos C, Gaymard F. 2015. Iron nutrition, biomass production, and plant product quality. Trends Plant Sci. 20:33–40. doi: 10.1016/j.tplants.2014.07.005
- Carter MR. 1993. Soil sampling and methods of analysis. CRC Press. Available from: https://books.google.fr/books?hl=fr&lr=&id=54lYLsV49zIC&oi=fnd&pg=PA1&dq=Carter,+M.+R.+(Ed.).+(1993).+Soil+sampling+and+methods+of+analysis.+CRC+Press.&ots=K2kH0JK0AQ&sig=WUJjWc8Vu0ruudBPvGsp2UqHDPM)
- Dahnke WC. 1993. Soil test interpretation. Commun Soil Sci Plant Anal. 24:11–27. doi: 10.1080/00103629309368778
- Darwish TM, Zurayk RA. 1997. Distribution and nature of red Mediterranean soils in Lebanon along an altitudinal sequence. CATENA. 28:191–202. doi:10.1016/S0341-8162(96)00037-9 doi: 10.1016/S0341-8162(96)00037-9
- De Caritat P, Reimann C. 2012. Comparing results from two continental geochemical surveys to world soil composition and deriving predicted empirical global soil (PEGS2) reference values. Earth Planet Sci Lett. 319:269–276. doi: 10.1016/j.epsl.2011.12.033
- El Chami D, El Moujabber M. 2014. Competitiveness of Lebanese wine: new shoots from ancient roots. J Wine Res. 25:298–311. doi: 10.1080/09571264.2014.959658
- Hell R, Stephan UW. 2003. Iron uptake, trafficking and homeostasis in plants. Planta. 216:541–551. doi:10.1007/s00425-002-0920-4
- Jana U. 2009. “Etude Des Interactions Entre La Plante Arabidopsis Thaliana (L.) Heynh et Le Ver de Terre Aporrectodea Caliginosa (Savigny): Application À La Phytoremédiation de L’arsenic et de L’antimoine.” Université Paris-Est.
- López-Rayo S, Hernández D, Lucena JJ. 2009. Chemical evaluation of HBED/Fe3+ and the novel HJB/Fe3+ chelates as fertilizers to alleviate iron chlorosis. J Agri Food Chem. 57:8504–8513. doi: 10.1021/jf9019147
- López-Rayo, S, Nadal P, Lucena JJ. 2015. Reactivity and effectiveness of traditional and novel ligands for multi-micronutrient fertilization in a calcareous soil. Front Plant Sci. 6. doi: 10.3389/fpls.2015.00752
- Ma R, Motekaitis RJ, Martell AE. 1994. Stability of metal ion complexes of N, N′-bis (2-hydroxybenzyl) ethylenediamine-N, N′-diacetic acid. Inorg Chim Acta. 224:151–155. doi: 10.1016/0020-1693(94)04012-5
- Mengel K. 1994. Iron availability in plant tissues-iron chlorosis on calcareous soils. Plant Soil. 165:275–283. doi:10.1007/BF00008070 doi: 10.1007/BF00008070
- Miller GW, Pushnik JC, Welkie GW. 1984. Iron chlorosis, a world wide problem, the relation of chlorophyll biosynthesis to iron. J Plant Nutr. 7:1–22. doi:10.1080/01904168409363172 doi: 10.1080/01904168409363172
- Nadal P, Hernández-Apaolaza L, Lucena JJ. 2009. Effectiveness of N,N′-bis(2-hydroxy-5-methylbenzyl) ethylenediamine-N,N′-diacetic acid (HJB) to supply iron to dicot plants. Plant Soil. 325:65–77. doi: 10.1007/s11104-009-0115-x
- Peverill, KI, Sparrow LA, Reuter DJ. 1999. Soil analysis: an interpretation manual. Collingwood: Csiro Publishing.
- Porra, RJ. 2005. “The chequered history of the development and use of simultaneous equations for the accurate determination of chlorophylls a and B.” In: Discoveries in photosynthesis. Springer; p. 633–640. http://link.springer.com/chapter/10.1007/1-4020-3324-9_56
- Pushnik JC, Miller GW, Manwaring JH. 1984. The role of iron in higher plant chlorophyll biosynthesis, maintenance and chloroplast biogenesis. J Plant Nutri. 7:733–758. doi:10.1080/01904168409363238 doi: 10.1080/01904168409363238
- Schenkeveld WDC, Hoffland E, Reichwein AM, Temminghoff EJM, van Riemsdijk WH. 2012. The biodegradability of EDDHA chelates under calcareous soil conditions. Geoderma. 173–174:282–288. doi:10.1016/j.geoderma.2011.12.007 doi: 10.1016/j.geoderma.2011.12.007
- Schenkeveld WDC, Reichwein AM, Temminghoff EJM, van Riemsdijk WH. 2014. Considerations on the shuttle mechanism of FeEDDHA chelates at the soil-root interface in case of Fe deficiency. Plant Soil. 379:373–387. doi:10.1007/s11104-014-2057-1
- Sharma A, Shankhdhar D, Shankhdhar SC. 2014. Growth promotion of the rice genotypes by PGPRS isolated from rice rhizosphere. J Soil Sci Plant Nutri. 14:505–517.
- “SOER 2001 – chpt11_S_L.pdf.” 2016. Available May 3, from: http://www.moe.gov.lb/ledo/soer2001pdf/chpt11_S_L.pdf
- Stein CE, De Santis Braga E. 2014. “CHLOROPHYLL A IN MACRO-VEGETABLE AS AN INDICATOR OF THE ENVIRONMENTAL IMPACTS IN THE CANANEIA-IGUAGE ESTUARINE-LAGOON SYSTEM–SP, BRAZIL.” In: Proceedings of safety, health and environment world Congress. Vol. 10. http://proceedings.copec.org.br/index.php/shewc/article/view/1721
- Wakim R, Bashour I, Nimah M, Sidahmed M, Toufeili I. 2010. Selenium levels in Lebanese environment. J Geochem Explor. 107:94–99. doi: 10.1016/j.gexplo.2010.09.008
- Yoshida S. 1981. Fundamentals of rice crop science. Los Baños: International Rice Research Institute. http://philpapers.org/rec/YOSFOR