ABSTRACT
In Indian traditional practice of medicines, numerous plants have been used against various neurodegenerative disorders like Alzheimer's disease (AD). Withania somnifera (Ashwagandha in Sanskrit) is one of the medicinal plants exhibiting broad spectrum activity against various diseases. Due to its ability to improve cognitive functions, aswagandha extracts are used in various memory supplements. In the present study, the pharmacological activity of various bioactive compounds of aswagandha has been studied with respect to AD. The blood brain barrier penetrability and central nervous system (CNS) activity of these bioactive compounds were predicted using in silico tools. The predicted pharmacological properties and structure activity relationship focuses that the compounds such as anaferine, anahygrine, cuscohygrine and isopelletierine can be CNS active and neuronal nicotinic acetylcholine receptor (nAChR) agonists. In order to investigate the structure activity relationship results, molecular modeling studies has been carried out with promising ligands against nAChR. It is a known fact that the agonists of nAChRs are capable to improve communication between neurons and hence these compounds may be useful as lead compounds for the development of drugs against neurodegenerative disorders. This study also indirectly focuses how ayurvedic formulations (those containing aswagandha) are effective against neurodegenerative disorders.
Introduction
Alzheimer’s disease (AD) is an age-related neurodegenerative disease, which is characterized by severe memory and cognitive impairment, behavioral and personality changes. Formation of amyloid-beta ()-peptide-containing plaques, neurofibrillary tangles and degeneration of cholinergic neurons on different parts of the brain are thought to play significant roles in the pathogenesis of AD (Selkoe Citation2001; Kar et al. Citation2004). Even though the etiology of disease is not well studied, a number of attempts have been done to limit the major pathologies of AD and there by curing it. Indian traditional medicines and ayurvedic formulations are very famous for a variety of life-threatening disease such as cancer, neurodegenerative disorder and bacterial infections like tuberculosis. Number of plant materials and their chemical constituents are already been found to have a direct action against various such disorders. For example, huperzine-A, berberine, curcumin and resveratrol are some of the well-studied chemical constituents isolated from different plant sources and are effectively studied against various disorders such as AD (Ringman et al. Citation2005; Tohda et al. Citation2005; Wu et al. Citation2010; Howes & Perry Citation2011; Shah et al. Citation2011). Many plant sources are now being considered as the repository for novel lead compounds against various disorders. Galantamine (isolated from the plant Galanthus woronowii) and rivastigmine (which is developed from physostigmine – a compound found in Physostigme Venensum) are two currently licensed drugs used for the treatment of AD (Heinrich and Teoh Citation2004; Kumar Citation2006).
Withania somnifera (known as ‘Ashwagandha’ in Sanskrit) is an important medicinal plant used in ayurveda, unani, and siddha for the last 3000 years (Asthana and Raina Citation1989; Singh and Kumar Citation1999). Ashwagandha is extensively used in memory-enhancing tonics in traditional ayurvedic medicines (Dhuley Citation2001). Ashwagandha extracts were reported to have antioxidant and free-radical-scavenging activities (Russo et al. Citation2001). They are also strong enough to increase the amount of acetylcholine and choline acetyltransferase activities in rats and effectively reverse the neuronal cell death by blocking the formation of amyloid peptide plaques (Bhattacharya et al. Citation1995; Kuboyama et al. Citation2005; Jayaprakasam et al. Citation2010; Kumar et al. Citation2012). Some of the existing medicinal properties of the ashwagandha relevant to AD are summarized in Table .
Table 1. Some of the medicinal properties reported for ashwagandha.
The pharmacological investigations of ashwagandha throw light into the presence of various classes of secondary metabolites such as steroidal lactones, phytosterols, sitoindosides and alkaloids (Elsakka et al. Citation1990; Atta-ur-Rahman et al. Citation1991, Citation1993; Choudary et al.,Citation1996; Rastogi & Mehrotra Citation1998; Kapoor Citation2001; Gupta & Rana Citation2007). It is believed that the cognitive and memory-enhancing properties of ashwagandha is because of the presence of various secondary metabolites and their positive action on cholinergic and glutamatergic receptors (Bhattacharya et al. Citation1997; Schliebs et al. Citation1997; Gupta et al. Citation2003; Kataria et al. Citation2012). Since the memory-related disorders are closely associated with the defects in cholinergic neurotransmission, their repair might be a promising treatment strategy for these kinds of disorders (Davies & Maloney Citation1976; Small & Fodero Citation2002). Defects in cholinergic neurotransmission can be fixed either by using acetylcholinesterase inhibitors or by acetylcholine receptors agonists (collectively called cholinergic ligands) (Bartus et al. Citation1982; Coyle et al. Citation1983; Krall et al. Citation1999; Wevers & Schröder Citation1999; Dani & Bertrand Citation2007; Fisher Citation2008). Even though several cholinergic ligands have been developed based on this concept, only a limited number of ligands was found useful in the treatment of AD. Hence the needs of identifying novel cholinergic ligands are highly demanding for the effective and safer treatment of AD (Rosini et al. Citation2005).
The -nicotinic acetylcholine receptor (
-nAChR) is a homo or heteropentameric protein belonging to the family of ligand-gated ion channel, which is widely distributed in the hippocampus and cortex regions of brain (Zarei et al. Citation1999). It is considered that
-nAChR is one of the essential part of the cholinergic nerve system. It was already proved that
-nAChR is associated with cholinergic anti-inflammatory pathway in termination of the parasympathetic nervous system (Miroslav Citation2012). The receptor is also known to be involved in the neuronal survival and synaptic plasticity (McKay et al. Citation2007). The studies suggested that the deficiency in the level of expression or in the function of the receptor may lead to the diseases such as AD, schizophrenia and epilepsy (Martin et al. Citation2004; Jensen et al. Citation2005; D’ Andrea & Nagele Citation2006).
The receptor mainly consists of three functional domains such as N terminal or ligand-binding domain (LBD), a transmembrane region and an intracellular region (Gotti & Clementi Citation2004). Among the different functional domains, the LBD received considerable attention due to their ability to trigger the biological responses of the receptor. Also the LBD is acting as a target for drugs that are used to paralyze the voluntary muscle (Paterson & Nordberg Citation2000; Sala et al. Citation2008).
Even though there are multiple nAChR, there is a special attention towards -nAChR for the drug discovery against neurological disorders. The main reason is that this receptor is consistently express during the AD progression (Davies & Feisullin Citation1981; Sugaya et al. Citation1990; Mahnir et al. Citation1998). Hence the target remains able to respond to the stimulation. Also it was proved that the
-nAChR channel is extremely permeable to the calcium ion (Kem Citation2000). When agonists are bound to the receptor, it stabilizes the ‘open conformation’ of receptor and allow to influx cations such as potassium, calcium and sodium (Itier & Bertrand Citation2001). Normally the calcium ion acts as secondary messenger inside the neuron to stimulate the signal transduction through the activation of protein kinase, calcineurins, nitric oxide synthetases, etc. (Kem Citation2000). Experimental techniques suggested that in order to stabilize the open conformation of nAChR, the agonists should be occupied at least two binding sites of the homo or heteropentamer (Lindstrom Citation2003). The studies proved that the agonists of
nAChR mediates neuroprotective action against various stresses (including
-amyloid and nerve growth factor depletion) and improve the communication between neurons (Meyer et al. Citation1998).
Normally agonist fits into the binding pocket wrought by loops called A, B and C, which is located in subunit and the adjacent subunit and depolarize the receptor. Hence it is suggested that the activation of
nAChRs may be a compensatory mechanism to maintain neuronal function and the compounds that can act as
-nAChR agonists would be potential therapeutic agents for AD.
Computational resources such as molecular docking, quantitative structure activity relationship (QSAR), structure-based ligand design are widely used in various steps of drug discovery pipeline. The effective uses of these computational resources are not only helps to fasten the drug discovery process but also helps to identify promising leads from a cluster of compounds. In the present study, a molecular-modeling-assisted investigation has been made to identify the possible agonistic/antagonistic activities of various reported secondary metabolites of Aswagandha against nAChR. The central nervous system (CNS) activity of reported secondary metabolites was also investigated.
Materials and method
Ligand collection, preparation and ADME analysis
The details of already reported bioactive compounds present in ashwagandha were collected from literature and their chemical structures were drawn using Chemsketch program (ACDLABS 11.0). The chemical structures of agonists such as nicotine, ABT-418, anabaseine, and epibatidine and antagonists such as gymnodimine, methyllycaconitine and spirolide were also downloaded from PubChem database and used for the studies. The geometries of all compounds (including generation of possible isomers and tautomers) were optimized using LigPrep module of the Schrödinger program with OPLS-2005 as force field. During the ligand preparations, the hydrogen atoms were added, the bond lengths and angles were also optimized.
Later in order to identify the drug likeliness (Lipinski rule of five), blood brain barrier (BBB) penetrability and CNS activity and ADME (Absorption, Distribution, Metabolism and Excretion) analysis has been done with the prepared ligands using the program QikProp. The information regarding the ADME properties plays an important role in the drug design process. QikProp program predicts a set of chemical descriptors relevant to the drug-likeness of the compounds. Minimized ligand structures were given as the input for ADME prediction. The acceptability of the compound as a drug based on Lipinski’s rule of five (Citation2001), BBB permeability, and CNS activity was also estimated from the results.
In order to predict the biological activities of CNS active ligands, Prediction of Activity Spectra for Substances (PASS) prediction server (http://www.pharmaexpert.ru/passonline/) was used. Usually PASS predicts 4000 kinds of biological activities such as pharmacological effects, mechanism of action, toxicity and adverse effects, interactions with metabolic enzymes and transporters influence on gene expressions. Even though PASS prediction server predicts the biological activities, it will not give any information about the stereo chemistry and binding affinity of the compounds towards a particular target. Hence we extended our studies to molecular docking to predict the binding affinity of the compounds towards nAChR.
Protein structure preparation, induced fit docking and binding energy analysis
In order to identify the binding affinity of secondary metabolites selected from Aswagandha towards nAChR, molecular docking studies has been carried out. Since the structure of extracellular ligand-binding domain of -nAChR was not available, the crystal structures of acetylcholine-binding protein (AChBP) from snail species such as Lymnaea stagnalis (Ls-AChBP) and Aplysia californica (Ac-AChBP) were taken for the docking studies. So far the 3D structure of LBD only solved and which have a good structural agreement with nAChR. The transmembrane and intracellular domain of the AChBP has not solved yet. It has been reported that the agonists and antagonists of nAChR can bind to AChBP. Therefore the structure of AChBP can be used instead of the N-terminal domain of the nAChRs for the docking studies (Paterson & Nordberg Citation2000; Brejc et al. Citation2001; Smit et al. Citation2001; Sala et al. Citation2008). In order to investigate the agonistic/ antagonistic properties of selected ligands, molecular docking studies have been carried out towards both agonist (open)- and antagonist (close)-bound conformations of the receptor. It was expected that the agonist will be stabilized only in the open conformation and antagonists in the closed conformation. The agonist-bound crystal structures such as Ls-AChBP in complex with nicotine (Protein databank ID (PDB ID): 1UW6) (Celie et al. Citation2004), Ac-AChBP in complex with anabaseine (PDB ID: 2WNL) (Ryan et al. Citation2009) and human
-nAChR-AChBP chimeric receptor in complex with epibatidine (PDB ID: 3SQ6) (Li et al. Citation2011) were downloaded from PDB. Similarly the crystal structures of antagonist-bound conformation such as Ac-AChBP in complex with methyllycaconitine (PDB ID: 2BYR) (Scott et al. Citation2005) and gymnodimine (PDB ID: 2X00) (Yves et al. Citation2010) from PDB were also downloaded from the PDB. All these protein structures were prepared for docking studies by adding hydrogen atoms, alleviating steric clashes, assigning partial charges, incorporating protonation states using protein preparation module of Schrödinger. The prepared structures were further optimized and minimized using OPLS 2005.
Binding of ligands to the ligand-binding sites may always induce some degrees of conformational changes and which can be reversed upon the dissociation of ligands. These kinds of induced fit effects were already been studied for different ligand-gated ion channels. So it was expected that induced fit docking can be implemented to inspect the agonistic and antagonistic nature of selected ligands. The induced fit docking (IFD) of prepared ligands was carried out with the program Schrödinger using a softened potential. Initially the ligands were allowed to search on an allowed grid space to get an optimum binding mode. The top 20 poses per ligand were generated and then applied plasticity to the residues (within 5 Å) located in the LBD using the prime module of the Schrödinger suite. Then the ligands were re-docked and the resultant poses were taken for further analysis.
After IFD, the docked poses were energy minimized and the binding free energy corresponding to each pose was calculated using the Prime MM-GBSA method. In this method, molecular mechanics energies (EMM), surface generalized born solvation model for polar solvation ( ), and a nonpolar solvation term (GNP) were being taken to calculate the total free energy. The term GNP includes nonpolar solvent accessible surface area and van der Waals interactions.
Molecular dynamics simulation
The result of induced fit docking studies has been confirmed by molecular dynamics (MD) simulation. The binding strength as well as residence of time of the selected ligands at the binding clefts of nAChR was also investigated through 15 ns MD simulation studies. The whole MD simulation studies were performed using Desmond molecular modeling package. The resultant protein–ligand complex obtained through IFD were prepared for MD simulation by soaking it into an orthorhombic box consisting TIP3P water molecules and ions such as Na+ and Cl− (for neutralizing the whole system). Intermediate structures of the protein–ligand complex during the MD simulations were saved at every 50 ps and superimposed to the original structure to measure the average change in displacement of atoms for a particular frame with respect to a reference frame. In that way, the root mean square deviation (RMSD) of ligands and proteins were deduced. Finally a graph was plotted by connecting the RMSD vs. time.
Results and discussion
Through the literature survey, the bioactive compounds of ashwagandha were explored and their names were given in Table . It is known that only CNS active compounds are eligible for the treatment of different neurodegenerative disorders. In order to prove the drug-like properties of the selected compounds with respect to neurological disorders, following parameters such as BBB penetrability, CNS activity, molecular weight, partition coefficient (LogP), number of hydrogen bond donors and acceptors (HBD and HBA), polar surface area (PSA), number of rotatable groups (rotor) and human oral absorption (HOA) were predicted using Qikprop (Pajouhesh & Lenz Citation2005). Among different compounds selected for studies, only four compounds (anaferine, anahygrine, cuscohygrine and isopelletierine) were possessed promising CNS activity and BBB penetrability. The physicochemical properties for all the selected compounds were summarized in Table . Later the structure activity relation of the promising ligands (anaferine, anahygrine, cuscohygrine and isopelletierine) was correlated with the biological activities using the program ‘PASS’. The program predicted that there is a highest probability for these compounds to act as nAChR.
Table 2. List of bioactive compounds of ashwagandha identified through literature survey.
Table 3. Predicted physicochemical properties of the selected compounds.
Since the PASS prediction results and Qikprop results positively correlates each other, it was assumed that these ligands can be taken against different neurodegenerative disorders where nAChR is the drug target. Hence the binding affinities of the compounds towards nAChR were investigated by induced fit docking and followed by MM-GBSA method. It was already proved that the ‘loop C’ (located near to the LBD) can adopt an ‘open’ or ‘close’ conformation upon binding to the antagonists and agonist (Celie et al. Citation2004; Hansen et al. Citation2004, Citation2005; Ulens et al. Citation2006). Hence, it is expected that the agonists prefer ‘closed’ conformation of the C loop for binding while the antagonists prefer ‘open’ conformation. This hypothesis was tested and validated by docking the known agonists (nicotine, ABT-418, anabaseine and epibatidine) and antagonists (gymnodimine, methyllycaconitine, spirolide) towards the closed and open conformations of AChBP structures (PDB IDs: 1UW6 (Celie et al. Citation2004) and 2WNL (Ryan et al. Citation2009). The binding free energy (shown in Table ) was selected as the criteria to differentiate the agonists and antagonists. The results confirmed that the adopted molecular docking experiment is smart enough to distinguish the agonists from antagonists. The agonists and antagonists exhibited best binding energies at their respective closed and open conformations. Even though the agonists bind to both closed and open conformations, a remarkable difference in the binding energies was observed when it binds to the closed conformations. Also, in the case of antagonists, they could be dock only to the open conformation.
Table 4. Binding energies (in kcal/mol) of some of the known agonists and antagonists towards AChBP in agonist and antagonist bound conformations.
This validated strategy was used for distinguishing the agonistic and antagonistic properties of selected ligands. Since the selected molecules contain an asymmetric center, all possible stereo isomers of the compounds were generated (Table ) and docked to open and closed conformations of the receptor. It has already been reported that S form of nicotine is more active than the R form. The docking studies of S and R form of nicotine on the open conformation of AChBP was also studied and revealed that (S)-nicotine (−68.90 kcal/mol) is better than that of (R)-nicotine (−59.37 kcal/mol). Similarly it was found that all the other selected agonists preferably bind to the open conformation of the receptor with highest affinity than the closed conformation. The binding energy obtained for all compounds in both conformations are given in Table .
Table 5. Chemical structures of all stereo isomers of the compounds selected for study.
Table 6. Binding energies (in kcal/mol) of the selected compounds on agonist and antagonist bound conformations of the target.
The ligand-binding domain of human -AChBP chimeric structure and human
receptor share highest structural similarities. The identity of two sequence has been predicted by sequence alignment and found to approximately 64% of identity and their sequence alignment is shown in Figure . It was also noted that the ligand-binding residues are also shares high structural similarities. Hence the binding of the selected ligands was carried out against human
-AChBP chimeric structure since it provides an accurate structural details of the ligand binding.
In the case of anaferine, 1, 3-di [(2R)-piperidin-2-yl] propan-2-one (RR-anaferine) was found to exhibit better binding energies (−69.88 kcal/mol) than other stereo forms. Binding involves a cation-pi interaction between protonated nitrogen atom of piperidine ring and W145 of A chain. The other piperidine ring forms hydrogen bonds with Q55, S34 and D160 of B chain. 61 van der Waals (vdW) contacts (≤ 4 Å) between the compound and the receptor were found.
The 1-[(2R)-1-methylpyrrolidin-2-yl]-3-[(2S)-piperidin-2-yl] propan-2-one (RS-anahygrine) showed better binding energy (−87.02 kcal/mol) compared to other forms of anahygrine. The binding is mainly stabilized by cation-pi interaction between positively charged nitrogen atom of pyrrolidine ring and the protein residues Y184, Y191 and W145. Moreover, a hydrogen bond with N atom of piperidine ring and Gln55 was also found. The number of van der Waal’s contacts was 64.
In the case of cuscohygrine, 1-[(2R)-1-methylpyrrolidin-2-yl]-3-[(2S)-1-methylpyrrolidin-2-yl] propan-2- one (RS-cuscohygrine) has exhibited highest binding energy (−74.09 kcal/mol) than other forms of cuscohygrine. Two hydrogen bonds and 58 van der Waal’s contacts (≤4 Å) were observed between the ligand and the protein.
In the case of isopelletierine, two stereo forms are available. The 1-[(2R)-piperidin-2-yl] propan-2-one (R-isopelletierine) is binding with slightly more negative energy (−53.39 kcal/mol) than S-isopelletierine (−51.56 kcal/mol). A cation-pi interaction with protonated N atom of piperidine ring and Y191 was seen between R-isopelletierine and the protein. Hydrogen bonds with Q114 and W145 were also observed. 47 vdW contacts (≤4 Å) were found between the compound and the receptor.
From the interactions studies, it was observed that the ligands are mediating a strong interaction with the key residues such as Y91, Y191 and Y184 of the loop C, thereby stabilizing the loop C in a closed conformation and enable the receptor to adopt open conformation and there by elicit a biological response. It was also observed that all the compounds were interacting with the residue W145, which plays a pivotal role in the binding of agonists. The binding modes of the compounds those exhibiting highest binding energy were shown in Figure . The binding parameters of RR anaferine, RS-anahygrine, RS-cuscohygrine and R-Isopelletierine on chimeric receptor are given in Table .
Figure 2. (a) The pentameric structure of the receptor (PDB ID:3SQ6), (b) The orientations of all compounds (RR-anaferine, RS-anahygrine, RS-cuscohygrine and R-isopelletierine) docked to the binding site at the interface of two monomers, Details of interaction of (c) RR-anaferine, (d) RS-anahygrine, (e) RS-cuscohygrine and (f) R-isopelletierine bound in the binding site of -AChBP chimeric structure (Ligands are given as thick lines, receptor as thin lines and hydrogen bonds are given as dashed lines).
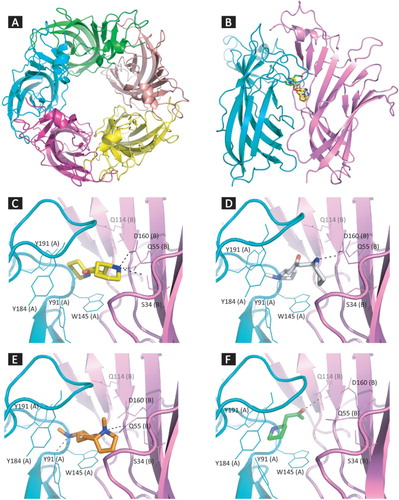
Table 7. Molecular and binding properties of the compounds on human
-AChBP chimeric structure.
Calculating interface area between the compounds and the receptor is one of the promising methods to identify how strongly a ligand is bound to its target. Hence the interface area of the above-discussed compounds has been calculated and observed that there is a drastic decrease in the interface area of the compounds at the agonist bound conformations when compared to the agonist-bound conformation. The results clearly support the agonistic nature of the ligands.
In order to substantiate the agonistic nature of promising ligands, their size (molecular volume and surface area) has been compared with already reported agonists (PDB IDs: 2BR7, 2BYQ, 2BYS (Scott et al. Citation2005), 1UW6 (Celie et al. Citation2004), 2PGZ, 2PH9 (Scott & Palmer Citation2007), 2WNL (Ryan et al. Citation2009), 2ZJU, 2ZJV (Ihara et al. Citation2008), 3C79 (Todd et al. Citation2008), 3C84 (Todd et al. Citation2008), 1UV6 (Celie et al. Citation2004), 2WN9, 2WNC (Ryan et al. Citation2009), 3SQ6 (Li et al. Citation2011), 2W8F (Brams et al. Citation2011) and 4DBM (Grimster et al. Citation2012) and antagonists (PDB IDs: 2BYR (Scott et al. Citation2005), 2WZY, 2XOO (Yves et al. Citation2010), 2XYS (Todd et al. Citation2008), 2XYT (Celie et al. Citation2004) 2WNJ (Ryan et al. Citation2009) and 4ALX (Scott et al. Citation2005). The results indicated that agonists tend to be small (molecular volume < 400 Å3), whereas antagonists were bulkier (molecular volume > 400 Å3 except for 2XYS) in nature. The molecular volume was also found to below 400 Å3, indicating that the size of the compounds was comparable to that of other known agonists.
Various molecular modeling experiments that are carried out on the selected ligands focuses to the possibilities to act as agonists to nAChR. Even though the agonistic natures of the ligands are confirmed, it has to investigate the stability of the agonists at the LBD. The residence of time of the ligands (such as RR anaferine, RS-anahygrine, RS-cuscohygrine and R-Isopelletierine) on the chimeric receptor complex was investigated by MD simulation studies. Overall 15 ns MD simulation studies revealed that the ligands are stable in their binding positions. Intermediate structures that are saved at different intervals were superimposed and deduced the RMSD (Figure ). The results suggested that none of the ligands were distorted more than 1.20 Å from their initial position. It was also noticed that majority of the interactions were retained during the simulations. Hence it can be easily inferred that the suggested ligands may be strong at their binding positions.
Conclusion
In the present study, computational resources were utilized to explain the possible biological activities of compounds from ashwagandha in connection with AD. Pharmacological and structure activity relationship predictions explains the following ligands such as anaferine, anahygrine, cuscohygrine and isopelletierine are possessing CNS activity and nAChR agonistic activity. nAChR agonists are always important in the treatment of neurological disorders such as AD. The agonist can fetch the nAChR to an open conformation and thereby triggering the biological activity. Hence the four ligands (anaferine, anahygrine, cuscohygrine and isopelletierine) including their isomers were subjected to molecular docking studies and from their binding energies an assumption was made that the selected ligands may be agonist to nAChR. Later this assumption was confirmed by comparing the ligand volumes and surface area with already reported agonists. All the reported agonists and proposed compounds have good agreement in the physical properties such as surface area and volume. Later the binding strength of these ligands was confirmed by investigating the residence of time of the ligand at the LBD. The MD simulation studies explained that the selected ligands are stable at their binding positions. All these investigations focus to the possibilities of these ligands to act as nAChR agonist.
Even though the traditional and folk medicines are famous for different life-threatening diseases, their exploitation has not been performed in the current era. The ignorance of their exact mode of action towards different drug targets retracted them from the direct testing against various disorders. It is a known fact that there is a lack of bridge between the modern medicines and these folk medicines. If a focused and converged investigation can be performed for these medicines, defiantly it will throw light to the presence of various important secondary metabolites and their mode of actions towards the target. Even if the molecular basis of investigation gives a better understanding to the mechanism of action, it will be often expensive. Here the in silico based investigation is promising and also it is the easiest way to bridge the folk medicine to the modern medicine. So similar kind of in silico based investigation can be employed on different traditional medicines and plant secondary metabolites for the identification of better leads against various disorders.
Acknowledgements
The authors gratefully acknowledge the ‘Bioinformatics Infrastructure Facility’ (supported by DBT, Govt. of India) located at the department of Biotechnology and Microbiology, Kannur University for providing the computational works. C.R. acknowledges Indian Council of Medical Research for her Senior Research Fellowship. K.V.D. acknowledges Indian Institute of Science, Education and Research for post-doctoral fellowship.
Disclosure statement
No potential conflict of interest was reported by the authors.
References
- Asthana R, Raina MK. 1989. Pharmacology of Withania somnifera (L.) Dunal-a review. Indian Drugs. 26:199–205.
- Atta-ur-Rahman, Abbas S, Dur-e-Shahwar, Jamal SA, Choudhary MI. 1993. New withanolides from Withania sp. J Nat Prod. 56:1000–1006. doi: 10.1021/np50097a003
- Atta-ur-rahman, Jamal SA, Choudhary MI, Asif E. 1991. Two withanolides from Withania somnifera. Phytochemistry. 30:3824–3825. doi: 10.1016/0031-9422(91)80125-K
- Bartus RT, Dean RL, Beer B, Lippa AS. 1982. The cholinergic hypothesis of geriatric memory dysfunction. Science. 217:408–417. doi: 10.1126/science.7046051
- Bhattacharya SK, Kumar A, Ghosal S. 1995. Effects of glycowithanolides from Withania somnifera on an animal model of Alzheimer’s disease and perturbed central cholinergic markers of cognition in rats. Phytother Res. 9:110–113. doi: 10.1002/ptr.2650090206
- Bhattacharya SK, Satyan KS, Ghosal S. 1997. Antioxidant activity of glycowithanolides from Withania somnifera. Indian J Exp Biol. 35:236–239.
- Brams M, Pandya A, Kuzmin D, Van Elk R, Krijnen L, Yakel JL, Tsetlin V, Smit AB, Ulens C. 2011. A structural and mutagenic blueprint for molecular recognition of strychnine and d-tubocurarine by different cys-loop receptors. Plos ONE Biology. 9:01034.
- Brejc K, van Dijk WJ, Klaassen RV, Schuurmans M, van Der Oost J, Smit AB, Sixma TK. 2001. Crystal structure of an ACh-binding protein reveals the ligand-binding domain of nicotinic receptors. Nature. 411:269–276. doi: 10.1038/35077011
- Celie PHN, van Rossum-Fikkert SE, van Dijk WJ, Brejc K, Smit AB, Sixma TK. 2004. Nicotine and carbamylcholine binding to nicotinic acetylcholine receptors as studied in AChBP crystal structures. Neuron. 41:907–914. doi: 10.1016/S0896-6273(04)00115-1
- Choudary MI, Abbas S, Jamal AS, Atta-ur-Rahman E. 1996. Withania somnifera – a source of exotic withanolides. Heterocycles. 42:555–563. doi: 10.3987/COM-94-6935
- Coyle JT, Price DL, Delong MR. 1983. Alzheimer’s disease: a disorder of cortical cholinergic innervation. Science. 219:1184–1190. doi: 10.1126/science.6338589
- D’Andrea MR, Nagele RG. 2006. Targeting the alpha 7 nicotinic acetylcholine receptor to reduce amyloid accumulations in Alzheimer’s disease pyramidal neurons. Curr Pharm Des. 12:677–684. doi: 10.2174/138161206775474224
- Dani JA, Bertrand D. 2007. Nicotinic acetylcholine receptors and nicotinic cholinergic mechanisms of the central nervous system. Annu Rev Pharmacol. 47:699–729. doi: 10.1146/annurev.pharmtox.47.120505.105214
- Davies P, Feisullin S. 1981. Postmortem stability of -bungarotoxin binding sites in mouse and human brain. Brain Res. 216:449–454. doi: 10.1016/0006-8993(81)90148-7
- Davies P, Maloney AJ. 1976. Selective loss of central cholinergic neurons in Alzheimer’s disease. Lancet. 2:1403–1404. doi: 10.1016/S0140-6736(76)91936-X
- Dhuley JN. 2001. Retracted: nootropic-like effect of ashwagandha (Withania somnifera L.) in mice. Phytother Res. 15:524–528. doi: 10.1002/ptr.874
- Elsakka M, Grigorescu E, Stanescu U. 1990. New data referring to chemistry of Withania somnifera species. Revista medico-chirurgicala a Societatii de Medici si Naturalisti din Iasi. 94:385–387.
- Fisher A. 2008. Cholinergic treatments with emphasis on M1 muscarinic agonists as potential disease-modifying agents for Alzheimer’s disease. Neurotherapeutics. 5:433–442. doi: 10.1016/j.nurt.2008.05.002
- Gotti C, Clementi F. 2004. Neuronal nicotinic receptors: from structure to pathology. Prog Neurobiol. 6:363–396. doi: 10.1016/j.pneurobio.2004.09.006
- Grimster NP, Stump B, Fotsing JR, Weide T, Talley TT, Yamauchi JG, Nemecz Kim C, Ho KY, Sharpless KB, Taylor P, Fokin VV. 2012. Generation of candidate ligands for nicotinic acetylcholine receptors via in situ click chemistry with a soluble acetylcholine binding protein template. J Am Chem Soc. 134:6732–6740. doi: 10.1021/ja3001858
- Gupta GL, Rana AC. 2007. Withania somnifera (Ashwagandha): a review. Pharmacognosy Review. 1:129–136.
- Gupta SK, Dua A, Vohra BP. 2003. Withania somnifera (Ashwagandha) attenuates antioxidant defense in aged spinal cord and inhibits copper induced lipid peroxidation and protein oxidative modifications. Drug Metabol Drug Interact. 19:211–222. doi: 10.1515/DMDI.2003.19.3.211
- Hansen SB, Sulzenbacher G, Huxford T, Marchot P, Taylor P, Bourne Y. 2005. Structures of Aplysia AChBP complexes with nicotinic agonists and antagonists reveal distinctive binding interfaces and conformations. The EMBO Journal. 24:3635–3646. doi: 10.1038/sj.emboj.7600828
- Hansen SB, Talley TT, Radic Z, Taylor P. 2004. Structural and ligand recognition characteristics of an acetylcholine-binding protein from Aplysia californica. J Biol Chem. 279:24197–24202. doi: 10.1074/jbc.M402452200
- Heinrich M, Teoh HL. 2004. Galanthamine from snowdrop – the development of a modern drug against Alzheimer’s disease from local Caucasian knowledge. J Ethnopharmacol. 92:147–162. doi: 10.1016/j.jep.2004.02.012
- Howes MJ, Perry E. 2011. The role of phytochemicals in the treatment and prevention of dementia. Drug Aging. 28:439–468. doi: 10.2165/11591310-000000000-00000
- Ihara M, Okajima T, Yamashita A, Oda T, Hirata K, Nishiwaki H, Morimoto T, Akamatsu M, Ashikawa Y, Kuroda S, et al. 2008. Crystal structures of Lymnaea stagnalis AChBP in complex with neonicotinoid insecticides imidacloprid and clothianidin. Invertebr Neurosci. 8:71–81. doi: 10.1007/s10158-008-0069-3
- Itier V, Bertrand D. 2001. Neuronal nicotinic receptors: from protein structure to function. FEBS Letters. 504:118–125. doi: 10.1016/S0014-5793(01)02702-8
- Jayaprakasam B, Padmanabhan K, Nair MG. 2010. Withanamides in Withania somnifera fruit protect PC-12 cells from beta-amyloid responsible for Alzheimer’s disease. Phytother Res. 24:859–863.
- Jensen AA, Frolund B, Liljefors T, Krogsgaard-Larsen P. 2005. Neuronal nicotinic acetylcholine receptors: structural revelations, target identifications, and therapeutic inspirations. J Med Chem. 48:4707–4745. doi: 10.1021/jm040219e
- Kapoor LD. 2001. Handbook of Ayurvedic medicinal plants. London: CRC Press; p. 337–338.
- Kar S, Slowikowski SP, Westaway D, Mount HT. 2004. Interactions between beta-amyloid and central cholinergic neurons: implications for Alzheimer’s disease. J. Psychiatry Neurosci. 29:427–441.
- Kataria H, Wadhwa R, Kaul SC, Kaur G. 2012. Water extract from the leaves of Withania somnifera protect RA differentiated C6 and IMR-32 cells against Glutamate-induced excitotoxicity. PLoS ONE. 7:e37080. 10.1371/journal.pone.0037080. doi: 10.1371/journal.pone.0037080
- Kem WR. 2000. The brain nicotinic receptor may be an important therapeutic target for the treatment of Alzheimer’s disease: studies with DMXBA (GTS-21). Behavioural Brain Research. 113:169–181. doi: 10.1016/S0166-4328(00)00211-4
- Krall WJ, Sramek JJ, Cutler NR. 1999. Cholinesterase inhibitors: a therapeutic strategy for Alzheimer disease. Ann Pharmacother. 33:441–450. doi: 10.1345/aph.18211
- Kuboyama T, Tohda C, Komatsu K. 2005. Neuritic regeneration and synaptic reconstruction induced by withanolide A. Br J Pharmacol. 144:961–971. doi: 10.1038/sj.bjp.0706122
- Kumar S, Harris RJ, Seal CJ, Okello EJ. 2012. An aqueous extract of Withania somnifera root inhibits amyloid β fibril formation in vitro. Phytother Res. 26:113–117. doi: 10.1002/ptr.3512
- Kumar V. 2006. Potential medicinal plants for CNS disorders: an overview. Phytother Res. 20:1023–1035. doi: 10.1002/ptr.1970
- Li SX, Huang S, Bren N, Noridomi K, Dellisanti CD, Sine SM, Chen L. 2011. Ligand-binding domain of an alpha 7-nicotinic receptor chimera and its complex with agonist. Nature Neurosci. 14:1253–1259. doi: 10.1038/nn.2908
- Lindstrom JM. 2003. Nicotinic acetylcholine receptors of muscles and nerves: comparison of their structures, functional roles, and vulnerability to pathology. Ann N Y Acad Sci. 998:41–52. doi: 10.1196/annals.1254.007
- Lipinski CA, Lombardo F, Dominy BW, Feeney PJ. 2001. Experimental and computational approaches to estimate solubility and permeability in drug discovery and development settings. Adv Drug Deliv Rev. 46:3–26. doi: 10.1016/S0169-409X(00)00129-0
- Mahnir V, Lin B, Prokai-Tatrai K, Kem WR. 1998. Pharmacokinetics and urinary excretion of DMXBA (GTS-21), a compound enhancing cognition. Biopharm Drug Disp. 19:147–151. doi: 10.1002/(SICI)1099-081X(199804)19:3<147::AID-BDD77>3.0.CO;2-9
- Martin LF, Kem WR, Freedman R. 2004. Alpha-7 nicotinic receptor agonists: potential new candidates for the treatment of schizophrenia. Psychopharmacology. 174:54–64. doi: 10.1007/s00213-003-1750-1
- McKay BE, Placzek AN, Dani JA. 2007. Regulation of synaptic transmission and plasticity by neuronal nicotinic acetylcholine receptors. Biochem Pharmacol. 74:1120–1133. doi: 10.1016/j.bcp.2007.07.001
- Meyer EM, King MA, Meyers C. 1998. Neuroprotective effects of 2,4-dimethoxybenzylidene anabaseine (DMXB) and tetrahydroaminoacridine (THA) in neocortices of nucleus basalis lesioned rats. Brain Res. 768:49–56. doi: 10.1016/S0006-8993(97)00536-2
- Miroslav P. 2012. Alpha7 nicotinic acetylcholine receptor is a target in pharmacology and toxicology. Int J Mol Sci. 13:2219–2238. doi: 10.3390/ijms13022219
- Pajouhesh H, Lenz GR. 2005. Medicinal chemical properties of successful central nervous system drugs. NeuroRx. 2:541–553. doi: 10.1602/neurorx.2.4.541
- Paterson D, Nordberg A. 2000. Neuronal nicotinic receptors in the human brain. Prog Neurobiol. 61:75–111. doi: 10.1016/S0301-0082(99)00045-3
- Rastogi RP, Mehrotra BN. 1998. Compendium of Indian Medicinal Plants. New Delhi: Central Drug Research Institute.
- Ringman JM, Frautschy SA, Cole GM, Masterman DL, Cummings JL. 2005. A potential role of the curry spice curcumin in Alzheimer’s disease. Curr Alzheimer Res. 2:131–136. doi: 10.2174/1567205053585882
- Rosini M, Andrisano V, Bartolini M, Bolognesi ML, Hrelia P, Minarini A, Tarozzi A, Melchiorre C. 2005. Rational approach to discover multipotent anti-Alzheimer drugs. J. Med Chem. 48:360–363. doi: 10.1021/jm049112h
- Russo A, Izzo AA, Cardile V, Borrelli F, Vanella A. 2001. Indian medicinal plants as antiradicals and DNA cleavage protectors. Phytomedicine. 8:125–132. doi: 10.1078/0944-7113-00021
- Ryan EH, Gerlind S, Jianxin S, Todd TT, Sandrine C, William RK, Palmer T, Pascale M, Yves B. 2009. Structural determinants for interaction of partial agonists with acetylcholine binding protein and neuronal nicotinic acetylcholine receptor. EMBO Journal. 28:3040–3051. doi: 10.1038/emboj.2009.227
- Sala F, Nistri A, Criado M. 2008. Nicotinic acetylcholine receptors of adrenal chromaffin cells. Acta Physiologica. 192:203–212. doi: 10.1111/j.1748-1716.2007.01804.x
- Schliebs R, Liebmann A, Bhattacharya SK, Kumar A, Ghosal S, Bigl V. 1997. Systemic administration of defined extracts from Withania somnifera (Indian Ginseng) and Shilajit differentially affects cholinergic but not glutamatergic and GABAergic markers in rat brain. Neurochem Int. 30:181–190. doi: 10.1016/S0197-0186(96)00025-3
- Scott BH, Gerlind S, Tom H, Pascale M, Palmer T, Yves B. 2005. Structures of Aplysia AChBP complexes with nicotinic agonists and antagonists reveal distinctive binding interfaces and conformations. The EMBO Journal. 24:3635–3646. doi: 10.1038/sj.emboj.7600828
- Scott BH, Palmer T. 2007. Galanthamine and non-competitive inhibitor binding to ACh-binding protein: evidence for a binding site on non -subunit interfaces of heteromeric neuronal nicotinic receptors. J Mol Biol. 369:895–901. doi: 10.1016/j.jmb.2007.03.067
- Selkoe D. 2001. Alzheimer’s disease: genes, proteins, and therapy. Physiol Rev. 81:741–766.
- Shah ZA, Nada SE, Dore S. 2011. Heme oxygenase 1, beneficial role in permanent ischemic stroke and in Gingko biloba (EGb 761) neuroprotection. Neuroscience. 180:248–255. doi: 10.1016/j.neuroscience.2011.02.031
- Singh S, Kumar S. 1999. Withania somnifera: the Indian ginseng Ashwagandha (Report). Lucknow, India: Central Institute of Medicinal and Aromatic Plants, 131–201.
- Small DH, Fodero LR. 2002. Cholinergic regulation of synaptic plasticity as a therapeutic target in Alzheimer’s disease. J Alzheimers Dis. 4:349–355.
- Smit AB, Syed NI, Schaap D, van Minnen J, Klumperman J, Kits KS, Lodder H, van der Schors RC, van Elk R, Sorgedrager B, et al. 2001. A glia-derived acetylcholine-binding protein that modulates synaptic transmission. Nature. 411:261–268. doi: 10.1038/35077000
- Sugaya K, Giacobini E, Chiappinelli VA. 1990. Nicotinic acetylcholine receptor subtypes in human frontal cortex; changes in Alzheimer’s disease. J Neurosci Res. 27:349–359. doi: 10.1002/jnr.490270314
- Todd TT, Michal H, Ryan EH, Zoran R, Motohiro T, John EC, Palmer T. 2008. Atomic interactions of neonicotinoid agonists with AChBP: molecular recognition of the distinctive electronegative pharmacophore. Proc Natl Acad Sci USA. 105:7606–7611. doi: 10.1073/pnas.0802197105
- Tohda C, Kuboyama T, Komatsu K. 2005. Search for natural products related to regeneration of the neuronal network. Neurosignals. 14:34–45. doi: 10.1159/000085384
- Ulens C, Hogg RC, Celie PH, Bertrand D, Tsetlin V, Smit AB, Sixma TK. 2006. Structural determinants of selective alpha-conotoxin binding to a nicotinic acetylcholine receptor homolog AChBP. Proc Natl Acad Sci USA. 103:3615–3620. doi: 10.1073/pnas.0507889103
- Wevers A, Schröder H. 1999. Nicotinic acetylcholine receptors in Alzheimer’s disease. J Alzheimers Dis. 1:207–219.
- Wu PF, Zhang Z, Wang F, Chen JG. 2010. Natural compounds from traditional medicinal herbs in the treatment of cerebral ischemia/reperfusion injury. Acta Pharmacol Sin. 31:1523–1531. doi: 10.1038/aps.2010.186
- Yves B, Zoran R, Rómulo A, Todd TT, Evelyne B, Denis S, Palmer T, Jordi M, Pascale M. 2010. Structural determinants in phycotoxins and AChBP conferring high affinity binding and nicotinic AChR antagonism. Proc Natl Acad Sci USA. 107:6076–6081. doi: 10.1073/pnas.0912372107
- Zarei MM, Radcliffe KA, Chen D, Patrick JW, Dani JA. 1999. Distributions of nicotinic acetylcholine receptor alpha7 and beta2 subunits on cultured hippocampal neurons. Neuroscience. 88:755–764. doi: 10.1016/S0306-4522(98)00246-2