ABSTRACT
One of the primary purposes of pine cones is the protection and distant dispersal of pine seeds. Pine cones open and release their embedded seeds on dry and windy days for long-distance dispersal. In this study, how the pine seeds attach to/detach from the pine cone scale for efficient seed dispersal was experimentally investigated by using an X-ray micro-imaging technique. The cone and seeds adhere to each other in the presence of water, which could be explained by the surface tension and the contact angle hysteresis. Otherwise, without water, the waterproof seed wing surface permits rapid drying for detach and dispersion. On the other hand, during wildfires, pine cones open their seed racks and detach the pine seeds from pine cones for rapid seed dispersal. Due to these structural advantages, pine seeds are released safely and efficiently in normal conditions. These advantageous structures could be mimicked in practical applications.
Introduction
Species reproduction and propagation are the most important objectives of every living organism, and pine trees propagate their species effectively with pine cone. Pines are gymnosperm and reproduce through cones that are specially adapted for seed dispersal (Owens et al. Citation1981; Lanner Citation1998; Owens Citation2006; Taiz & Zeiger Citation2010; Fernando Citation2014). Pine cone has advantageous structures for effective propagation, and the pine cone geometry increases the frequency of seed pollination, thus enhancing the fertilization potential (Niklas Citation1982). Not only the structures of pine cone, but also the structures of pine seed have influence on efficient seed dispersal (Benkman Citation1995; Vander Wall Citation2003): shapes, weights and sizes of seeds affect dispersal distances, substrate choices and microhabitat choices.
In addition, pine cone moves in response to the external condition. On rainy days, pine cones fold their scales, which prevent the seeds from spreading under wet condition. On the other hand, gaps between these scales are open, which release the seeds on sunny and dry days (Dawson et al. Citation1997). As a result of these structures and movements, the seeds are dispersed far from the parent tree, which enhances the survival of species. To date, only the mechanism underlying these folding and opening movements has been studied by researchers in various fields. When water is either absorbed or expelled in response to humidity, the tissue expands or shrinks anisotropically in a direction that is perpendicular to the orientation of the fibril (Allen & Wardrop Citation1964; Harlow et al. Citation1964; Fahn & Werker Citation1972; Bar-On et al. Citation2014). At the organ level, the asymmetric fibril orientation converts local swelling and shrinking into global bending movements (Reyssat & Mahadevan Citation2009). This change occurs in a finely tuned manner. Dawson et al. reported that hygroscopic expansion coefficient (0.06 ± 0.02 in their experiment) of fiber in the cone is significantly lower than that of the sclereids (0.20 ± 0.04 in their experiment) for only a 1% change in relative humidity at 23°C (Citation1997). Hygroexpansion was evaluated in terms of humidity expansion coefficients along three orthogonal directions. The stiffness and expansion coefficients of each layer are functions of the component compositions and the microfibrill angle with respect to the cell axis (Skaar Citation1988).
Such microscopic humidity-induced tension causes the cells to undergo macroscopic changes, and the mechanism used to induce movement of pine cone scales and their structures has been mimicked (Reyssat & Mahadevan Citation2009; Andres et al. Citation2014).
Only the pine cone movements have received substantial attention from many scientists; however, one of the primary functions of the pine cone is seed dispersal. Indeed, without cone movements, the seeds would be released on rainy and humid days, which would lead to short-range dispersal (Sharpe & Fields Citation1982). As a result, both the parent tree and the progeny would struggle to survive. It is problematic for species, as the viability of the parent tree may be threatened by sharing nutrients with its progeny, or disturbances to the parent tree may inhibit germination (Lanner Citation1998). Consequently, since efficient pine seed dispersal is very important to pine reproduction, the interaction between pine seeds and pine cone scales is also important. Sometimes, the seeds should be attached to the pine cone for preventing dispersal and sometimes, the seeds should be released from the pine cone for rapid dispersal. In this study, we examined the relation between pine cones and seeds with regard to what kinds of advantageous structure do they have for efficient dispersal. The tested pine cones and seeds were Japanese red pine (Pinus densiflora), which is common in Korea, Japan and northeastern China (Lian et al. Citation2001). Structural advantages and interactions between the cones and seeds are primarily discussed. This type of systematic approach will be useful in understanding the ecology of pines, and the structural advantages identified can be applied to practical engineering and industrial fields.
Results
Structural characteristics of pine cone and its seeds
Pine cones have specific structures for pine seeds (Owens et al. Citation1981; Owens Citation2006; Fernando Citation2014). Pine seeds are fertilized and matured in the gap between the cone’s central frame and scales, and wings are developed on the surface of the scales ((a)).
Figure 1. Pine seed morphology and dispersal from the pine cone into the air. (a) A pine seed matures in a pine cone. (b) The pine seed consists of the seed and seed wing. (c,d) Schematics showing how pine cone scales respond to dry and rainy weather; the figures were generated using the 3ds Max software (Autodesk Inc., USA). (c) On a dry and sunny day, cone scales open and disperse pine seed. (d) In contrast, on a humid and rainy day, the cone folds its scales and prevents seed release.
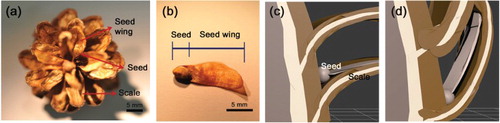
Depending on dispersal methods, the sizes and shapes of seed wings are different. For example, a wind-pollinated seed has a long wing, which is helpful to long-distance flight. The pine seed comprises two parts: the seed and the wing ((b)). As a result of their light weight and the attached wing structure, pine seeds can be dispersed up to great distances by wind (Cremer Citation1971; Lanner Citation1985; Benkman Citation1995). In spite of helpful pine seed shape for long-distance dispersal, unsuitable environmental conditions lead to short-range dispersal that can result in a critical situation for both the parent tree and the seeds.
To avoid this kind of situation, the cone scales are only opened and seeds are released on sunny dry days (Dawson et al. Citation1997) ((c)). Furthermore, as the seeds cannot be distantly dispersed and may be damaged by wet weather condition, the cone scales were folded due to the humidity and hold the seeds inside ((d)). Consequently, the movements of the cone are strongly related to the wind-blown dispersal of the seeds.
Pine seeds attach to pine cone using water attractive force
Under dry conditions, the pine seed wing and scale usually are usually detached. We elevated the angle between the pine seed wing and scale slowly for a simple experiment ((a–d)). When the angle reaches φs = 56.9 ± 4.5°, the seed wing begins to separate from the scale and rolls downward ((b)).
Figure 2. Adhesion between pine seeds and pine cone scales. (a–d) The angle (φ) of the pine seed on the scale was gradually increased to test its adhesion with and without water. (a) A dry seed is laid on the pine cone scale and the angle of slide (φ) is gradually elevated. (b) A dry seed is started to slip as the slide angle increases over φs = 56.9°. (c,d) In contrast, a wet seed firmly adheres to the scale. Even at φ = 90°, the pine seed is not detached from the pine cone scale. (e) The notion of contact angle hysteresis explains the observed adhesion of pine seed to the glass with 2 separated water films observed in experiment. Here, we assume that the gravity creates advancing and receding angles θwa > θwr at the pine seed–water interface, and θga > θgr at the water–glass interface, located at specified positions. The contact angles for the second water film are assumed to be the same as those for the first water film. We denote the length of the pine seed to be L. (f) There is a gap between the dry scale and the seed wing. (g) When the gap is filled with water, the attractive force of water makes the seed wing attach to the scale.
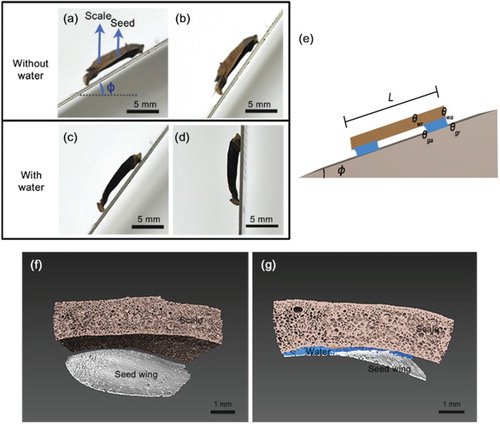
In contrast, the presence of water between the pine seed wing and scale drastically alters the result. When the gap between the seed wing and the scale is filled with water, the seed wing does not get separated from the scale even at an angle of φ = 90° ((d)). Moreover, the seed wing and the scale remain attached to each other even after being overturned and shaken.
The morphology of the pine seed wing and scale, under dry and wet conditions, was observed three dimensionally using synchrotron X-ray computed tomography. Under regular dry conditions, a gap between the seed wing and scale exists ((f)); however, under wet conditions, the gap between the seed wing and scale is filled with water ((g)). Therefore, the pine seed wing and scale are firmly attached to each other due to the attractive forces between water and the seed wing and scale.
To explain the observed adhesion due to the capillary effect of water, we use the notion of contact angle hysteresis. In the experiment, there are two areas with thin water film between pine seed and the glass ((d,e)), and the water films would have different advancing and receding angles due to gravity, that is, the phenomenon of contact angle hysteresis (Joanny & De Gennes Citation1984; Gao & McCarthy Citation2006). Since the glass and the pine seed have different surface properties and thus a different wettability, the advancing and receding angles would not be the same between the pine seed–water and the water–glass interfaces. Let θwa, θwr be the advancing and receding angles, respectively, at the pine seed–water interface, and θga, θgr be those at the water–glass interface. Then by definition θwa > θwr and θga > θgr, but θwa, θga would locate at diagonal position since the gravity on pine seed drags water to make the lower contact angle at pine seed–water interface smaller, but the gravity on water drags itself to make the upper contact angle at water–glass interface smaller ((e)). Now, the adhesion forces can be calculated from the contact angles and the surface tension of water. Notice that the adhesion is 2-fold; pine seed attaches to the glass only when pine seed adheres to the water films and the pine seed and water films adhere to the glass. To see whether the pine seed adhered to the water films, we could calculate the maximum surface tension force achieved by the maximum advancing angle and minimum receding angle
:
(1)
where γw is the surface tension of water–gas interface and a is the width of the pine seed (). If
which is the gravity force on the seed, with m being the mass of the pine seed ((e)), then the pine seed adheres to the water films. The most challenging part of this calculation is to determine the values of
The contact angles at water–wood interface usually come from direct measurements rather than theoretical deductions (Gindl et al. Citation2001). While the direct measurement of advancing and receding angles is possible, it could be difficult when the droplet is under motion due to the roughness and absorbability of wood, let alone measuring the contact angles of a thin water film between a wood plate and a glass (Gardner et al. Citation1991). Here we use the contact angles measured in to show that even the surface properties of the pine seed are not carefully engineered and the light weight of the pine seed still allows adhesion. Plugging in
into Equation (1), one would obtain
which means that the pine seed adheres to the water films even on a slope vertical to the ground.
Table 1. Experiment parameter for surface tension estimation.
To theoretically deduce the observed adhesion, we would have to show that the pine seed and the water films adhere to the glass. The contact angles at water–glass interface are well documented (Dettre & Johnson Citation1965), and we have for uncoated glass. The measurement of the thickness of the water films, on the other hand, is difficult during the sliding experiment, so here we calculate the maximal thickness
below which the adhesion becomes possible:
(2)
where
is the total length of the water films measured from (d) and ρw is the density of water. From Equation (2), we obtain
which is comparable to the gap width 0.3 mm between pine seed and the pine scale (). This calculation shows that the adhesion is physically possible between pine seed and the glass with a thin layer of water, even if glass exhibits less contact angle hysteresis than wood in general (Gardner et al. Citation1991).
The thin water layer between the pine seed and pine cone scale is essential for the capillary adhesion. This is demonstrated by the rather small slope angle of φs = 56.9 ± 4.5°, at which a dry pine seed would slide off. Even though there is water in the gap, if the shapes of the pine seed and pine cone scale are not well matched, it may be impossible to hold the pine seed. It is because the gravity force would be much larger if the water films become thicker for the adhesion between the water films and the glass, or the pine scale in the real situation. The small average gap of ≈300 µm between the pine seed and the pine cone scale might be deliberately maintained as a strategy to keep the pine seed from detachment in rainy days. The friction constant is defined on the ratio of the friction force between two bodies and the force pressing them together. It depends on the properties of the materials. Since the tested pine seed is slipped away at φs = 56.9 ± 4.5° under dry condition, the friction constant between seed wing and water, estimated by , is about 1.6 ± 0.3 ().
Rapid water evaporation from the wet pine seed wing for detaching
On a rainy day, the pine seed wing attaches to the scale using the attractive force of water and is not washed away by the rain; however, on a sunny and dry day, the water evaporates from the pine seed wing and the seed is released. A simple evaporation experiment was performed to access this process. The contact angle between the water droplet and the pine seed wing is approximately 63.9 ± 3.8° ((a)), and the weight of the water droplet decreases in proportion with evaporation time ((b)). The water droplet evaporation velocity is 1.4 × 10−3 ± 0.3 × 10−4 mg/s; the trend line matches well (R2 = 0.9), and the evaporation velocity rapidly increases in a dry and airy room.
Figure 3. Water evaporation from pine seed. (a) A water droplet falls down onto the surface of a pine seed wing. (b) The amount of water evaporation increases proportionally with time. (c) Symbol of ‘1st’ and ‘2nd’ and ‘CA’ indicate first evaporation experiment, second evaporation experiment and contact angle, respectively. The evaporation velocity and the contact angle of the pine seed wing are maintained even after three experiments; in contrast, the evaporation velocity and the contact angle of the maple seed rapidly decrease from the second experiment. Error bars indicate standard deviation (n = 5). (e) Wing tomogram shows that air spaces are maintained during water immersion.
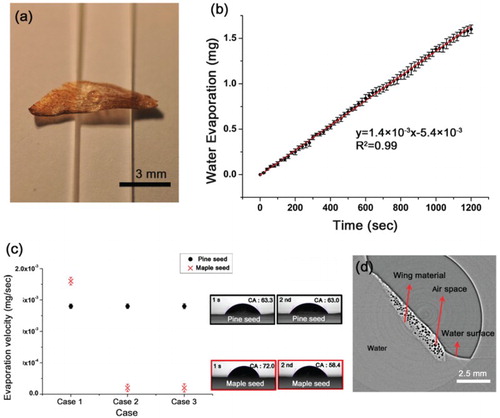
The hydrophilic and hydrophobic surface characteristics of a material are determined by the contact angle: the surface is hydrophobic when the contact angle is greater than 90° yet hydrophilic when the contact angle is less than 90° (Quéré Citation2008; Drelich et al. Citation2011). Since the pine seed wing contact angle is 63.9 ± 3.8°, the surface is hydrophilic.
When we repeated the water evaporation experiments without pausing, we observed an interesting trend. Therefore, we also tested a maple seed wing, which has a shape similar to that of a pine seed, for comparison. During the first trial, case 1, the maple seed wing showed a greater evaporation velocity compared with the pine seed (1.8 × 10−3 ±0.5 × 10−4 mg/s) ((c)); however, the evaporation velocity of the maple seed wing rapidly decreased to 0.1 × 10−3 ± 0.5 × 10−4 mg/s in the second trial, case 2. In contrast, the evaporation velocity of the pine seed wing was fairly stable. In addition, the contact angle of the maple seed wing decreases by 13.6 ± 0.7° but that of the pine seed wing decreases by 0.3 ± 0.1° in the second trial, case 2.
It occurs, because the maple seed absorbs enough water after the first experiment. Thus, the wet surface of the maple seed turns to be hydrophilic one (contact angle was decreased) and the evaporation velocity is rapidly decreased. On the other hand, the pine seed with coated surface does not absorb water and it maintains the surface characteristics including contact angle. After removing water droplet from the surface of the pine seed, the surface retains the same dry condition as the initial condition.
It indicates that the capacity for water evaporation is maintained irrespective of the frequency of rainy days. Under favorable weather conditions, water evaporates from the pine seed wing surface rapidly recovered to suitable conditions for dispersal. It is related to water proof surface structural characteristics that prevent the pine seed from wetting. Owing to the structural characteristics, the water does not invade into the pine seed: the waterproof pine seed wing does not absorb the water, thereby preserving the air spaces when the seed wing contacts water ((d)). Moreover, as this feature prevents the internal wing from becoming wet, water can rapidly evaporate from its surface.
To sum up, the pine seed has interesting features. As a result, the pine seed wing stably attaches to the scale via interaction with water; however, as the surface of the pine seed wing is waterproof, water cannot invade the seed’s internal structures, the air spaces in the pine seed wing are preserved, and the water rapidly evaporates. Most importantly, the waterproof surface protects the pine seed wing from transformation: when water invades some materials (e.g. paper), broken hydrogen bonds or varying expansion coefficients lead to structural modification such as bending and twisting. Additionally, the pine seed wing can float continuously on water for more than a month, whereas other plant part such as leaves cannot (Neinhuis & Barthlott Citation1997). It is surprising to note that floatable leaves usually sink into water within 4–6 weeks due to wax erosion. Moreover, although pine seed wings may lose their water repellence, they recover this characteristic immediately after drying.
Pine seeds extremely detach from pine cone in forest fire
There is the one condition when the pine seeds extremely detach from the pine cone. Pine seeds remain embedded in the pine cone epiphysis until dispersal ((a)). In a normal environment, the seeds are released on a clear and dry day. However, it changes a lot in the high temperature. Some studies have reported substantial seed dispersal of serotinous cones in the area surrounding huge fires (Clements Citation1910; Lotan Citation1976; Perry & Lotan Citation1977; Knapp & Anderson Citation1980). Different from other pine cones, these serotinous cones do not open scales at maturity, but remain closed until the resin bond between cone scales is melted by the high temperature (Lamont et al. Citation1991; Nathanls & Ne’eman Citation2000); since resin bonds of other pine cones are weak, their seed release is not restricted. When the environmental temperature is above 45°C, the resin binding in epiphysis of the Lodgepole pine (Pinus contorta var. latifolia Engelm.) cone scale is melted to open and release their seeds (LeMaitre Citation1984; Keeley & Zedler Citation1998).
Figure 4. Pine cone morphological changes in response to heat. (a) Pine seeds were maintained in the seed racks of pine cone that were placed between bract scale and scale. (b–e) Changes in seed rack morphology were observed using X-ray tomography. (b,d) The epiphysis of the cone scale is closed under normal conditions. (c,e) However, the gap in epiphysis of the cone scale is wide open following heating. (f,g) Cross-sectional images show that morphological changes of gap in the epiphysis of the cone scale response to heating: heating changes the epiphysis from a closed round circle to a splay shape.
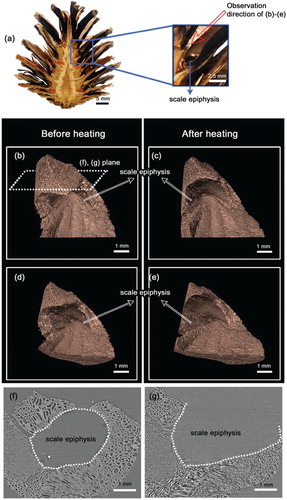
In this study, we investigated the effects of heating on the morphology of non-serotinous cones. Although the pine cones we tested were not serotinous cones but from Japanese red pine, the epiphysis of the cone scale opens wide in response to heating due to the positional change in the bract scale position ((b,c)). Consequently, the volume of the heated gap in the epiphysis of the cone scale is obviously greater than that observed under normal temperatures ((d,e)). As shown in our tomograms, the round epiphysis of the cone scale opens, and the surface area occupied by empty space in the epiphysis increases by 0.8 ± 0.3-fold ((f,g)). Due to the loosen gap in epiphysis, the seeds are easily released from the pine cone. It explains how the pine seeds easily exit the epiphysis during forest fires.
Although the non-serotinous cones from Japanese red pine exhibit opening motion in response to heating, anatomic differences between serotinous cones and non-serotinous cones affect opening mechanism under fire or dry conditions (Johnson & Gutsell Citation1993; Moya et al. Citation2008): non-serotinous cones scales are more dense and flexible than serotinous cones and it makes opening easy.
Discussion
Previous studies have primarily studied the pine cone movement and gave inspired engineering and industrial applications (Dawson et al. Citation1997; Reyssat & Mahadevan Citation2009; Song et al. Citation2015). However, as one of the primary functions of the pine cone is wide seed dispersion, this study concentrated on the effectiveness of the cone and seed structures in propagation. The trial of pine cone for dispersing the seed in an appropriate condition might lead to increase probability of species reproduction.
On a sunny day, the pine seed wing is configured to fly great distances from the parent tree: the pine seed flew 249.6 mm along the windward direction for 280 ms at a wind speed of 5 mm/ms in a wind tunnel experiment (Figure S1(a,b)). Although the seed flew far distance, the altitude only decreased by 18.7 mm; wing structure leads to long-distance gliding flight by the wind with reduced altitude descent. Pine cones fold their scales, which prevent pine seed dispersal on rainy days. And on a wet condition, interactions between water, the scale and the seed wing fix firmly in place to prevent wandering. This might be the answer to a remaining question from a previous study (Song et al. Citation2015): although the scale is primarily involved in the cone motion, the scale surface does not absorb water. If the surface of the scales absorbs water completely, the seeds would not remain attached to the scales; it needs few minutes to fully fold the scales and protect the seeds. Due to the peculiar surface characteristics of the pine seed wing and scales, the seeds are not washed away and attached to pine cone scales under a watery environment. Furthermore, this structural advantage is also helpful in promoting rapid drying, and the pine seed wings and scales detach easily after drying, without any structural damage. On the other hand, when the pine cone is exposed to wildfire, epiphysis is opened and releases its seeds for promoting propagation and conservation of the species.
Living organisms, including plants, respond to changes in their external environment; however, plant seeds remain in stasis until germination and thus cannot themselves handle and overcome difficulties. For this reason, seeds such as those of pine were designed to cope with the difficulties caused by environmental changes; it is expected that various engineering applications could mimic these types of structural advantages.
Materials and methods
X-ray tomography
The 3D morphological structures of pine seeds and cones were observed using X-ray micro-computed tomography at the Pohang Accelerator Laboratory beamline 6C. A 23-mm filter and a 1.5-mm-thick silicon wafer were positioned in the X-ray beam path to minimize photothermal damage to the test samples. The field of view was 4 mm × 3.5 mm, and the test sample and camera were 20 cm apart. The X-ray images were consecutively acquired using an sCMOS camera with 2560 × 2160 pixel resolution (Andor’s Zyla, UK). The spatial resolution was 1.6 µm/pixel, as evaluated based on the camera pixel size with a 4× objective lens. The test sample was fixed to a sample holder, and the stage was rotated between 0° and 180° at 0.5° intervals.
Erroneous spots were removed from the X-ray images, and the tomogram of each acquired image was converted into a sinogram using a modified Bronnikov algorithm filter in the Octopus imaging software (Gent, Belgium) to reconstruct the sample with improved spatial resolution. The air spaces and interior structures in the reconstructed images were then labeled and quantitatively analyzed using the Amira software (Visualization Science Group, USA).
Droplet evaporation from the seed wing surface
The evaporation experiment was performed in an air-conditioned room maintained at 21.5°C and 47.5% humidity. Pine and maple seed wings were attached to a glass, and a 0.25-µl water droplet was deposited on the seed wing surface using a pipet (Axygen, Inc., USA); their weights were monitored using an analytical balance (CAS CAUW 220D, Korea) every 10 s for 20 min of evaporation time. The amount of water evaporation was calculated by subtracting the initial weight. Sequential experiments were performed without delay after removing the droplet with Kimtech wipers.
Contact angle on the seed wing surface
The contact angles were measured in an air-conditioned room maintained at 23.0°C and 62.5% humidity. Pine and maple seed wings were attached to a glass and a 4-µl water droplet was deposited on the seed wing surface using a pipet (Axygen, Inc., USA). The contact angles were measured using a SmartDrop Lab (FemtoFab Co., Ltd, Korea). Since the wing surfaces of pine seeds were not flat, contact angles at the left and right sides were slightly different. Thus, the average value of the left and right side contact angles was used as the contact angle of the seed wing. Sequential experiments were performed without delay after removing the droplet with Kimtech wipers.
Heating on pine cone
We acquired tomograms of a pine cone under normal conditions and then wrapped the pine cone in a foil for uniform heat transfer. The wrapped pine cone was heated at 75°C for 30 min using a hot plate (Daihan Scientific Co., Ltd, MaXtir 500H, Korea). Finally, the foil was removed and X-ray tomograms of the heated pine cone were obtained.
Acknowledgments
The authors are grateful for valuable assistance with the X-ray imaging experiments performed at the 6C beamlines of the Pohang Accelerator Laboratory (Pohang, Korea).
Disclosure statement
No potential conflict of interest was reported by the authors.
Additional information
Funding
References
- Allen R, Wardrop A. 1964. The opening and shedding mechanism of the female cones of Pinus radiata. Aust J Bot. 12:125–134. doi: 10.1071/BT9640125
- Andres CM, Zhu J, Shyu T, Flynn C, Kotov NA. 2014. Shape-morphing nanocomposite origami. Langmuir. 30:5378–5385. doi: 10.1021/la404955s
- Bar-On B, Sui X, Livanov K, Achrai B, Kalfon-Cohen E, Wiesel E, Wagner HD. 2014. Structural origins of morphing in plant tissues. Appl Phys Lett. 105:033703. doi: 10.1063/1.4891191
- Benkman CW. 1995. Wind dispersal capacity of pine seeds and the evolution of different seed dispersal modes in pines. Oikos. 73:221–224. doi: 10.2307/3545911
- Clements FE. 1910. The life history of lodgepole burn forests. US Dept. of Agriculture, Forest Service.
- Cremer K. 1971. Speeds of falling & dispersal of seed of Pinus radiata and Pinus contorta. Aust For Res. 5:29–32.
- Dawson C, Vincent JFV, Rocca AM. 1997. How pine cones open. Nature. 390:668–668. doi: 10.1038/37745
- Dettre RH, Johnson Jr RE. 1965. Contact angle hysteresis. IV. Contact angle measurements on heterogeneous surfaces. J. Phys. Chem. 69:1507–1515. doi: 10.1021/j100889a012
- Drelich J, Chibowski E, Meng DD, Terpilowski K. 2011. Hydrophilic and superhydrophilic surfaces and materials. Soft Matter. 7:9804–9828. doi: 10.1039/c1sm05849e
- Fahn A, Werker E. 1972. Anatomical mechanisms of seed dispersal. New York: Academic.
- Fernando DD. 2014. The pine reproductive process in temperate and tropical regions. New Forests. 45:333–352. doi: 10.1007/s11056-013-9403-7
- Gao L, McCarthy TJ. 2006. Contact angle hysteresis explained. Langmuir. 22:6234–6237. doi: 10.1021/la060254j
- Gardner DJ, Generalla NC, Gunnells DW, Wolcott MP. 1991. Dynamic wettability of wood. Langmuir. 7:2498–2502. doi: 10.1021/la00059a017
- Gindl M, Sinn G, Gindl W, Reiterer A, Tschegg S. 2001. A comparison of different methods to calculate the surface free energy of wood using contact angle measurements. Colloids Surf A Physicochem Eng Asp. 181:279–287. doi: 10.1016/S0927-7757(00)00795-0
- Harlow WM, Cote W, Day A. 1964. The opening mechanism of pine cone scales. J For. 62:538–540.
- Joanny J, De Gennes P-G. 1984. A model for contact angle hysteresis. J Chem Phys. 81:552–562. doi: 10.1063/1.447337
- Johnson E, Gutsell S. 1993. Heat budget and fire behaviour associated with the opening of serotinous cones in two Pinus species. J Veg Sci. 4:745–750. doi: 10.2307/3235610
- Keeley JE, Zedler PH. 1998. Evolution of life histories in Pinus. Cambridge: Cambridge University Press.
- Knapp AK, Anderson JE. 1980. Effect of heat on germination of seeds from serotinous lodgepole pine cones. Am Midl Nat. 104:370–372. doi: 10.2307/2424879
- Lamont BB, Le Maitre D, Cowling R, Enright N. 1991. Canopy seed storage in woody plants. Bot Rev. 57:277–317. doi: 10.1007/BF02858770
- Lanner RM. 1985. Effectiveness of the seed wing of Pinus flexilis in wind dispersal. Gt Basin Nat. 45:318–320.
- Lanner RM. 1998. Seed dispersal in Pinus. In: Richardson DM, editor. Cambridge: Cambridge University Press.
- LeMaitre D. 1984. Current interpretations of the term serotiny. S Afr J Surg. 81:284–290.
- Lian C, Miwa M, Hogetsu T. 2001. Outcrossing and paternity analysis of Pinus densiflora (Japanese red pine) by microsatellite polymorphism. Heredity. 87:88–98. doi: 10.1046/j.1365-2540.2001.00913.x
- Lotan JE. Cone serotiny–fire relationships in lodgepole pine. Tall Timbers Fire Ecology Conference Proceedings 14, Tall Timbers Research Station & Land Conservancy, Tallahassee (FL); 1976 Jan. 1.
- Moya D, Saracino A, Salvatore R, Lovreglio R, de Las Heras J, Leone V. 2008. Anatomic basis and insulation of serotinous cones in Pinus halepensis Mill. Trees. 22:511–519. doi: 10.1007/s00468-008-0211-1
- Nathanls R, Ne’eman G. 2000. Seronity, seed dispersal and seed predation in Pinus Halepensis ecology, biogeography and management of Pinus halepensis and Pinus brutia forest ecosystems in the Mediterranean Basin.
- Neinhuis C, Barthlott W. 1997. Characterization and distribution of water-repellent, self-cleaning plant surfaces. Ann Bot. 79:667–677. doi: 10.1006/anbo.1997.0400
- Niklas KJ. 1982. Simulated and empiric wind pollination patterns of conifer ovulate cones. Proc Natl Acad Sci USA. 79:510–514. doi: 10.1073/pnas.79.2.510
- Owens JN. 2006. The reproductive biology of lodgepole pine. Forest Renewal BC.
- Owens JN, Simpson SJ, Molder M. 1981. Sexual reproduction of Pinus contorta. I. Pollen development, the pollination mechanism, and early ovule development. Can J Bot. 59:1828–1843. doi: 10.1139/b81-244
- Pallas N, Harrison Y. 1990. An automated drop shape apparatus and the surface tension of pure water. Colloids Surf. 43:169–194. doi: 10.1016/0166-6622(90)80287-E
- Perry DA, Lotan JE. 1977. Opening temperatures in serotinous cones of lodgepole pine. Dept. of Agriculture, Forest Service, Intermountain Forest and Range Experiment Station.
- Quéré D. 2008. Wetting and roughness. Annu Rev Mater Res. 38:71–99. doi: 10.1146/annurev.matsci.38.060407.132434
- Reyssat E, Mahadevan L. 2009. Hygromorphs: from pine cones to biomimetic bilayers. J R Soc Interface. 6:951–957. doi: 10.1098/rsif.2009.0184
- Sharpe DM, Fields DE. 1982. Integrating the effects of climate and seed fall velocities on seed dispersal by wind: a model and application. Ecol Modell. 17:297–310. doi: 10.1016/0304-3800(82)90036-9
- Skaar C. 1988. Wood–water relations. Berlin: Springer: 283:352–353.
- Song K, Yeom E, Seo S-J, Kim K, Kim H, Lim J-H, Lee SJ. 2015. Journey of water in pine cones. Sci Rep. 5:9963. doi: 10.1038/srep09963
- Taiz L, Zeiger E. 2010. Plant physiology. 5th ed. Sunderland (MA): Sinauer Associates, Inc.
- Vander Wall, BS. 2003. Effects of seed size of wind-dispersed pines (Pinus) on secondary seed dispersal and the caching behavior of rodents. Oikos. 100:25–34. doi: 10.1034/j.1600-0706.2003.11973.x