ABSTRACT
Two specific characteristics of stem cells, self-renewal and their differentiation capacity have made them important for vast scientific areas, particularly in the molecular and cell biology of stem cells, as well as regenerative medicine. Gene targeting plays a pivotal role in creating modified stem cells, which are considered the mainstay in regenerative medicine. The phiC31 integrase system, as a site-specific recombinase, could be a straightforward and efficient approach for this aim. According to the high potential of the application of phiC31 integrase system in stem cells, the present paper reviews previous studies, placing the focus on stem cell types and their application.
Introduction to stem cells
Stem cells are progenitor cells capable of self-renewal and differentiation into multiple different cell lineages. These cells have been used for treating a wide range of diseases (Fontes and Thomson Citation1999).When a stem cell divides, each daughter cell has the potential either to remain a stem cell or to become another type of cells with a more specialized function, such as blood, brain, heart muscle or bone cells.
Most of the advances in stem cell research have been concentrated on understanding the etiology of diseases, regenerative medicine and drug screening.
Mammalian embryonic and adult stem cells are two types of stem cells, which can be isolated from the inner cell mass of the blastocyst, and various adult tissues, respectively. Adipose tissue, bone marrow and blood are three different sources of adult tissue, which are currently used for the isolation of stem cells. Stem cells can also be harvested from umbilical cord blood, just after birth.
Overview of genome modification in stem cells
Genetic engineering techniques have been applied in stem cell genome modification by several techniques (Giudice and Trounson Citation2008; Tenzen et al. Citation2010; Fontes and Lakshmipathy Citation2013; Nowakowski et al. Citation2013; Zhou and Zeng Citation2013). These techniques are capable of modifying stem cell genome in random or site-specific manners. Random gene integration method is a popular method for random genomic integration of an exogenous gene which in several cases, provides stable gene expression. One of the main drawbacks for this strategy is the limitation in transfection efficiency, which could be overcome by using viral vectors as a vehicle in the genome modification technology (Ma, et al. Citation2003). These viral-based vectors are useful research tools for disease models and basic research studies due to their ease of use and high reprogramming efficiency. However, their appliance is limited for eventual therapeutic uses.
Non-viral-based vectors systems, such as the Sleeping Beauty, Tol2 and piggyBac have been successfully used to generate modified human stem cells (Wilber et al. Citation2007; Orban et al. Citation2009). These systems integrate the gene of interest randomly and, usually, in transcriptionally inactive regions (Huang et al. Citation2010).
Non-integrating systems, such as episomal and minicircle vectors, also provide safe engineering platforms in the vast range of studies, particularly in gene therapy (Fontes and Lakshmipathy Citation2013). On the other hand, variable expression patterns and copy number variation can be considered as disadvantages for these approaches. Zinc-finger nucleases (ZFNs), transcription activator like effector nucleases (TALENs), as well as clustered regulatory interspaced short palindromic repeat (CRISPR)/Cas-based RNA-guided are newly introduced systems which have been successfully used for gene targeting via homologous recombination technology (Nieminen et al. Citation2010; Fontes and Lakshmipathy Citation2013). Despite the widespread use of ZFNs, it is restricted due to the challenges associated with designing DNA sequences for finger design, which confer sequence specificity, therefore eliminating the target effects (Holt et al. Citation2010). The TALENs recognize DNA in a modular manner. This method holds a huge potential for the modification of induced pluripotent stem cells for various downstream applications (Hockemeyer et al. Citation2011). The CRISPR/CAS, which has superior advantages compared to the previous gene targeting systems especially in terms of specificity and efficiency, allows the simultaneous editing of multiple target loci in the mammalian genome (Wang et al. Citation2013). However, these techniques are technically challenging, expensive and may sometimes result in potential genome off-target (Gaj, et al. Citation2013).
For site-specific genomic integration; Adeno-associated virus (AAV) and phiC31 integrase systems have been used for stem cells. Several studies investigating the application of phiC31 integrase system in both human and animal models have been summarised in Tables and , respectively.
Table 1. Summary of some application of phiC31 integrase system for stem cell in human.
Table 2. Summary of some application of phiC31 integrase system for stem cell in animal models.
Introduction to phiC31 integrase system
The phage of Streptomyces soil bacteria starts its life cycle by inserting its whole genome into the host bacterial genome (Kuhstoss and Rao Citation1991; Rausch and Lehmann Citation1991). The phiC31 integrase is a member of serine-catalyzed enzymes encoded by this phage and mediates the aforementioned phage genome insertion(Smith and Thorpe Citation2002). This enzyme inserts exogenous DNA into a particular location by using the recognition of two sequences. These sites are termed attB and attP and have been found to be 34 and 39 base pairs long and naturally exist into phage and bacteria, respectively (Kuhstoss and Rao Citation1991; Rausch and Lehmann Citation1991). The phiC31 integrase-mediated recombination has been illustrated in Figure . Unlike the other site-specific integrase families, the phiC31 integrase system recombines two recognized sites in a unidirectional manner and it requires no host-specific co-factors. This feature turns phiC31 integrase into a good candidate for gene targeting and in vitro site-specific integration (Thorpe and Smith Citation1998; Ishikawa et al. Citation2006). Groth et al.(Citation2000) showed that phiC31 integrase may be able to recognize a series of sites in the mammalian genome which have a partial identity to native attB (Figure ). These sites, named pseudo-attP sites, have been found to be extended throughout the mammalian genome (Thyagarajan and Calos Citation2005; Chalberg et al. Citation2006). The location of pseudo-attP sites, place in safe positions and free of chromatin blockage, and they are transcriptionally active in the host mammalian genome (Thyagarajan et al. Citation2001) (Figure ). A more interesting issue for this system was presented when scientist showed that pseudo-attP also exist in the human genom (Groth et al. Citation2000; Thyagarajan et al. Citation2001; Bi et al. Citation2013), which opened a new window for applying this system to several areas such as production of human recombinant proteins (Thyagarajan and Calos Citation2005), gene therapy (Chalberg, et al. Citation2005; Ishikawa et al. Citation2006; Olivares et al. Citation2002; Quenneville et al. Citation2004; Keravala et al. Citation2011), cell therapy and human embryonic stem cell bioinginery (Olivares et al. Citation2002; Kong et al. Citation2009; Liu et al. Citation2009; Sivalingam et al. Citation2010). One of the main concerns of gene transfer methods in all the above mentioned areas is random ectopic gene integration (Thyagarajan and Calos Citation2005; Chalberg et al. Citation2006). Random gene integration elicits several questions concerning gene silencing, variable gene copy number per cell, unpredictable gene expression patterns or altered regulation of gene expression(Thyagarajan and Calos Citation2005; Kong et al. Citation2009). This problematic issue might be overcome appropriately by the phiC31 integrase system.
Figure 1. Schematic diagrams of phiC31 integrase-mediated recombination. (A) phiC31 integrase determines the two keys (attB and attP) in Streptomyces and phage genome recombined these two sites. Right and left recombinant sites which create by phiC31 integrase termed ‘attR’ and ‘attL’ and do not mediate enzymatic reaction by phiC31 reversibility. (B) One of the recognition sites by phiC31 could exist in mammalian which named pseudo-attP site. If the other key (attB) has been located in a construct harboring gene of interest and pseudo and attB sites exposed to phiC31 integrase, the site-specific integration has been conducted in the same manner take placed naturally.
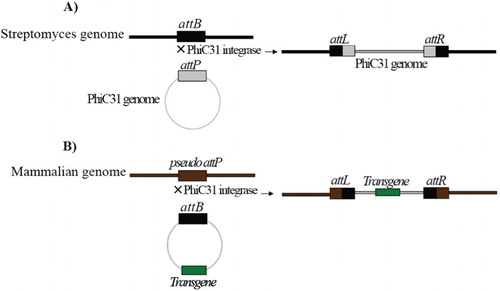
Figure 2. Sequence alignment between wild attB/attP sites with some mammalian pseudo-attP sites. Most of the matches occur within a 28-bp region, which was used to calculate a percent identity between attB/attP and mammalian pseudo-attP sites.
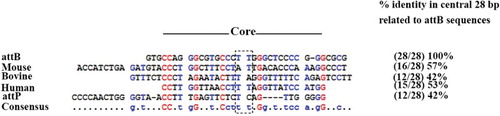
Figure 3. Schematic picture which illustrates the particular location of pseudo-attP sites in mammalian genome. As indicated by an arrow the appropriate pseudo-attP sites exist in open chromatin region in the chromosome. These sites are accessible for transcription machinery which allow over integrated gene expression.
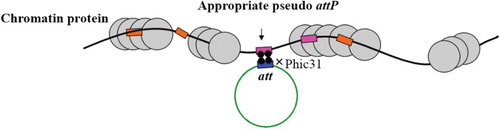
Recombinase-mediated cassette exchange by phiC31 integrase in stem cells
Recombinase-mediated cassette exchange (RMCE) is a potent method for achieving gene targeting at a single mammalian locus, which could be mediated by the phiC31 integrase system. In this method, the gene of interest (GOI) could be replaced in the donor predetermined genome sequence by flanking GOI at the two identical attB sites in the same direction (Figure ). Belteki and his colleagues used the cassette exchange approach by phiC31 integrase system for the creation of mouse embryonic stem cells. This method was successfully applied for the creation of chimeric transgenic mice with the ability to transfer ectopic transgenes to the next generation (Belteki et al. Citation2003). In addition, this method has been performed efficently for the insertion of promoter sequences at single-copy sites within defined chromosome positions in mouse embryonic stem cells (Chen et al. Citation2011). In another study, with the aim of creating mouse embryonic cell lines with endogenously controlled expression of p53 mutants, the phiC31 RMCE achieved >60% efficiency without selecting against random selection (Wei, et al. Citation2011). Recently, Zhu et al. (Citation2014) describded a novel system for precise genetic modification of human embryonic stem cells (hESCs) and induced pluripotent stem cells (iPSCs). This system, medaited by phiC31 and Bxb1 integrases, was named dual integrase cassette exchange (DICE).The authors sugested that the DICE system is rapid, efficient and the precise genome modification approches in ESC and iPSC is particulary well suited for repeated modification of the same locus (Zhu et al. Citation2014).
Genetic engineering to create animal model
There is a growing need for site-specific recombinases that function in the mammalian environment to catalyze genomic integration and excision. For example, in the development of transgenic organisms and the study of gene function in vivo, phiC31 integrase could be a straightforward and efficient approach in the field of biotechnology. In this regard, mRNA encoding phiC31 integrase could be injected into mouse embryos during the unicellular stage, leading to the integration of co-injected attB-carrying plasmid DNA into pseudo-attP sites (Hollis et al. Citation2003). The phiC31 integrase system has also been applied in primary bovine fibroblasts, with the expected application of creating transgenic cattle by somatic cell nuclear transfer (Ma et al. Citation2006). The focus of this section is to survey the application of phiC31 integrase system for the achievement of transgenic stem cells. The first study was conducted by stable integration of a reporter gene obtained using phiC31 integrase at the immunogolobulin light chain locus in chicken primordial germ cells (PGCs). Despite acommon transcriptional repression in transgenic PGCs, targeted PGCs by phiC31 integrase system showed considerable levels of expression. The results of this study indicated that phiC31 integrase could be used for the generation of transgenic chicken (Leighton et al. Citation2008). The phiC31 integrase system-based approach was applied to produce transgenic mice via a pronuclear injection, whereby an intact single-copy transgene could be inserted into predetermined chromosomal loci with high efficiency (up to 40%) and faithfully transmitted through generations (Tasic et al. Citation2011). In another study, Imayoshi and his colueages (Citation2012) generated transgenic mice that express the codon-optimized phiC31 integrase in neural stem/progenitor cells or tyrosine hydroxylase expressing catecholaminergic neurons. PhiC31 was functional in these cells and capable of excising a transcriptional stop cassette flanked by phiC31-specific attP/B recognition sites. The results of this study have shown that phiC31 integrase was functional in transgenic mice and was suitable for mosaic recombination in restricted cell populations (Imayoshi et al. Citation2012).
Cell and gene therapy
In this regards, a study was concentarated on junctional epidermolysis bullosa (JEB) patients. An attB-containing laminin 5 beta3 expression plasmid was integrated into the genomes of primary keratinocytes from genetically characterized JEB patients. PhiC31 integrase supported genomic integration into epidermal progenitor cells. Regeneration of human skin on immune deficient mice, using these cells, produced human skin tissue with restored laminin 5 expression. Furthermore, corrected JEB tissue restored hemidesmosome formation and abolished histologic evidence of subepidermal blistering. These findings provide an approach to durable non-viral correction of JEB (Ortiz-Urda et al. Citation2003). Keravala and her colleagues (Citation2005) used phiC31 integrase systems for the genomic modification of human mesenchymal stem cells (hMSCs). The hMSCs have emerged as a promising new tool in gene therapy/cell therapy strategies due to their potential use in a wide variety of applications, including both short- and long-term regeneration. For genomic integration, integrase has been utilized in different species including human keratinocytes, mouse liver, rat retina and also rabbit joints. Thier results suggested that phiC31 integrase as a non-viral method shows and effiecent approach for genetic modification of hMSCs, could be a good candidate for preclinical trials and and also clinical applications. (Keravala et al. Citation2005). Quenneville and his co-workers showed co-nucleofection of the phiC31 integrase plasmid and a large plasmid containing the attB sequence and the gene for an eGFP – full-length dystrophin fusion protein 1.
In 2012, Lan and colleagues reported for the first time the feasibility of site-specific genetic integration in human cardiac progenitor cells and human endothelial cells using the phiC31 integrase system. They found that the unique chromosomal integration site with stable expresion of the reporter gene is accompnay with no noticeable genomic instabillity or adverse changes in cell phynotype. Namely, phiC31-modified human cardiac progenitor cells were unchanged in their differentiation propensity, cellular proliferative rate, and global gene expression profile (Lan et al. Citation2012).
Evaluation of biosafety and efficacy in transgenic cells
There are several studies regarding the phiC31 integrase system safety in different cell lines. In this aspect, the first research on stem cell was conducted by Liu et al. in 2009. The goal of their research was to study the mechanisms involved in possible chromosomal abnormalities in human adult fibroblasts. They showed that the phiC31 integrase induces chromosome rearrangements in primary human adult cells (Liu et al. Citation2009). The results confirmed their previous data obtained by using primary human embryonic fibroblasts (Liu et al. Citation2006). Interestingly, they showed that the phiC31 integrase, in contrast to the SB transposase, leads to significant up-regulation of H2AX phosphorylation, and H2AX phosphorylation leads to recruitment of repair factors for the damaged DNA (Celeste et al. Citation2002). Moreover, the dosage dependent effects of integrase are still unknown and need to be further clarified.
Sivalingam et al. (Citation2010) evaluated the genotoxic potential of phiC31 bacteriophage integrase-mediated transgene integration in cord-lining epithelial cells (CLECs) readily cultured from the outer membrane of human umbilical cords. The main reason to use this cell line was the ready availability and potential of CLECs, as cellular carriers of therapeutic transgenes. The results demonstrated that phiC31 integrase induced minimal genomic and transcriptomic alterations in CLECs. Using hemophilia as a model for cell therapy revealed that phiC31 integrase-mediated integration of a human factor VIII (FVIII) transgene in CLEC induced stable FVIII secretion and corrected the phenotype of FVIII-deficient hemophilic mice (Sivalingam et al. Citation2010).
Generation of engineered and modified stem cell lines
The first study in the field of stem cells engineering was conducted by Thyagarajan et al. in 2007. The work was meant to set a foundation for a broader cell engineering effort that combines rapid assembly of complex expression vectors and their site-specific insertion into the cells. In this study, they have shown that the phage phiC31 integrase can be used to successfully target sites in the genome of hESC and lead to the generation of transgenic lines. They have also shown that integration into pseudo-attP sites leads to sustained expression of the introduced transgene without significant silencing and that these lines retain their pluripotency. Moreover, the results indicate that the profile of the pseudo-attP site in hESC is clearly distinct from that seen in differentiated cells (Thyagarajan et al. Citation2008).
Another study, which is reported by Pauline et al. in 2009, is focused on the development of cell-based assays in human embryonic stem cells using the phiC31/R4 system. They described a system that enables genetic material to be targeted at a specific and predetermined locus and demonstrated the expected protein function in three cell lines: CHO-S, HEK293, and hESC/BGO1 V. The overall process allows the generation of reproducible and high expression clones and pools in a very short time frame due to the specificity and efficiency of cell engineering and the generation of expression vectors via Multisite Gateway technology. The adoption of this technique will allow researchers to assemble multiple reporters and promoters, as well as deliver multiple genetic elements at a specific locus, thereby enhancing the process of stable cell lines development for drug screening, bio-production, and cell-based therapies (Lieu et al. Citation2009).
In 2011, Wilber and colleagues suggested phiC31 (as well as the SB transposon) as an alternative to viral methods of gene transfer into multipotent adult progenitor cells, as well as other types of stem cells. They used a plasmid-encoding GFP and neomycin phosphotransferase, along with recognition sequences for both phiC31 and SB integrating systems, to demonstrate that both systems effectively mediated integration in cultured human fibroblasts and in rat multipotent adult progenitor cells (Wilber et al. Citation2011).
Recently, a new mESC cell reporter for cell imaging has been created. This new reporter system is functional and can be studied by both fluorescence microscopy and flow cytometry (Ghorbani et al. Citation2013). Zhu et al. (Citation2014) used phiC31 and Bxb1 integrase systems spontaneously to create H9 ESC and iPSC lines derived from a Parkinson's disease patient. These cell lines successfully expressed series of the neural transcription factors LMX1a, FOXA2 and OTX2 (Zhu et al. Citation2014).
PhiC31 integrase system for reprogramming
Ye et al. (Citation2010) showed that the phage integrase system can be used to generate iPSC from mouse and human fetal somatic cells. They indicated that integrase-mediated reprogramming was similar to the piggyBac transposon method in a way that both methods yield stable transformants without viral vectors (Ye et al. Citation2010).Ye and his co-workers described a method using bacteriophage phiC31 integrase to reprogram mouse embryonic fibroblasts and human amniotic fluid cells into iPSC. These iPSCs showed all ESC features and successfully formed teratomas with three germ layers. Importantly, these iPSCs have only a single integration site in each cell line. The locations of integration favor the intergenic regions, and their distances from the adjacent genes extended from several hundred to >1 million bp (Ye et al. Citation2010).
Recently, Merkl et al. (Citation2013) reported iPSC generation using a non-viral vector containing the murine reprogramming factors Oct4, Sox2, c-Myc and Klf4, controlled by a bidirectional doxycycline-inducible promoter and equipped by the bacteriophage phiC31 attB site. A significant advance in rat iPSC technology is the efficient generation of rat iPSC using a single non-viral vector that allows tight control over reprogramming factor expression. The established iPSC lines were self-sustaining and had activated the endogenous pluripotency sequence. Methods have been developed to improve rat iPSC viability and successful generation of differentiated iPSC derivatives in vitro (Merkl et al. Citation2013).
Future perspective for application of phiC31 integrase system in stem cells biology and technology
To compare availble alternatives for genome modification in stem cells, methods relying on random integration have the serious limitation of lack of control overt the genomic target site, which leads to position effects on endogenous gene expression. The use of a homolougus recombination to control the target site will relieve these problems. This approch is safe, but relatively inefficient. Recombination stimulated by ZFNs, TALENs and (CRISPR)/Cas-based RNA-guided technology increases recombination efficiency, although off-targeting effects, introduction of mutations near the target site, as well as the required high technical support level, are several potential drawbacks of this system(Gaj et al. Citation2013). These techniques could be replaced or at least combined in many stem cell reaserch areas with the simpler, less technology demanding phiC31 integrase system.The phiC31 integrase system provides a relatively quick, simple, economical and reliable method for stem cell genome modification.
Disclosure statement
No potential conflict of interest was reported by the authors.
References
- Belteki G, Gertsenstein M, Ow DW, Nagy A. 2003. Site-specific cassette exchange and germline transmission with mouse ES cells expressing ϕC31 integrase. Nature Biotech. 21(3):321–324. doi: 10.1038/nbt787
- Bi Y, Liu X, Zhang L, Shao C, Ma Z, Hua Z, Zhang L, Li L, Hua W, Xiao H, et al. 2013. Pseudo attP sites in favor of transgene integration and expression in cultured porcine cells identified by Streptomyces phage phiC31 integrase. BMC Mol Biol. 14(1):1–20. doi: 10.1186/1471-2199-14-20
- Celeste A, Petersen S, Romanienko PJ, Fernandez-Capetillo O, Chen HT, Sedelnikova OA, Difilippantonio MJ, Redon C. 2002. Genomic instability in mice lacking histone H2AX. Science. 296(5569):922–927. doi: 10.1126/science.1069398
- Chalberg TW, Portlock JL, Olivares EC, Thyagarajan B, Kirby PJ, Hillman RT, Hoelters J, Calos MP. 2005. Φc31 integrase confers genomic integration and long-term transgene expression in rat retina. Invest Ophthalmol Vis Sci. 46(6):2140–2146. doi: 10.1167/iovs.04-1252
- Chalberg TW, Portlock JL, Olivares EC, Thyagarajan B, Kirby PJ, Hillman RT, Hoelters J, Calos MP. 2006. Integration specificity of phage φC31 integrase in the human genome. J Mol Biol. 357(1):28–48. doi: 10.1016/j.jmb.2005.11.098
- Chen C-m, Krohn J, Bhattacharya S, Davies B. 2011. A comparison of exogenous promoter activity at the ROSA26 locus using a phiC31 integrase mediated cassette exchange approach in mouse ES cells. PloS one. 6(8):1–8.
- Fontes A, Lakshmipathy U. 2013. Advances in genetic modification of pluripotent stem cells. Biotechnol Adv. 7:994–1001. doi: 10.1016/j.biotechadv.2013.07.003
- Fontes PA, Thomson AW. 1999. Stem cell technology. Br Med J. 319(7220):1308–1352. doi: 10.1136/bmj.319.7220.1308
- Gaj T, Gersbach CA, Barbas III CF. 2013. ZFN, TALEN, and CRISPR/Cas-based methods for genome engineering. Trends in Biotechnol. 7:397–405. doi: 10.1016/j.tibtech.2013.04.004
- Ghorbani R, Emamzadeh A, Khazaie Y, Dormiani K, Ghaedi K, Rabbani M, Foruzanfar M, Karbalaie K, Karamali F, Lachinani L, et al. 2013. Constructing a mouse Oct4 promoter/EGFP vector, as a whole-cellular reporter to monitor the pluripotent state of cells. Avicenna J Med Biotechnol. 5(1):2–9.
- Giudice A, Trounson A. 2008. Genetic modification of human embryonic stem cells for derivation of target cells. Cell Stem Cell. 2(5):422–433. doi: 10.1016/j.stem.2008.04.003
- Groth, AC, Olivares, EC, Thyagarajan, B, Calos, MP. (2000). A phage integrase directs efficient site-specific integration in human cells. Proc Natl Acad Sci USA,97(11), 5995–6000. doi: 10.1073/pnas.090527097
- Hockemeyer D, Wang H, Kiani S, Lai CS, Gao Q, Cassady JP, Cost GJ, Zhang L, Santiago Y, Miller JC, et al. 2011. Genetic engineering of human pluripotent cells using TALE nucleases. Nature Biotechnology. 29(8):731–734. doi: 10.1038/nbt.1927
- Hollis RP, Stoll SM, Sclimenti CR, Lin J, Chen-Tsai Y, Calos MP. 2003. Phage integrases for the construction and manipulation of transgenic mammals. Reprod Biol Endocrinol. 1(1):79–84. doi: 10.1186/1477-7827-1-79
- Holt N, Wang J, Kim K, Friedman G, Wang X, Taupin V, Crooks GM, Kohn DB, Gregory PD, Holmes MC, et al. 2010. Human hematopoietic stem/ progenitor cells modified by zinc-finger nucleases targeted to CCR5 control HIV-1 in vivo. Nature Biotechnol. 28(8):839–847. doi: 10.1038/nbt.1663
- Huang X, Guo H, Tammana S, Jung Y-C, Mellgren E, Bassi P, Cao Q, Tu ZJ, Kim YC, Ekker SC, et al. 2010. Gene transfer efficiency and genome-wide integration profiling of Sleeping Beauty, Tol2, and piggyBac transposons in human primary T cells. Mol Ther. 18(10):1803–1813. doi: 10.1038/mt.2010.141
- Imayoshi I, Hirano K, Kitano S, Miyachi H, Kageyama R. 2012. In vivo evaluation of phiC31 recombinase activity in transgenic mice. Neurosci Res. 73(2):106–114. doi: 10.1016/j.neures.2012.02.008
- Ishikawa Y, Tanaka N, Murakami K, Uchiyama T, Kumaki S, Tsuchiya S, Kugoh H, Oshimura M, Calos MP, Sugamura K. 2006. Phage ϕC31 integrase-mediated genomic integration of the common cytokine receptor gamma chain in human T-cell lines. J Gene Med. 8(5):646–653. doi: 10.1002/jgm.891
- Keravala A, Chavez C, Hu G, Woodard L, Monahan P, Calos M. 2011. Long-term phenotypic correction in factor IX knockout mice by using phiC31 integrase-mediated gene therapy. Gene Ther. 18(8):842–848. doi: 10.1038/gt.2011.31
- Keravala A, Hoelters J, Jarrahian S, Chalberg TW, Ciccarella M, Neth P, Calos MP. 2005. 1103. Site-specific chromosomal integration in human mesenchymal stem cells mediated by phiC31 integrase. Mol Ther. 11(S1):S425–S425.
- Keravala A, Ormerod BK, Palmer TD, Calos MP. 2008. Long-term transgene expression in mouse neural progenitor cells modified with φC31 integrase. J Neurosci Methods. 173(2):299–305. doi: 10.1016/j.jneumeth.2008.06.005
- Kong Q, Wu M, Huan Y, Zhang L, Liu H, Bou G, Liu Z, Mu Y, Liu Z. 2009. Transgene expression is associated with copy number and cytomegalovirus promoter methylation in transgenic pigs. PloS one. 4(8):1–10. doi: 10.1371/journal.pone.0006679
- Kuhstoss S, Rao RN. 1991. Analysis of the integration function of the streptomycete bacteriophage ϕC31. J Mol Biol. 222(4):897–908. doi: 10.1016/0022-2836(91)90584-S
- Lan F, Liu J, Narsinh KH, Hu S, Han L, Lee AS, Karow M, Nguyen PK, Nag D, Calos MP. 2012. Safe genetic modification of cardiac stem cells using a site-specific integration technique. Circulation. 126(11 Suppl. 1):S20–S28. doi: 10.1161/CIRCULATIONAHA.111.084913
- Leighton PA, van de Lavoir MC, Diamond JH, Xia C, Etches RJ. 2008. Genetic modification of primordial germ cells by gene trapping, gene targeting, and ϕC31 integrase. Mol Reprod Dev. 75(7):1163–1175. doi: 10.1002/mrd.20859
- Lieu PT, Machleidt T, Thyagarajan B, Fontes A, Frey E, Fuerstenau-Sharp M, Thompson DV, Swamilingiah GM, Derebail SS, Piper D, et al. 2009. Generation of site-specific retargeting platform cell lines for drug discovery using phiC31 and R4 integrases. J Biomol Screening. 14(10):1207–1215. doi: 10.1177/1087057109348941
- Liu J, Jeppesen I, Nielsen K, Jensen T. 2006. Φc31 integrase induces chromosomal aberrations in primary human fibroblasts. Gene Ther. 13(15):1188–1190. doi: 10.1038/sj.gt.3302789
- Liu J, Skjørringe T, Gjetting T, Jensen TG. 2009. PhiC31 integrase induces a DNA damage response and chromosomal rearrangements in human adult fibroblasts. BMC Biotechnol. 9(1):1–8. doi: 10.1186/1472-6750-9-31
- Ma Y, Ramezani A, Lewis R, Hawley RG, Thomson JA. 2003. High-level sustained transgene expression in human embryonic stem cells using lentiviral vectors. Stem Cells. 21(1):111–117. doi: 10.1634/stemcells.21-1-111
- Ma Q-w, Sheng H-q, Yan J-b, Cheng S, Huang Y, Chen-Tsai Y, Ren ZR, Huang SZ, Zeng YT. 2006. Identification of pseudo attP sites for phage φC31 integrase in bovine genome. Biochem Biophys Res Commun. 345(3):984–988. doi: 10.1016/j.bbrc.2006.04.145
- Merkl C, Saalfrank A, Riesen N, Kühn R, Pertek A, Eser S, Hardt MS, Kind A, Saur D, Wurst W. 2013. Efficient generation of rat induced pluripotent stem cells using a non-viral inducible vector. PloS one. 8(1):1–13. doi: 10.1371/journal.pone.0055170
- Nieminen M, Tuuri T, Savilahti H. 2010. Genetic recombination pathways and their application for genome modification of human embryonic stem cells. Exp Cell Res. 316(16):2578–2586. doi: 10.1016/j.yexcr.2010.06.004
- Nowakowski A, Andrzejewska A, Janowski M, Walczak P, Lukomska B. 2013. Genetic engineering of stem cells for enhanced therapy. Acta Neurobiol Exp. 73:1–18.
- Olivares EC, Hollis RP, Chalberg TW, Meuse L, Kay MA, Calos MP. 2002. Site-specific genomic integration produces therapeutic factor IX levels in mice. Nature Biotechnol. 20(11):1124–1128. doi: 10.1038/nbt753
- Orban TI, Apati A, Nemeth A, Varga N, Krizsik V, Schamberger A, Szebenyi K, Erdei Z, Várady G, Karaszi E, et al. 2009. Applying a “double-feature” promoter to identify cardiomyocytes differentiated from human embryonic stem cells following transposon-based gene delivery. Stem Cells. 27(5):1077–1087. doi: 10.1002/stem.45
- Ortiz-Urda S, Thyagarajan B, Keene DR, Lin Q, Calos MP, Khavari PA. 2003. Φc31 integrase-mediated nonviral genetic correction of junctional epidermolysis bullosa. Human Gene Therapy. 14(9):923–928. doi: 10.1089/104303403765701204
- Quenneville SP, Chapdelaine P, Rousseau J, Beaulieu J, Caron NJ, Skuk D, Mills P, Olivares ES, Calos PM, Tremblay JP. 2004. Nucleofection of muscle-derived stem cells and myoblasts with φC31 integrase: stable expression of a full-length-dystrophin fusion gene by human myoblasts. Mol Ther. 10(4):679–687. doi: 10.1016/j.ymthe.2004.05.034
- Quenneville SP, Chapdelaine P, Rousseau J, Tremblay JP. 2007. Dystrophin expression in host muscle following transplantation of muscle precursor cells modified with the phiC31 integrase. Gene Ther. 14(6):514–522. doi: 10.1038/sj.gt.3302887
- Rausch H, Lehmann M. 1991. Structural analysis of the actinophae ФC31 attachment site. Nucleic Acids Research. 19(19):5187–5189. doi: 10.1093/nar/19.19.5187
- Sivalingam J, Krishnan S, Ng WH, Lee SS, Phan TT, Kon OL. 2010. Biosafety assessment of site-directed transgene integration in human umbilical cord–lining cells. Mol Ther. 18(7):1346–1356. doi: 10.1038/mt.2010.61
- Smith M, Thorpe HM. 2002. Diversity in the serine recombinases. Mol Microbiol. 44(2):299–307. doi: 10.1046/j.1365-2958.2002.02891.x
- Tasic, B, Hippenmeyer, S, Wang, C, Gamboa, M, Zong, H, Chen-Tsai, Y, Luo, L. (2011). Site-specific integrase-mediated transgenesis in mice via pronuclear injection. Proc Natl Acad Sci USA, 108(19), 7902–7907. doi: 10.1073/pnas.1019507108
- Tenzen T, Zembowicz F, Cowan CA. 2010. Genome modification in human embryonic stem cells. J Cell Physiol. 222(2):278–281. doi: 10.1002/jcp.21948
- Thorpe HM, Smith MC. 1998. In vitro site-specific integration of bacteriophage DNA catalyzed by a recombinase of the resolvase/invertase family. Proc Natl Acad Sci USA. 95(10):5505–5510. doi: 10.1073/pnas.95.10.5505
- Thyagarajan B, Calos MP. 2005. Site-specific integration for high-level protein production in mammalian cells therapeutic proteins. California: Springer; p. 99–106.
- Thyagarajan B, Liu Y, Shin S, Lakshmipathy U, Scheyhing K, Xue H, Ellerström C, Strehl R, Hyllner J, Rao MS, et al. 2008. Creation of engineered human embryonic stem cell lines using phiC31 integrase. Stem Cells. 26(1):119–126. doi: 10.1634/stemcells.2007-0283
- Thyagarajan B, Olivares EC, Hollis RP, Ginsburg DS, Calos MP. 2001. Site-Specific Genomic Integration in Mammalian Cells Mediated by Phage φC31 Integrase. Mol Cell Biol. 21(12):3926–3934. doi: 10.1128/MCB.21.12.3926-3934.2001
- Wang H, Yang H, Shivalila CS, Dawlaty MM, Cheng AW, Zhang F, Jaenisch R. 2013. One-step generation of mice carrying mutations in multiple genes by CRISPR/Cas-mediated genome engineering. Cell. 4:910–918. doi: 10.1016/j.cell.2013.04.025
- Wei Q-X, Odell AF, van der Hoeven F, Hollstein M. 2011. Rapid derivation of genetically related mutants from embryonic cells harboring a recombinase-specific Trp53 platform. Cell Cycle. 10(8):1261–1270. doi: 10.4161/cc.10.8.15303
- Wilber A, Linehan JL, Tian X, Woll PS, Morris JK, Belur LR, McIvor RS, Kaufman DS. 2007. Efficient and stable transgene expression in human embryonic stem cells using transposon-mediated gene transfer. Stem Cells. 25(11):2919–2927. doi: 10.1634/stemcells.2007-0026
- Wilber A, Ulloa Montoya F, Hammer L, Moriarity BS, Geurts AM, Largaespada DA, Verfaillie CM, McIvor RS, Lakshmipathy U. 2011. Efficient non-viral integration and stable gene expression in multipotent adult progenitor cells. Stem Cells International. 1:1–14. doi: 10.4061/2011/717069
- Ye, L, Chang, JC, Lin, C, Qi, Z, Yu, J, Kan, YW. (2010). Generation of induced pluripotent stem cells using site-specific integration with phage integrase. Proc Natl Acad Sci USA,107(45), 19467–19472. doi: 10.1073/pnas.1012677107
- Zhou Y-y, Zeng F. 2013. Integration-free methods for generating induced pluripotent stem cells. Genomics, Proteomics & Bioinformatics. 11(5):284–287. doi: 10.1016/j.gpb.2013.09.008
- Zhu F, Gamboa M, Farruggio AP, Hippenmeyer S, Tasic B, Schüle B, Chen-Tsai Y, Calos MP. 2014. DICE, an efficient system for iterative genomic editing in human pluripotent stem cells. Nucleic Acids Res. 42:1–13. doi: 10.1093/nar/gku978.