ABSTRACT
Salmonella is the leading cause of typhoidal and non-typhoidal infections in the world. The entry of Salmonella into the bloodstream causes an invasive disease state, resulting to high morbidity and mortality rates, especially in children. Owing to the misuse of antibiotics, certain Salmonella serovars are multi-drug resistant and do not respond to traditional antibiotics, such as ampicillin and trimethoprim-sulphamethoxazole, presenting a significant challenge for healthcare practitioners in treating and controlling the spread of this disease. Therefore, expensive third-generation cephalosporins, such as ceftriaxone, are currently used to treat Salmonella infection. However, a novel serovar of Salmonella that resists ceftriaxone was recently identified in Saudi Arabia, indicating wide spread Salmonella resistance. A comprehensive literature review on ceftriaxone resistance in Salmonella is therefore necessary to reflect upon current challenges. In this report, we provide a summary of Salmonella incidence, mechanisms of ceftriaxone resistance in Salmonella, and current treatment options.
Introduction
Infectious diseases remain a major cause of morbidity and mortality. The rising numbers of pathogens, particularly Salmonella that have developed resistance to a wide range of treatment options have been widely studied (Davies and Davies Citation2010). Salmonella infections are becoming difficult to treat and manage due to the development of multi-drug resistance.
Salmonella is a gram-negative bacterium that affects warm-blooded animals worldwide (Velge et al. Citation2012). There are approximately 2,600 different serovars of Salmonella categorized as either typhoidal or non-typhoidal. Salmonella enterica serovar Typhi or Salmonella enterica serovar Paratyphi A, B, and C cause typhoid and paratyphoid fever, whereas, Salmonella serovars Typhimurium and Enteritidis cause non-typhoidal fever with symptoms of self-limiting gastroenteritis (Alexander et al. Citation2009; Velge et al. Citation2012; Eng et al. Citation2015; Smith et al. Citation2016). Typhoidal fever is endemic in the developing world and non-typhoidal disease is present worldwide. Infants and young children are more susceptible to Salmonella infections than adults (Eng et al. Citation2015; Smith et al. Citation2016).
Salmonella infection is usually caused by the ingestion of contaminated water or food. Once bacteria pass from the stomach to the intestines, they enter the cells lining the intestinal epithelium and migrate to the intestinal spaces of the lamina propria. Non-invasive Salmonella cause self-limiting acute gastroenteritis (Chen et al. Citation2013). Some Salmonella serovars are up taken by different phagocytes and rapidly spread to lymph nodes and move through the blood stream to the spleen and liver (Figure ). Several factors facilitate the transmission of some Salmonella serotypes from the intestines to the blood stream (Velge et al. Citation2012).
Figure 1. Fates of Salmonella infection inside the host. After ingestion, Salmonella passes to the stomach and intestinal spaces of the lamina propria through cells lining the intestinal epithelium. Different serovars spread to lymph nodes by phagocytes and through the blood stream to the liver and spleen.
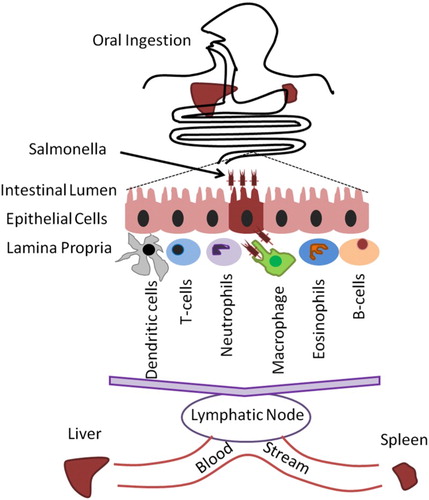
Salmonella enters the host's phagocytic and non-phagocytic cells by interacting with the receptors on host cell membranes using different mechanisms (additional details about the mechanisms concerning Salmonella entry into the host cell are described in other studies) (Valdez et al. Citation2009; Velge et al. Citation2012; Wiedemann et al. Citation2014). Once inside the cell, bacteria override existing signaling pathways to alter gene expression and protein function to facilitate the invasion of epithelial cells and the survival and replication of Salmonella inside host cells. Salmonella might be protected from antibiotics, such as gentamycin, which cannot penetrate host cells. Therefore, Salmonella infection poses a significant burden on the healthcare system worldwide. It has been difficult to circumvent the spread of Salmonella since it is highly adaptable, has a high tolerance to environmental stress, is distributed widely, and has multi-drug resistance (Chen et al. Citation2013). In a recent study (under publication), we verified that ceftriaxone resistant was presented by a novel Salmonella serotype. This study provides strong evidence that ceftriaxone resistance is expressed in different clinical Salmonella isolates (15.3%) from Indian labor in Saudi Arabia.
In this review, we provide an overview of the mechanism of Salmonella treatment by ceftriaxone and shed light on the mechanism by which Salmonella may develop resistance against ceftriaxone. Furthermore, we present the challenges of ceftriaxone resistance in Salmonella with other treatment options.
Incidence of Salmonella
Salmonella infections are caused by both typhoidal and non-typhoidal serovars, which are one of the leading causes of morbidity and mortality around the world (Kariuki, Gordon, et al. Citation2015). Globally, in 2010 there were approximately 100 million cases of gastroenteritis due to Salmonella infection with 155,000 deaths (Figure (A)) (Majowicz et al. Citation2010). In addition, there were approximately 28 million illnesses and over 200,000 deaths caused by typhoidal Salmonella (Figure (B)) (Crump et al. Citation2004; Kariuki, Gordon, et al. Citation2015).
Figure 2. The global burden of Salmonella deaths and illnesess by region. A. Africa has the highest incidence of non-typhoidal death, while the typhoidal and paratyphoidal deaths are highest in the eastern Mediterranean region. The Southeast Asia has the highest rates of Salmonella illness (B). AFR: African Region; AMR: America Region; EMR: Eastern Mediterranean Region; EUR: European Region; SEAR: Southeast Asia Region; WPR: Western Pacific Region.
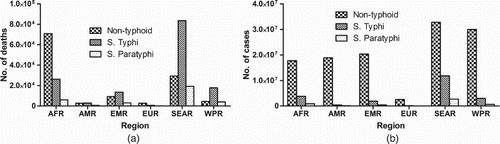
Africa has the highest incidence rate of non-typhoidal Salmonella infections, and a troubling link between invasive non-typhoidal Salmonella disease, malaria and HIV has emerged (Smith et al. Citation2016). In 2010, Africa had approximately 2 million cases of invasive non-typhoidal Salmonella infections, with the highest incidence in children and young adults, which resulted in approximately 600,000 deaths (Feasey et al. Citation2012; Ao et al. Citation2015). High rates of infection and death in both children and adults are partly due to co-infection with HIV or malaria (Takem et al. Citation2014; Ao et al. Citation2015; Oneko et al. Citation2015). Accordingly, a reduction in malaria infections has led in a reduction in invasive non-typhoidal infections; a similar trend is expected in the HIV population as well (Mtove et al. Citation2011; Ao et al. Citation2015). In North America each year, there are approximately 1.4 million cases of invasive non-typhoidal Salmonella infection; approximately 22% of these cases require medical attention (Voetsch et al. Citation2004).
Different factors facilitate the spread of salmonella illness in Eastern Mediterranean Region (EMR). However, multidrug-resistance to Salmonella is the main factor that responsible for an increase in human salmonellosis in EMR. Increased poultry production, active international livestock trade, weak surveillance and investigation of Salmonella outbreaks, and lack of restricted roles concerning food handling and processing also contribute to the rising incidence (Eng et al. Citation2015).
The most important factors of invasive typhoidal and non-typhoidal Salmonella infections include traditional first-line drugs (ampicillin, trimethoprim-sulfamethoxazole, and chloramphenicol) and adaptation to immune-suppressed patients, especially those with HIV (Ao et al. Citation2015). These factors are of global concerns due to the decreased susceptibility of Salmonella to other types of antibiotics, such as ciprofloxacin and ceftriaxone. In fact, The National Antimicrobial Resistance Monitoring System NARMS and Centers for Disease Control and Prevention (CDC) have monitored the resistance of Salmonella from humans, animals, and food products since 1996 (Crump et al. Citation2011; CDC Citation2013). The World Health Organization (WHO) also established a specific project in 2000, termed the WHO Global Salm-Surv (WHO GSS) program, to perform global surveillance and study Salmonella antimicrobial resistance (Hendriksen et al. Citation2009).
The CDC reported that non-typhoidal Salmonella (NTS) resistance and extended-spectrum cephalosporins (ESCs) were 0.1% in 1996, 0.4% in 1997, and 0.5% in 1998 (Dunne et al. Citation2000; Yates and Amyes Citation2005; CDC Citation2013). However, NARMS indicated that NTS ceftriaxone susceptibility rates were 4.4% in 2002 and 2003, 3.3% in 2004 and 2007, and 2.9% in 2005 and 2010. Other studies have reported outbreaks, in a very wide range from 1998 to 2008, caused by the most abundant NTS serovars due to consumption of diverse food commodities, such as chicken, eggs, beef, fruit, and vegetables (Jackson et al. Citation2013). Therefore, it is necessary to understand the mechanism behind the ceftriaxone action and how Salmonella resists to develop a rapid and accurate therapeutic treatment against Salmonella.
Mechanism of treatment of Salmonella by ceftriaxone
β-Lactam antibiotics for Salmonella infection treatment are used to target bacterial cell walls and inhibit its activity (Wise and Park Citation1965). In response to drugs, synthesizing of the β-lactamase enzyme by bacteria leads to β-lactams resistance. This enzyme cleaves the β-lactam ring and inhibits activity of the antibiotic. The third-generation cephalosporin, ceftriaxone, has been extensively used for the treatment of invasive Salmonella infection (Al-Mashhadani et al. Citation2011).
Ceftriaxone is a cephalosporin that has a 72%–97% cure rate, and no relapses are observed with treatment over 8–14 days (Butler Citation2011). Ceftriaxone is highly potent against a wide range of gram-negative and gram-positive bacteria and has a mechanism of action similar to other beta-lactam antibiotics and acts by inhibiting the peptidoglycan layer of the bacterial cell wall (Hall et al. Citation1981) (Figure ). It contains a beta-lactam ring that mimics the structure of the D-alanyl-D-alanine amino acid used to build peptidoglycan. The cross-linking of peptidoglycan polymers to construct the bacterial cell wall is catalyzed by transpeptidases, penicillin-binding proteins. Due to the structural similarity of ceftriaxone with D-alanyl-D-alanine, transpeptidases irreversibly bind to ceftriaxone and neutralizes their activity. Since transpeptidases are irreversibly inhibited, the final cross-linking of peptidoglycan cannot take place and the bacterial cell wall collapses, leading to bacterial cell lysis (Barriere and Flaherty Citation1984). The pharmacokinetic profile of ceftriaxone has a long elimination half-life of 5.8–8.7 h; this long half-life allows for once-daily dosing. Ceftriaxone is rapidly and completely absorbed and penetrates most body tissues and fluids (Barriere and Flaherty Citation1984; Patel and Kaplan Citation1984).
Figure 3. Overview of ceftriaxone mechanism.The mechanism of action of ceftriaxone is similar to that of other beta-lactam antibiotics. It inhibits the peptidoglycan layer of the bacterial cell wall catalyzed by transpeptidases. D-alanyl-D-alanine is structurally similar to ceftriaxone; however, transpeptidases irreversibly bind to ceftriaxone. Therefore, the final cross-linking of peptidoglycan is blocked, which collapses the bacterial cell wall leading to eventual bacterial cell lysis.
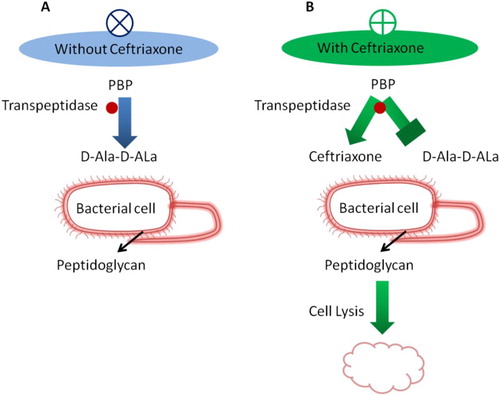
Mechanism behind the development of Salmonella resistance
Salmonella can become resistant to cephalosporins by overproducing cephalosporinases, enzymes that degrade cephalosporins. The majority of these cephalosporinases are extended-spectrum β-lactamases (ESBLs) and their genes are located on conjugative plasmids, either on transposons or integrins (Su et al. Citation2004; Michael et al. Citation2006; Chen et al. Citation2013) (Figure ). Since ESBLs are located on mobile genetic elements that can spread horizontally between bacteria by obtaining ESBL genes from resistance bacteria, sensitive bacteria can acquire resistance to cephalosporins (Figure ). In addition, the use of continuous antibiotics for therapeutic or preventative measures in farm animals plays an important role in the development of higher resistance rates (Li et al. Citation2007). Using genotyping and plasmid analysis, researchers have determined that both clonal dissemination and horizontal transfer of a resistance gene blaCMY-2 contribute to the cephalosporin resistance of Salmonella enterica strains (Su et al. Citation2003; Yan et al. Citation2003; Li et al. Citation2007). Other genes like blaCTX-M-15, blaCTX-M-2, blaOXA-1, and blaTEM-1confer resistance to beta-lactams, often found on a novel plasmid pKST313 that carried class 1 integrons (Kariuki, Okoro, et al. Citation2015; Olaitan et al. Citation2015).
Figure 4. Overview of the mechanism of Salmonella resistance. Generations of different types of hybrid virulence plasmids associated with antibiotic resistance genes are responsible for Salmonella survival, even under unfavorable drug environments.
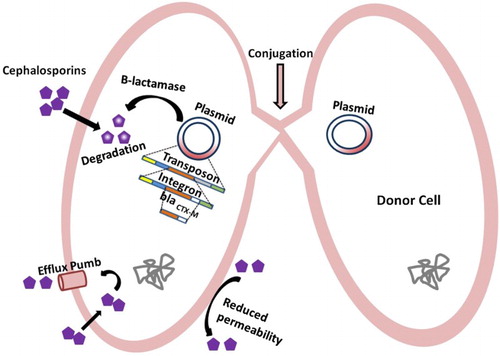
To study the effect of ceftriaxone treatment on Salmonella strains containing the blaCMY-2 gene, researchers treated seven Salmonella enterica strains with ceftriaxone using a protocol that simulated patient treatment. Following treatment, all seven strains showed less sensitivity to ceftriaxone treatment; in addition, they had increased expression of antimicrobial resistance genes, blaCMY-2 and floR (Hamilton et al. Citation2012). Several evidences suggest that non-typhoidal Salmonella strains S. Choleraesuis and S. Typhimurium generate different types of hybrid virulence plasmids containing antibiotic resistance genes, increasing their ability to survive under unfavorable drug environments. The ability of Salmonella to integrate new resistance genes in its virulence plasmid poses a serious threat to public health.
To better understand the mechanism behind ceftriaxone resistance in Salmonella, researchers subjected increasing concentrations of ceftriaxone in nine clinical ceftriaxone-susceptible S. Typhimurium to create ceftriaxone-resistant strains to stepwise selection. They identified a silent mutation in the acrR gene that encodes for a repressor for the efflux pump; however, there were no mutations in the virulence-associated genes. Furthermore, an increase in the expression of the AcrAB-ToiC efflux pump was detected (Yang WC et al. Citation2016). In another study, the acquisition of ceftriaxone resistance was explored by isolating Salmonella enterica serotype Anatum and Escherichia coli from diabetic patients. Both bacteria were susceptible to ceftriaxone at first isolation; however, they developed resistance to ceftriaxone after two weeks of ceftriaxone treatment due to the in vivo acquisition of a plasmid containing the blaCTX-M-3 gene (Su et al. Citation2003).
Ceftriaxone resistance in Salmonella
Emerging ceftriaxone resistance in typhoidal and non-typhoidal diseases is an alarming and challenging concern for health practitioners (Salmon-Rousseau et al. Citation2016). Generally, most reported cases of resistance are due to Salmonella Typhimurium, Salmonella Enteritidis, and Salmonella Newport and result in non-typhoid fever. These serovars are the most prevalent serotypes in many countries, are spread worldwide, and are associated with resistance in both humans and animals (Arlet et al. Citation2006; Eng et al. Citation2015).
The first ceftriaxone-resistant Salmonella infection was acquired from cattle in a 12-year-old boy in the USA (Fey et al. Citation2000). Since then, several cases of ceftriaxone resistance have emerged worldwide. A 5-year study from 2005 to 2009 in Slovakia identified 10 ceftriaxone-resistant isolates among 858 total isolates (Majtan et al. Citation2010). A study of 174 patients conducted in India showed that 8% of the S. Typhi isolates were beta-lactamase producers and 12% were resistant to ceftriaxone (Kumar et al. Citation2008). In a study from Taiwan, Salmonella enterica serotype Oranienburg developed resistance against ceftriaxone during treatment (Yang WC et al. Citation2016). While the rate of efficacy of ceftriaxone is very high against Salmonella infections, new cases of Salmonella serovars resistance are emerging worldwide, with over 43 countries reporting cases; several cases of ceftriaxone resistance have been reported in the USA, France, Spain, Italy, Turkey, Kenya, Western Kenya, Saudi Arabia, the Middle East and Central Asia, Pakistan, Bangladesh, India, Taiwan,and China (Yildirmak et al. Citation1998; Saha et al. Citation1999; Nastasi et al. Citation2000; Cruchaga et al. Citation2001; Yan et al. Citation2002, Citation2003; Egorova et al. Citation2008; Crump et al. Citation2011; Zaki and Karande Citation2011; Medalla et al. Citation2013; Wong et al. Citation2013; Zowawi et al. Citation2013; Krueger et al. Citation2014; Qamar et al. Citation2014; Rahman et al. Citation2014; Yang B et al. Citation2014; Kariuki, Okoro, et al. Citation2015; Olaitan et al. Citation2015; Oneko et al. Citation2015). The rates of resistance in Salmonella to ceftriaxone are shown in Table .
Table 1. Rates of resistance to ceftriaxone.
In regions with a large immigrant population, such as the Gulf Cooperation Council (GCC), USA, and UK, most ceftriaxone-resistant strains are imported from regions with a high prevalence, such as Africa, India, Pakistan, Bangladesh, and, particularly, southeast Asia (Saha et al. Citation1999; Rotimi et al. Citation2008; Kumarasamy et al. Citation2010; Van et al. Citation2012; Zowawi et al. Citation2013). Therefore, global transportation has played an important role in the spread of antimicrobial resistance when people travel from regions where the risk for the acquisition and dissemination of infectious diseases is high to regions with lower levels of infectious diseases (van der Bij and Pitout Citation2012). The Hajj pilgrimage in Saudi Arabia gathers 2–3 million people from over 180 countries annually, with documented cases of outbreaks of several infectious diseases (Olaitan et al. Citation2015). A number of respiratory tract infectious diseases, such as MERS CoV, whooping cough, and pyogenic pneumonia, have been reported, in addition to common outbreaks of waterborne infections, including gastroenteritis (Salmon-Rousseau et al. Citation2016).
Furthermore, some Salmonella serotypes that are resistant to a third-generation antibiotic ceftriaxone have also been discovered in pilgrims from Hajj (Olaitan et al. Citation2015). In fact, these travelers act as carriers for Salmonella serovars resistant to ceftriaxone and then spread these serovars across different regions of the world. In a recent study, 102 non-typhoidal Salmonella serotypes (B or D) were isolated from 1,696 children. Resistance to ceftriaxone emerged in 6.2% in 2009 and 2010; however, the rate of resistance increased to 56.5% in 2012 and 2013 (Oneko et al. Citation2015). Therefore, the spread of antimicrobial-resistant is a critical issue that must be addressed to implement effective public health interventions.
These resistance cases were associated with increased risk of invasive disease, longer hospital stay, and increased challenges in treatment, suggesting arise of Salmonella serovars that could be resistant to all available antibiotics; it is also an inevitable side effect of the use of antibiotics, since there is an arms race between the adaptability of bacteria and the introduction of new antibiotics (Fullybright Citation2016). Furthermore, prolonged therapeutic use, improper dispensing, and misusing antibiotics in livestock have been major factors in the emergence of antibiotic resistance bacteria.
Other treatment options
Historically, chloramphenicol, ampicillin, and trimethoprim-sulphamethoxazole were prescribed to patients to treat Salmonella infections; however, they are no longer used due to the emergence of plasmid-mediated resistance in Salmonella serotype Typhimurium or Newport (Su et al. Citation2004; Butler Citation2011). Since the 1980s, fluoroquinolones and cephalosporins have been the drugs of choice due to their efficacy against the multi-drug resistant strains of Salmonella. Ciprofloxacin, ofloxacin, and gatifloxacin are fluoroquinolones that were prescribed more often than cephalosporins due to their oral use and low cost. However, in the last decade, new strains of Salmonella have developed resistant to fluoroquinolones (Kariuki, Gordon, et al. Citation2015; Pham et al. Citation2016).
Several strains that developed resistance to ceftriaxone treatments are also showing resistance to other antibiotic treatments. For example, nine isolates of different S. Typhimurium are resistant to ceftriaxone; they are also resistant to ampicillin, chloramphenicol, cefuroxime, aztreonam, cefepime, sulfamethoxazole-trimethoprim, and cefpodoxime (Kariuki, Okoro, et al. Citation2015). Moreover, several cases of resistance against nalidixic acid have been reported, with resistance rates of 11% (Majtan et al. Citation2010; Krueger et al. Citation2014). The more recent drug for the treatment of Salmonella infections is azithromycin, which has a cure rate of 81%–100% and is a promising alternative to ciprofloxacin and ceftriaxone (Butler Citation2011; John Citation2011). Resistance rates are the lowest for azithromycin since several cases have begun to emerge of Salmonella serovars (Wong et al. Citation2013; Wong et al. Citation2014; Lin et al. Citation2015)
Future outlook
Antimicrobial resistance to Salmonella strains represents a huge burden on the health system worldwide, specifically in underdeveloped countries. While targets for non-typhoidal and typhoidal Salmonella have been identified, the detection process is not sensitive enough to identify small amounts of bacteria in the blood (Feasey et al. Citation2012). A promising method proposes to identify the chromosomal oriC locus common to all Salmonella enterica subspecies enterica serovars (Tennant, Zhang, et al. Citation2011).
A potential approach to target Salmonella is the development of a vaccine against typhoidal and non-typhoidal Salmonella strains, which worked well for cholera by using inactivated whole bacterial cells (Lebens et al. Citation2011). For typhoid fever, three different vaccines are currently recommended for travelers to endemic areas as follows: the Vi polysaccharide capsule-based vaccine, the live attenuated oral Ty21a vaccine, and the killed whole cell parenteral vaccine (Germanier and Fuer Citation1975; Tacket et al. Citation1986; Engels et al. Citation1998; Dave and Sefton Citation2015). A major drawback is that these vaccinations are unavailable for children under the age of 2 years, who are at high risk of infection in endemic areas (Zaki and Karande Citation2011). Furthermore, there are currently no vaccines against paratyphoid fever or non-typhoidal Salmonella disease; however, research is being carried out to address this particular problem. For non-typhoidal Salmonella, attenuated Salmonella enterica serovars Typhimurium and Enteritidis strains that can serve as live oral vaccines can be used for prevention. These vaccines have been tested in mice and have shown promising results, indicating that a highly effective, broad-spectrum vaccine against Salmonella can be developed (Tennant, Wang, et al. Citation2011). Since different Salmonella serotypes express immunologically distinct O sidechains, it will be challenging to create a multi-antigen vaccine or multiple vaccines targeting different serotypes. It should be a high priority to develop vaccines against typhoidal and non-typhoidal Salmonella strains to combat their deathly effects (Feasey et al. Citation2012; Martin Citation2012).
Conclusion
Patients with invasive typhoidal and non-typhoidal Salmonella infections require anti-microbial therapy. Due to the high rates of resistance to conventional drugs, such as ampicillin, chloramphenicol, and sulfamethoxazole-trimethoprim, the use of antibiotics for salmonellosis is now limited to ceftriaxone and azithromycin. The increase in rates of resistance to ceftriaxone is extremely worrisome and will result in patients relying solely on azithromycin. This trend will likely cause an increase in the burden of Salmonella infections worldwide and higher rates of morbidity and mortality. This effect will likely worsen as Salmonella strains develop resistance to azithromycin and other bacteria acquire the resistance genes. Therefore, there is an imperative need to control the spread of Salmonella, avoid the misuse and overuse of antimicrobial agents, and accelerate the development of new treatment options against salmonellosis.
Acknowledgments
The National Natural Science Foundation of China (NSFC) supported this work under grants 81271780 and 81471915.
Disclosure statement
No potential conflict of interest was reported by the authors.
ORCID
Ayman Ahmad Al kraiem http://orcid.org/0000-0001-9976-6062
Additional information
Funding
References
- Alexander KA, Warnick LD, Wiedmann M. 2009. Antimicrobial resistant Salmonella in dairy cattle in the United States. Vet Res Commun. 33(3):191–209. doi: 10.1007/s11259-008-9170-7
- Al-Mashhadani M, Hewson R, Vivancos R, Keenan A, Beeching NJ, Wain J, Parry CM. 2011. Foreign travel and decreased ciprofloxacin susceptibility in Salmonella enterica infections. Emerg Infect Dis. 17(1):123–125. doi: 10.3201/eid1701.100999
- Ao TT, Feasey NA, Gordon MA, Keddy KH, Angulo FJ, Crump JA. 2015. Global burden of invasive nontyphoidal Salmonella disease, 2010(1). Emerg Infect Dis. 21(6):941–949. doi: 10.3201/eid2106.140999
- Arlet G, Barrett TJ, Butaye P, Cloeckaert A, Mulvey MR, White DG. 2006. Salmonella resistant to extended-spectrum cephalosporins: prevalence and epidemiology. Microbes Infect. 8(7):1945–1954. doi: 10.1016/j.micinf.2005.12.029
- Barriere SL, Flaherty JF. 1984. Third-generation cephalosporins: a critical evaluation. Clin Pharmacol. 3(4):351–373.
- Butler T. 2011. Treatment of typhoid fever in the 21st century: promises and shortcomings. Clin Microbiol Infec. 17(7):959–963. doi: 10.1111/j.1469-0691.2011.03552.x
- CDC. 2013. National antimicrobial resistance monitoring system for enteric bacteria (NARMS): the 2011 NARMS annual human isolates report.
- Chen HM, Wang Y, Su LH, Chiu CH. 2013. Nontyphoid salmonella infection: microbiology, clinical features, and antimicrobial therapy. Pediatr Neonatol. 54(3):147–152. doi: 10.1016/j.pedneo.2013.01.010
- Cruchaga S, Echeita A, Aladuena A, Garcia-Pena J, Frias N, Usera MA. 2001. Antimicrobial resistance in salmonellae from humans, food and animals in Spain in 1998. J Antimicrob Chemother. 47(3):315–321. doi: 10.1093/jac/47.3.315
- Crump JA, Luby SP, Mintz ED. 2004. The global burden of typhoid fever. Bull World Health Organ. 82(5):346–353.
- Crump JA, Medalla FM, Joyce KW, Krueger AL, Hoekstra RM, Whichard JM, Barzilay EJ, Emerging Infections Program NWG. 2011. Antimicrobial resistance among invasive nontyphoidal Salmonella enterica isolates in the United States: national antimicrobial resistance monitoring system, 1996 to 2007. Antimicrob Agents Chemother. 55(3):1148–1154. doi: 10.1128/AAC.01333-10
- Dave J, Sefton A. 2015. Enteric fever and its impact on returning travellers. Int Health. 7(3):163–168. doi: 10.1093/inthealth/ihv018
- Davies J, Davies D. 2010. Origins and evolution of antibiotic resistance. Microbiol Mol Biol Rev. 74(3):417–433. doi: 10.1128/MMBR.00016-10
- Dunne EF, Fey PD, Kludt P, Reporter R, Mostashari F, Shillam P, Wicklund J, Miller C, Holland B, Stamey K. 2000. Emergence of domestically acquired ceftriaxone-resistant Salmonella infections associated with AmpC β-lactamase. JAMA. 284(24):3151–3156. doi: 10.1001/jama.284.24.3151
- Egorova S, Timinouni M, Demartin M, Granier SA, Whichard JM, Sangal V, Fabre L, Delaune A, Pardos M, Millemann Y, et al. 2008. Ceftriaxone-resistant salmonella enterica serotype Newport. France. Emerg Infect Dis. 14(6):954–957. doi: 10.3201/eid1406.071168
- Eng S-K, Pusparajah P, Ab Mutalib N-S, Ser H-L, Chan K-G, Lee L-H. 2015. Salmonella: A review on pathogenesis, epidemiology and antibiotic resistance. Front Life Sci. 8(3):284–293. doi: 10.1080/21553769.2015.1051243
- Engels EA, Falagas ME, Lau J, Bennish ML. 1998. Typhoid fever vaccines: a meta-analysis of studies on efficacy and toxicity. Br Med J. 316(7125):110–116. doi: 10.1136/bmj.316.7125.110
- Feasey NA, Dougan G, Kingsley RA, Heyderman RS, Gordon MA. 2012. Invasive non-typhoidal salmonella disease: an emerging and neglected tropical disease in Africa. Lancet. 379(9835):2489–2499. doi: 10.1016/S0140-6736(11)61752-2
- Fey PD, Safranek TJ, Rupp ME, Dunne EF, Ribot E, Iwen PC, Bradford PA, Angulo FJ, Hinrichs SH. 2000. Ceftriaxone-resistant salmonella infection acquired by a child from cattle. Eng J Med. 342(17):1242–1249. doi: 10.1056/NEJM200004273421703
- Fullybright R. 2016. The slippery difficulty of ever containing drug resistance with current practices. Eur J Clin Microbiol Infect Dis. 36(4):603–609. doi: 10.1007/s10096-016-2855-x
- Germanier R, Fuer E. 1975. Isolation and characterization of Gal E mutant Ty 21a of Salmonella typhi: a candidate strain for a live, oral typhoid vaccine. J Infect Dis. 131(5):553–558. doi: 10.1093/infdis/131.5.553
- Hall MJ, Westmacott D, Wong-Kai-In P. 1981. Comparative in-vitro activity and mode of action of ceftriaxone (Ro 13-9904), a new highly potent cephalosporin. J Antimicrob Chemother. 8(3):193–203. doi: 10.1093/jac/8.3.193
- Hamilton RD, Hulsebus HJ, Akbar S, Gray JT. 2012. Increased resistance to multiple antimicrobials and altered resistance gene expression in CMY-2-positive Salmonella enterica following a simulated patient treatment with ceftriaxone. Appl Environ Microbiol. 78(22):8062–8066. doi: 10.1128/AEM.02077-12
- Hendriksen RS, Mikoleit M, Carlson VP, Karlsmose S, Vieira AR, Jensen AB, Seyfarth AM, DeLong SM, Weill F-X, Wong DMALF. 2009. WHO global salm-surv external quality assurance system for serotyping of Salmonella isolates from 2000 to 2007. J Clin Microbiol. 47(9):2729–2736. doi: 10.1128/JCM.02437-08
- Jackson BR, Griffin PM, Cole D, Walsh KA, Chai SJ. 2013. Outbreak-associated Salmonella enterica serotypes and food commodities, United States, 1998–2008. Emerg Infect Dis. 19(8):1239–1244. doi: 10.3201/eid1908.121511
- John TJ. 2011. Efficacy and safety of azithromycin for typhoid fever. Indian J Pediatr. 48(10):823–824.
- Kariuki S, Gordon MA, Feasey N, Parry CM. 2015. Antimicrobial resistance and management of invasive Salmonella disease. Vaccine. 33(Suppl 3):C21–C29. doi: 10.1016/j.vaccine.2015.03.102
- Kariuki S, Okoro C, Kiiru J, Njoroge S, Omuse G, Langridge G, Kingsley RA, Dougan G, Revathi G. 2015. Ceftriaxone-resistant Salmonella enterica serotype typhimurium sequence type 313 from Kenyan patients is associated with the blaCTX-M-15 gene on a novel IncHI2 plasmid. Antimicrob Agents Chemother. 59(6):3133–3139. doi: 10.1128/AAC.00078-15
- Krueger AL, Greene SA, Barzilay EJ, Henao O, Vugia D, Hanna S, Meyer S, Smith K, Pecic G, Hoefer D, et al. 2014. Clinical outcomes of nalidixic acid, ceftriaxone, and multidrug-resistant nontyphoidal salmonella infections compared with pansusceptible infections in FoodNet sites, 2006–2008. Foodborne Pathog Dis. 11(5):335–341. doi: 10.1089/fpd.2013.1642
- Kumar S, Rizvi M, Berry N. 2008. Rising prevalence of enteric fever due to multidrug-resistant Salmonella: an epidemiological study. J Med Microbiol. 57(10):1247–1250. doi: 10.1099/jmm.0.2008/001719-0
- Kumarasamy KK, Toleman MA, Walsh TR, Bagaria J, Butt F, Balakrishnan R, Chaudhary U, Doumith M, Giske CG, Irfan S. 2010. Emergence of a new antibiotic resistance mechanism in India, Pakistan, and the UK: a molecular, biological, and epidemiological study. Lancet Infect Dis. 10(9):597–602. doi: 10.1016/S1473-3099(10)70143-2
- Lebens M, Karlsson SL, Kallgard S, Blomquist M, Ekman A, Nygren E, Holmgren J. 2011. Construction of novel vaccine strains of vibrio cholerae co-expressing the Inaba and Ogawa serotype antigens. Vaccine. 29(43):7505–7513. doi: 10.1016/j.vaccine.2011.06.121
- Li XZ, Mehrotra M, Ghimire S, Adewoye L. 2007. Beta-Lactam resistance and beta-lactamases in bacteria of animal origin. Vet Microbiol. 121(3-4):197–214. doi: 10.1016/j.vetmic.2007.01.015
- Lin D, Chen K, Wai-Chi Chan E, Chen S. 2015. Increasing prevalence of ciprofloxacin-resistant food-borne Salmonella strains harboring multiple PMQR elements but not target gene mutations. Sci Rep. 5:14754. doi: 10.1038/srep14754
- Majowicz SE, Musto J, Scallan E, Angulo FJ, Kirk M, O'Brien SJ, Jones TF, Fazil A, Hoekstra RM. 2010. International collaboration on enteric disease ‘Burden of Illness S. 2010. The global burden of nontyphoidal Salmonella gastroenteritis. Clin Infect Dis. 50(6):882–889. doi: 10.1086/650733
- Majtan J, Majtanova L, Majtan V. 2010. Increasing trend of resistance to nalidixic acid and emerging ceftriaxone and ciprofloxacin resistance in salmonella enterica serovar Typhimurium in Slovakia, 2005 to 2009. Diag Microb Infect Dis. 68(1):86–88. doi: 10.1016/j.diagmicrobio.2010.04.009
- Martin LB. 2012. Vaccines for typhoid fever and other Salmonelloses. Curr Opin Infect Dis. 25(5):489–499. doi: 10.1097/QCO.0b013e328356ffeb
- Medalla F, Hoekstra RM, Whichard JM, Barzilay EJ, Chiller TM, Joyce K, Rickert R, Krueger A, Stuart A, Griffin PM. 2013. Increase in resistance to ceftriaxone and nonsusceptibility to ciprofloxacin and decrease in multidrug resistance among Salmonella strains, United States, 1996–2009. Foodborne Pathog Dis. 10(4):302–309. doi: 10.1089/fpd.2012.1336
- Michael GB, Butaye P, Cloeckaert A, Schwarz S. 2006. Genes and mutations conferring antimicrobial resistance in Salmonella: an update. Microbes Infect. 8(7):1898–1914. doi: 10.1016/j.micinf.2005.12.019
- Mtove G, Amos B, Nadjm B, Hendriksen IC, Dondorp AM, Mwambuli A, Kim DR, Ochiai RL, Clemens JD, von Seidlein L, et al. 2011. Decreasing incidence of severe malaria and community-acquired bacteraemia among hospitalized children in Muheza, north-eastern Tanzania, 2006–2010. Malaria J. 10:320. doi: 10.1186/1475-2875-10-320
- Nastasi A, Mammina C, Cannova L. 2000. Antimicrobial resistance in Salmonella enteritidis, Southern Italy, 1990–1998. Emerg Infect Dis. 6(4):401–403. doi: 10.3201/eid0604.000415
- Olaitan AO, Dia NM, Gautret P, Benkouiten S, Belhouchat K, Drali T, Parola P, Brouqui P, Memish Z, Raoult D, et al. 2015. Acquisition of extended-spectrum cephalosporin- and colistin-resistant Salmonella enterica subsp. enterica serotype Newport by pilgrims during Hajj. Int J Antimicrob Agents. 45(6):600–604. doi: 10.1016/j.ijantimicag.2015.01.010
- Oneko M, Kariuki S, Muturi-Kioi V, Otieno K, Otieno VO, Williamson JM, Folster J, Parsons MB, Slutsker L, Mahon BE, et al. 2015. Emergence of community-acquired, multidrug-resistant invasive nontyphoidal Salmonella disease in rural western Kenya, 2009–2013. Clin Infect Dis. 61(Suppl 4):S310–S316. doi: 10.1093/cid/civ674
- Patel IH, Kaplan SA. 1984. Pharmacokinetic profile of ceftriaxone in man. Am J Med. 77(4C):17–25.
- Pham TD, Karkey A, Dongol S, Ho Thi N, Thompson CN, Rabaa MA, Arjyal A, Holt KE, Wong V, Tran Vu Thieu N, et al. 2016. A novel ciprofloxacin-resistant subclade of H58 Salmonella Typhi is associated with fluoroquinolone treatment failure. eLife. 5:e14003.
- Qamar FN, Azmatullah A, Kazi AM, Khan E, Zaidi AK. 2014. A three-year review of antimicrobial resistance of Salmonella enterica serovars Typhi and Paratyphi A in Pakistan. J Infect Dev Countr. 8(8):981–986.
- Rahman BA, Wasfy MO, Maksoud MA, Hanna N, Dueger E, House B. 2014. Multi-drug resistance and reduced susceptibility to ciprofloxacin among Salmonella enterica serovar Typhi isolates from the Middle East and Central Asia. New Microbes New Infect. 2(4):88–92. doi: 10.1002/nmi2.46
- Rotimi VO, Jamal W, Pal T, Sovenned A, Albert MJ. 2008. Emergence of CTX-M-15 type extended-spectrum β-lactamase-producing Salmonella spp. in Kuwait and the United Arab Emirates. J Med Microbiol. 57(7):881–886. doi: 10.1099/jmm.0.47509-0
- Saha SK, Talukder SY, Islam M, Saha S. 1999. A highly ceftriaxone-resistant Salmonella typhi in Bangladesh. Pediatric Infect Dis J. 18(4):387. doi: 10.1097/00006454-199904000-00018
- Salmon-Rousseau A, Piednoir E, Cattoir V, de La Blanchardière A. 2016. Hajj-associated infections. Med Maladies Infect. 46(7):346–354. doi: 10.1016/j.medmal.2016.04.002
- Smith S, Seriki A, Ajayi A. 2016. Typhoidal and non-typhoidal Salmonella infections in Africa. EJCMID. 35(12):1913–1922. doi: 10.1007/s10096-016-2760-3
- Su LH, Chiu CH, Chu C, Ou JT. 2004. Antimicrobial resistance in nontyphoid Salmonella serotypes: a global challenge. Clin Infec Dis. 39(4):546–551. doi: 10.1086/422726
- Su LH, Chiu CH, Chu C, Wang MH, Chia JH, Wu TL. 2003. In vivo acquisition of ceftriaxone resistance in Salmonella enterica serotype anatum. Antimicrob Agents Chemother. 47(2):563–567. doi: 10.1128/AAC.47.2.563-567.2003
- Tacket CO, Ferreccio C, Robbins JB, Tsai CM, Schulz D, Cadoz M, Goudeau A, Levine MM. 1986. Safety and immunogenicity of two Salmonella typhi Vi capsular polysaccharide vaccines. J Infect Dis. 154(2):342–345. doi: 10.1093/infdis/154.2.342
- Takem EN, Roca A, Cunnington A. 2014. The association between malaria and non-typhoid salmonella bacteraemia in children in sub-saharan Africa: a literature review. Malaria J. 13:400. doi: 10.1186/1475-2875-13-400
- Tennant SM, Wang JY, Galen JE, Simon R, Pasetti MF, Gat O, Levine MM. 2011. Engineering and preclinical evaluation of attenuated nontyphoidal salmonella strains serving as live oral vaccines and as reagent strains. Infect Immun. 79(10):4175–4185. doi: 10.1128/IAI.05278-11
- Tennant SM, Zhang Y, Galen JE, Geddes CD, Levine MM. 2011. Ultra-fast and sensitive detection of non-typhoidal Salmonella using microwave-accelerated metal-enhanced fluorescence (“MAMEF”). PloS One. 6(4):e18700. doi: 10.1371/journal.pone.0018700
- Valdez Y, Ferreira RB, Finlay BB. 2009. Molecular mechanisms of Salmonella virulence and host resistance. Curr Top Microbiol Immunol. 337:93–127.
- Van TTH, Nguyen HNK, Smooker PM, Coloe PJ. 2012. The antibiotic resistance characteristics of non-typhoidal Salmonella enterica isolated from food-producing animals, retail meat and humans in South East Asia. Int J Food Microbiol. 154(3):98–106. doi: 10.1016/j.ijfoodmicro.2011.12.032
- van der Bij AK, Pitout JD. 2012. The role of international travel in the worldwide spread of multiresistant enterobacteriaceae. J Antimicrob Chemother. 67(9):2090–2100. doi: 10.1093/jac/dks214
- Velge P, Wiedemann A, Rosselin M, Abed N, Boumart Z, Chausse AM, Grepinet O, Namdari F, Roche SM, Rossignol A, et al. 2012. Multiplicity of Salmonella entry mechanisms, a new paradigm for Salmonella pathogenesis. MicrobiologyOpen. 1(3):243–258. doi: 10.1002/mbo3.28
- Voetsch AC, Van Gilder TJ, Angulo FJ, Farley MM, Shallow S, Marcus R, Cieslak PR, Deneen VC, Tauxe RV. 2004. Emerging infections Program FoodNet Working G. 2004. FoodNet estimate of the burden of illness caused by nontyphoidal Salmonella infections in the United States. Clin Infect Dis. 38(Suppl 3):S127–S134. doi: 10.1086/381578
- Wiedemann A, Virlogeux-Payant I, Chausse AM, Schikora A, Velge P. 2014. Interactions of Salmonella with animals and plants. Frontiers Microbiol. 5:791.
- Wise EM, Park JT. 1965. Penicillin: its basic site of action as an inhibitor of a peptide cross-linking reaction in cell wall mucopeptide synthesis. PNAS. 54(1):75–81. doi: 10.1073/pnas.54.1.75
- Wong MH, Yan M, Chan EW, Biao K, Chen S. 2014. Emergence of clinical Salmonella enterica serovar Typhimurium isolates with concurrent resistance to ciprofloxacin, ceftriaxone, and azithromycin. Antimicrob Agents Chemother. 58(7):3752–3756. doi: 10.1128/AAC.02770-13
- Wong MH, Zeng L, Liu JH, Chen S. 2013. Characterization of Salmonella food isolates with concurrent resistance to ceftriaxone and ciprofloxacin. Foodborne Pathog Dis. 10(1):42–46. doi: 10.1089/fpd.2012.1266
- Yan JJ, Chiu CH, Ko WC, Chuang CL, Wu JJ. 2002. Ceftriaxone-resistant Salmonella enterica serovar Hadar: evidence for interspecies transfer of blaCMY-2 in a Taiwanese university hospital. J Formos Med Assoc. 101(9):665–668.
- Yan JJ, Ko WC, Chiu CH, Tsai SH, Wu HM, Wu JJ. 2003. Emergence of ceftriaxone-resistant Salmonella isolates and rapid spread of plasmid-encoded CMY-2-like cephalosporinase. Taiwan. Emerg Infect Dis. 9(3):323–328.
- Yang WC, Chan OW, Wu TL, Chen CL, Su LH, Chiu CH. 2016. Development of ceftriaxone resistance in Salmonella enterica serotype oranienburg during therapy for bacteremia. J Microb Immunol Infect. 49(1):41–45. doi: 10.1016/j.jmii.2014.01.011
- Yang B, Wang Q, Cui S, Wang Y, Shi C, Xia X, Xi M, Wang X, Shi X, Wang D, et al. 2014. Characterization of extended-spectrum beta-lactamases-producing salmonella strains isolated from retail foods in Shaanxi and Henan Province, China. Food Microbiol. 42:14–18. doi: 10.1016/j.fm.2014.02.003
- Yates C, Amyes S. 2005. Extended-spectrum β-lactamases in non-typhoidal Salmonella spp. isolated in the UK are now a reality: why the late arrival? JAC. 56(2):262–264.
- Yildirmak T, Yazgan A, Ozcengiz G. 1998. Multiple drug resistance patterns and plasmid profiles of non-typhi salmonellae in Turkey. Epidemiol Infect. 121(2):303–307. doi: 10.1017/S0950268898001253
- Zaki SA, Karande S. 2011. Multidrug-resistant typhoid fever: a review. J Infect Dev Countr. 5(5):324–337.
- Zowawi HM, Balkhy HH, Walsh TR, Paterson DL. 2013. β-Lactamase production in key gram-negative pathogen isolates from the Arabian Peninsula. Clin Microb Rev. 26(3):361–380. doi: 10.1128/CMR.00096-12