Abstract
Excessive reactive oxygen species (ROS) induced by myocardial ischemia reperfusion (IR) injury exert detrimental effects on cardiomyocytes. Antioxidant peptides can scavenge free radicals such as 2,2′-azinobis(3-ethylbenzothiazoline-6-sulfonic acid) (ABTS+), 2,2-diphenyl-1-picrylhydrazyl (DPPH) and nitic oxide (NO) in vitro, but little is known about their functions in vivo. Here, we evaluated the effects of an antioxidant peptide (antioxidant-RL) used at the beginning of reperfusion on myocardial IR injury. We performed 2, 3, 5-triphenyltetrazolium chloride staining (TTC) to determine myocardial infarct size. Creatine kinase isoenzyme-MB (CK-MB), cardiac troponin-T (CTnT), tumor necrosis factor-alpha (TNF-α), and interleukin-6 (IL-6) levels were determined with enzyme-linked immunosorbent assays (ELISAs). The levels of ROS were measured with a fluorometric Intracellular ROS kit. The expression levels of apoptosis-related proteins in cardiomyocytes were detected by western blotting. Antioxidant-RL (10 mg/kg) reduced the infarct size and levels of CK-MB and CTnT in rats. The levels of TNF-α and IL-6 also decreased. Furthermore, ROS levels were ameliorated and the expression of apoptosis-related proteins in myocytes was down-regulated by antioxidant-RL (100 µg/mL). Antioxidant-RL exerted protective effects on myocardial IR injury by scavenging excessive ROS, suppressing inflammatory factors, and inhibiting cardiomyocytes apoptosis. Thus, antioxidant-RL may serve as a potent candidate for the treatment of IR injury.
GRAPHICAL ABSTRACT
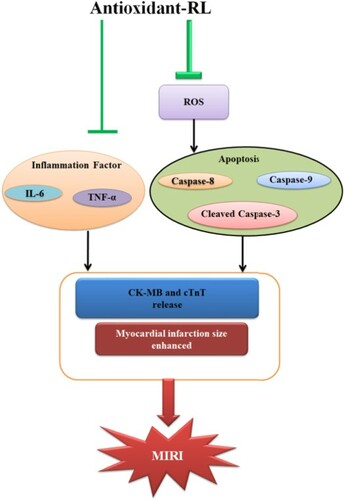
Introduction
Acute myocardial infarction is a major cause of disabling morbidity and death worldwide (Hausenloy and Yellon Citation2013). Effective therapy for acute myocardial infarction involves timely reperfusion (Reimer et al. Citation1977), which may also induce cardiac ischemia reperfusion (IR) injury (Braunwald and Kloner Citation1985). Reperfusion causes arrhythmias and reversible contractile dysfunction (stunning), initiates a ?>microvascular no-reflow, and contributes to myocardial infarction, thereby acting as a major determinant of prognosis (Heusch Citation2013). Several pathological mechanisms are known to contribute to reperfusion injury (Kloner Citation1988; Richard et al. Citation1990; Imahashi et al. Citation2005; Matejikova et al. Citation2009; Kaljusto et al. Citation2010; Gao et al. Citation2017; Scofield et al. Citation2019), including excessive formation of reactive oxygen species (ROS), intracellular calcium overload, mitochondrial dysfunction, activation of intracellular proteolysis, and uncoordinated excessive contractile activity. ROS exhibit signaling functions in small amounts but could be detrimental when in excess. Excessive ROS could attack cellular components and induce apoptosis and necrosis (Murphy and Steenbergen Citation2008). Therefore, inhibition of excessive ROS may be an important intervention to prevent IR injury. However, no clinical antioxidant drug is yet available for cardioprotection.
Amphibian antioxidant peptides are a group of fast-acting antioxidants in the skin. These peptides possess the ability to rapidly and constantly eliminate free radicals such as 2,2′-azinobis(3-ethylbenzothiazoline-6-sulfonic acid (ABTS+), 2,2-diphenyl-1-picrylhydrazyl (DPPH), and nitic oxide (NO) within a few seconds and exert anti-inflammatory effects. In amphibians, these peptides protect the animal skin from infection or ROS injury (Yang et al. Citation2009). Antioxidant-RL, a short 14 residue antioxidant peptide (AMRLTYNRPCIYAT) extracted from the skin secretions of Rana pleuraden, has the ability to eliminate ABTS+ in a few seconds. Investigation of the underlying antioxidant mechanism has indicated the crucial role of Tyr6, Cys10, and Tyr12 residues in radical elimination (Liu et al. Citation2010). Both Tyr6 and Tyr12 covalently interact with ABTS+ via their tyrosyl groups and form a purple product. The free thiol group in Cys10 is responsible for the rapid scavenging of ABTS+ through the donation of electrons to radicals (Liu et al. Citation2010). Although antioxidant-RL can scavenge free radicals in vitro, no study has evaluated its function in vivo.
Here, we investigate the potential of antioxidant-RL to reduce myocardial IR injury by scavenging excessive ROS. We measured the infarct size and the level of creatine kinase isoenzyme MB (CK-MB) and cardiac troponin-T (CTnT) (the most sensitive markers for minor myocardial damage) in a rat model of myocardial IR injury after treatment with antioxidant-RL. We examined the ability of antioxidant-RL to eliminate excessive ROS induced by IR injury. In addition, we determine the profiles of inflammatory markers in plasma samples and apoptosis rate of cardiomyocytes. Our results demonstrate that antioxidant-RL protects rats against IR injury by preventing the IR-induced excessive production of ROS.
Materials and methods
Peptide synthesis
Antioxidant-RL (AMRLTYNRPCIYAT) was synthesized by the peptide synthesizer GL Biochem Ltd (Shanghai, China). The synthetic peptide was purified and analyzed with high-performance liquid chromatography (HPLC) and matrix-assisted laser desorption/ionization (MALDI)-time of flight (TOF) mass spectrometry (MS) to confirm that the purity was higher than 98%.
Animal and ethics
Healthy male Sprague-Dawley (SD) rats weighting 220–280 g were obtained from the Kunming Medical University Animal Experiment Center (license No.53004100000079). These specific pathogen-free (SPF) rats were provided with water and food according to experimental animal standards. The study was performed in accordance with the recommendations in the Guidance for the Care and Use of Laboratory Animals issued by the Laboratory Animal Ethics Committee of Kunming Medical University (Figure ).
Figure 1. Schematic representation of the experimental protocol in rats. Myocardial ischemia was induced by the occlusion of LAD branch. Reperfusion of ischemic myocardium for 120 min after 60 min of ischemia was completed by loosening the silk thread. After reperfusion inception, antioxidant-RL (10 mg/kg) and NAC (150 mg/kg) were administered via the tail vein.
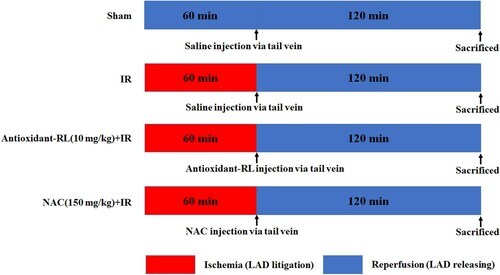
IR model in rats
Rats were anesthetized with sodium pentobarbital (50 mg/kg, intraperitoneal) and intubated before being artificially ventilated with room air at a frequency of 80 inflations/min on a tidal volume of 1 mL/100 g. Lead II of electrocardiogram (ECG) was recorded via cutaneous needle electrodes. Heart was accessed through left thoracotomy in the fourth intercostal space. The pericardium was incised, and the heart was exposed. In the IR group, occlusion of the left anterior descending (LAD) branch was maintained for 60 min by lifting a 5/0 silk thread passing below the LAD through a piece of polyethylene tube. Criteria for confirmation of successful coronary occlusion included appearance of pale color in the distal myocardium and ST elevation on ECG (Figure (A)). In all IR groups, reperfusion of ischemic myocardium was allowed for 120 min after 60 min of ischemia via loosening of the silk thread. Antioxidant-RL (10 mg/kg) and N-acetylcysteine (NAC; 150 mg/kg) were administered via the tail vein at the beginning of reperfusion. Blood samples were collected to obtain plasma after 2 h reperfusion. The expression of cardiac markers CK-MB and CTnT and serum levels of inflammatory cytokines tumor necrosis factor alpha (TNF-α) and interleukin-6 (IL-6) were evaluated with enzyme-linked immunosorbent assays (ELISAs).
Figure 2. Antioxidant-RL reduces myocardial IR injury in rats. (A) ST-segment elevation was the most important indicator of LAD branch of coronary artery occlusion. ST-segment was elevated after the occlusion of LAD but reduced after LAD recanalization. No significant difference was observed between the groups. (B) Antioxidant-RL (5, 10, and 15 mg/kg) and NAC (150 mg/kg) reduced the infarct size, as evident from TTC staining, in rats subjected to ischemia reperfusion (IR) injury. The white regions represent the infracted area. Data are presented as mean ± SD (n = 5). (C and D) Antioxidant-RL lowered the plasma concentrations of CK-MB and CTnT in different groups. *P < 0.05 represents significant differences between IR and Sham group; #P < 0.05 represents significant difference between antioxidant-RL + IR versus IR group.
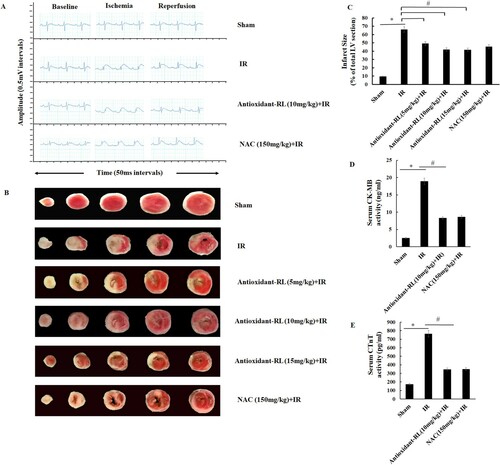
Staining with 2,3,5-triphenyltetrazolium chloride (TTC) to determine infarct area
TTC staining accurately reflects the extent of irreversible myocardial ischemic damage. After washing with phosphate-buffered saline (PBS), the left ventricle was frozen at −20°C and cut into slices (2 mm thickness). The sections were incubated with a 1% TTC (Solarbio Science & Technology Co., Ltd, Beijing, China) and urethane (0.1 g TTC dissolved in 5 mL PBS, Sigma) solution at pH 8.5 and 37°C for 30 min. The infarcted area showed no staining, while the normal tissue showed reddish brown appearance. Image pro plus 6.0 software was used to calculate the infarct size.
IR model with H9c2 cells
The rat H9c2 cardiomyocytes were generously provided by Professor Qiu-Xiong LING (Research Center of Medical Sciences, Guangdong Academy of Medical Sciences, Guangzhou, China). H9c2 cardiomyocytes were cultured in Dulbecco’s modified Eagle’s medium (DMEM)/F12 (Gibco/BRL, Gaithersburg, MD, USA) supplemented with 10% fetal bovine serum (FBS; HyClone, Logan, UT, USA) and antibiotics (100 IU/mL penicillin and 100 mg/mL streptomycin) at a density below 5 × 105 cells/mL and maintained at 37°C in a humidified incubator with 5% CO2. To harvest H9c2 cardiomyocytes, cells were trypsinized (0.25% trypsin/ethylenediaminetetraacetic acid [EDTA] in PBS), centrifuged (400 × g for 10 min), and resuspended in serum-free DMEM/F12. Cells were counted with a hemocytometer; trypan blue staining (0.4% trypan blue in PBS) showed more than 98% viable cells. Cells (approximately 1 × 106 cells/mL) were seeded in six-well plates for 24 h before treatment inception.
To simulate IR injury, we performed IR with H9c2 cells. The cultured H9c2 cells were incubated in DMEM/F12 high glucose medium (4.5 g/L) with 10% FBS for 24 h and then subjected to oxygen-glucose deprivation/reperfusion (OGD/R) to mimic myocardial IR. Experiments in H9c2 cardiomyocytes were carried out at a cell density of 5 × 105 cells/mL. H9c2 cardiomyocytes were cultured in DMEM/F12 supplemented without glucose and serum for 40 min in a humidified incubator with 0.5% O2 (for mimicking the stage of OGD). The cells were cultured in DMEM/F12 supplemented with high glucose and 10% FBS for 2 h in a humidified incubator with 5% CO2 (for mimicking the stage of reperfusion). Antioxidant-RL at 100 µg/mL and NAC at 0.1 M were added 1 h before mimicking OGD. This time point was chosen to minimize the possibility of any direct interactions between antioxidant-RL and serum deprivation condition.
Measurement of intracellular ROS production
Intracellular ROS level was measured using a fluorometric intracellular ROS assay kit (Sigma-Aldrich, St. Louis, USA), which provides a sensitive, one-step fluorometric assay to detect intracellular ROS (especially superoxide and hydroxyl radical) in live cells within 1 h incubation. ROS react with a cell-permeable sensor and produce a fluorometric product (λex = 540 nm/λem = 570 nm). The intensity of the colored product is proportional to the amount of ROS. The assay can be performed in a convenient 96-well or 384-well microtiter-plate format.
Western blot analysis
After reperfusion, great vessels and atria of the heart were trimmed away and the ventricles were cut open, weighed, snap-frozen, and stored at −80°C until use. At the end of reperfusion, proteins from cardiomyocytes and the heart were extracted with ice-cold lysis buffer and centrifuged at 12,000 × g for 10 min. The resultant supernatant was assayed using bicinchoninic acid (BCA) protein assay kit and bovine serum albumin (BSA) was used as a standard. Equal amounts of total protein (40 µg) were loaded, separated by sodium dodecyl sulfate polyacrylamide gel electrophoresis (SDS-PAGE), and transferred onto polyvinylidene fluoride (PVDF) membranes. The membranes were blocked with 5% tris-buffered saline and Tween-20 (TBST) fat-free milk (blocking buffer) for 2 h, briefly washed, and incubated overnight at 4°C with primary antibodies against caspase-3 (1:2000, no. ab2758369, Abcam, Cambridge, MA, USA), caspase-8 (1:2000, no. ab25901, Abcam, Cambridge, MA, USA), and caspase-9 (1:2000, ab32539, Abcam, Cambridge, MA, USA). Blots were then probed with an anti-rabbit secondary antibody (1:1000) for 2 h. Proteins were detected using a chemiluminescence system, according to the manufacturer’s instructions. Band intensities were quantified using Imaging System Analysis software (VersaDocMp5000; Bio-Rad). Relevant band intensities were quantified after normalization to the amount of β-actin protein as a positive control.
Statistical analysis
All values are expressed as the mean ± standard deviation (S.D). Comparison between multiple-group means was performed using one-way analysis of variance (ANOVA). Multiple comparison between groups was performed using the least significant difference method. A value of P < 0.05 was considered statistically significant. All statistical analyses were performed using SPSS version 17.0 (International Business Machines Corp., Armonk, NY, USA).
Results
Antioxidant-RL protects the heart against myocardial IR injury
We observed an elevation in the ST-segment after the occlusion of the LAD branch, and a drop in the ST-segment was reported after the loosening of the LAD branch. No significant difference in the change in the ST-segment was observed in different myocardial IR groups (Figure (A)).
We investigated the effect of antioxidant-RL treatment on myocardial IR injury through the analysis of infarct size and CK-MB and CTnT levels. The infarcted myocardium appeared white after TTC staining. The infarct size was significantly larger in IR group (65.98% ± 2.00%) than in Sham group (9.58% ± 0.39%) (Figure (B and C), P < 0.05). However, a remarkable decrease in the infarct size was observed in antioxidant-RL + IR and NAC + IR groups (45.34% ± 2.38% and 41.92% ± 1.51%, respectively) as compared to that in IR group (Figure (B and C), P < 0.05).
The levels of CK-MB and CTnT were higher in IR group (CK-MB: 18.08 ± 0.56 ng/mL; CTnT: 765.40 ± 15.19 pg/mL) than in Sham group (CK-MB: 2.32 ± 0.14 ng/mL; CTnT: 172.40 ± 12.05 pg/mL) (Figure (D and E), P < 0.05), but the antioxidant-RL treatment ameliorated the elevated levels of CK-MB and CTnT (CK-MB: 8.44 ± 0.20 ng/mL; CTnT: 347.20 ± 10.68 pg/mL). The levels of CK-MB (79.4 ± 1.81 ng/mL) and CTnT (348.4 ± 6.46 pg/mL) in NAC + IR group also decreased as compared to those reported in IR group (Figure (D and E), P < 0.05).
Antioxidant-RL suppresses the levels of inflammatory factors
We observed an increase in the level of TNF-α in IR group (156.80 ± 8.19 pg/mL) as compared with that in Sham group (29.60 ± 1.77 pg/mL). However, antioxidant-RL could reduce the elevated levels of TNF-α caused by IR (108.80 ± 7.37 pg/mL) (Figure (A), P < 0.05).
Figure 3. Antioxidant-RL reduces inflammatory factors in rats. Antioxidant-RL (10 mg/kg) reduced the plasma concentrations of TNF-α and IL-6 in different groups. *P < 0.05 represents significantly difference between IR and Sham group; #P < 0.05 represents significant difference between antioxidant-RL + IR and IR group.
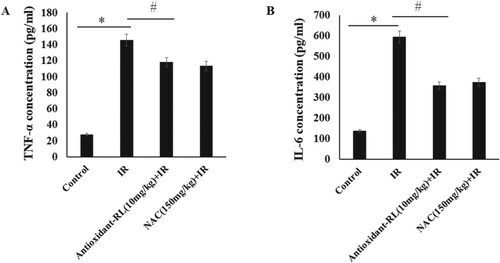
The level of IL-6 in IR group (604.56 ± 15.74 pg/mL) was higher than that in Sham group (138.2 ±6.09 pg/mL), but the treatment with antioxidant-RL reduced the elevated level of IL-6 (354.64 ± 9.93 pg/mL) (Figure (B), P < 0.05).
Antioxidant-RL alleviates oxidative stress in IR H9C2 cardiomyocytes
ROS overproduction and oxidative stress have detrimental effects on the heart in myocardial IR injury. Thus, we performed studies to understand the relationship between ROS level and myocardial IR injury. We found that ROS level greatly increased after myocardial IR (21.94% ± 1.10%) as compared with Sham group (3.06% ± 0.49%). However, these elevated ROS levels decreased after antioxidant-RL treatment (9.00% ± 0.40%) (Figure (A and B), P < 0.05).
Figure 4. Antioxidant-RL reduces the level of ROS induced by IR injury in H9C2 cardiomyocytes. Antioxidant-RL (100 µg/mL) decreased IR-induced ROS levels in H9C2 cardiomyocytes. *P < 0.05 represents significant difference between IR and control group; #P < 0.05 represents significant difference between antioxidant-RL + IR and IR group.
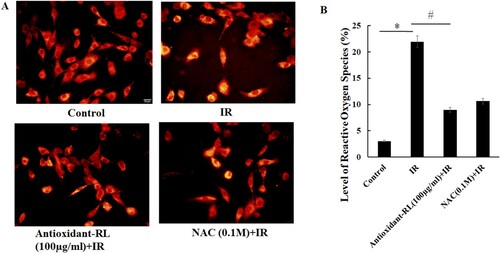
Antioxidant-RL suppresses myocardial cell apoptosis in IR injury
The primary mechanism underlying cell death in myocardial IR is apoptosis. Thus, we explored the effects of antioxidant-RL treatment on myocardial IR rats and H9C2 cardiomyocytes through the analysis of the expression levels of apoptosis-related proteins, cleaved caspase-3, caspase-8, and caspase-9. As a result, we found that the expression levels of cleaved caspase-3, caspase-8, and casapase-9 were higher for H9c2 cardiomyocytes from IR group than those from Sham group. Antioxidant-RL decreased the expression of caspase-3, caspase-8, and csapase-9 in IR H9C2 cardiomyocytes (Figure (A–D), P < 0.05). Similarly, it also decreased the expression of caspase-3, caspase-8, and csapase-9 in myocardial IR rats (Figure (E–H), P < 0.05).
Figure 5. Antioxidant-RL reduces the apoptosis of cardiomyocytes after IR injury. (A–D) The expression levels of apoptosis-related proteins, caspase-8, caspase-9, and cleaved caspase-3, in different H9C2 cardiomyocyte groups. Antioxidant-RL (100 µg/mL) treatment reduced the expression of apoptosis-related proteins caspase-8, caspase-9, and cleaved caspase-3 in H9c2 cardiomyocytes. (E–H) The results of the expression of caspase-8, caspase-9, and cleaved caspase-3 in myocardial IR rats were similar to those reported in H9C2 cardiomyocytes. Antioxidant-RL (10 mg/kg) decreased the levels of caspase-8, caspase-9, and cleaved caspase-3 in SD rats. *P < 0.05 represents significant difference between IR and Sham group; #P < 0.05 represents significant difference between antioxidant-RL + IR and IR group.
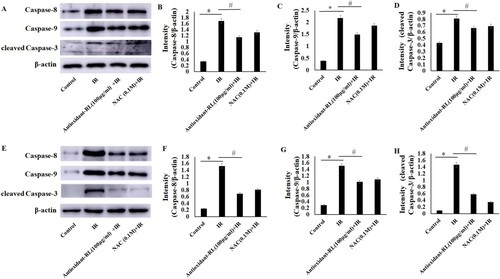
Discussion
Myocardial reperfusion injury is the process that can induce cardiomyocyte death, and no effective therapy is yet approved against this pathophysiology. In the present study, the function of an amphibian antioxidant peptide (antioxidant-RL) was evaluated in a rat model of myocardial IR injury. Different doses of antioxidant-RL (5, 10, and 15 mg/kg) were used to investigate the cardioprotective effects in rats after myocardial IR injury. The results indicated that the effect of antioxidant-RL on reducing myocardial infarct size was more obvious at 10 mg/kg than at 5 mg/kg; however, the highest dose of antioxidant-RL (15 mg/kg) had no extra protective effects. These observations demonstrate that antioxidant-RL (10 mg/kg) could reduce myocardial infarct size and decrease the levels of two IR injury markers (CK-MB and CTnT), indicative of its cardioprotective role in vivo.
Oxidative stress is one of key mechanisms in myocardial IR injury (Bagheri et al. Citation2016; Sinning et al. Citation2017). Excessive ROS can increase myocardial infarct size and deteriorate ventricular function (Chen et al. Citation2018). Overproduction of ROS after myocardial IR can damage cells by lipid peroxidation, protein denaturation, and nucleic acid deterioration and severely affect the overall metabolism (Cadenas Citation2018). Thus, antioxidant peptides with rapid radical-scavenging ability may serve as attractive agents to reduce the level of ROS and subsequently alleviate myocardial IR injury. Our data show that antioxidant-RL decreased the level of ROS induced by IR injury in cardiomyocytes (Figure ), and exerted protective effects on rat hearts through the scavenging of excessive ROS.
Inflammation is considered as the most important cause of tissue injury in organs subjected to ischemia (Eefting Citation2004; Ballard-Croft et al. Citation2008). Antioxidant-RL reduced the levels of TNF-α and IL-6 in myocardial IR injury (Figure ). The suppression of inflammatory factors could lead to alleviation of myocardial IR injury. Apoptosis is a crucial event that can initiate reperfusion-induced inflammation and subsequent tissue injury (Hamacher-Brady et al. Citation2006; Peng et al. Citation2011). Therefore, inhibition of myocardial apoptosis is a key protective mechanism against myocardial IR injury. The process of apoptosis mainly relies on the activation of caspases (Kaufmann and Hengartner Citation2001). The downstream caspases (caspase-3, caspase-6, and caspase-7) are activated to stimulate the caspase cascade and induce cell death upon the activation of the upstream caspases (caspase-8, caspase-9, and caspase-10) (Kavurma et al. Citation2005). Caspase-8 and caspase-9 are activated in myocardial IR injury and subsequently trigger the activation of downstream effectors caspases such as caspase-3. Caspase-3 is mainly responsible for mediating the typical pathophysiological and morphological changes in the cells undergoing apoptosis (Brar et al. Citation2001). Antioxidant-RL (AMRLTYNRPCIYAT) is a cationic host defense peptide (HDP) with a net charge of +2. HDPs perform multifaceted functions during inflammation and immune modulation (Hancock et al. Citation2016). Recent studies have reported that endogenous cathelicidins (CRAMP and LL-37), a major family of HDPs, are involved in cardiovascular diseases through the regulation of inflammatory response, microvascular dysfunction (Zheng et al. Citation2019), and myocardial apoptosis upon IR injury (Bei et al. Citation2019). The CRAMP peptide was shown to inhibit cardiomyocyte apoptosis through the activation of protein kinase B (Akt) and extracellular signal-regulated kinase (ERK1/2) and phosphorylation and nuclear export of FoxO3a (Bei et al. Citation2019). The increase in LL-37 level was thought to serve as an attractive therapeutic strategy for cardiac ischemic injury (Bei et al. Citation2019). Our results show that aside from its antioxidant capacity, antioxidant-RL functions like the CRAMP peptide and inhibits cardiomyocyte apoptosis by decreasing the expression of apoptosis-related proteins (cleaved caspase-3, caspase-8, and csapase-9) (Figure ). Such a bi-functional agent that inhibits apoptosis and rapidly scavenges free radicals could offer more advantages than the antioxidant agents with only radical-scavenging function for the treatment of IR injury. Further investigations are warranted to determine the mechanisms underlying the effects of antioxidant-RL on myocardial apoptosis upon IR injury.
In conclusion, our data demonstrate that antioxidant-RL could reduce myocardial infarct size, decrease levels of markers of IR injury (CK-MB and CTnT), and suppress inflammatory factors (TNF-α and IL-6) and apoptosis-associated proteins (cleaved caspase-3, caspase-8, and csapase-9) in myocardial IR injury. The protective effects of antioxidant-RL on IR injury were mediated through the amelioration of oxidative stress, inflammatory factors, and myocardial apoptosis. The combination of these properties deems antioxidant-RL as an attractive candidate for the treatment of myocardial IR injury.
Disclosure statement
No potential conflict of interest was reported by the authors.
Ethical approval
All animal procedures were approved by and performed as per the Guidelines for the Care and Use of Animals as stipulated by Kunming Medical University. The experiments were also performed according to the Chinese National Institutes of Health Guidelines for the Use of Laboratory Animals. Animal Certificate Number: 5300410000079.
Additional information
Funding
References
- Bagheri F, Khori V, Alizadeh AM, Khalighfard S, Khodayari S, Khodayari H. 2016. Reactive oxygen species-mediated cardiac-reperfusion injury: mechanisms and therapies. Life Sci. 165:43–55. doi: https://doi.org/10.1016/j.lfs.2016.09.013
- Ballard-Croft C, Locklar AC, Keith BJ, Mentzer RM Jr, Lasley RD. 2008. Oxidative stress and adenosine A1 receptor activation differentially modulate subcellular cardiomyocyte MAPKs. Am J Physiol Heart Circ Physiol. 294(1):H263–H271. doi: https://doi.org/10.1152/ajpheart.01067.2007
- Bei Y, Pan LL, Zhou Q, Zhao C, Xie Y, Wu C, Meng X, Gu H, Xu J, Zhou L, et al. 2019. Cathelicidin-related antimicrobial peptide protects against myocardial ischemia/reperfusion injury. BMC Med. 17(1):1–20. doi: https://doi.org/10.1186/s12916-019-1268-y
- Brar BK, Stephanou A, Pennica D, Latchman DS. 2001. CT-1 mediated cardioprotection against ischaemic re-oxygenation injury is mediated by PI3 kinase, Akt and MEK1/2 pathways. Cytokine. 16(3):93–96. doi: https://doi.org/10.1006/cyto.2001.0951
- Braunwald E, Kloner RA. 1985. Myocardial reperfusion: a double-edged sword? J Clin Invest. 76(5):1713–1719. doi: https://doi.org/10.1172/JCI112160
- Cadenas S. 2018. ROS and redox signaling in myocardial ischemia-reperfusion injury and cardioprotection. Free Radic Biol Med. 117:76–89. doi: https://doi.org/10.1016/j.freeradbiomed.2018.01.024
- Chen Q, Younus M, Thompson J, Hu Y, Hollander JM, Lesnefsky EJ. 2018. Intermediary metabolism and fatty acid oxidation: novel targets of electron transport chain-driven injury during ischemia and reperfusion. Am J Physiol Heart Circ Physiol. 314(4):H787–H795. doi: https://doi.org/10.1152/ajpheart.00531.2017
- Eefting F. 2004. Role of apoptosis in reperfusion injury. Cardiovasc Res. 61(3):414–426. doi: https://doi.org/10.1016/j.cardiores.2003.12.023
- Gao Y, Yang H, Chi J, Xu Q, Zhao L, Yang W, Liu W, Yang W. 2017. Hydrogen gas attenuates myocardial ischemia reperfusion injury independent of postconditioning in rats by attenuating endoplasmic reticulum stress-induced autophagy. Cell Physiol Biochem. 43(4):1503–1514. doi: https://doi.org/10.1159/000481974
- Hamacher-Brady A, Brady NR, Gottlieb RA. 2006. The interplay between pro-death and pro-survival signaling pathways in myocardial ischemia/reperfusion injury: apoptosis meets autophagy. Cardiovasc Drugs Ther. 20(6):445–462. doi: https://doi.org/10.1007/s10557-006-0583-7
- Hancock RE, Haney EF, Gill EE. 2016. The immunology of host defence peptides: beyond antimicrobial activity. Nat Rev Immunol. 16(5):321–334. doi: https://doi.org/10.1038/nri.2016.29
- Hausenloy DJ, Yellon DM. 2013. Myocardial ischemia-reperfusion injury: a neglected therapeutic target. J Clin Invest. 123(1):92–100. doi: https://doi.org/10.1172/JCI62874
- Heusch G. 2013. Cardioprotection: chances and challenges of its translation to the clinic. Lancet. 381(9861):166–175. doi: https://doi.org/10.1016/S0140-6736(12)60916-7
- Imahashi K, Pott C, Goldhaber JI, Steenbergen C, Philipson KD, Murphy E. 2005. Cardiac-specific ablation of the Na+-Ca2+ exchanger confers protection against ischemia/reperfusion injury. Circ Res. 97(9):916–921. doi: https://doi.org/10.1161/01.RES.0000187456.06162.cb
- Kaljusto ML, Rutkovsky A, Stenslokken KO, Vaage J. 2010. Postconditioning in mouse hearts is inhibited by blocking the reverse mode of the sodium-calcium exchanger. Interact Cardiovasc Thorac Surg. 10(5):743–748. doi: https://doi.org/10.1510/icvts.2009.217083
- Kaufmann SH, Hengartner MO. 2001. Programmed cell death: alive and well in the new millennium. Trends Cell Biol. 11:526–534. doi: https://doi.org/10.1016/S0962-8924(01)02173-0
- Kavurma MM, Bhindi R, Lowe HC, Chesterman C, Khachigian LM. 2005. Vessel wall apoptosis and atherosclerotic plaque instability. J Thromb Haemost. 3(3):465–472. doi: https://doi.org/10.1111/j.1538-7836.2005.01120.x
- Kloner RA. 1988. Introduction to the role of oxygen radicals in myocardial ischemia and infarction. Free Radic Biol Med. 4(1):5–7. doi: https://doi.org/10.1016/0891-5849(88)90004-4
- Liu C, Hong J, Yang H, Wu J, Ma D, Li D, Lin D, Lai R. 2010. Frog skins keep redox homeostasis by antioxidant peptides with rapid radical scavenging ability. Free Radic Biol Med. 48(9):1173–1181. doi: https://doi.org/10.1016/j.freeradbiomed.2010.01.036
- Matejikova J, Kucharska J, Pinterova M, Pancza D, Ravingerova T. 2009. Protection against ischemia-induced ventricular arrhythmias and myocardial dysfunction conferred by preconditioning in the rat heart: involvement of mitochondrial K(ATP) channels and reactive oxygen species. Physiol Res. 58(1):9–19.
- Murphy E, Steenbergen C. 2008. Mechanisms underlying acute protection from cardiac ischemia-reperfusion injury. Physiol Rev. 88(2):581–609. doi: https://doi.org/10.1152/physrev.00024.2007
- Peng YW, Buller CL, Charpie JR. 2011. Impact of N-acetylcysteine on neonatal cardiomyocyte ischemia-reperfusion injury. Pediatr Res. 70(1):61–66. doi: https://doi.org/10.1203/PDR.0b013e31821b1a92
- Reimer KA, Lowe JE, Rasmussen MM, Jennings RB. 1977. The wavefront phenomenon of ischemic cell death. 1. Myocardial infarct size vs duration of coronary occlusion in dogs. Circulation. 56(5):786–794. doi: https://doi.org/10.1161/01.CIR.56.5.786
- Richard VJ, Murry CE, Jennings RB, Reimer KA. 1990. Oxygen-derived free radicals and postischemic myocardial reperfusion: therapeutic implications. Fundam Clin Pharmacol. 4(1):85–103. doi: https://doi.org/10.1111/j.1472-8206.1990.tb01019.x
- Scofield SLC, Dalal S, Lim KA, Thrasher PR, Daniels CR, Peterson JM, Singh M, Singh K. 2019. Exogenous ubiquitin reduces inflammatory response and preserves myocardial function 3 days post-ischemia-reperfusion injury. Am J Physiol Heart Circ Physiol. 316(3):H617–H628. doi: https://doi.org/10.1152/ajpheart.00654.2018
- Sinning C, Westermann D, Clemmensen P. 2017. Oxidative stress in ischemia and reperfusion: current concepts, novel ideas and future perspectives. Biomark Med. 11(11):11031–11040. doi: https://doi.org/10.2217/bmm-2017-0110
- Yang H, Wang X, Liu X, Wu J, Liu C, Gong W, Zhao Z, Hong J, Lin D, Wang Y, Lai R. 2009. Antioxidant peptidomics reveals novel skin antioxidant system. Mol Cell Proteom. 8:571–583. doi: https://doi.org/10.1074/mcp.M800297-MCP200
- Zheng X, Peng M, Li Y, Wang X, Lu W, Wang X, Shan Y, Li R, Gao L, Qiu C. 2019. Cathelicidin-related antimicrobial peptide protects against cardiac fibrosis in diabetic mice heart by regulating endothelial-mesenchymal transition. Inter J Biol Sci. 15(11):2393–2407. doi: https://doi.org/10.7150/ijbs.35736