Salt-tolerant cell lines of Ailanthus altissima were selected from callus derived protoplasts. Murashige–Skoog (MS) liquid medium incorporated with various concentrations of NaCl was employed to enrich salt-tolerant A. altissima cell lines. Salt-resistant A. altissima cells were transferred on MS solid medium supplemented with 2.5 μM 2,4-dichlorophenoxy acetic acid (2,4-D), 0.5 μM benzyl adenine (BA) and various NaCl concentrations. The callus was cultured on MS medium containing NaCl for 5 months, to determine the survival rate as an index of salt tolerance. The measurement of growth parameters for salt-tolerant cells showed that the selected plant cell lines grew better than the unselected ones at all levels of NaCl tested. The salt-tolerant callus accumulated proline in correlation to the concentration of salts. Media supplemented with BA induced shoot differentiation of salt-resistant A. altissima cells.
Introduction
The biochemical responses of plants to salt stress have been studied intensively. Variant cell lines have been selected from a population of cultured somatic cells of higher plants enduring various stresses (Ben-Hayyim and Kochba Citation1983). Induction of resistance to disease, herbicides, and pesticides through tissue culture has provided potentially useful strains of plants with specific capability to tolerate the test compound (Saxena and Beg Citation1988). Advantages of using plant tissue culture for assessing physiological effects of salt at the cellular level have indicated that the complex organ–organ and plant–environmental interactions can be removed or controlled (Park Citation1984; Peak et al. Citation1988; Winicov et al. Citation1989). The use of plant tissue cultures for the selection of salt-tolerant lines may offer considerable potential provided their regenerative capacity was maintained and inheritable. Although cellular salt tolerance was infrequently lost in culture (Chandler and Vasil Citation1984), the regeneration of a salt-tolerant plant from the selected cells has been difficult. The inheritance of the salt-tolerance trait from NaCl-tolerant cell lines through regeneration, and the level of their resistance remained higher than that of the cell cultures (Nabors et al. Citation1980). Also, Nanakorn et al. (Citation2003) presented that the selected tolerant calli could regenerate into plants that resisted higher levels of NaCl than plants regenerated from non-selected calli. Although the regeneration of Ailanthus altissima derived from callus has been reported (Park and Lee Citation1989), the plant differentiated from NaCl-tolerant plant line has not been reported.
Ailanthus altissima Swingle is a tree that grows worldwide with rapid growth rate and substantial reproductive ability that frequently out-competes native vegetation (Knapp and Canham Citation2000). The species is also described as a characteristic woody pioneer of disturbed sites, being able to grow in hard packed soils and polluted atmospheres (Pan and Bassuk Citation1986). A. altissima can tolerate salts, drought soil alternity but not sodicity (Appleton et al. 1999). The objective of this study was to select of a salt-tolerant A. altissima line and also to regenerate it by in vitro methods.
Materials and methods
Cell culture and conditions
Callus of A. altissima was cultured on Murashige–Skoog (MS) medium (Murashige and Skoog Citation1962) supplemented with 4.5 μM 2,4-dichlorophenoxy acetic acid (2,4-D), 0.5 μM benzyl adenine (BA), and 30 g−1 sucrose. The medium was adjusted to pH 5.8, solidified with 7.5 g−1 agar, and autoclaved at 121°C for 15 minutes. Cultures were maintained under a 16/8 hour (light/dark) photoperiod at 25 ± 1°C in the dark. After establishment of the callus they were subcultured at 4-week intervals on the same media.
Suspension cultures were established by inoculating friable callus (1 g fresh weight) into 100 ml Erlenmeyer flask containing 20 ml MS liquid medium kept on a rotary shaker at a temperature of 25 ± 1°C and 120 rpm.
NaCl was included (0, 0.1, 0.5, 1.0, 1.5, and 2.0%) in the basal MS liquid medium. Cell growth was measured by determining packed cell volume after 25 days. Salt-adapted cells were transferred to solid agar medium supplemented with 4.5 μM 2,4-D, 0.5 μM BA and 30 g− 1 sucrose. After 30 days, the fresh weight (FW) of callus from each treatment was measured. The remaining callus was subcultured on the same medium containing salt to select soft and friable callus.
Determination of salt tolerance of cells
The callus was cultured on salt-supplemented MS medium for 5 months, and survival rate was measured as salt tolerance. Briefly, the callus growth in salt-containing medium was measured after every 3 weeks by determining fresh weight for each individual salt treatment. The dry weight (DW) of the callus was determined after drying to constant weight at 60°C for 24 hours. Each experiment was repeated three times. The selected line was subcultured every 4 weeks in fresh medium supplemented with NaCl.
Estimation of proline
The proline content of the callus was found after its homogenization of fresh callus (0.5 to 1.0 g) in 4 ml methanol:chloroform:water (12:5:1 v/v). To the homogenate a further 1.5 ml of water and 1 ml chloroform were added, mixed thoroughly and centrifuged. Finally, a 0.2 ml aliquot of the upper phase was withdrawn with a micropipette and diluted to 1.0 ml with distilled water. The proline in this extract was determined by the modified spectrophotometric assay of Wren and Wiggall (Citation1965) at 518 nm.
Callus differentiation
Callus differentiation was undertaken on MS medium with or without NaCl and growth regulator BA. After the shoot induction, the regenerating callus was transferred on MS medium with 30 g− 1 sucrose and 2.5 μM BA. When the shoots had reached 2 cm length, they were excised and subcultured on MS medium without growth regulators.
Results
Growth of salt-tolerant callus
Protoplasts derived from callus of A. altissima were inoculated to MS liquid medium supplemented with NaCl. The effect of salt on the cell growth of A. altissima between 0 and 2.0% of NaCl is compared (). NaCl at 0.5% concentration had little influential on cell growth. The higher NaCl (2.0%) levels were however inhibitory and A. altissima cells stopped growing.
Suspended A. altissima cells for each treatments were plated on MS solid medium with NaCl (0, 0.1, 0.5, 1.0, 1.5, and 2.0%), 4.5 μM 2,4-D and 0.5 μM BA. After 30 days of culturing, the callus was formed on the solid media. An excess of 1.0% NaCl in the medium had a potent effect on the A. altissima cell growth. Also cells remained compact even after being subcultured for 25 days. The high salt concentration in general turned the A. altissima callus spongy with reduced growth rate.
Growth rate of salt-tolerant cells
The growth and survival rates of the salt-tolerant cell line of A. altissima are given in . As the levels of NaCl increased, the survival rate of callus decreased. In general, all NaCl treatments led to a decrease in cell growth rate. In the control experiments the cells did not survive above 1.0% NaCl.
Table 1. Growth and survival rate of salt-selected A. altissima callus.
The A. altissima callus growth decreased with the increasing of NaCl strength (). Salt below 0.5% NaCl had a negative influence and at above 1.0% NaCl the growth was severely inhibited. The exposure of callus to 1.0% NaCl did not influence the growth over the entire culture period.
Salt-tolerance of selected and unselected cells was compared (). The salt-tolerant cells had better growth rates than the unselected cells. Fresh weight of A. altissima declined with increasing levels of NaCl.
Proline content of salt-tolerant callus
The proline accumulation patterns of. A. altissima callus in response to NaCl are shown in . NaCl-free medium had a low concentration of proline. However, the callus that was cultured in medium containing salt induced proline accumulation. Proline accumulations showed a linear increase with increased levels of NaCl in the growth medium, 1.0% of NaCl being especially effective.
Differentiation of callus
The differentiation of salt-tolerant A. altissima callus was carried out in vitro (). During the subculture on shoot regeneration medium, a large proportion of callus ceased growing and turned brown. Shoot multiplication of regenerants was promoted on MS medium supplemented with BA. Among the various concentrations of BA, 2.5 μM of BA effectively induced organogenesis (). The shoot numbers from callus regenerants in a medium with high-concentration NaCl were lower than those in a medium without salt. Plant regeneration was achieved when A. altissima callus was cultured in a growth medium containing 0.5% NaCl.
Figure 5. Organogenesis in salt-tolerant A. altissima callus line. A: further growth of shoot primordial. B: development of a micro-shoot.
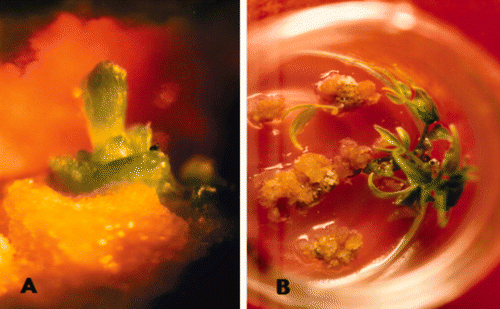
Table 2. Regeneration of salt-tolerant A. altissima callus.
Discussion
A. altissima protoplast-derived callus showed better growth on a MS medium supplemented with 4.5 μM 2,4-D and 0.5 μM BA. This callus of this type will be supported with a homogeneous cell line rather than original callus derived from cambium of A. altissima (Lee and Park Citation1989), thus being prevented from occurrence of fluctuation during the selection process.
Jungaklang et al. (Citation2004) reported that the fresh weight of the shoots was reduced with increasing salt strength. As shown in , the growth of A. altissima suspended cells decreased with the increasing of NaCl. These results are in agreement with those reported by Ben-Hayyim (Citation1987). In addition, the elevated salt levels produce water deficit, ion toxicity, nutrient imbalance, and oxidative stress (Serrano and Gaxiola Citation1994; Niu et al. Citation1995; Serrano Citation1996; Blumwald Citation2000; Zhu Citation2001).
The A. altissima callus formation from suspended cells was achieved on salt containing MS agar medium. This appears to make the selection process more effective and seems to select for NaCl tolerance. Some cell lines have been developed in which the salt tolerance character was reported to be stable, after growth for a number of passages in the absence of salt (Bressan et al. Citation1985, Citation1987; Watad et al. Citation1985). The salt tolerance trait was found to be stable at the cellular level in the cells derived from plants regenerated from the NaCl-tolerant cell line (Watad et al. Citation1991).
Suspension derived from callus grown in the presence of an elevated salt concentration progressively reduced the growth regardless of the salt type. A similar result has been also reported in Nicotiana tabacum (Pua and Thorpe Citation1986; Chandler and Thorpe Citation1987). Cells in suspension culture derived from plants regenerated from salt-tolerant cells exhibit a higher degree of salt tolerance than wild-type cells. This difference in response was not masked by the variation due to the growth cycle stage (Bressan et al. Citation1987). The plant cells may be accumulating toxic ions in response to high concentrations of salt. Ben-Hayyim (Citation1987) and Walker et al. (Citation1983) reported that toxic effect is due to the accumulated of Cl− or Na+ . Flowers and Yeo (Citation1992) and Marschner (Citation1995) report that high NaCl concentrations affect growth because of the influence of low water potentials and the high levels of Na+ and Cl− on cell elongation, carbon/energy metabolism, water relations and nutrient uptake and allocation.
In this study, the A. altissima callus, even after being maintained on salt medium for 5 months, exhibited highest cell growth. But the effect of unselected and selected callus was not significantly different with respect to fresh weight. However, survival of callus in NaCl media was different for subcultures. Chandler and Vasil (Citation1984) reported that tolerant callus was able to grow and tolerate excess ions; these regulatory processes are however accompanied by other physiological and/or biochemical changes.
Proline accumulation in plant cells exposed to salt or water stress is a widespread phenomenon. Proline contents in tolerant A. altissima callus showed linear increases on high salt media. In similar results, levels of cations and proline were closely correlated with the concentration of Na+ in the medium (Peak et al. Citation1988).
The callus of A. altissima retained their organogenic potential even after drastic NaCl exposure. The higher tolerance is related to microglobular callus development. Reina et al. (Citation1988) concluded that NaCl tolerance in Lycopersicon esculentum was related to the meristemic status of the cells.
Plant differentiation from NaCl-tolerant callus has been achieved on MS medium containing 0.5% NaCl. This response was lower than that of rice (Kim et al. Citation1988) and tomato (Rahman and Kaul Citation1989) with respect to NaCl concentrations. Garcia-Reina et al. (Citation1988) have reported that excess of NaCl in the medium over 5.8 g− 1 had strong effect on the variability and organogenic potential of the callus. This result may be characteristic of the particular tree species.
Korea also experienced the similar exploitative era of wetland dominated by agriculture, residence, and industry before the 1990s, and then after suffering from conflicts arising from large reclamation projects such as Sihwa and Saemangum areas from the early 1990s to 2005, also began the era of transition toward ecological focus with efforts to introduce a new wetland management system as in developed countries.
Conclusion
Ailanthus altissima salt-tolerant cell lines were selected by employing a salt-rich medium. The experimental observations indicate that the selected salt-tolerant line is a true genetic variant that has acquired a stable trait through a prolonged procedure of passages on a medium containing salt. Further investigation on the tolerant line is required to accelerate its growth rate at higher salt concentrations. The identification of the phenotype and genotype variations for the regenerated plants adapted to high salt conditions also remains to be established.
Acknowledgements
This study was carried out with the support of Forest Science & Technology Projects (Project No. S211011L20120) provided by Korea Forest Service and the construction of the basis for practical application of herbal resources funded by the Korea Institute of Oriental Medicine (KIOM) to the Ministry of Education, Science, and Technology (MEST) in Republic of Korea.
References
- Appleton , CC , Maurihungirire , M and Gouws , E. 1999 . The distribution of helminth infections along the coastal plain of Kwazulu-Natal province, South Africa . Ann Trop Med Parasitol , 93 : 859 – 868 .
- Ben-Hayyim , G and Kochba , J . 1983 . Aspects of salts tolerance in a NaCl selected stable cell line of Citrus sinensis . Plant Physiol , 72 : 685 – 690 .
- Ben-Hayyim , G . 1987 . Relationship between salt-tolerance and resistance to polyethylene glycol-induced water stress in cultured citrus cells . Plant Physiol , 85 : 430 – 433 .
- Blumwald , E . 2000 . Sodium transport and salt tolerance in plants . Curr Opin Cell Biol , 12 : 431 – 434 .
- Bressan , R A , Singh , N K , Handa , A K , Mount , E , Clithero , J and Hasegawa , P M . 1987 . “ Stability of altered genetic expression in cultured plant cells ” . In Drought resistance in plants: physiological and genetic aspects , Edited by: Monti , L and Poru , E . Brussels : CEC .
- Bressan , R A , Singh , N K , Handa , A K , Kononowicz , A and Hasegawa , P M . Stable and unstable tolerance to NaCl in cultured tobacco cells . Plant genetics: Proceedings of ARCO and UCLA Symposia on Plant Biology. 3rd Keystone . Edited by: Freeling , M . pp. 755 – 769 . New York : A.R. Liss .
- Chandler , S F and Vasil , I K . 1984 . Selection and characterization of NaCl tolerant cells from embryogenic cultures of Pennisetum purpureum Schum (Napier grass) . Plant Sci Lett , 37 : 157 – 164 .
- Chandler , S F and Thorpe , T A . 1987 . Characterization of growth, water relations, and proline accumulation in sodium sulfate tolerant callus of Brassica napus L. cv. Wester (Canola) . Plant Physiol , 84 : 106 – 111 .
- Flowers , T J and Yeo , A R . 1992 . Solute transport in plants , London : Chapman and Hall .
- Garcia Reina , G , Moreno , V and Lugue , A . 1988 . Selection for NaCl tolerance in cell culture of three Canary Island Tomato Land Races. I. Recovery of tolerant plantlets from NaCl-tolerant cell strains . J Plant Physiol , 133 : 1 – 6 .
- Jungaklang , J , Sunohara , Y and Matsumoto , H . 2004 . Antioxidative enzymes response to NaCl stress in salt-tolerant Sesbania rostrata . Weed Biol Manage , 4 ( 2 ) : 81 – 85 .
- Kim , Y C , Chung , T Y and Choi , W Y . 1988 . Increased regeneration from NaCl-tolerant callus in rice . Euphytica , 39 : 207 – 212 .
- Knapp , L B and Canham , C D . 2000 . Invasion of an old-growth forest in New York by Ailanthus altissima: Sapling growth and recruitment in canopy gaps . J Torrey Bot Soc , 127 : 307 – 331 .
- Lee , S G and Park , Y G . 1989 . Plant regeneration from cambium callus of Ailanthus altissima Swingle . J Kor For Soc , 78 ( 4 ) : 412 – 418 .
- Marschner , H . 1995 . Mineral nutrition of higher plants , 2nd ed. , London : Academic Press .
- Murashige , T and Skoog , F . 1962 . Revised medium for rapid growth and bioassays with tobacco tissue cultures . Physiol Plant , 15 : 473 – 497 .
- Nabors , M W , Gibbs , S E , Bernstein , C S and Meis , M E . 1980 . NaCl tolerant tobacco plants from cultured cells . Z Pflanzenphysiol , 97 : 13 – 17 .
- Nanakorn , M , Jiamjetjaroon , W , Suwanawong , S , Wongwattana , C and Shim , I S . 2003 . In vitro selection of salt-tolerant cell lines in kallar grass [Diplachne fusca (L.) Beauv] . Weed Biol Manage , 3 ( 1 ) : 49 – 52 .
- Niu , X , Bressan , R A , Hasgawa , P M and Pardo , J M . 1995 . Ion homeostasis in NaCl stress environments . Plant Physiol , 109 : 735 – 742 .
- Pan , E and Bassuk , N . 1986 . Establishment and distribution of Ailanthus altissimain the urban environment . J Environ Hort , 4 : 1 – 4 .
- Park , Y G . 1984 . Variation in salt-tolerance among five species in Genus Pinus by means of in vitro techniques . Kor J Plant Tissue Cult , 11 : 1 – 4 .
- Park , Y G and Lee , S G . 1989 . Plantlet regeneration from protoplasts of Ailanthus altissima Swingle. 8th International Protoplast Symposium . 1991 June 16–20; Uppsala, Sweden. Physiol Plant 82:A43
- Peak , K Y , Chandler , S F and Thorpe , T A . 1988 . Physiological effects of Na2SO4 and NaCl on callus cultures of Brassica campestris (Chinese cabbage) . Physiol Planta , 72 : 160 – 166 .
- Pua , E C and Thorpe , T A . 1986 . The effect of various salts on multiplication of Nicotiana tabaccum L. shoot cultures of different Na2SO4 tolernce . J Plant Physiol , 124 : 465 – 472 .
- Rahman , M M and Kaul , K . 1989 . Differentiation of sodium chloride tolerant cell lines of tomato (Lycopersicon esculentum Mill) cv. Jet star . J Plant Physiol , 133 : 710 – 712 .
- Reina , G G , Moreno , V and Lugue , A . 1988 . Selection for NaCl tolerance in cell cultures of three canary island Tomato land races. I. Recovery of tolerant plantlets from NaCl tolerant cell strains . J Plant Physiol , 133 : 1 – 6 .
- Saxena , R P and Beg , M U . 1988 . Selection of endosulfan-tolerant gram cell line . Plant Cell Tiss Org Cult , 12 : 273 – 277 .
- Serrano , R . 1996 . Salt tolerance in plants and microorganisms; toxicity targets and defense responses . Int Rev Cytol , 165 : 1 – 52 .
- Serrano , R and Gaxiola , R . 1994 . Microbial models and salt stress tolerance in plants . Crit Rev Plant Sci , 13 : 121 – 138 .
- Walker , R R , Torokfalvy , E , Grieve , A M and Prior , L D . 1983 . Water relations and ion concentrations of leaves on salt-stressed citrus plants . J Plant Physiol , 10 : 265 – 277 .
- Watad , A A , Lerner , H R and Reinhold , L . 1985 . Stability of the salt-resistance character in Nicotiana cell lines adapted to grow in high NaCl concentrations . Physiol Veg , 23 : 887 – 894 .
- Watad , A A , Swartzberg , D , Bressan , R A , Izhar , S and Hasegawa , P M . 1991 . Stability salt tolerance at the cell level after regeneration of plants from a salt toleranr tobacco cell line . Physiol Planta , 83 ( 2 ) : 307 – 313 .
- Winicov , I , Waterborg , J H , Harrington , R E and Mecoy , T T . 1989 . Messenger RNA induction in cellular salt tolerance of alfalfa (Medicago sativa) . Plant Cell Rep , 189 ( 8 ) : 6 – 11 .
- Wren , J J and Wiggall , P H . 1965 . An improved colormetic method for determination of proline in the presence of other ninhydrin-positive compounds . Biochem J , 94 : 216 – 220 .
- Zhu , J K . 2001 . Plant salt tolerance . Trends Plant Sci , 6 : 66 – 71 .