Abstract
To initiate embryogenic suspensor mass (ESM) in Pinus densiflora, immature seeds were collected on dates from 31 May to 20 July in 2004, 2005 and 2006, and their embryo developmental stages were checked. In materials from 2004, the highest frequency (0.88%) of ESM initiation was obtained with explants collected on 28 June, and the seeds contained zygotic embryos in the proembryo stage (100%). The highest ESM initiation frequencies were 0.88% (28 June 2004, Suwon), 1.4% (1 July 2005, Suwon), 2.31% (1 July 2005, Anmyeondo) and 0.91% (1 July 2006, Suwon) and all embryos were at the proembryo stage, regardless of seed collection year (2004, 2005 or 2006) or collection location (Suwon or Anmyeondo). Histological analysis from zygotic embryos (proembryos) of seeds, which were harvested at various times or locations, revealed no significant morphological differences at various developmental stages. These results show that seed collection time could be critical for obtaining the higher rate of ESM initiation.
Introduction
The Japanese red pine (Pinus densiflora) is an evergreen conifer that grows in Eastern Asia (including Korea) and is primarily used for reforestation and landscaping. In Korea, it is widely cultivated both for timber and ornamental trees. However, a pine wilt disease, caused by the pinewood nematode Bursaphelenchus xylophilus, has attacked the species and spread to the entire country. As this disease is a serious problem in pines, a long-term pine breeding project was initiated in order to select and propagate resistant clones in large numbers. Although many attempts have been made to propagate the clones, the results were not so successful. With advancement of biotechnological techniques, e.g. the micro-propagation of elite selections, somatic embryogenesis (SE) is a more promising technique, since it has the capability to produce an unlimited numbers of propagules (Sutton Citation2002). Embryogenic suspensor mass (ESM) initiation is the most critical step in the application of SE to conifer trees, including the Japanese red pine. Unfortunately, most Pinus species have shown a very low ESM initiation frequency, limiting the number of genotypes that can be regenerated through SE, which may represent a serious constraint to the successful commercial application of this technology.
Becwar et al. Citation(1990) reported that several factors play a role in enhancing ESM in immature zygotic embryos of Pinus species: genotype (Becwar et al. Citation1990), collection time or developmental stage of zygotic embryos (Becwar et al. Citation1990), Phytagel level (Li et al. Citation1998), brassinolide (Pullman et al. Citation2003b), abscisic acid (ABA) and silver nitrate (Pullman et al. Citation2003a) and vitamins B12 and E (Pullman et al. Citation2006). Although various kinds of basal media and plant growth regulator combinations have been effective in the initiation of ESM, the most important factor – collection time/developmental stage of zygotic embryos – has not been studied fully; nor has the optimal collection date for immature seeds that would result in the highest frequency of ESM initiation been determined.
Few reports have been published on SE in P. densiflora (Ishii et al. Citation2001; Taniguchi Citation2001; Maruyama et al. Citation2005; Shoji et al. Citation2006) and they do not provide sufficiently detailed information on the close relationship between embryo developmental stages (shown by histological sections) and collection dates/sites for successful ESM initiation in this species. Therefore, the objective of this study was to determine optimal seed collection dates for the initiation of ESM, by using embryo developmental stages and seed sections.
Materials and methods
Plant material
Immature seeds of Japanese red pine were collected from four trees in the experimental garden at the Korea Forest Research Institute located in Suwon (latitude 37°15′N, longitude 126°57′E), Kyeonggi province, Korea, between 31 May and 20 July in 2004. In 2005, seed collection was performed at Suwon as well as a seed orchard located in Anmyeondo (latitude 36°29′N, longitude 126°23′W) at the Interior Breeding Station of the Korea Forest Research Institute in Chungnam Province, Korea, on 28 June, 1 July and 5 July. In 2006, immature seeds were harvested in Suwon on 28 June, 1 July and 5 July (see ).
Table 1. Collection locations, dates and developmental stages of embryos used for ESM initiation in P. densiflora.
Microscopic observation of zygotic embryo development
Before ESM initiation experiments, 15–20 seeds from each collection date were sampled at random, bisected longitudinally, and the developmental stage of zygotic embryos determined as defined by Owens and Blake Citation(1985) using a stereomicroscope. The developmental stages of the embryos were correlated with collection date/location and used as indicators for the collection of the most responsive explants for ESM initiation.
Additionally, immature seeds from different collection dates were fixed for 3 months in glutaraldehyde (1.5%, Electron Microscopy Sciences) and paraformaldehyde (1.6%, Sigma) in phosphate buffer (0.05 M, Sigma), pH 6.8, under refrigeration (4 °C). Samples were dehydrated at room temperature through a graded series of alcohol, and infiltrated with Historesin (Technovit 7100, Kluzer, Germany) overnight at room temperature. Serial sections (3 μm) were prepared using a rotary microtome with a tungsten-carbide knife, and the sections were floated in water and dried on a hot plate (40 °C). Sections were double stained with 0.1% PAS (periodic acid (Sigma) and Schiff (Electron Microscopy Sciences) and 0.05% Toluidine blue O (Sigma) and examined by brightfield microscopy (Leica D.M.R., Germany).
ESM initiation from zygotic embryos
Seeds extracted from cones were disinfected in 70% (w/v) ethanol for 2 min and NaClO (2%, w/v) for 10 min, followed by five washes with sterile distilled water. The seed coat and nucellus tissue were removed and the intact megagametophytes (containing zygotic embryos) were placed on P6 medium (Teasdale et al. Citation1986) containing full-strength macro- and micro-salts, vitamins, 1.0 g−1 L-glutamine (Sigma) and 30 g−1 sucrose plus 9.0 μM 2,4-Dichlorophenoxyacetic acid and 4.5 μM Benzyladenin, all solidified with 0.2% (w/v) gellan gum (Phytagel, Sigma). The cultures were kept in darkness at 24 ± 1 °C for 8 weeks without transferring to fresh medium. An ESM may extrude from the corrosion cavity of the megagametophyte at the micropylar end of the seed, and the frequency of ESM initiation was recorded after 8 weeks of culture.
Statistical analysis
Data recorded during in vitro culture were subjected to an analysis of variance, and significant differences between means were tested by Duncan's multiple range test at P = 0.05.
Results and discussion
ESM initiation frequency based on collection date
The developmental stages and collection dates were used to determine the optimal stage for ESM initiation, which was dependent on the developmental stage of the embryos at the time of culture. As shown in for the 2004 study, the ESM initiation frequencies for the collection dates of 21 June, 28 June and 5 July were 0.57%, 0.88% and 0.33%, respectively, which were all (100%) in the proembryo stage ( and c, e, g). In 5 July seeds, the frequency of proembryo appearance declined to 70% and showed the lowest frequency of ESM initiation (0.33%). For subsequent collections from 13 July and 20 July, no proembryos were observed, and therefore no ESM was initiated from samples collected on either date (). These results indicated that the proembryo stage was the optimal stage for ESM initiation in this species. The 2.3% initiation rate () was in agreement with those of Pinus densiflora (1.0%) (Ishii et al. Citation2001) and P. sylvestris (Keinonen-Mettala et al. Citation1996), where proembryo to early-stage embryos were collected in late June and early July for ESM experiments. However, these results disagreed with previous reports for P. densiflora (1.4%) (Taniguchi Citation2001) and P. pinaster (Arya et al. Citation2000; Miguel et al. Citation2004), where precotyledonary embryos were collected in mid/late July.
Figure 1. Developmental embryo stages of P. densiflora. (a) Archegonia (arrows) from a 31 May seed. (b) No embryos found in 7 June seeds. (c) Early-stage embryos in corrosion cavity (13 June). (d) Magnified picture of Figure 1c. One small globular zygotic embryo in corrosion cavity, e: embryonal head, s: suspensor, m: micropyle. (e) Proembryos in the corrosion cavity (cc), s: suspensor (21 June). (f) Numerous suspensors in the corrosion cavity (magnified Figure 1e). (g) Late-stage proembryos in corrosion cavity (28 June). (h) Embryonal head of each embryo (arrow) has more divided cells and longer suspensors than shown in those of Figure g. (i) One surviving dominant embryo (arrow); the other embryo has degenerated (5 July). (j) Magnified picture of Figure c. (k) A zygotic embryo developed more vigorously (13 July). (l) Cotyledon-stage embryo in the corrosion cavity (20 July). Bars: 420 μm (a–d), 179 μm (e), 159 μm (i), 140 μm (j), 117 μm (k), and 147 μm (l).
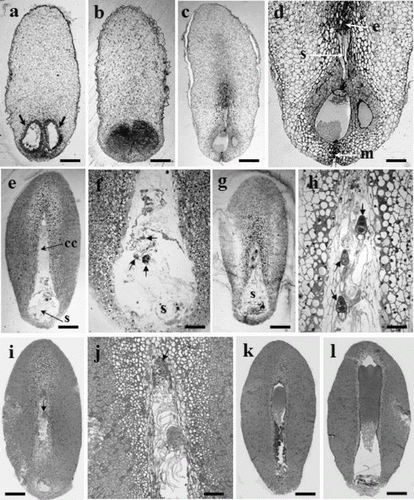
As seeds developed to the precotyledon stage, the frequency of ESM initiation decreased sharply from 0.88% (28 June) to 0.33% (5 July). Over half (66.7%) of the explants from 13 July collection were at the cotyledon stage and the rest were at the globular stage (33.3%) (), and no embryogenic lines could be produced from seeds collected on 13 or 20 July. Based on the results shown in , seeds for this species should be collected before the development of globular-stage embryos.
Collection date for 2005 seeds (three collection dates and one more collection location – Anmyeondo) can be seen in . The highest frequencies of ESM initiation were 1.4% (1 July, Suwon) and 2.31% (1 July, Anmyeondo) and no differences were seen between the two locations (2005) with respect to embryo developmental stage (). In 2006, the highest frequency of ESM initiation was 0.91% (Suwon, 1 July) and corresponded with the proembryo stage (100%). In all cases, the proembryo stage was associated with the highest rate of ESM initiation, even though the seeds were from different years (i.e. 2004, 2005 and 2006) ().
In conclusion, the frequency of ESM initiation was influenced by the original explant developmental stage as demonstrated by the samples from 2004 (). Some attempts have been made to determine the optimal developmental embryo stage for ESM initiation in Pinus nigra (Salajova et al. Citation1999), P. pinaster (Miguel et al. Citation2004), and P. roxburghii (Arya et al. Citation2000).
Development of zygotic embryos in sampling periods
For seeds collected on 31 May 2004 (a), the megagametophytes were translucent with visible archegonia (arrows). No zygotic embryos were observed until the seeds collected on 7 June were examined (b), where the two archegonia were larger than those of seeds collected on 31 May (b). In the seeds from June 13, one early proembryo (resulting from fertilization) was seen in the upper part of one archegonium (c), where the embryonic heads of the zygotic embryos were composed of several cells, their suspensors linked to one archegonium head (c, d) and the other archegonium having started to shrink/degenerate (d). In the seeds from 21 June, some zygotic embryos (arrows) were found with numerous suspensors throughout the corrosion cavity, particularly near the micropylar region (e, f). The size of the corrosion cavity was expanded longitudinally towards the chalazal end (f). From the seeds of 28 June (g), three late proembryos (arrows) (polyembryogenesis) were found in the corrosion cavity (h), one dominant embryo (arrow) had continued to develop and reached the chalazal end, and the others had started to degenerate (5 July) (i, j). By elongation and development of apical shoot primordia, one dominant embryo (k) developed to the precotyledon (13 July) or cotyledon stage (l, 20 July). After 8 weeks in culture, the translucent to white mucilaginous ESM might extrude from the opening at the micropylar end of the megagametophyte (). In seeds from Suwon (2006), some proembryos were found in the corrosion cavity (a). In 2005 (e, f, g, h), regardless of collection time (28 June, 1 July and 5 July), the corrosion cavity in the seeds was expanded longitudinally to the chalazal end and showed numerous suspensors throughout the corrosion cavity (Suwon, a; or Anmyeondo, b). No differences in development (length and shape of corrosion cavity or embryo stage) were found in the 2005 and 2006 seeds with respect to collection date, year or location (a, b). In contrast, when compared with seeds from 2006, the length of the corrosion cavity was a half less developed than those of 2005 seeds (c, 28 June or 1 July, two on the left or right side, respectively), and some embryos with suspensors were restricted to the round-shaped corrosion cavity (c, 5 July). However, even though the zygotic embryogenesis in 2006 seeds lagged behind those of 2005 (), all seeds collected on those dates (i.e. 28 June, 1 July or 5 July in 2005 or 2006) were fully able to initiate ESM with a high frequency ().
Figure 2. Mucilaginous ESM extruded from the micropyle end of megagametophyte after 8 weeks in culture. Bar: 8 mm.
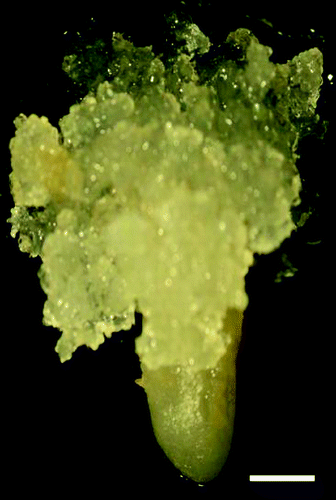
Figure 3. Sections of immature P. densiflora embryos correlated with collection dates and locations. (a) Two embryos on the left (28 June 2005, Suwon), two in middle (1 July 2005, Suwon), two on the right (5 July 2005, Suwon). (b) Two embryos on left (28 June 2005, Anmyeondo), two in middle (1 July 2005, Anmyeondo), two on right (5 July 2005, Anmyeondo) (c) two embryo on left (28 June 2006, Suwon), two in middle (1 July 2006, Suwon), two on right (1 July 2006, Suwon). Bar: 1.2 mm (a–c).
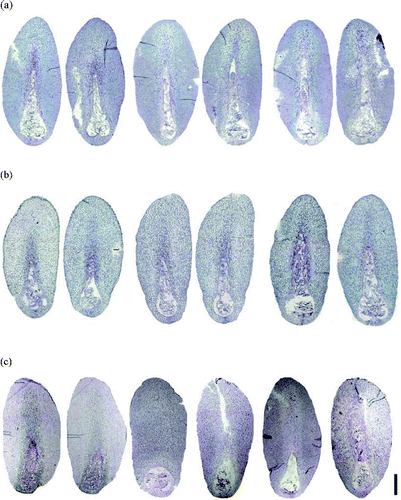
Correlating the developmental stage with season may be ineffective because size and developmental stage differed, even among seeds from a single tree, and variations (likely due to open pollination) can be observed between trees (Arya et al. Citation2000). Even though seed development may vary from year to year by latitude and elevation, histology suggested that the optimum stage of embryo development for ESM initiation could be determined by using sections from different collection dates.
As for other conifer species, the developmental stage of the embryo has proven to be a critical factor for ESM initiation in Pinus densiflora, and was limited to the proembryo stage. Initiation of ESM from a more advanced stage of embryo development has been previously described for the precotyledon stage in P. pinaster (Miguel et al. Citation2004) and P. roxburghii (Arya et al. Citation2000) or even from mature zygotic embryos in P. koraiensis (Bozhkov et al. Citation1997), while in P. densiflora, the highest initiation was obtained from proembryos (). In rare cases, the fertilization date has been used as a reference point (Keinonen-Mettala et al. Citation1996). For P. sylvestris, the appropriate period was about 2 weeks after fertilization. However, the precise time of fertilization is more difficult to determine than the embryo developmental stage and is further complicated by temporal variations in development among different mother trees (Lelu et al. Citation1999).
References
- Arya S, Kalia RK, Arya ID. 2000. Induction of somatic embryogenesis in Pinus roxburghii Sarg. Plant Cell Rep. 19:775–780.
- Becwar MR, Nagmani R, Wann SR. 1990. Initiation of embryogenic cultures and somatic development in loblolly pine (Pinus taeda). Can J For Res. 20:810–817.
- Bozhkov PV, Ahn JS, Park YG. 1997. Two alternative pathways of somatic embryo origin from polyembryonic mature store seeds of Pinus koraiensis Sieb et Zucc. Can J Bot. 75: 509–512.
- Ishii K, Maruyama E, Hosoi Y. 2001. Somatic embryogenesis of Japanese conifers. In: Morohoshi N, Komamine A, editors. Molecular breeding of woody plants. Amsterdam, The Netherlands, Elsevier Science BV 297–304.
- Keinonen-Mettala K, Jalonen P, Eurola P, von Arnold S, von Weissenberg K. 1996. Somatic embryogenesis of Pinus sylvestris. Scand J For Res. 11:242–250.
- Lelu MA, Bastien C, Drugeault A, Gouez M, Klimaszewska K. 1999. Somatic embryogenesis and plantlet development in Pinus sylvestris and Pinus pinaster on medium with and without growth regulators. Physiol Plant. 105:719–728.
- Li Y, Huang FH, Gbur Jr EE. 1998. Effect of basal medium, growth regulators and phytagel concentration on initiation of embryogenic cultures from immature zygotic embryos of loblolly pine (Pinus taeda L.). Plant Cell Rep. 17:298–301.
- Maruyama E, Hosoi Y, Ishii K. 2005. Propagation of Japanese red pine (Pinus densiflora Zieb. et Zucc.) via somatic embryogenesis. Prop Ornam Plants. 5:199–204.
- Miguel C, Goncalves S, Tereso S, Marum L, Maroco J, Oliveira MM. 2004. Somatic embryogenesis from 20 open-pollinated families of Portuguese plus trees of maritime pine. Plant Cell Tiss Org Cult. 76:121–130.
- Owens JN, Blake MD. 1985. Forest tree seed production. In: Petawawa National Forestry Institute, Information Report PI-X-53. Chalk River, Ont, Canada: Petawawa National Forestry Institute; p. 161, ISBN 0-662-14485-7.
- Pullman GS, Chopra R, Chase, KM. 2006. Loblolly pine (Pinus taeda L.) somatic embryogenesis: Improvement in embryogenic tissue initiation by supplementation of medium with organic acids, Vitamins B12 and E. Plant Sci. 170:648–658.
- Pullman GS, Namjoshi K, Zhang Y. 2003a. Somatic embryogenesis in loblolly pine (Pinus taeda L.): improving culture initiation with abscisic acid and silver nitrate. Plant Cell Rep. 22:85–95.
- Pullman GS, Zhang Y, Phan BH. 2003b. Brassinolide improves embryogenic tissue initiation in conifers and rice. Plant Cell Rep. 22:96–104.
- Salajova T, Salaj J, Kormutak A. 1999. Initiation of embryogenic tissue and plantlet regeneration from somatic embryos of Pinus nigra Arn. Plant Sci. 145:33–40.
- Shoji M, Sato H, Nakagawa R, Funada R, Kubo T, Ogita S. 2006. Influence of osmotic pressure on somatic embryo maturation in Pinus densiflora. J For Res. 11:449–453.
- Sutton BCS. 2002. Commercial delivery of genetic improvement to conifer plantations using somatic embryogenesis. Ann For Sci. 59:657–661.
- Taniguchi T. 2001. Plant regeneration from somatic embryos in Pinus thunbergii (Japanese black pine) and Pinus densiflora (Japanese red pine). In: Morohoshi N, Komamine A, editors. Molecular breeding of woody plants. Amsterdam, The Netherlands, Elsevier Science BV 319–324.
- Teasdale RD, Dawson PA, Woolhouse, HW. 1986. Mineral nutrient requirements of a loblolly pine (Pinus taeda) cell suspension culture. Plant Physiol. 82:942–945.