Abstract
The present work aimed to study the physiological effects of mixed heavy metals, cadmium (Cd), copper (Cu), chromium (Cr), and zinc (Zn) in four poplar hybrids. Poplar hybrids were treated once with six different concentrations (0, 5, 50, 100, 200, and 500 ppm) of mixed heavy metals for a period of 3 months. The photosynthetic and transpiration rates were measured before and during treatment using an LCi–SD portable photosynthesis system whereas the photosynthetic pigments were analytically determined. According to the results obtained, poplar hybrids exhibited a decrease in chlorophyll content and carotenoids except for hybrid 1 (Eco 28) which showed an increase at 200 and 500 ppm heavy metal concentrations. The photosynthetic rate decreased with increasing heavy metal concentrations; however, an increase was observed at the highest concentration of 500 ppm at 14.54 µmol m−2 s−1. Transpiration rates were significantly affected by increased heavy metals. Hybrid 1 (Eco 28) was deduced as the hybrid that has the ability to tolerate high levels of mixed heavy metals. Among the four poplar hybrids tested, hybrid 1 (Eco 28) was the best candidate for phytoremediative experiments under greenhouse conditions. These results are valuable in understanding how various tree hybrids respond to mixed heavy metal stress, especially when using them as bio indicators for phytoremediation experiments.
Introduction
Heavy metal contamination of the environment from various sources, including industry and agriculture, is an area of concern around the world. Although heavy metals are natural components in soils at trace levels, various anthropogenic activities such as increased environmental pollution from industry, agriculture, and mining have contributed to undesirable toxic accumulations of heavy metals.
Phytoremediation is a cleanup technology for metal contaminated soils. Some model phytoremediators include various varieties of trees; examples include cottonwood, poplar, and willows. Trees are ideal in the remediation of heavy metals because they can withstand higher concentrations of pollutants due to their large biomass (Paz-Alberto and Sigua Citation2013). They have the ability to accumulate large amounts of the contaminants in their systems because of their size and extensive roots systems. Furthermore, trees have the ability to stabilize an area, prevent erosion, and minimize the spread of the contaminants. They can be easily harvested and removed from the area with minimal risk, allowing the effective removal of contaminants (Paz-Alberto and Sigua Citation2013).
Some heavy metals such as copper (Cu), zinc (Zn), cobalt (Co), and iron (Fe) are essential in trace amounts for various metabolic activities in plants. However, excess of any kind of metal (essential or non-essential) adversely affects plant metabolism (Hall Citation2002). In plants, metals exert their toxic action mostly by damaging chloroplasts and disturbing photosynthesis. Inhibition of photosynthesis is the consequence of interference of metal ions with photosynthetic enzymes and chloroplast membranes (Aggarwal et al. Citation2012). In higher plants, photosynthesis is indirectly reduced by heavy metal accumulation in leaves which influences the functioning of the stomata and hence affects photosynthesis and transpiration rates overall. Photosynthetic pigment reduction by heavy metals affects photosynthesis indirectly, hence the use of non-destructive methods and the ease of measurement allows photosynthetic pigments to be frequently used to determine stress for regulatory purposes (Aggarwal et al. Citation2012).
The sensitivity of plants to heavy metals depends on an interrelated network of physiological and molecular mechanisms such as: (i) uptake and accumulation of metals through binding of extracellular exudates and cell wall constituents; (ii) efflux of heavy metals from cytoplasm to extra nuclear compartments including vacuoles; (iii) complexation of heavy metal ions inside the cell of various substances, for example, organic acids, amino acids, phytochelatins, and metallothioneins; (iv) accumulation of osmolytes and osmoproteins and induction of antioxidative enzymes; and (v) activation or modification of plant metabolism to allow adequate functioning of metabolic pathways and rapid repair of damaged cell structures (Cho et al. Citation2003).
Various poplar genotypes have been used to determine their responses at physiological and molecular levels in different heavy metals. Sebastiani et al. (Citation2004) looked at the effects of heavy metal (Zn, Cu, chromium [Cr], and cadmium [Cd]) enriched organic waste on biomass portioning and heavy metal accumulation in plant organs in two poplar clones (Populus deltoids x maximowiczii – clone Eridano and P. x euramericana – clone I-214). Gaudet et al. (Citation2011) compared the physiological and molecular responses of Cd stress in two Populus nigra L. genotypes originating from contrasting environments. Robinson et al. (Citation2007) investigated the potential of hybrid poplar for boron (B) phytomanagement using a lysimeter experiment and a field trial on B contaminated wood waste. Zacchini et al. (Citation2011) studied the effects of Cd accumulation and tolerance in P. nigra and Salix alba. Despite numerous studies, the specific mechanisms involved in heavy metal accumulation and detoxification are yet to be well described. Characterization of these mechanisms could be important in improving the potential of Salicaceae plants for phytoremediation.
The main aims of this study were to use non-destructive and easy access parameters to determine the effects of mixed heavy metals (Cd, Cr, Cu, and Zn) stress, under greenhouse conditions, on: (i) leaf chlorophyll a (chl a), chlorophyll b (chl b), total chlorophyll, chlorophyll a/b ratio, and carotenoids; and (ii) photosynthesis and transpiration rates.
Materials and methods
Plant materials and experimental conditions
The experiment was conducted under greenhouse conditions in Ilsan, located northwest of Seoul, South Korea during the months of April–September 2014. Four Populus hybrids were selected based on genetic diversity, growth, and wellbeing in early spring 2014. The details of the clones are provided in . Cuttings of approximately 15 cm lengths from the four hybrids were planted in 2 L pots filled with sandy loam soil in which the original plants were growing in the field. After 2 months to allow sprouting and roots to stabilize, the plantlets were treated with heavy metals of various concentrations ranging from 0 ppm as control followed by 5, 50, 100, 200, and 500 ppm. Mixed heavy metals utilized were chromium III chloride (CrCl3), copper (II) chloride (CuCl2), cadmium chloride (CdCl2), and zinc chloride (ZnCl2). Each heavy metal was prepared separately and equal amounts of the same concentrations were mixed for treatment. Plantlets were treated with 20 ml of mixed heavy metal per plantlet once only. Plantlets were regularly watered over the 3 month treatment period. The treated experimental pots were set up according to a complete randomized block design (CRBD). Each treatment was replicated six times and appeared once in each block.
Table 1. Poplar hybrids selected for greenhouse phytoremediative experiment.
Photosynthesis and transpiration rates
An LCi–SD (ADC Bioscientific Ltd., Hoddesdon, UK) portable photosynthesis system was used to measure the photosynthetic and transpiration rates of the plants, which were recorded before treatment and 2 months after treatment to determine any changes that might occur over time.
Photosynthetic pigments
To estimate photosynthetic pigments chl a, chl b, and carotenoids, plant materials (leaves) were harvested from the greenhouse and 0.1 g was placed in 8 ml of 80% acetone for 7 days under dark conditions in the refrigerator. After 7 days absorbances were taken with UV/VIS spectrophotometer at 663, 645, and 470 nm (Skanlt software 3.2 for Multiskan Co., Thermo Fisher Scientific, Waltham, MA, USA). Chlorophyll content was estimated according to Arnon (Citation1949) and carotenoid content according to Lichtenthaler (Citation1987).
Statistical analysis
Collected data are expressed as mean ± SEM. All data were analysed by the Holm-Sidak ANOVA multiple comparison statistics using GraphPad Prim 6.0 software. Statistical significance was accepted at p ˂ 0.05.
Results
Photosynthesis and transpiration rates
The exposure of mixed heavy metals to poplar hybrids under greenhouse conditions resulted in an increase in photosynthetic rate with increase in heavy metal concentrations (). Hybrid 1 had the highest photosynthetic rate of 14.54 µmol m−2 s−1 at 500 ppm of mixed heavy metal concentration, being the highest photosynthetic rate among all four hybrids. No other significant differences were observed among other heavy metal concentrations for hybrid 1. For hybrid 2, fluctuations in photosynthetic rate were observed across the concentrations; however, the lowest photosynthetic rate of 7.75 µmol m−2 s−1 was observed at 5 ppm mixed heavy metal concentration and the highest of 12.60 µmol m−2 s−1 at 200 ppm. Hybrid 3 had the lowest photosynthetic rate of 2.61 µmol m−2 s−1 at 200 ppm which increased 5 times to 10.08 at 500 ppm. However, hybrid 4 had a significantly higher photosynthetic rate at 5 ppm of 8.20 µmol m−2 s−1 which decreased to 6.84 and 7.23 for 50 and 100 ppm, respectively. A clear pattern can be observed for all four poplar hybrids with the photosynthetic rate increasing with increased mixed heavy metal concentrations, the majority being significant around 200–500 ppm mixed heavy metal concentrations.
Table 2. Photosynthetic rates of four poplar hybrids under mixed heavy metal (Cd, Cr, Cu, and Zn) treatment in greenhouse conditions.
Transpiration rates varied across the hybrids, the highest value coinciding with 50 ppm for hybrid 1 at 11.23 mmol m−2 s−1 followed by 500 ppm at 8.89 mmol m−2 s−1 (). Heavy metal concentrations of 5 ppm and 200 ppm had significant transpiration rates when compared to control (0 ppm). Hybrid 2 had the highest transpiration rate at 9.99 mmol m−2 s−1 at 200 ppm followed by 9.61 at 50 ppm. Transpiration rates significantly different from control were at the low mixed heavy metal concentrations of 5 ppm and 100 ppm. The transpiration rates of hybrid 3 were most significant at all concentrations. The highest of 10.87 mmol m−2 s−1 at 50 ppm followed by 10.14 mmol m−2 s−1 at 100 ppm and the lowest of 3.42 mmol m−2 s−1 at 200 ppm. The lowest transpiration rates were observed in hybrid 4 where the apparent lowest transpiration rate was at 50 ppm (3.55 mmol m−2 s−1) and the highest was at 200 ppm (8.14 mmol m−2 s−1). No specific relationship was observed for transpiration rates across the different mixed heavy metal concentrations in hybrid 4. Significant transpiration rates were observed across all four poplar hybrids. However, the differences varied for each hybrid; in general, a decrease in transpiration rate was observed with an increase in mixed heavy metal concentrations.
Table 3. Transpiration rates of four poplar hybrids under mixed heavy metal (Cd, Cr, Cu, and Zn) treatment in greenhouse conditions.
Photosynthetic pigments
The exposure of poplar hybrids to heavy metals for phytoremediation greenhouse experiments overall resulted in significant reductions in chlorophyll and carotenoid contents in leaves (). The chlorophyll content in hybrid 1 showed a general decreasing trend for chl a, chl b, and total chlorophyll, with increasing concentrations of heavy metals up to 200 ppm after which the chlorophyll contents increased. The initial decrease in pigment contents chl a and total chlorophyll were highly significant. However, the carotenoid contents decreased with increasing heavy metal concentrations and at 500 ppm increased dramatically to 3.17 mg/g, although the increase was not significant. In hybrid 2 and hybrid 3 the chl a, chl b, and total chlorophyll contents, as well as carotenoid contents, decreased as compared to control. This suggests that the various concentrations of heavy metals affect the pigments contents. The most significant change in leaf chl a, chl b, and carotenoid contents was observed in hybrid 4 at 500 ppm of 9.00 mg/g, 3.71 mg/g and 1.45 mg/g respectively, being the lowest significant values across all hybrids. Overall, slight decreases in chl a and b were observed; however, 500 ppm heavy metal concentration had the lowest photosynthetic pigments in hybrid 4.
Table 4. Mixed heavy metal (Cd, Cr, Cu, and Zn) treatment effects on photosynthetic pigment concentrations in four poplar hybrids.
There were no significant changes in chlorophyll a/b ratio across all concentrations in all four hybrids (results not shown). An overall decrease in carotenoid content was observed for all poplar hybrids; however, no significant changes were observed in leaf carotenoid contents in all hybrids across all heavy metal concentrations.
Discussion
Heavy metal stress alters many physiological and metabolic processes in plants. The data presented demonstrate that mixed heavy metal exposure leads to significant decreases in photosynthetic pigments in poplar hybrids. Chlorophyll content is often measured in plants to assess the impact of environmental stress, as changes in pigment content are linked to visual symptoms of plant illness and photosynthetic productivity (Parekh et al. Citation1990).
In the present investigation, the photosynthetic rates decreased for all poplar hybrids across all heavy metal concentrations; however, only hybrid 1 (Eco 28) had a high photosynthetic rate at 500 ppm (). Decline in photosynthetic rate has been exhibited in other plants, due to the reduction in photosynthetic pigments by the heavy metals. Heavy metals such as mercury (Hg), Cu, Cr, Cd, and Zn have been found to decrease the chlorophyll content in various plants in most cases (Aggarwal et al. Citation2012). This decline in photosynthetic pigments is most probably due to the inhibition of the reductive steps in the biosynthetic pathways of photosynthetic pigments due to the high redox potential of many heavy metals. In addition, the key enzyme, protochlorophyllide reductase, which is involved in the reduction of protochlorophyll to chlorophyll is well known to be inhibited by heavy metals (De Filippis and Pallaghy Citation1994). Various authors have reported similar decreases in chlorophyll content under heavy metal stress in cyanobacteria, unicellular chlorophytes (Chlorella), gymnosperms such as Picea abies and angiosperms, such as Zea mays, Quercus palustrus and Acer rubrum, sunflower as well as almond (Siedlecks and Krupa Citation1996; Zengin and Munzuroglu Citation2006; Elloumi et al. Citation2007). A few reports show an enhancement of pigments after exposure to heavy metals (Devi Prasad and Devi Prasad Citation1982).
Figure 1. Effects of mixed heavy metal treatment on photosynthetic rate of four poplar hybrids under greenhouse conditions.
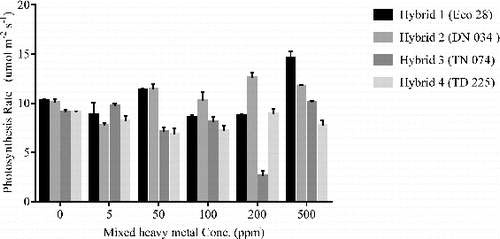
Figure 2. Effects of mixed heavy metal treatment on transpiration rate of four poplar hybrids under greenhouse conditions.
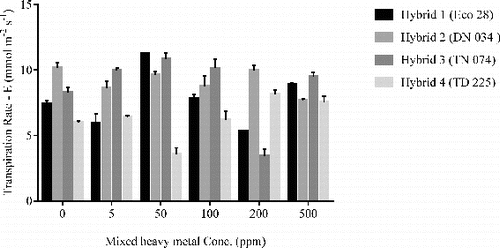
Figure 3. Effects of mixed heavy metals on chl a, chl b, total chlorophyll (a+b), and carotenoid contents in leaves of the four poplar hybrids at different heavy metal concentrations. Values are mean ± SEM, significance at p ˂ 0.05 (n = 4).
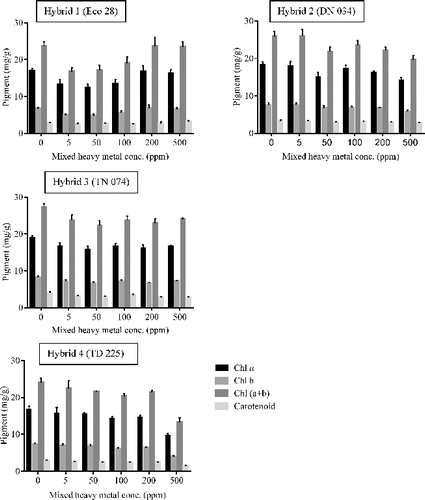
The contradictory segments of the data are probably due to the effects of mixed heavy metals, which compete with each other in the soil–water medium. Uptake by the roots and their levels of accumulation in the leaves vary depending on the hybrid, hence showing an overall expected decline in hybrids 2, 3, and 4. Whereas hybrid 1 had the highest photosynthetic rate, decreases for 5, 50, and 100 ppm were observed when compared to control; however, an increase higher than control is observed at 500 ppm heavy metal concentration. The significant increase in photosynthetic and transpiration rates () for hybrid 1 are also supported by the increase in photosynthetic pigments (). The overall biological accumulation coefficient of heavy metals in the plant would be a contributing factor in terms of explaining how hybrid 1 overall responds to high concentrations of mixed heavy metals. This points to the need for a better understanding of the form of heavy metal ions in the soil solution, their interaction with the plant roots, their eventual absorption into the system, and, finally, their translocation to aboveground parts.
Various authors have studied the effects of single heavy metals on different plant species. The effects of Cd and lead (Pb) on Brassica juncea L. exhibited a decline in growth, chlorophyll content, and carotenoids; however Cd was found to be more detrimental than Pb (John et al. Citation2009). According to the study of physiological effects of Cd and Cu on pea (Pisum sativum), photosynthetic pigments and photosynthetic rates declined at all concentrations of Cd and at high Cu concentrations (Hattab et al. Citation2009).
Heavy metals such as Cu and Zn are essential for normal plant growth and development since they are constituents of many enzymes and other proteins. However, elevated concentrations of any heavy metal either essential or non-essential can lead to toxic symptoms and growth inhibition in most plants. This decrease in plant photosynthetic efficiency could be partly responsible for the overall decrease in plant growth and biomass production.
Conclusion
In conclusion, it can be deduced that the short term exposure of poplar hybrids to mixed heavy metals does seriously affect the overall photosynthetic pigments and photosynthesis, as investigated by the studied parameters. This confirms that the use of photosynthetic parameters provide helpful information on plant response to mixed heavy metal exposure. Hybrid 1 (Eco 28), which is a local hybrid, had higher chl a, carotenoid content, and photosynthetic rate at the highest mixed heavy metal concentration of 500 ppm, which probably suggests that further investigation needs to be conducted with this hybrid in order to understand the mechanisms involved that could make it a suitable candidate for phytoremediative field work. These results, together with the fact that Populus euramericana hybrids have been found to be suitable candidates for earlier phytoremediative work suggests that there are probably certain genetic factors that contribute to higher tolerance of P. euramericana hybrids when compared to other poplar hybrids.
Disclosure statement
No potential conflict of interest was reported by the authors.
References
- Aggarwal A, Sharma I, Tripati BN, Munjal AK, Baunthiyal M, Sharma V. 2012. Metal toxicity and photosynthesis. In: Photosynthesis: overviews on recent progress & future perspectives. 1st ed. New Delhi: I K International Publishing House Pvt. Ltd; p. 229–236.
- Arnon DI. 1949. Copper enzymes in isolated chloroplast, polyphenol oxidase in Beta vulgaris. Plant Physiol. 24:1–15.
- Cho M, Chardonnes AN, Dietz KJ. 2003. Differential heavy metal tolerance of Arabidopsis halleri and Arabidopsis thaliana: a leaf slice test. New Phytol. 158:287–293.
- De Filippis LF, Pallaghy CK. 1994. Heavy metals: sources and biological effects. Stuttgart: E. Schweizerbarts'sche, Verlagsbuchhandlung.
- Devi Prasad PV, Devi Prasad PS. 1982. Effects of cadmium, lead and nickel on three freshwater green algae. Water Air Soil Poll. 17:263–268.
- Elloumi N, Ben F, Rhouma A, Ben B, Mezghani I, Boukhris M. 2007. Cadmium-induced growth inhibition and alteration of biochemical parameters in almond seedlings grown in solution culture. Acta Physiologiae Plantarum 29:57–62.
- Gaudet M, Pietrini F, Beritognolo I, Iori V, Zacchini M. 2011. Intraspecific variation of physiological and molecular response to cadmium stress in Populus nigra L. Tree Physiol. 31:1309–1318.
- Hall JL. 2002. Cellular mechanisms for heavy metal detoxification and tolerance. J Exp Bot. 53:1–11.
- Hattab S, Dridi B, Chouba L, Kheder MB, Bousetta H. 2009. Photosynthesis and growth responses of Pisum sativum L. under heavy metal stress. J Env Sci. 2: 1552–1556.
- John R, Ahmad P, Gadgil K, Sharma S. 2009. Heavy metal toxicity: effect on plant growth, biochemical parameters and metal accumulation by Brassica juncea L. Int J Plant Prod. 3:65–75.
- Lichtenthaler HK. 1987. Chlorophylls and carotenoids: pigments of photosynthetic biomembranes. Method Enzymol. 148:350–382.
- Parekh D, Puranik RM, Srivastava HS. 1990. Inhibition of chlorophyll biosynthesis by cadmium in greening maize leaf segments. Biochemie Physiologie der Pflanzen 186:239–242.
- Paz-Alberto AM, Sigua GC. 2013. Phytoremediation: a green technology to remove environmental pollutants. Am J Clim Change 2:71–86.
- Robinson BH, Green SR, Chancerel B, Mills TM, Clothier BE. 2007. Poplar for the phytomanagement of boron contaminated sites. Environ Pollut. 150:225–233.
- Sebastiani L, Scebba F, Tognetti R. 2004. Heavy metal accumulation and growth responses in poplar clones Eridano (Populus deltoides x maximowiczii) and I-214 (P. euramericana) exposed to industrial waste. Environ. Exp Bot. 52:79–88.
- Siedlecks A, Krupa Z. 1996. Interactions between cadmium and iron and its effects on photosynthetic capacity of primary leaves of Phaseolus vulgaris. Plant Physiol. 34:833–841.
- Zacchini M, Iori V, Mugnozza GS, Pietrini F, Massacci A. 2011. Cadmium accumulation and tolerance in Populus nigra and Salix alba. Biologia Plantarium 55:383–386.
- Zengin FK, Munzuroglu O. 2006. Toxic effects of cadmium (Cd++) on metabolism of sunflower (Helianthus annuus L.) seedlings. Acta Agriculturae Scandinavica, Section B: Soil & Plant Science 56:224–229.