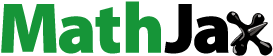
Abstract
Physiological responses of 1-year-old Pinus densiflora and Larix kaempferi seedlings were measured under open-field warming and precipitation manipulation. Air temperature of warming plots was 3 °C higher, while precipitation manipulation plots received ±40% of the precipitation than control plots. Seedlings were planted in May, and temperature and precipitation were manipulated from June 2017. Total chlorophyll content (Chlt), net photosynthetic rate (Pn), transpiration rate (E), and stomatal conductance (gs) were measured between July and September 2017. For P. densiflora and L. kaempferi, Chlt increased by 11.75% and 11.64%, and Pn decreased by 9.14% and 2.17% under warming, respectively. E and gs were lower under warming in P. densiflora, but higher in L. kaempferi. The lower Pn in P. densiflora resulted from stomatal closure, while that of L. kaempferi resulted from reduced vitality. Lower precipitation increased Chlt and Pn by 11.64% and 2.66% for P. densiflora, and by 6.40% and 4.32% for L. kaempferi, respectively. Conversely, higher precipitation decreased Pn of P. densiflora by 5.72%, and decreased Chlt and Pn of L. kaempferi by 8.24% and 4.55%, respectively. These results can be attributed to concentrated precipitation. In this study, two species responded differently even when they were exposed to the same environmental conditions, and this was due to the species-specific mechanisms to water stress derived from the high temperature.
Introduction
According to the Intergovernmental Panel on Climate Change (IPCC), the climate is clearly changing, and the change is being observed across the world (IPCC Citation2014). The IPCC predicts a global increase in temperature, and either an increase or decrease in precipitation, depending on the region (IPCC Citation2014). These changes are expected to modify terrestrial ecosystems (Solomon et al. Citation2007). It is predicted that climate change will particularly affect forest ecosystems, including the energy flow, carbon and water circulation, and vegetation dynamics of forest ecosystems (Dale et al. Citation2001; Bonan Citation2008; Lindner et al. Citation2010).
Climate change affects the physiological characteristics of trees, as these respond noticeably to changes in temperature and precipitation (Hughes Citation2000). Physiological characteristics affect the competitiveness and growth of tree species (Xu et al. Citation2012), which in turn may affect the dynamics of forest ecosystems. Studies on physiology can therefore help identify and predict ecosystem changes due to climate change (Pörtner and Farrell Citation2008; Somero Citation2010).
The responses of trees to climate change varies (Allen et al. Citation2010). In previous studies, physiological variables such as chlorophyll content and photosynthetic rate of trees either increased (Michelsen et al. Citation1996; Tang et al. Citation2016), decreased (Mihailović et al. Citation1997; Chaves et al. Citation2009; Mafakheri et al. Citation2010; Choat et al. Citation2012), or showed no difference (Michelsen et al. Citation1996; Ultra et al. Citation2012) under increasing temperature or changes in precipitation. These diverging results may be due to different species responding differently to environmental changes (Xu et al. Citation2014).
Experimental studies can identify the actual responses of plants to specific environmental factors. In addition, seedlings are easy to manipulate, and are particularly sensitive to environmental conditions, and thus greatly affected by changes in the environment (Fisichelli et al. Citation2014). Therefore, using open-field experimental warming and precipitation manipulation, we investigated the nature and extent of the physiological responses of seedlings of Pinus densiflora and Larix kaempferi to manipulated climate change conditions similar to actual environment. We chose to use P. densiflora and L. kaempferi seedlings in our experiments because these are the main nursery species in the Republic of Korea.
The following hypotheses were tested in this study: (1) Warming and precipitation manipulation would cause either an increase or decrease in physiological characteristics of P. densiflora and L. kaempferi, and (2) the two species would respond differently to the experimental conditions.
Materials and methods
Study site
The study was conducted in an open-field nursery site located in the Forest Technology and Management Research Center, Pocheon, Korea (37°45′38.9″N 127°10′13.4″E). The annual mean air temperature and precipitation in 2017 for this area were 10.15 °C and 1112.0 mm, respectively. Initial soil pH, total carbon concentration, total nitrogen concentration, and carbon exchange capacity (CEC) of the study site were 6.24, 0.24%, 0.016%, and 5.57 cmolc kg−1, respectively.
Experimental design
On May 18, 2017, 90 1-year-old P. densiflora seedlings and 64 1-year-old L. kaempferi seedlings were planted in separate 1 m × 1 m plots according to the guidelines for seed and seedling management (Korea Forest Service Citation2015). The experimental system to manipulate temperature and precipitation began operating on June 28, 2017, after the seedlings rooted. Open-field experimental warming and precipitation manipulation systems were designed to simulate the temperature and precipitation condition of Pocheon in 2060 based on the Representative Concentration Pathways (RCP) 8.5 climate change scenario (Korea Global Atmosphere Watch Center Citation2005). The experiment was conducted with a combination of temperature and precipitation treatments on experimental plots. Each treatment had three replicates, and treatments included two temperature levels [control (TC) and warming (TW)], and three precipitation levels [control (PC), increased precipitation (PI), and reduced precipitation (PR)]. The air temperature in TW plots was designed to be 3 °C higher than in TC plots. Warming of TW plots was achieved by using infrared heaters (FTE-1000, Mor Electric Heating Association, USA), which were controlled by data loggers (CR-1000, Campbell Inc., USA) and relays (SDM-CD16AC, Campbell Inc., USA), based on the temperature monitored by infrared temperature sensors (SI-111, Campbell Inc., USA). Precipitation in PR plots was reduced by covering 40% of plot areas with transparent panels, which were installed over PR plots to block precipitation. Precipitation in PI plots was increased by irrigating PI plots with the water collected from the coverings in the PR plots using an irrigation system. In order to monitor the soil temperature and soil water content, reflectometers (CS655, Campbell Inc., USA) were installed at a depth of 5 cm in the soil of each plot.
Measurements of physiological responses
After the experimental system to manipulate temperature and precipitation began operating, physiological responses of the seedlings were measured. Three randomly selected seedlings in each plot were used to measure net photosynthetic rate, transpiration rate, and stomatal conductance every month from July to September 2017. Each physiological response was measured at ambient temperature, using a portable photosynthesis system (CIRAS-2, PP Systems, UK) with conifer leaf cuvettes (PLC-6, PP Systems, UK) from 8:30 AM to 11:30 AM. During the measurement, 400 ppm of reference CO2 and 1100–1200 µmol m−2 s−1 of photosynthetically active radiation (PAR) were artificially provided. The leaf area was measured using a scanner (Perfection V700, Epson, Japan) and a leaf area analysis program (WinSEEDLE, Regent, Canada). Subsequently, the net photosynthetic rate, transpiration rate and stomatal conductance per unit area of the leaf were calculated. In August and September, 0.2 (±0.01) g of needles were collected to measure the total chlorophyll content. Needles were cut into pieces with length of 2 mm and incubated in 5 mL of dimethyl sulfoxide (DMSO) for 6 h in a water bath at 65 °C in the dark (Hiscox and Israelstam Citation1979; Barnes et al. Citation1992). In order to measure the absorbance of chlorophyll extracts at 665 nm and 648 nm, a spectrophotometer (U-1100, Hitachi, Japan) was used. Total chlorophyll content per unit weight of the needles was then calculated.
Statistical analysis
All statistical analyses were performed using SAS 9.4 (SAS Institute Inc., USA). Differences in environmental data, physiological variables by warming treatment, precipitation treatments and species with repeated month were analyzed by repeated measurement analysis of variance (ANOVA) tests (n = 3) by using PROC GLM. All results were considered statistically significant at p < 0.05. Tukey’s test was used for the post-hoc analysis. The model equation of the repeated measurement ANOVA was as below.
Colons were used to notate interaction terms. µ: mean response, e: random error, TC: temperature control, TW: temperature warming, PC: precipitation control, PR: reduced precipitation, PI: increased precipitation.
Environmental data
The air temperature was significantly affected by the warming treatment in all TW plots, but not by either precipitation treatment, nor by the interaction between warming and precipitation treatments (). Air temperature increased by 2.80 °C and 2.88 °C in TW plots of P. densiflora and L. kaempferi compared to TC plots of each species, respectively (). Soil water content differed significantly in plots under the precipitation treatments (). Warming and the interaction of warming and precipitation treatments had no significant effect on soil water content (; ). Soil water content decreased by 6.56% and 6.86% in PR plots, and increased by 25.36% and 32.53% in PI plots of P. densiflora and L. kaempferi compared to PC plots of each species, respectively (). As the precipitation was reduced from the top of the seedlings in PR plots while it was directly irrigated to the soil in PI plots, the change rate of soil water content compared to PC plots in PI plots were greater than that in PR plots.
Figure 1. Air temperature (a, c) and soil water content (b, d) for Pinus densiflora and Larix kaempferi plots under warming and precipitation manipulation from July to September, 2017. TC: temperature control, TW: temperature warming, PR: reduced precipitation, PC: precipitation control, PI: increased precipitation. *p < 0.05, **p < 0.01, ***p < 0.001.
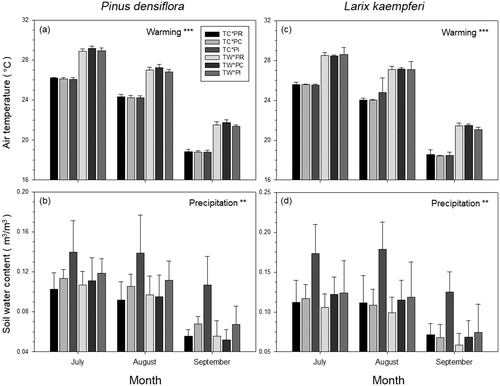
Table 1. Results of repeated measurement analysis of variance (ANOVA) tests for air temperature and soil water content of experimental plots.
Results
Total chlorophyll content, net photosynthetic rate, transpiration rate, and stomatal conductance of P. densiflora and L. kaempferi were significantly affected by the warming treatment, and total chlorophyll content and net photosynthetic rate were also significantly affected by the precipitation treatments. There was no interaction effect between warming and precipitation treatments on any physiological response (). The interaction between warming and species had a significant effect on transpiration rate and stomatal conductance ().
Table 2. Results of repeated measurement analysis of variance (ANOVA) tests for physiological responses of Pinus densiflora and Larix kaempferi seedlings.
Total chlorophyll content of P. densiflora was significantly higher in TW plots by 11.75% than in TC plots (). In TW plots, the net photosynthetic rate, transpiration rate, and stomatal conductance of P. densiflora were significantly higher by 9.14%, 30.33%, and 39.11%, respectively, than in TC plots (). In the case of L. kaempferi, total chlorophyll content was significantly higher by 18.54% in TW plots than in TC plots (). The net photosynthetic rate of L. kaempferi was significantly lower in TW plots (by 2.17%) than in TC plots (). In contrast to P. densiflora, transpiration rate and stomatal conductance of L. kaempferi in TW plots were significantly higher by 14.96% and 17.69%, respectively, than in TC plots ().
Figure 2. Total chlorophyll content (a, e), net photosynthetic rate (b, f), transpiration rate (c, g) and stomatal conductance (d, h) of Pinus densiflora and Larix kaempferi seedlings under warming and precipitation manipulation from July to September, 2017. Abbreviations of the treatments are as in . *p < 0.05, **p < 0.01, ***p < 0.001.
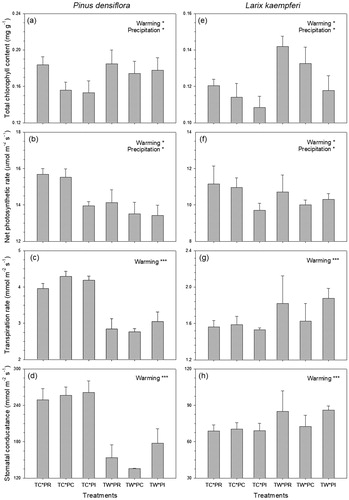
With regard to the precipitation treatments, total chlorophyll content of P. densiflora was significantly higher in PR plots by 11.64% than in PC plots (). The net photosynthetic rate of P. densiflora was significantly higher in PR plots by 2.66% than in PC plots, and was significantly lower by 5.72% in PI plots than in PC plots (). Total chlorophyll content of L. kaempferi was significantly higher by 6.40% in PR plots than in PC plots, and was significantly lower by 8.24% in PI plots than in PC plots (). The net photosynthetic rate of L. kaempferi was significantly higher in PR plots by 4.32%, while the net photosynthetic rate of L. kaempferi was 4.55% lower in PI plots than in PC plots (). Precipitation treatments did not affect transpiration rate or stomatal conductance in either species ().
Discussion
Physiological responses to warming treatment
The increase in air temperature under the warming treatment may have affected the physiological characteristics of P. densiflora and L. kaempferi. The higher total chlorophyll content in both species under the warming treatment could be attributed to the increased chlorophyll synthesis when the range of optimal temperature for the synthesis is reached (Ormrod et al. Citation1999; Zhao and Liu Citation2008).
Despite the fact that both species of trees had higher total chlorophyll content under the warming treatment, the net photosynthetic rate of both species was lower under the warming treatment. Photosynthetic rate of P. densiflora and L. kaempferi mostly showed decrease under warming in Korea (An et al. Citation2016; Park et al. Citation2016; Yun et al. Citation2016), and this could be due to the increased air temperature acting as a stressor on the seedlings. At higher temperatures, a reduction in the activation state of RuBisCO (Crafts-Brandner and Salvucci Citation2000; Cen and Sage Citation2005), and a reduction in CO2 uptake by diminished electron transport capacity increase the rate of CO2 generation by photorespiration (Farquhar and Sharkey Citation1982). This seems to have affected the net photosynthetic rate under the warming treatment. In addition, the net photosynthetic rate also appears to be influenced by stomatal control.
However, the response of stomata to warming and its influence on the net photosynthetic rate were different in each species. Increases in temperature augment vapor pressure deficits (VPD), and an increase in VPD can accelerate the evapotranspiration rate, which, in turn, can cause water stress in leaves (Allen et al. Citation1998). Isohydric species initially respond to this water stress by closing stomata (Collatz et al. Citation1991; Tardieu and Simonneau Citation1998), and P. densiflora is an isohydric species (Matyssek et al. Citation2014). Since photosynthesis can be limited by stomatal closure, it is assumed that the transpiration rate, stomatal conductance, and net photosynthetic rate of P. densiflora decreased as a result of this closure (Farquhar and Sharkey Citation1982). However, effects of warming on transpiration rate and stomatal conductance in L. kaempferi showed contrasting results with those of P. densiflora. Unlike P. densiflora, Larix spp. are anisohydric species (Matyssek et al. Citation2014). Anisohydric species maintain stomatal openings until they reach an extreme near-death state, even in cases of water stress (Tardieu and Simonneau Citation1998). Under stressful temperature conditions where the leaf temperature is very high, stomata of plants open to lower leaf temperatures through transpirational cooling (Gates Citation1968), and L. kaempferi may have undergone such a process. However, by not closing their stomata, L. kaempferi are more exposed to water stress, which is thought to result in a gradual decrease in vitality. The net photosynthetic rate of L. kaempferi appeared to be gradually reduced by this reduction in vitality, and therefore it showed a smaller reduction in the net photosynthetic rate than P. densiflora, which reduced its net photosynthetic rate in the early stages of the experiment due to stomatal closure.
Physiological responses to precipitation treatments
Physiological responses to precipitation treatments are thought to be affected by changes in soil water content. In most previous studies, chlorophyll content decreased due to a reduction in chlorophyll synthase and increase in chlorophyllase under drought stress (Mihailović et al. Citation1997; Mafakheri et al. Citation2010). However, in this study, the total chlorophyll content in P. densiflora and L. kaempferi was higher under the reduced precipitation treatment, and lower under the increased precipitation treatment, compared to the control treatments. This may be due to the fact that precipitation was applied to the plants in excess of the water requirements during the study period, even in control plots, because in Korea, annual precipitation is concentrated in summer (Seo et al. Citation2011). Accumulated precipitation from July to September in the study site was 894.5 mm, which is 80.44% of the total annual precipitation (Open Data Portal Citation2018). Excessive water content can lead to plant stress and increased protein degradation, C losses, and reduced quantum yield, which can affect chlorophyll content (d'Oro and Trippi Citation1987; Belnap et al. Citation2004). In a previous study, precipitation manipulation did not affect the photosynthetic rate of P. densiflora when the amount of precipitation in the research year was less than usual Korean precipitation (Park et al. Citation2016).
In general, a reduction in soil water content reduces the transpiration rate and stomatal conductance (Tardieu and Simonneau Citation1998; Prieto et al. Citation2009). However, in the present study, transpiration rate and stomatal conductance were not affected by changes in soil water content. Since large amounts of water were supplied to the seedlings due to the concentrated precipitation, the experimental reduction in precipitation did not seem to reduce the soil water content sufficiently to affect the transpiration rate and stomatal conductance.
The decrease and increase in the net photosynthetic rate caused by increased and reduced precipitation in both species are contrary to most previous results (Siddique et al. Citation2000; Chaves et al. Citation2009). Since there was no significant change in transpiration rate and stomatal conductance, it is thought that the net photosynthetic rate is affected by the chlorophyll content under precipitation treatments (Kabanova and Chaika Citation2001).
Conclusion
In this study, physiological responses (changes in total chlorophyll content, net photosynthetic rate, transpiration rate, and stomatal conductance) of P. densiflora and L. kaempferi seedlings under open-field experimental warming and precipitation manipulation were investigated. Under warming treatment, P. densiflora and L. kaempferi showed contrasting results in terms of transpiration rate and stomatal conductance, and the decrease in the net photosynthetic rate under warming treatment seems to have occurred through different mechanisms. In P. densiflora, which is an isohydric species, the net photosynthetic rate may have been reduced by stomatal closure, while the net photosynthetic rate of L. kaempferi, which is an anisohydric species, may have been reduced by lost vitality caused by water stress derived from high temperatures under warming. On the basis of the results of this study, we confirmed that changes in precipitation and temperature affect the physiology of P. densiflora and L. kaempferi, and the two species responded to the same temperature and precipitation conditions through different mechanisms. Therefore, the response of each species should be taken into account when devising countermeasures against climate change.
Disclosure statement
No potential conflict of interest was reported by the author(s).
Additional information
Funding
References
- Allen CD, Macalady AK, Chenchouni H, Bachelet D, McDowell N, Vennetier M, Gonzalez P. 2010. A global overview of drought and heat-induced tree mortality reveals emerging climate change risks for forests. For Ecol Manag. 259(4):660–684.
- Allen RG, Pereira LS, Raes D, Smith M. 1998. Crop evapotranspiration-Guidelines for computing crop water requirements-FAO Irrigation and drainage paper 56. FAO, Rome. 300(9):D05109.
- An J, Chang H, Park MJ, Han SH, Hwang J, Cho MS, Son, Y. 2016. Effect of experimental warming on physiological and growth responses of Larix kaempferi seedlings. J Clim Change Res. 7(1):77–84 (in Korean, with English abstract).
- Barnes JD, Balaguer L, Manrique E, Elvira S, Davison AW. 1992. A reappraisal of the use of DMSO for the extraction and determination of chlorophylls a and b in lichens and higher plants. Environ Exp Bot. 32(2):85–100.
- Belnap J, Phillips SL, Miller ME. 2004. Response of desert biological soil crusts to alterations in precipitation frequency. Oecologia. 141(2):306–316.
- Bonan GB. 2008. Forests and climate change: forcings, feedbacks, and the climate benefits of forests. Science. 320(5882):1444–1449.
- Cen YP, Sage RF. 2005. The regulation of Rubisco activity in response to variation in temperature and atmospheric CO2 partial pressure in sweet potato. Plant Physiol. 139(2):979–990.
- Chaves MM, Flexas J, Pinheiro C. 2009. Photosynthesis under drought and salt stress: regulation mechanisms from whole plant to cell. Ann Bot. 103(4): 551–560.
- Choat B, Jansen S, Brodribb TJ, Cochard H, Delzon S, Bhaskar R, Bucci SJ, Feild TS, Gleason SM, Hacke UG., et al. 2012. Global convergence in the vulnerability of forests to drought. Nature. 491(7426):752.
- Collatz GJ, Ball JT, Grivet C, Berry JA. 1991. Physiological and environmental regulation of stomatal conductance, photosynthesis and transpiration: a model that includes a laminar boundary layer. Agric For Meteorol. 54(2-4):107–136.
- Crafts-Brandner SJ, Salvucci ME. 2000. Rubisco activase constrains the photosynthetic potential of leaves at high temperature and CO2. Proc Natl Acad Sci. 97(24):13430–13435.
- Dale VH, Joyce LA, McNulty S, Neilson RP, Ayres MP, Flannigan MD, Simberloff D. 2001. Climate change and forest disturbances: climate change can affect forests by altering the frequency, intensity, duration, and timing of fire, drought, introduced species, insect and pathogen outbreaks, hurricanes, windstorms, ice storms, or landslides. AIBS Bull. 51(9):723–734.
- d'Oro GM, Trippi VS. 1987. Effect of stress conditions induced by temperature, water and rain on senescence development. Plant Cell Physiol. 28(8):1389–1396.
- Farquhar GD, Sharkey TD. 1982. Stomatal conductance and photosynthesis. Ann Rev Plant Physiol. 33(1):317–345.
- Fisichelli N, Wright A, Rice K, Mau A, Buschena C, Reich PB. 2014. First-year seedlings and climate change: species-specific responses of 15 North American tree species. Oikos. 123(11):1331–1340.
- Gates DM. 1968. Transpiration and leaf temperature. Ann Rev Plant Physiol. 19(1):211–238.
- Hiscox JD, Israelstam GF. 1979. A method for the extraction of chlorophyll from leaf tissue without maceration. Can J Bot. 57(12):1332–1334.
- Hughes L. 2000. Biological consequences of global warming: is the signal already apparent? Trends Ecol Evol. 15(2):56–61.
- Intergovernmental Panel on Climate Change (IPCC). 2014. Climate Change 2014: Synthesis Report. Contribution of working groups I, II and III to the fifth assessment report of the Intergovernmental Panel on Climate Change. UK: Intergovernmental Panel on Climate Change.
- Kabanova SN, Chaika MT. 2001. Correlation analysis of triticale morphology, chlorophyll content and productivity. J Agron Crop Sci. 186(4):281–285.
- Korea Forest Service. 2015. Guidelines for seed and seedling management. Korea: Korea Forest Service.
- Korea Global Atmosphere Watch Center. 2005. RCP Korean peninsula scenario. Korea: Korea Global Atmosphere Watch Center.
- Lindner M, Maroschek M, Netherer S, Kremer A, Barbati A, Garcia-Gonzalo J, Lexer MJ. 2010. Climate change impacts, adaptive capacity, and vulnerability of European forest ecosystems. For Ecol Manag. 259(4):698–709.
- Mafakheri A, Siosemardeh A, Bahramnejad B, Struik PC, Sohrabi Y. 2010. Effect of drought stress on yield, proline and chlorophyll contents in three chickpea cultivars. Aust J Crop Sci. 4(8):580–585.
- Matyssek R, Kozovits AR, Wieser G, Augustaitiene I, Augustaitis A. 2014. Biological reactions of forests to climate change and air pollution. Eur J For Res. 133(4):671–673.
- Michelsen A, Jonasson S, Sleep D, Havström M, Callaghan TV. 1996. Shoot biomass, δ 13 C, nitrogen and chlorophyll responses of two arctic dwarf shrubs to in situ shading, nutrient application and warming simulating climatic change. Oecologia. 105(1):1–12.
- Mihailović N, Lazarević M, Dželetović Z, Vučković M, Đurđević M. 1997. Chlorophyllase activity in wheat, Triticum aestivum L. leaves during drought and its dependence on the nitrogen ion form applied. Plant Sci. 129(2):141–146.
- Open Data Portal. 2018. Automatic Weather Station (AWS) Hourly observations. https://www.data.go.kr/dataset/15025428/openapi.do (2018. 03. 29)
- Ormrod DP, Lesser VM, Olszyk DM, Tingey DT. 1999. Elevated temperature and carbon dioxide affect chlorophylls and carotenoids in Douglas-fir seedlings. Int J Plant Sci. 160(3): 529–534.
- Park MJ Yun SJ, Yun HM, Chang H, Han SH, An J, Son Y. 2016. Effects of open-field artificial warming and precipitation manipulation on physiological characteristics and growth of Pinus densiflora seedlings. J Clim Change Res. 7(1):9–17 (in Korean, with English abstract).
- Pörtner HO and Farrell AP. 2008. Physiology and climate change. Science. 322(5902):690–692.
- Prieto P, Penuelas J, Llusia J, AsensioD, Estiarte M. 2009. Effects of long-term experimental night-time warming and drought on photosynthesis, Fv/Fm and stomatal conductance in the dominant species of a Mediterranean shrubland. Acta Physiol Plant. 31(4):729–739.
- Seo KH, Son JH, Lee JY. 2011. A new look at Changma. Atmosphere. 21(1):109–121 (in Korean, with English abstract).
- Siddique MRB, Hamid A, Islam MS. 2000. Drought stress effects on water relations of wheat. Bot Bull Acad Sinica. 41:35–39.
- Solomon S, Qin D, Manning M, Averyt K, Marquis M. 2007. Climate change 2007-the physical science basis: Working group I contribution to the fourth assessment report of the IPCC. Vol 4. Cambridge, United Kingdom: Cambridge University Press. pp. 503.
- Somero GN. 2010. The physiology of climate change: how potentials for acclimatization and genetic adaptation will determine ‘winners’ and ‘losers’. J Exp Biol. 213(6):912–920.
- Tang B, Yin C, Wang Y, Sun Y, Liu Q. 2016. Positive effects of night warming on physiology of coniferous trees in late growing season: Leaf and root. Acta Oecol. 73(2016):21–30.
- Tardieu F, Simonneau T. 1998. Variability among species of stomatal control under fluctuating soil water status and evaporative demand: modelling isohydric and anisohydric behaviours. J Exp Bot. 49:419–432.
- Ultra Jr. VU, Han SH, Kim DH, Aggangan NS. 2012. Changes in growth, photosynthetic parameters, carbon and nitrogen uptake of Pinus densiflora S. et Z. under elevated temperature and CO2 level. Asia Life Sci. 21(2):523–536.
- Xu Z., Hu T, Zhang Y. 2012. Effects of experimental warming on phenology, growth and gas exchange of treeline birch (Betula utilis) saplings, Eastern Tibetan Plateau, China. Eur J For Res. 131(3):811–819.
- Xu Z., Shimizu H., Ito S., Yagasaki Y., Zou C., Zhou G, Zheng, Y. 2014. Effects of elevated CO2, warming and precipitation change on plant growth, photosynthesis and peroxidation in dominant species from North China grassland. Planta. 239(2):421–435.
- Yun SJ, Han S, Han SH, Kim S, Li G, Park M, Son Y. 2016. Short-term effects of warming treatment and precipitation manipulation on the ecophysiological responses of Pinus densiflora seedlings. Turk J Agric For. 40(4):621–630.
- Zhao C, Liu Q. 2008. Growth and photosynthetic responses of two coniferous species to experimental warming and nitrogen fertilization. Can J For Res. 39(1):1–11.