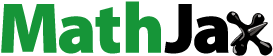
Abstract
Chamaecyparis obtusa, Chamaecyparis pisifera, and Thuja occidentalis produce essential oils of good wood and industrial benefits, including food additives, perfumes, odorants and disinfectants. To identify a single antifungal monoterpene with antifungal activity against Botrytis cinerea, we identified a single antifungal compound by chemically profiling essential oils from three coniferous species. The antifungal activity of coniferous essential oil was slightly different depending on the species but showed high antifungal activity, and T. occidentalis essential oil had the highest antifungal activity among the three essential oils. The chemical composition of essential oils from three coniferous leaves was profiled using GC-MS. The chemical profiles of C. obtusa and C. pisifera were very similar, but T. occidentalis was relatively different from the two species. The chemical composition of the three conifer oils contained 20 each. As a result of profiling three essential oil components with high antifungal activity, the compounds present in all three species were bornyl acetate and terpinyl acetate. These compounds of C. obtusa essential oil were 26.49%, C. pisifera essential oil 25.04% and T. occidentalis essential oil 12.60%. These two compounds were estimated to be antifungal agents. The antifungal activity of the six monoterpenes was different, and the single monoterpene with the highest antifungal activity was bornyl acetate followed by terpinyl acetate. Therefore, it was possible to search for antifungal activity monoterpene components by chemical profiling. Terpinyl acetate and bornyl acetate can be used for environmental-friendly insecticidal active materials.
Introduction
Essential oils are extracted from plants as a complex mixture containing different antibacterial compounds (Hanen et al. Citation2020). In this regard, monoterpenes such as bornyl acetate, citronellyl acetate, eugenol, geranyl acetate, terpinolene, and terpinyl acetate, which are the major components of essential oils, are known to provide a defense mechanism that exerts antifungal, antibacterial, and antimicrobial effects (Miranda et al. Citation2016; Cazella et al. Citation2019; Ghavam et al. Citation2021).
Natural repellent and antifungal compounds have been screened from many plants (Uma et al. Citation2017). Oliveira Filho et al. (Citation2021) investigated the chemical composition of essential oils of Cymbopogon martinii and Mentha spicata, and suggested that two essential oils could be an alternative for the control of gray mold caused by Botrytis cinerea in strawberries. In addition, Wawrzyńczak et al. (Citation2020) investigated the major constituents (monoterpene hydrocarbons) of essential oils extracted from leaves and flowers of Myrica gale and found that these essential oils exhibit antimicrobial activity against bacterial pathogens such as Staphylococcus aureus, Escherichia coli, and Enterococcus faecalis.
A widespread fungus of B. cinerea that affects not only vegetable crops, but also a large number of shrubs, trees, flowers and weeds. This fungus causes vase, fruit rot, decay, shoot rot and stem rot or rot (Lee et al. Citation2007). Botrytis blight damages fruits, vegetables, etc., so an environmentally friendly control method is needed. The best way is to use essential oils with high safety. However, there have been few reports of antifungal activity of essential oils against Botrytis blight.
Chemical profiling as a tool to study secondary metabolism has traditionally been used as a phytochemical tool for rigorous separation and identification of individual and unknown secondary metabolites (Blount et al. Citation2002). It has been used as a tool to study molecular aspects (Frydman et al. Citation2004). The volatile metabolites of the plants were profiled using gas chromatography/mass spectrometry (Nam et al. Citation2019). Differences in the number and amount of volatile metabolites were observed in many plant species. This is applied identification of plant pathogen related compounds (Prithiviraj et al. Citation2004) and discrimination of interspecies (Schulz et al. Citation1998). Most of the studies have focused on analyzing the components by only chemical profiling, but few studies have identified bioactive substances.
Chamaecyparis obtusa and Chamaecyparis pisifera trees belong to the genus Cypressaceae. The wood of C. obtusa and C. pisifera has excellent material and has a unique scent, so it is used not only as a building material and high-quality furniture, but also contains essential oils tissues such as leaves fruits, roots, and stems (Yang et al. Citation2007). Thuja occidentalis, commonly known as arbor vitae or white cedar, is indigenous to eastern North America and is growing in Europe as an ornamental tree. In folk medicine, T. occidentalis has been used to treat bronchial catarrh, enuresis, cystitis, psoriasis, uterine carcinomas, amenorrhea, and rheumatism (Johnston Citation1990) and chronic infections of the upper respiratory tract (Naser et al. Citation2005) and antibiotics (Zimmer and Gezielte Citation1985; Badawy and Abdelgaleil Citation2014). This species of essential oil has been reported to have antibacterial activity such as antibacterial activity against E. carotovora var. carotovora, and A. tumefaciens, but there are few studies on antibacterial activity against fungi such as Gray-mold rot.
The present study was conducted to investigate the antifungal activity of B. cinerea of three conifer essential oils and to identify a single monoterpene with potent antimicrobial activity through chemical profiling.
Materials and methods
Plant material
The healthy and mature leaves from 30 to 40 old C. obtusa, C. pisifera, and T. occidentalis were collected in May 2008 from Experiment Forest of Gyeongsang National University in Korea. The identification of common species was carried out with the advice of Professor Hyunsik Moon of Gyeongsang National University, who majored in plant taxonomy. After the collected leaves were weighed, essential oils were extracted immediately.
Extraction of essential oil
Essential oils were extracted from the three coniferous species leaves by the method of Seo et al. (Citation2003). Essential oil extraction was pretreated in two ways. First, the essential oil was extracted by cutting the leaves into 2 cm size. Second, we tested the extraction efficiency by grinding the leaves cut into 2 cm pieces for 1 min with a blender. Each of the pretreated leaves was diluted with 500 ml distilled water in a 2 l round flask and steam distilled (100 °C). Steam distillation continued for 7 h. Essential oil yield was calculated according to the dry weight of the plant material. The extracted oil was stored in a refrigerator at 4 °C. The resulting oil solution was used for composition analysis and antifungal experiments. The extraction efficiency of essential oil was calculated by the following equation.
Chemical profiling of essential oils through GC-MS and selection of putative antifungal substances
The volatile components separated by GC-MS were identified using the correlation between retention time (RT) and retention index (RI) and the mass spectrum. Each essential oil of conifer tree was analyzed on gas chromatograph (HP5890 SERIES II) – mass spectrometer (GC-MS, HP 5971 SERIES MSD) for chemical profiling. The GC column was a 60 mm × 0.25 mm × 0.25 µm i. d. HP-1 fused silica capillary column. The GC conditions were as follows: injecter temperature is 250 °C; column temperature, isothermal at 50 °C for 5 min, then programmed to 240 °C at 3 min and held at this temperature for 10 min; ion source temperature, 230 °C. Helium was used as the carried gas at the rate of 1 ml/min. The effluent of the GC column was introduced directly into the source of the MS. Spectra were obtained in the EI monde with 70 eV ionization energy. The sector mass analyzer was set to scan from 50 to 800 amu for 2 s. The identification was carried out by comparison of mass spectra with those in the mass spectra library (The Wiley Registry of Mass Spectral Data, 6th ed.) Contents of terpenoid compounds were determined through relatively area (%) on analyzed peaks. Also, the retention index (RI) of volatile components was checked. RI was obtained by referring to analysis data (Van Den Dool and Kratz Citation1963; National Institute of Standards and Technology (NIST Citation2021) Chemistry Webbook) (). As for the estimated antifungal substance, a compound containing all three species with the highest content in the essential oils of three kinds of coniferous trees was selected.
Fungal strain and determination of antifungal activity
The B. cinerea (KCTC 6973) used in this study were obtained from the Korea Collection for Type Culture (). Cultures of the fungi were maintained on potato dextrose agar (PDA) medium and stored at 4 °C. PDA plates were prepared using plastic Petri dishes (87 mm). Pellet of B. cinerea (φ 6.0 mm) was inoculated at the center of the dish in the dark at 25 °C for 5 days. Fungal isolates were identified by standard microbiology methods and stored on potato dextrose agar (PDA) broth with glycerol at −70 °C. Prior to antifungal susceptibility testing, each isolate was inoculated on PDA to ensure optimal growth characteristics and purity.
Antifungal assays were performed by the methods used in our previous studies (Chang et al. Citation1999, Citation2000) with slight modifications. Inhibition of the mycelial growth of B. cinerea was determined by daily measuring the radial growth on potato dextrose broth (PDB) plates containing the respective essential oils at a range of concentrations for 3, 6, and 9 days, respectively. The experiments were conducted at the center of the 35 × 10 mm Petri dishes and inoculated with fungi pellet vaccination (φ 6.0 mm) from cultures on the PDB medium on which conidia had been allowed to germinate. Briefly, 0, 1, 2, 4, 8, 16, and 32 µl essential oil of coniferous tree were added to PDB in Petri dish. Petri dishes were sealed with parafilm to prevent the leak of three essential oils. All samples were incubated in the dark at 25 ± 1 °C. When the fungi had reached the edges of the control petri dishes (those without essential oils), the antifungal indices were calculated by digimatic caliper (Mitutoyo, Japan, CD-15CP). Each sample was repeated three times, and the data were averaged. The formula of antifungal indices is shown as where Da is the diameter of the growth zone in the experimental dish (mm) and Db is the diameter of the growth zone in the control dish (mm).
Confirmation of antifungal activity of a single monoterpene
As a result of chemical profiling of the essential oils of three coniferous trees, the compounds with the highest content and all presents were bornyl acetate and terpinyl acetate. We investigated the antifungal activity against bornyl acetate and terpinyl acetate, respectively, as the single ingredient with the highest antifungal activity against B. cinerea among conifer essential oil components. Compounds used in the experiment, namely bornyl acetate (95%, Sigma Aldrich) and terpinyl acetate (Fluka), were purchased and used. Treatment concentrations were 0, 1, 2, 4, 8, 16, and 32 μl, and two types of monoterpene were added to the PDB of a Petri dish and pipetted. Antifungal activity was measured in the same way as the antifungal activity of essential oils.
In addition, the antifungal activity of a single monoterpene other than the above two compounds was investigated. The monoterpenes used in the experiment were four types of commercially available compounds. The monoterpene used in this study was (+)-2-carene (97%, Sigma Aldrich), (R)-(+)-limonene (97%, Sigma Aldrich), 1-decene (94%, Sigma Aldrich), and P-cymene (99+%, Acros organics).
The treatment concentration was the same as for the aforementioned bornyl acetate and terpinyl acetate. All samples were incubated in the dark at 25 ± 1 °C. The antifungal activity was measured in the same way as in the antifungal activity of essential oils.
Statistical analysis
Data are expressed as an average of five separate experiments. The bars indicate standard deviation from the mean of each replicate treatment. One-way analysis of variance (ANOVA) was conducted, and means were compared using Duncan’s multiple-range test (DMRT) at 0.05 level of probability. Principal component analysis (PCA) based on the contents of the volatile components was performed to classify the three coniferous species. Statistical approaches were conducted using IBM SPSS Statistics 19.0 (IBM, Armonk, NY, USA).
Results and discussion
Extraction yield of essential oil
The yields of essential oils in the leaves of the three coniferous species were different (). C. obtuse had the highest essential oil extraction yield, and T. occidentalis had the lowest. The two species of Chamaecyparis spp. showed almost the same yield of essential oil extraction. There was also a difference in yield according to the pretreatment method for essential oil extraction. As for the pretreatment method, the grinding method, which finely grinds the leaves in a grinder, has higher extraction efficiency than the cutting method, which cuts the leaves into small sizes.
Table 1. Effect of pretreatment on essential oil yield of leaves.
Antifungal activity of three coniferous species essential oils
As a result of antifungal activity analysis, coniferous species essential oil had a strong antifungal effect against B. cinerea (). 3 The antifungal activity of coniferous species essential oil was slightly different among the species. Essential oils of three coniferous species showed differences in antifungal activity at various concentrations (1, 2, 4, 8, 16 and 32 μl/l). In particular, treatment with essential oil of 8 μl/l or more showed 70% antifungal activity. C. pisifera and T. occidentalis essential oils showed strong antifungal activity in treatments under 8 μl/l. In particular, 1 μl/l T. occidentalis essential oil treatment inhibited 55.9% of fungi, and at 2 μl, 32 μl of T. occidentalis essential oil inhibited 69.3% of fungi. As a result, 3 coniferous species essential oils were found to have strong antifungal activity.
Figure 1. Antifungal activity against B. cinerea on various concentration of essential oils. B. cinerea cultured on PDB within 6 days and plates were incubated in controlled environment champers maintained at 25 °C. Values represent the mean ± SD (n = 3). Means followed by the same letters are not significantly different by Duncan’s test (p < 0.05).
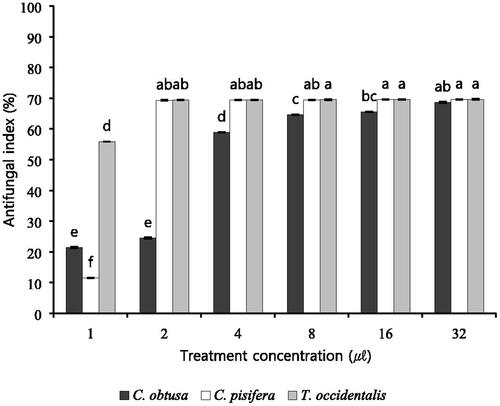
The antifungal activity of three essential oils against B. cinerea was investigated according to treatment time (). Thuja occidentalis essential oil, which showed the strongest antifungal activity, showed strong fungal growth inhibition from the initial 3 h of treatment. C. obtusa essential oil showed strong antifungal activity from the initial 3 h of treatment at a concentration of 8 µl or higher, and mild growth occurred after 6 h of treatment at a concentration lower than that, but it had antifungal activity. C. pisifera essential oil showed slightly antifungal activity at 1 μl treatment, but at higher concentration, it showed strong antifungal activity from 3 h of treatment. The antifungal activity of these three types of essential oil showed strong antifungal activity upon treatment, and the antifungal activity was concentration dependent.
Figure 2. Impacts on various concentrations of three essential oils. (A) T. occidentalis. (B) C. obtuse. (C) C. pisifera. Colony growth (mm) of B. cinerea raised on PDB within 9 days. And plates were incubated in controlled environment champers maintained at 25 °C. Values represent the mean ± SD (n = 3). Means followed by the same letters are not significantly different by Duncan’s test (p < 0.05).
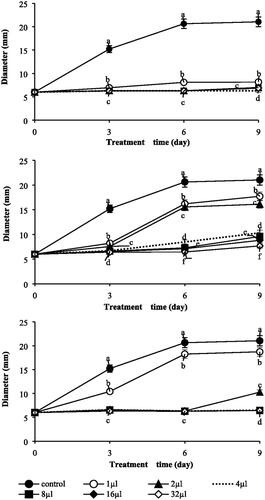
Profiling of essential oils components
The chemical composition of essential oils from three types of conifer leaves was profiled using GC-MS ( and ). The chemical profiles of three coniferous species essential oils were very similar to those of C. obtusa and C. pisifera, but T. occidentalis was relatively different from the two species.
Figure 3. Capillary gas chromatogram of three species leaves volatiles obtained by steam distillation. (A) C. cbtusa, (B) C. pisifera, and (C) T. occidentalis. The GC column was a 60 mm × 0.25 mm × 0.25 μm i. d. HP-1 fused silica capillary column. The peak numbers correspond to the numbers in . 1: I-Phellandrene, 2: Tricyclene, 3: α-Pinene, 4: Camphene, 5: Sabinene, 6: β-Pinene, 7: β-myrcene, 8: Myrcene, 9: 3-Carene, 10: Benzene, 11: Bicyclo, 12: Thujone, 13: α-Thujone, 14: Camphor, 15: 3-Cyclohexen-1-1-ol, 16: Verbenol, 17: Benzenemethanol, 18: 3-Cyclohexen-1-methan, 19: 1,4-Methanoazulene, 20: Caryophyllene, 21: Bornyl acetate, 22: 2-Cyclohexen-1, 23: Terpinyl acetate, 24: trans-2-Caren, 25: α-Cedrane, 26: 1-methyl-4, 27: Widdrene, 28: methyl, 29: Naphthalene, 30: Cyclohexanemethanol, 31: Phenanthrene, 32: α-cedrol, 33: 2-Naphthalenemethanol, 34: 7-Methanoazulen, 35: β-Eudesmol, 36: Humulen, 37: Norkaur, 38: Limonene
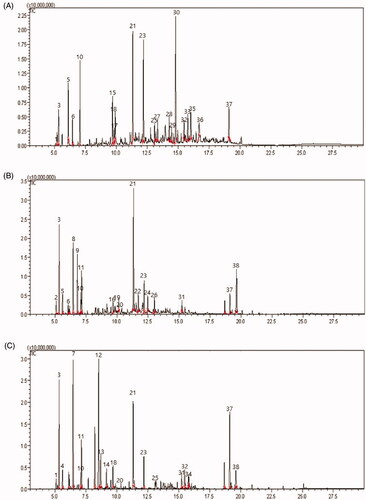
Table 2. Volatile compounds in steam-distilled essential oil of the leaves from C. obtuse and C. pisifera identified by gas chromatography-mass spectrometrya.
The chemicals of essential oils extracted from the leaves of C. obtusa and C. pisifera each identified 20 compounds. The main components of C. obtusa essential oil were cyclohexane methanol (15.95%), bornyl acetate (14.45%), terpinyl acetate (12.04%) and benzene (7.25%). C. pisifera essential oils are bornyl acetate (20.43%), α-pinene (10.79%), myrcene (10.55%) and terpinyl acetate (4.61%). Twenty volatiles have also been identified in essential oils extracted from the leaves of T. occidentalis. The main components are thujone (16.58%), b-myrcene (14.62%), bornyl acetate (9.31%) and terpinyl acetate (3.29%).
Selection of putative antifungal monoterpenes from the essential oil
As a result of profiling three essential oil components with strong antifungal activity, the compounds present in all three species were bornyl acetate and terpinyl acetate (). The two compounds in C. obtusa essential oil were 26.49%, C. pisifera essential oil 25.04%, and T occidentalis essential oil 12.60%. Once these two compounds were presumed to be antifungal actives.
Confirmation of antifungal activity against bornyl acetate and terpinyl acetate
The antifungal activity of terpinyl acetate showed a concentration-dependent trend, but there was no significant difference (). The antifungal activity of bornyl acetate also showed a similar trend as that of terpinyl acetate. However, bornyl acetate treatment showed slightly stronger antifungal activity than terpinyl acetate. Also, the antifungal activity of terpinyl acetate and bornyl acetate was not concentration-dependent. Although the mixture of terpinyl acetate and bornyl acetate was not concentration-dependent, the antifungal activity of essential oils was similar. In addition, the antifungal activity of these two compounds was slightly higher than that of the single treatment group.
Table 3. Antifungal activity of terpinyl acetate, bornyl acetate, a mixture of two monoterpenes Antifungal activity according to each treatment concentration.
The antifungal activity of terpinyl acetate was 60–91% compared to that of essential oil. In addition, the antifungal activity of BA was 88–101% compared to that of essential oil. The mixture of terpinyl acetate and bornyl acetate showed higher antifungal activity compared to essential oil.
The antifungal activity of the six monoterpenes was different (). The monoterpene with the highest antifungal activity was bornyl acetate, followed by terpinyl acetate. 2-carene, decene, and p-cymene were next, and limonene had the lowest antifungal activity. The antifungal activity according to the treatment concentration of six monoterpenes was not concentration-dependent. Antifungal activity was not different at concentrations above 2 µl treatment.
Figure 5. Antifungal activity of B. cinerea on various concentration of six individual monoterpene. B. cinerea raised on PDB within 6 days and plates were incubated in controlled environment champers maintained at 25 °C. Values represent the mean ± SD (n = 3). Means followed by the same letters are not significantly different by Duncan’s test (p < 0.05).
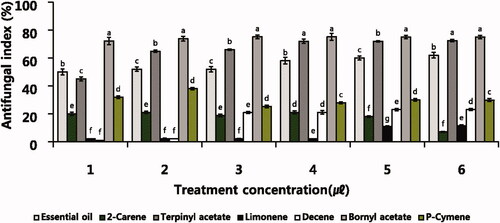
Principal components analysis (PCA) was performed to confirm the correlation of each chemical component (). shows the distribution divided into the first main component (PC 1) and the second main component (PC 2) of the principal component analysis, and shows the location of each species. The changes in PC1 and PC2 were accounted for 61% and 38%, accounting for 99% of the total change. In general, when the number of principal components is two, it is judged that it has sufficient explanatory power if the total explanatory rate is 75% or more.
Figure 6. Antifungal activity of B. cinerea on various concentration of six individual monoterpene. A: Distribution divided into first principal component (PC1) and second principal component (PC2). B: Location of each species.
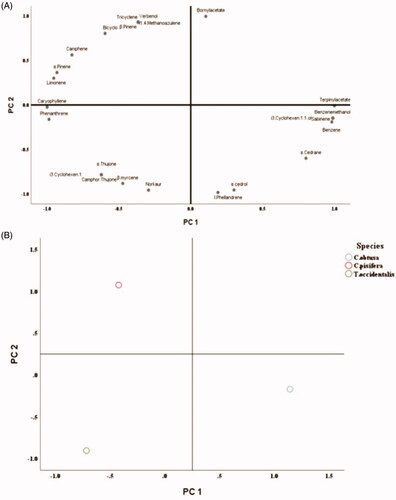
Components such as terpinyl acetate, 3 cyclohexen.1.1.ol, benzenemethanol, methyl, naphthalene, and benzene were characteristic of C. obtusa. Components such as bornyl acetate, tricyclene, verbenol, 1.4. methanoazulene, bicyclo, and camphene were characteristic of C. pisifera. It was found that these ingredients are related to distinguishing the two species. In addition, what distinguishes T. occidentalis from the other two varieties mainly consisted of components such as bicyclo, camphor, 3. cyclohexen.1, thujone, and α.thujone.
Discussion
Extraction yield of essential oil
Conifer essential oil has been studied for a long time. Kang et al. (Citation1993) extracted essential oils from seven conifers, including C. obtusa, and analyzed their chemical composition. Among them, C. obtusa essential oil has been reported to have strong antifungal activity similar to that of copper sulfate, which is a raw material for pesticides.
The essential oil yield of plant species varies depending on the region and season. Among the coniferous species growing in Turkey, in pinecone was reported as 0.13–0.48 ml/100 g, fir was 0.42–0.59 ml/100 g, spruce was 0.36 ml/100 g, and cedar was 0.37 ml/100 g (Tumen et al. Citation2010). In our previous study, the seasonal refinery yield was 4.5% in winter for C. obtusa trees and 5.3% in autumn for C. pisifera trees (Yang et al. Citation2002). In addition, chopping has been reported to have higher extraction efficiency of essential oil than the cutting method.
Antifungal activity of three coniferous species essential oils
The antifungal activity of the three coniferous species was concentrated, but the activity increased as the concentration increased, but it was very strong. C. pisifera and T. occidentalis essential oils showed potent antifungal activity at treatments below 8 μl/l. In particular, in the case of T. occidentalis essential oil, even at 1 μl/l treatment concentration, 55.9% was inhibited, and in the 2 μl to 32 μl treatment group, 69.3% of fungi were suppressed.
Felšöciová et al. (Citation2020) reported that three essential oils, such as Carum carvi L., Satureja hortensis L., and Pimpinella anisum L., exhibited antifungal activity against penicillium sp. at a concentration of 0.75–0.125 μl/ml. Although the microorganisms tested for antifungal activity are different, it can be said that the essential oil component of this study has a very strong antibacterial activity about 100 times.
Three kinds of coniferous essential oil showed strong antifungal effect against B. cinerea, which causes spoilage of fruits and foods. There are few reports of plant essential oils antifungal activity against B. cineria. Vitoratos et al. (Citation2013) suggested that lemon, thyme and oregano essential oils inhibit the mycelium growth and spore germination of postharvest pathogens (B. cinerea, P italicum, and P digitatum). Mohammadi et al. (Citation2014) also reported that among the four essential oils (fennel, black caraway, peppermint, and thyme), black caraway and fennel essential oils had the strongest antifungal activity in B. cinerea and R. stolonifer. However, very few cases have reported antifungal activity of coniferous species essential oil against B. cinerea. The reason is presumed to be known because the antifungal spectrum for microorganisms is narrow for the characteristics of plant antifungal substances.
There was also a difference in antifungal activity against B. cinerea among the three coniferous species, and among them, T occidentalis showed the strongest antifungal activity. This difference in antifungal activity appears to be due to differences in the chemical composition of the essential oils. The chemical composition of the essential oils of the three conifers showed a slight difference, and above all, it seems to be closely related to the content. In many aromatic plants, the quantitative composition of essential oils is strongly influenced by genotype and habitat such as harvest time, plant age, and planting density (Marotti et al. Citation1994), plant species, chemotypes, and climatic conditions (Thompson et al. Citation2003). The essential oil extracted from the leaves of T. occidentalis, which showed the highest antifungal effect in this study, is distilled from the leaves and branches and used as medicines and perfumes. The aroma of this essential oil is very similar to camphor, and this essential oil species is the most important commercial essential oil in North America (Simon et al. Citation1987).
Profiling of essential oils components
The terpenoids components extracted from the leaves of three coniferous species were identified by retention time (RT) and retention index (RI). The retention index outside the retention time was proposed by the Swiss chemist Ervin Kovats in the analysis of volatile compounds using gas chromatography, and it expresses the retention index at equal intervals based on straight-chain alkanes (Kovats Citation1958). Determining a compound's retention index (RI) and comparing it to a collection of available retention data is a common method of confirming compound identification (Garcia-Dominguez et al. Citation2006). Conservation data is more useful when used in conjunction with mass spectrometry. The reason is that this combination allows almost unambiguous identification of isomers that are difficult to use with mass spectra alone. Compounds can be identified usefully in the compound analysis of conifer essential oil in this study.
The chemical composition of essential oils extracted from coniferous leaves was profiled using GC-MS. The chemical profiles of the three coniferous essential oils were very similar for C. obtusa and C. pisifera, but T. occidentalis was relatively different from the two species. The chemical compositions revealed composition of T. occidentalis leaves was similar to those of other EMEA (Citation1999) and Wallach (Citation1887), which the major components were d-alpha thujone and l-fenchone, d-alpha pinene and 1-borneol (free and as the acetate). Gas chromatography-mass spectrometry (GC-MS) is essential to evaluate the biological activity of essential oils and can perform qualitative and quantitative analysis (Daferera et al. Citation2000).
Chemicals in essential oils extracted from the leaves of three types of conifers each identified 20 compounds. The number of compounds contained in essential oils varies from species to species. The essential oil extracted from pine contains more than 50 components, among which five are the main components: α-pinene, β-pinene, camphene, 3-karene, and myrcene (Silori et al. Citation2019). The essential oils of Greek aromatic plants also contained 18 essential oils (Daferera et al. Citation2000).
Selection of putative antifungal monoterpenes from the essential oil
Profiling three essential oil components with potent antifungal activity showed that the compounds present in all three species were bornyl acetate and terpinyl acetate. These compounds were 12.60–26.49%. As a result of the antifungal activity assay of six monoterpenes, these two compounds were identified as antifungal substances due to the high levels of bornyl acetate and terpinyl acetate.
The essential oil component consists of more than 20 compounds, but contains the main component. Silori et al. (Citation2019) also investigated each major compound in various pine species around the world and said that it was five components: α-pinene, β-pinene, camphene, 3-carene, and myrcene. Lingan (Citation2018) also showed that the main components of Prangos latiloba Korov essential oil, a drought tolerant plant, were geranial (26.8%), methyl chavicol (22.8%) and neral (22.6%), which was different from previous studies on the same species. This indicates that the chemical composition of essential oils of the same species may change depending on different conditions such as plant harvesting altitude, harvesting area, soil composition, lipids, harvesting time, and different hydraulic distillation methods (Dahham et al. Citation2016)
Confirmation of antifungal activity against bornyl acetate and terpinyl acetate
As a result of profiling three essential oil components with potent antifungal activity, the compounds present in all three species were bornyl acetate and terpinyl acetate, and due to their high content, these two compounds were presumed to have antifungal activity. Kusumoto et al. (Citation2014) evaluated the antifungal properties of 14 major oleoresin constituent terpenoids of Norwegian spruce (Picea abies) and found that terpineol and bornyl acetate were more potent than monoterpene hydrocarbons such as (+)-a-pinene. reported to have high antifungal activity. These results are consistent with our results. Tešević et al. (Citation2009) analyzed Douglas fir oil and found that it contained 10 compounds, of which bornyl acetate was the most common compound (34.65%). Also, Douglas fir oil has been reported to have strong antifungal activity against Phomopsis helianthi and Penicillium sp.
As a result of examining the antifungal activity against monoterpenes including terpinyl acetate and bornyl acetate, it was found that terpinyl acetate and bornyl acetate had strong antifungal activity.
Terpinyl acetate and other short-chain esters are natural flavors widely used in food, cosmetic and pharmaceutical industries as food additives, perfume, odorant and disinfectant (Banthorpe Citation1994). Besides, it has heath protection efficiency such as anti-bacterial, anti-infection, anti-nociceptive and anti-oxidization. In cosmetology, it even can accelerate vasoconstriction, astriction and getting rid of the bad smell of perspiration (Bauer et al. Citation1990). The production of terpene esters has been traditionally achieved by chemical synthesis since extraction from plant materials or the production by fermentation is at present too expensive for commercial exploitation (Dhavalikar and Bhattacharyya Citation1966; Gainsford et al. Citation2001).
Bornyl acetate is chemically classified as a monoterpene ester. It shares the same carbon backbone as borneol, a highly researched monoterpene alcohol. The only difference between the two compounds is that the alcohol group is replaced with an acetate ester group. Bornyl acetate is an ingredient in essential oils of the Pinaceae family and causes food additives, flavors and odors. In addition, bornyl acetate, isolated from C. japonica essential oil, is known to have potential as a cosmetic material as it has a skin whitening effect (Kim et al. Citation2013). Various research groups have found that bornyl acetate affects gene expression and protein activity in different cell types in a way that can soothe a normal inflammatory response (Yang et al. Citation2014). It is also effective for supporting cellular health in gastric cells (Kim et al. Citation2013).
In general, treatment of major components among essential oils is known to have lower antifungal activity than those treated with essential oils (Letessier et al. Citation2001). However, in our results, there was no difference in antifungal activity between the essential oil treatment and terpinyl acetate and bornyl acetate treatment. This means that eco-friendly antifungal agents can be treated more economically. However, more detailed antifungal mechanisms and safety of these acetate compounds need to be studied. In addition, in this study, GC-MS results were calculated as retention times, which requires further analysis using Kovats indices.
In conclusion, essential oils from three coniferous trees have been shown to have antifungal action against Gray-mold rot or Botrytis blight, which is widely infected with fruits and vegetables. In addition, terpinyl acetate and bornyl acetate discovered through chemical profiling are expected to have strong antifungal properties, enabling the development of new antifungal agents.
Acknowledgements
This study was carried out by the Forest Service's Forest Convergence Specialist Training Project (Support for Forest Industry Characterization Research, FTIS Assignment No. 2020186A00-2022-AA02).
Disclosure statement
No potential conflict of interest was reported by the author(s).
References
- Badawy MEI, Abdelgaleil SAM. 2014. Composition and antimicrobial activity of essential oils isolated from Egyptian plants against plant pathogenic bacteria and fungi. Ind Crops Prod. 52:776–782.
- Banthorpe DV. 1994. Terpenoids. In: Mann J, Davidson RS, Hobbs JB, Banthorpe DV, Harborne JB, editors. Natural products. Their chemistry and biological significance. Longman scientific & technical. Harlow, UK: Longman Group; p. 289–359.
- Bauer K, Garbe D, Surburg H. 1990. Common fragrance and flavor materials. 2nd ed. New York: VCH Publishers.
- Blount JW, Masoud S, Sumner LW, Huhman D, Dixon RA. 2002. Over-expression of cinnamate 4-hydroxylase leads to increased accumulation of acetosyringone in elicited tobacco cell-suspension cultures. Planta. 214(6):902–910.
- Cazella LN, Glamočlija J, Sokovic M, Gonçalves JE, Linde GA, Colauto NB, Gazim ZC. 2019. Antimicrobial activity of essential oil of Baccharis dracunculifolia DC (Asteraceae) aerial parts at flowering period. Front Plant Sci. 10:27.
- Chang ST, Wang SY, Wu CL, Chen PF, Kuo YH. 2000. Comparison of the antifungal activity of cadinane skeletal sesquiterpenoids from Taiwania (Taiwania cryptomerioides Hayata) heartwood. Holzforschung. 54(3):241–245.
- Chang ST, Wang SY, Wu CL, Su YC, Kuo YH. 1999. Antifungal compounds in the ethyl acetate soluble fraction of the extractives of Taiwania (Taiwania cryptomerioides Hayata) heartwood. Holzforschung. 53(5):487–490.
- Dahham SS, Tabana YM, Sandai D, Ahmed MA, Abdul M. 2016. In vitro anti-cancer and anti-angiogenic activity of essential oils extracts from Agarwood (Aquilaria crassna). Med Aromat Plants. 05(04):256.
- Dhavalikar RS, Bhattacharyya PK. 1966. Microbiological transformations of terpenes. Fermentation of limonene by a soil pseudomonad. Indian J Biochem. 3(3):144–157.
- Daferera DJ, Ziogas BN, Polissiou MG. 2000. GC-MS analysis of essential oils from some Greek aromatic plants and their fungitoxicity on Penicillium digitatum. J Agric Food Chem. 48(6):2576–2581.
- EMEA (The European Agency for the Evaluation of Medicinal Products). 1999. Committee for veterinary medicinal products—Thuja occidentalis. Summary report.
- Felšöciová S, Vukovic N, Jeżowski P, Kačániová M. 2020. Antifungal activity of selected volatile essential oils against Penicillium sp. Open Life Sci. 15(1):511–521.
- Frydman A, Weisshaus O, Bar-Peled M, Huhman DV, Sumner LW, Marin FR, Lewinsohn E, Fluhr R, Gressel J, Eyal Y. 2004. Citrus fruit bitter flavors: isolation and functional characterization of the gene Cm1,2RhaT encoding a 1,2 rhamnosyltransferase, a key enzyme in the biosynthesis of the bitter flavonoids of citrus. Plant J. 40(1):88–100.
- Gainsford GJ, Hosie CF, Weston RJ. 2001. Conversion of a-pinene to terpinyl acetate over H-beta zeolites. Appl Catal A. 209(1–2):269–277.
- Garcia-Dominguez JA, Lebron-Aguilar R, Quintanilla-Lopez JE. 2006. An accurate and easy procedure to obtain isothermal Kováts retention indices in gas chromatography. J Sep Sci. 29(18):2785.
- Ghavam M, Manconi M, Manca ML, Bacchetta G. 2021. Extraction of essential oil from Dracocephalum kotschyi Boiss. (Lamiaceae), identification of two active compounds and evaluation of the antimicrobial properties. J Ethnopharmacol. 267:113513.
- Hanen F, Mariem BJB, Mariem S, Riadh K. 2020. Essential oils: a promising eco-friendly food preservative. Food Chem. 330:127268.
- Johnston WF. 1990. Thuja occidentalis L: Northern White-Cedar. In: Russell MB, Barbara HH, editors. Silvics of North America. Washington (DC): Forest Service United States Department of Agriculture; p. 580–589.
- Kang HY, Lee SS, Choi IG. 1993. The antifungal activity of coniferous needle oil. J Environ. 13(2):71–77.
- Kim SH, Lee SY, Hong KS, Gwak KS, Park MJ, Smith D, Choi IG. 2013. Whitening and antioxidant activities of bornyl acetate and nezukol fractionated from Cryptomeria japonica essential oil. Int J Cosmet Sci. 35(5):484–490.
- Kovats E. 1958. Characterization of organic compounds by gas chromatography. Part 1. Retention indices of aliphatic halides, alcohols, aldehydes and ketones. Helv Chim Acta. 41(7):1915–1932.
- Kusumoto N, Zhao T, Swedjemark G, Ashitani T, Takahashi K, Borg-Karlson AK. 2014. Antifungal properties of terpenoids in Picea abies against Heterobasidion parviporum. For Path. 44(5):353–361.
- Lee SO, Park IK, Choi GJ, Lim HK, Ja KS, Cho KY, Shin SC, Kim JC. 2007. Fumigant activity of essential oils and components of Illicium verum and Schizonepeta tenuifolia against Botrytis cinerea and colletotrichum gloeosporioides. J Microbiol Biotechnol. 17(9):1568–1572.
- Letessier MP, Svoboda KP, Walters DR. 2001. Antifungal activity of the essential oil of Hyssop (Hyssopus officinalis). J Phytopathol. 149(11–12):673–678.
- Lingan K. 2018. A review on major constituents of various essential oils and its application. Transl Med. 08:1.
- Marotti M, Piccaglia R, Giovanelli E, Deans SG, Eaglesham E. 1994. Effects of planting time and mineral fertilization on peppermint (Mentha x piperita L.) essential oil composition and its biological activity. Flavour Fragr J. 9(3):125–129.
- Miranda CASF, Cardoso MG, Batista LR, Rodrigues LMA, Figueiredo ACS. 2016. Essential oils from leaves of various species: antioxidant and antibacterial properties on growth in pathogenic species. Rev Cienc Agron. 47(1):213–220.
- Mohammadi S, Aroiee H, Aminifard MH, Tehranifar A, Jahanbakhsh V. 2014. Effect of fungicidal essential oils against Botrytis cinerea and Rhizopus stolonifer rot fungus in vitro conditions. Arch Phytopathol Pflanzenschutz. 47(13):1603–1610.
- Nam KH, Kim DY, Pack IS, Park JH, Seo JS, Choi YD, Cheong JJ, Kim CH, Kim CG. 2019. Global metabolite profiling based on GC–MS and LC–MS/MS analyses in ABF3-overexpressing soybean with enhanced drought tolerance. Appl Biol Chem. 62(1):15.
- Naser B, Bodinet C, Tegtmeier M, Lindequist U. 2005. Thuja occidentalis (Arbor vitae): a review of its pharmaceutical, pharmacological and clinical properties. Evid Based Complement Alternat Med. 2(1):69–78.
- National Institute of Standards and Technology (NIST). 2021. Chemistry Webbook, SRD 69.
- Oliveira Filho J. G d, da Cruz Silva G, de Aguiar AC, Cipriano L, de Azeredo HMC, Bogusz Junior S, Ferreira MD. 2021. Chemical composition and antifungal activity of essential oils and their combinations against Botrytis cinerea in strawberries. Food Measure. 15(2):1815–1825.
- Prithiviraj B, Vikram A, Kushalappa AC, Yaylayan V. 2004. Volatile metabolite profiling for the discrimination of onion bulbs infected by Erwinia carotovora ssp. carotovora, Fusarium oxysporum and Botrytis allii. Eur J Plant Pathol. 110(4):371–377.
- Schulz H, Krüger H, Liebmann J, Peterka H. 1998. Distribution of volatile sulfur compounds in an interspecific hybrid between Onion (Allium cepa L.) and Leek (Allium porrum L.). J Agric Food Chem. 46(12):5220–5224.
- Seo WT, Yang JK, Kang BK, Park WJ, Hong SC, Kang YM, Jung HY, Kim YD, Kang SM, Kim SW, Choi MS. 2003. Extraction and biological activities of essential oil from Thuja occidentalis leaves. Kor J Medicin Crop Sci. 11(5):364–370.
- Silori G, Kushwaha N, Kumar V. 2019. Essential oils from pines: chemistry and applications. In: Malik S, editor. Essential oil research, trends in biosynthesis, analytics, industrial applications and biotechnological production. Cham (Switzerland): Springer; p. 275–297.
- Simon DZ, Beliveau J, Aube C. 1987. Cedarleaf oil estractèd by hydrodiffusion and stearn distillationa a Cornparison of oils produced by both processes. Int J Crude Drug Res. 25(1):4–6.
- Tešević V, Milosavljević SM, Vajs V, Đorđević I, Soković M, Lavadinović V, Novaković M. 2009. Chemical composition and antifungal activity of the essential oil of Douglas fir (Pseudosuga menziesii mirb. Franco) from Serbia. Glas Hem Drus Beogr. 74(10):1035–1040.
- Thompson JD, Chalchat JC, Michet A, Linhart YB, Ehlers B. 2003. Qualitative and quantitative variation in monoterpene co-occurrence and composition in the essential oil of Thymus vulgaris chemotypes. J Chem Ecol. 29(4):859–880.
- Tumen I, Hafizoglu H, Kilic A, Dönmez IE, Sivrikaya H, Reunanen M. 2010. Yields and constituents of essential oil from cones of Pinaceae spp. natively grown in Turkey. Molecules. 15(8):5797–5806.
- Uma K, Xin H, Kumar BA. 2017. Antifungal effect of plant extract and essential oil. Chin J Integr Med. 23(3):233–239.
- Van Den Dool H, Kratz PD. 1963. A generalization of the retention index system including linear temperature programmed gas-liquid partition chromatography. J Chromatogr Aug. 11:463–471.
- Vitoratos A, Bilalis D, Karkanis A, Efthimiadou A. 2013. Antifungal activity of plant essential oils against Botrytis cinerea, Penicillium italicum and Penicillium digitatum. Not Bot Hort Agrobot Cluj. 41(1):86–92.
- Wallach O. 1887. Zur Kenntniss der Terpene und der ätherischen Oele. Justus Liebigs Ann Chem. 238(1–2):78–89.
- Wawrzyńczak K, Sadowska B, Więckowska-Szakiel M, Kalemba D. 2020. Composition and Antimicrobial activity of Myrica gale L. leaf and flower essential oils and hydrolates. RecNatProd. 15(1):35–45.
- Yang H, Zhao R, Chen H, Jia P, Bao L, Tang H. 2014. Bornyl acetate has an anti-inflammatory effect in human chondrocytes via induction of IL-11. IUBMB Life. 66(12):854–859.
- Yang JK, Choi MS, Seo WK, Rinker DL, Han SW, Cheong GW. 2007. Chemical composition and antimicrobial activity of Chamaecyparis obtusa leaf essential oil. Fitoterapia. 78(2):149–152.
- Yang JK, Kang BK, Kim TH, Hong SC, Seo WT, Choi MS. 2002. Efficient extraction methods and analysis of essential oil from softwood leaves. Kor Soc Biotechnol Bioengin. 17(4):357–364.
- Zimmer M, Gezielte K. 1985. Therapie der akuten sinusitis in der HNO-Praxis. Therapiewo. 35(36):4024–4028.