Abstract
Excessively high temperatures and droughts after winter dormancy can affect the physiological responses of plant seedlings. In the present study, an open-field experiment was conducted to investigate the impact of spring warming and drought treatments on the short-term physiological responses of 1-year-old Larix kaempferi seedlings. The warming treatment was designed to increase the air temperature by 4 °C compared to that of the temperature control plots and was carried out for seven days each in the second and fourth week of May 2020. Moreover, the drought treatment was designed to completely block precipitation for four weeks in May 2020. After the first warming treatment period, stomatal conductance, transpiration rate, and net photosynthetic rate decreased by 35.16%, 29.53%, and 13.34% in the temperature warming plots compared to those in the temperature control plots, respectively. After the resting stage, stomatal conductance and transpiration rate increased by 101.47% and 72.80% in the temperature warming plots compared to those in the temperature control plots, respectively. Stomatal conductance, transpiration rate, and net photosynthetic rate tended to decrease in the drought treatment. The total chlorophyll content did not change under the warming treatment, but it increased by 20.29% in the drought treatment plots compared to that in the precipitation control plots in the fourth week; this may have resulted from chlorophyll hormesis. Furthermore, the correlation and principal component analyses showed that seedling physiological responses and environmental conditions were closely related. We found that spring warming and drought treatments can reduce stomatal conductance, transpiration rate, and net photosynthetic rate, thus affecting seedling growth. This study is expected to be the basis for more in-depth studies on the effects of warming and drought treatments on the growth and phenology of L. kaempferi seedlings.
Introduction
According to the Intergovernmental Panel on Climate Change (IPCC), since 1970, the mean air temperature has risen at a rate of 1.7 °C per century (IPCC Citation2018). Warming greater than the global average has already been experienced in many regions and seasons (IPCC Citation2018). In Seoul, South Korea, the mean air temperature during spring is currently 12.9 °C and is expected to increase to 16.6 °C by 2100 under the Representative Concentration Pathway (RCP) 8.5. Furthermore, in Seoul, the maximum number of consecutive dry days is currently 18 days (in 2019) and is expected to reach 30 days by 2100 (Korea Meteorological Administration, Citation2020). Heat and drought are the major stresses influencing plant growth and physiological responses (Li Citation1998). Excessively high temperatures and droughts after winter dormancy can negatively affect the physiological responses such as stomatal conductance (gs), transpiration rate (E), net photosynthetic rate (Pn), and total chlorophyll content (Chlt) of plant seedlings (Lewis et al. Citation2001; Saxe et al. Citation2001; Lipiec et al. Citation2013).
Generally, experimental warming and drought studies have been conducted in indoor or open-field experiments (Lee et al. Citation2013). An indoor experiment was conducted to study plants’ response to changes in atmospheric or soil conditions in a greenhouse (Ambebe et al. Citation2010). In contrast, a previous open-field experiment involved artificially changing atmospheric or soil conditions outdoors using infrared lamps, heat wires, chambers, or precipitation blocking systems to observe plant reactions (Jo et al. Citation2011; Chung et al. Citation2013). Despite the difficulties in controlling temperature and precipitation in open-field experiments, these kinds of experiments have the advantage of maintaining other environmental factors, i.e. maintaining natural conditions (Chung et al. Citation2013). To better understand the interaction between plants and biogeochemical cycles in forest ecosystems, further investigations using an open-field climate change manipulation approach are needed (Chung et al. Citation2013). Moreover, open-field experiments on climate change involving two or more factors are increasing (Wu et al. Citation2011; Chang et al. Citation2019).
Larix kaempferi accounts for 36% of the total plantation area in South Korea. This species is a major forest resource for timber production with consistent cultivation (Korea Forest Service Citation2020a, Citation2020b). However, the distribution of coniferous forests in South Korea is expected to decrease rapidly and become endangered with rising temperatures (NIFoS (National Institute of Forest Science) Citation2014). Trees are relatively sensitive to changes in environmental conditions at the seedling stage because their roots and stems are not fully developed (Fisichelli et al. Citation2014). In addition, the physiological attributes of seedlings influence their transplanting and growth (Cuesta et al. Citation2010).
Studies on the physiological responses of L. kaempferi to warming and drought treatments have been conducted worldwide (Crane et al. Citation1983; Yura Citation1989). However, few studies have been conducted in the spring season only, and few have explored how the cessation of warming treatment affects L. kaempferi. It is necessary to understand how excessively high temperatures and drought can affect seedlings during spring after winter dormancy and how their response changes when the high-temperature period ends. Therefore, in the present study, we investigated the effects of warming, warming cessation, and drought on the physiology of L. kaempferi seedlings. We hypothesized that warming and drought treatments would reduce gs, E, Pn, and Chlt, and the reduction trend would decrease during the resting stage of the warming treatment.
Materials and methods
Study site and experimental design
In April 2020, an open-field nursery was built in the Korea University, Seoul, South Korea (37° 35′ 36″ N, 127° 1′ 31″ E). The mean annual air temperature and precipitation in the region for a 30-year period (1990–2019) were 12.8 °C and 1,410 mm, respectively. The soil texture at the study site was sandy loam. The soil acidity (pH) and soil carbon and nitrogen concentrations (%) were 6.52, 0.22, and 0.05, respectively.
Twenty open-field experimental plots (1.0 m × 1.5 m) were constructed by combining two temperature levels (temperature control, TC, and warming, TW) and two precipitation levels (precipitation control, PC, and drought, PD) with five replicates. Eighty 1-year-old L. kaempferi seedlings were planted in each experimental plot. An expected 4 °C increase in temperature and the maximum number of consecutive dry days in 30 days in spring 2100 based on RCP 8.5 was simulated in the experiment. Two infrared heaters (FT-1000, Mor Electric Heating Association Inc., USA) were installed in the TW 60 cm above the average seedling height, as described by Kimball et al. (Citation2008). Data loggers (CR1000X, Campbell Scientific Inc., USA) and relays were used to keep the air temperature of TW 4 °C higher than that of TC. The warming treatment was conducted for seven days each in the second and fourth week of May 2020. In order to completely block precipitation, PD was designed to automatically unfold the precipitation blocking cover when precipitation was detected for four weeks ().
Figure 1. Open-field warming and drought manipulation system. TC: temperature control plots, TW: temperature warming plots, PC: precipitation control plots, PD: drought plots.
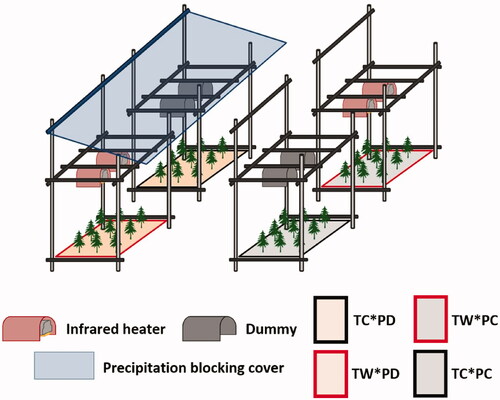
Infrared thermometers (SI-111, Campbell Scientific Inc., USA) and soil temperature moisture sensors (CS655, Campbell Scientific Inc., USA) were installed in each plot to monitor air and soil temperatures and soil moisture content. Environmental data were collected every 30 min using a data logger.
Measurements
Leaf gas exchange rates (gs, E, and Pn) were measured once a week during the experiment. Three seedlings were randomly chosen from each plot, and the leaf gas exchange rate was measured from 7:30 am to 11:30 am using a portable photosynthetic meter (LI-6800, LI-COR Inc., USA). To calculate the leaf gas exchange per unit area, the leaf area used for gas exchange measurement was measured using the Perfection 4990 scanner (EPSON, Japan) and WinSEEDLE (Regent, Canada). To measure Chlt, the collected leaves were cut into small pieces of <2 mm, weighed at 20 mg, and deposited in 5 mL of dimethyl sulfoxide. Each sample was heated for 6 h at 65 °C in a digital constant-temperature water bath (HQ-DW22, Coretech Korea Co., Korea). Absorbance was then measured at 648 nm and 665 nm using a spectrophotometer (UH5300, Hitachi Inc., Japan), after which Chlt was calculated (Barnes et al. Citation1992).
Statistical analyses
The effects of warming and drought on gs, E, Pn, and Chlt of L. kaempferi seedlings were analyzed by repeated-measures ANOVA using the PROC GLIMMIX procedure, and the Tukey post-hoc test was performed to test for statistically significant differences. The simple correlation coefficient was calculated using the Pearson correlation coefficient to determine the relationships between the studied variables and to confirm multicollinearity. Principal component analysis (PCA) was conducted to establish the correlations between different treatments. All analyses were performed using SAS version 9.4 (SAS Institute Inc., USA) at a 0.05 significance level.
Results and discussion
Environmental conditions
During the warming treatment period (second and fourth week), the mean air temperature showed significant differences among the treatments in the following order TW*PD > TW*PC > TC*PD > TC*PC. It was significantly increased in TW (by 3.95 °C) compared to that in TC, and it was also significantly increased in PD (by 0.90 °C) compared to that in PC (). Without interaction between their effects, both warming and drought treatments significantly affected the air temperature. The effect of the drought treatment on temperature indicated that the moisture in PC prevented the temperature rise from being as high as observed in PD. Generally, higher air temperatures tend to be observed in plots with reduced precipitation (Flanagan et al. Citation2013). In the present study, during the first and third weeks, there was no significant difference in mean air temperature between TC and TW following the treatments ().
Table 1. Mean weekly air and soil temperatures (°C) during four weeks of warming and drought treatments in May 2020. In each column, values with different letters indicate significant differences among the four treatments, with p < .05 being considered significantly different.
During the warming treatment period (second and fourth week), the mean soil temperature also showed significant differences among the treatments in the following order TW*PD > TW*PC > TC*PD > TC*PC. In TW, mean soil temperature increased significantly by about 4.78 °C compared to that in TC, and in PD, it increased significantly by about 1.65 °C compared to that in PC (). Our results indicated that compared to air temperature, soil temperature had a stronger response to experimental warming. This is likely due to the fast lateral energy exchange between the warmed plots and the ambient atmosphere (Wan et al. Citation2002). We found that both warming and drought treatments in the second and fourth weeks significantly affected the soil temperature without any interaction between their effects. The p values of the interaction effect in the second and fourth weeks are 0.9601 and 0.0743, respectively. Meanwhile, at the resting stage (third week), the soil temperature in TC*PC was significantly lower than that in the other treatment plots () owing to the high soil moisture content caused by precipitation ().
Stomatal conductance (gs) and transpiration rate (E)
The treatment effect on gs and E varied over time (). In the second week, the warming treatment did not affect these two variables. However, their values were lower in PD than in PC. In the third week, both variables decreased in both TW and PD. In the fourth week, they were significantly increased in TW compared to those in TC. There was no significant interaction between the effects of warming and drought on gs and E.
Figure 3. Stomatal conductance (a), transpiration rate (b), net photosynthetic rate (c), and total chlorophyll content (d) of Larix kaempferi seedlings. All the values are the mean of fifteen replicates. Error bars are the standard errors of the means, and asterisk (*) indicates significant difference among treatments (*p < .05, **p < .01, and ***p < .001). TC: temperature control plots, TW: temperature warming plots, PC: precipitation control plots, PD: drought plots.
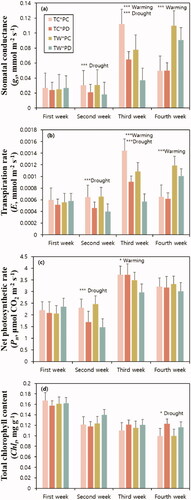
The temperature did not affect gs and E in the second week, but in the third week, they were significantly decreased (p < .0001). The decreases in mean gs and E were 35.16% and 29.53%, respectively, in TW compared to those in TC. This was possibly due to a delay in the effect of the warming treatment. According to Weih (Citation2000), the growth responses of Betula pubescens seedlings did not appear immediately with stress occurrences and can be delayed to the next year. In addition to the growth reaction, physiological responses such as gs and Pn were delayed due to stress (Bae et al. Citation2009). Our results also show a delayed response of one week. Although the warming treatment was performed again during the fourth week, gs and E were significantly increased by 101.47% and 72.80%, respectively, in TW compared to those in TC. This may have been resulting from the delay of the third week’s recovery reaction, which is the resting stage without treatments. This was likely related to the recovery reaction after the stress treatment (Hao et al. Citation2015). If stress is not severe, plants under stress conditions tend to grow healthier than those without stress when the conditions return to normal (Kwak and Yoon Citation2008).
We believe that the observed decrease in gs and E in seedlings under the drought treatment was due to decreased leaf water content, resulting from a decrease in soil moisture content. Precedent simulations also confirmed that leaf drying resulted in stomata closure and a decrease in gs (Collatz et al. Citation1991). A decrease in gs is known to result in a decrease in E (Farquhar and Sharkey Citation1982).
Net photosynthetic rate (Pn)
In the present study, Pn was lower in PD during the second week than in PC (). This was because of decreased photosynthetic rate due to drying stress caused by decreased soil moisture content (Kim et al. Citation2014). The difference in soil moisture content between PC and PD in the second week was the greatest during the entire experimental period (). In the third week, TW showed a 13.34% lower Pn than TC (). In previous warming studies, various effects of warming treatments on Pn have been reported; with increasing temperature, Pn decreased (He and Dong Citation2003; Chang et al. Citation2019) or increased (Jo et al. Citation2011; Xu et al. Citation2012). In addition, some studies did not observe a clear trend in Pn following the warming treatment (Llorens et al. Citation2004). The effects of increased temperature on Pn seem to be species-specific (Xu et al. Citation2012).
However, we found that the warming treatment had no significant effect on Pn in the second week, but in the third week, Pn significantly decreased in TW compared to TC. The gs and E responses to the warming treatment were delayed and observed in the third week; therefore, we considered that Pn decreased due to decreased gs and E. On the other hand, in the fourth week, the Pn of TW was not significantly different from that of TC, and the increase in gs and E of TW during the resting stage of the warming treatment was observed in the fourth week. This seems to have enabled the Pn of TW to be as high as that of TC in the fourth week (Oh et al. Citation2005).
Total chlorophyll content (Chlt)
Contrary to our hypothesis, in the third and fourth week, Chlt was higher in PD than in PC, but this difference was statistically significant only in the fourth week (). In general, water stress reduces Chlt (Guerfel et al. Citation2009; Khaleghi et al. Citation2012). However, in the present study, PD showed higher Chlt than PC, which was contrary to the general trend. The increase in Chlt with water stress might be related to hormesis effects (Agathokleous et al. Citation2020). The warming treatment had no significant influence on Chlt (). In previous studies, various changes in Chlt following warming treatments have been reported. Han et al. (Citation2012) reported that the warming treatment of L. kaempferi and Betula costata decreased chlorophyll a and Chlt in TW, which resulted from temperature stress. Contrarily, Zhao and Liu (Citation2009) reported that chlorophyll a, chlorophyll b, and Chlt significantly increased after the warming treatment in Picea asperata and Pinus tabulaeformis, explaining that this increasing trend might be a consequence of the increase in pigment synthesis with increasing temperature. Considering that Chlt is used as a stress indicator (Zarco-Tejada et al. Citation2004), a 4 °C temperature rise in spring might be insufficient to decrease Chlt in L. kaempferi as a reaction to temperature stress.
Correlations among variables
Bivariate correlations among the nine parameters are shown in . The results showed a significant correlation among most variables related to the physiological responses of seedlings and environmental factors. Air temperature was positively correlated with soil temperature (r = 0.323, p < .001), accumulated temperature (r = 0.436, p < .001), and drought duration (r = 0.210, p < .05), but showed a negative correlation with Chlt (r = −0.272, p < .001). However, no correlations were observed between the air temperature and soil moisture content gs, E, and Pn. Soil temperature was negatively correlated with soil moisture content (r = −0.288, p < .001), accumulated temperature (r = −0.355, p < .001), gs (r = −0.443, p < .001), E (r = −0.364, p < .001), and Pn (r = −0.610, p < .001). The significant correlation between air and soil temperatures suggested that the increase in air temperature led to an increase in soil temperature. Various physiological responses correlated more strongly with soil temperature than with air temperature, which was in accordance with a previous study indicating that soil temperature is more important than air temperature for plant growth (Xu and Huang Citation2000). Furthermore, regarding the general tendency, gs, E, and Pn were significantly negatively correlated with soil temperature but showed a positive correlation with soil moisture content and accumulated temperature. In contrast, Chlt was positively correlated with soil temperature but negatively correlated with soil moisture content and accumulated temperature, indicating that Chlt increased in an environment stressful for L. kaempferi growth, which resulted from the hormesis effect (Agathokleous et al. Citation2020). Thus, these parameters can be used to predict the physiological response such as gs, E, Pn, and Chlt of seedlings with values of environmental factors derived from future climate change prediction models.
Table 2. Bivariate correlations among the measured parameters.
PCA
The existence of multicollinearity was identified through the results of correlations among variables, and PCA was conducted to overcome multicollinearity without reducing the number of variables. Results from the PCA indicated that three PCs with an eigenvalue greater than 1 were extracted, explaining 75.7% of the total variance (). For each factor, characteristics with a PC loading >0.400 were considered significant. According to the results, seedling physiological response parameters including gs, E, and Pn were prevalent in PC1; environmental variables including AT1, SM, AT2, and DD were significant in PC2; and PC3 mainly represented the variables related to directly controlled environmental factors, including AT1 and SM.
Table 3. Eigenvalues, proportion of total variability, as well as correlations between the original variables and the first 3 principal components (PCs).
In addition, a PCA biplot was depicted based on the first two main PCs (accounting for 60.3% of the variance) (). Biplots generated by PCA are used to interpret the differences among accessions based on the effective characters in the first two components, and the ordination of accessions in close proximity within the ordination plot indicates their similarity (Vichi and Saporta Citation2009). Treatments with PC1 scores >0 were identified as having greater physiological performance, and those with PC1 scores <0 were identified as having poorer physiological performance (). PC2, which was related to environmental conditions and higher PC2 scores, indicated a harsher environment for seedlings. Group 1 consisted of TC*PC and TW*PC, which had greater physiological performance and better environmental conditions, with higher PC1 and lower PC2 scores. Group 2 consisted of TC*PD and TW*PD, which had poorer physiological performance and harsher environmental conditions, with lower PC1 and higher PC2 scores. This suggested that four weeks of drought were more stressful for L. kaempferi than a 4 °C increase in temperature biweekly for seven days.
Conclusion
This study was conducted to investigate the short-term physiological responses of L. kaempferi seedlings in spring to warming treatment, the resting stage of the warming treatment, and drought treatment. L. kaempferi seedlings responded differently to the timing of the measurement. We found that gs and E decreased after the warming and drought treatments and increased after the resting stage of the warming treatment. Chlt showed no significant difference before and after the warming and drought treatments until the third week but increased in the fourth week of the drought treatment. We believe that the drought treatment did not cause a high level of stress and that the hormesis effect of chlorophyll occurred. In addition, we confirmed that Pn decreased in the warming and drought treatments. The effect of warming may not be immediately visible and may be delayed. Consequently, warming and drought treatments can reduce gs, E, and Pn, affecting seedling growth. Based on the observed correlations among the studied variables, we concluded that environmental factors influenced seedling physiological responses, and soil temperature was more influential than air temperature. Furthermore, the PCA biplot divided the treatments into two groups. The PC groups had better environmental conditions and greater physiological performance, whereas the PD group had harsher environmental conditions and poorer physiological performance. This suggested that four weeks of drought were more stressful for L. kaempferi than a 4 °C increase in temperature biweekly for seven days. Finally, it is noteworthy that this study had a design limitation; the number of plots was insufficient to compare the warming treatment with and without the resting stage. In future studies, it is necessary to clarify our understanding of the resting stage by simultaneously measuring the warming treatment with and without the resting stage. This study is expected to be the basis for further in-depth studies on related topics, e.g. the effects of spring warming and drought on the growth response or phenology of L. kaempferi seedlings.
Acknowledgments
This study was carried out with the support of “R&D Program for Forest Science Technology [Project No. ‘2020181A00-2122-BB01’]” provided by Korea Forest Service (Korea Forestry Promotion Institute).
Disclosure statement
No potential conflict of interest was reported by the author(s).
Correction Statement
This article has been corrected with minor changes. These changes do not impact the academic content of the article.
Additional information
Funding
References
- Agathokleous E, Feng Z, Peñuelas J. 2020. Chlorophyll hormesis: are chlorophylls major components of stress biology in higher plants? Sci Total Environ. 726:138637.
- Ambebe TF, Dang Q-L, Li J. 2010. Low soil temperature inhibits the effect of high nutrient supply on photosynthetic response to elevated carbon dioxide concentration in white birch seedlings. Tree Physiol. 30(2):234–243.
- Bae H, Sicher RC, Kim MS, Kim SH, Strem MD, Melnick RL, Bailey BA. 2009. The beneficial endophyte Trichoderma hamatum isolate DIS 219b promotes growth and delays the onset of the drought response in Theobroma cacao. J Exp Bot. 60(11):3279–3295.
- Barnes JD, Balaguer L, Manrique E, Elvira S, Davison AW. 1992. A reappraisal of the use of DMSO for the extraction and determination of chlorophylls a and b in lichens and higher plants. Environ Exp Bot. 32(2):85–100.
- Chang H, Han SH, An J, Park MJ, Son Y. 2019. Relationship between soil water and physiological and growth responses of Pinus densiflora seedlings under open-field experimental warming and precipitation manipulation. ksccr. 10(2):145–152.
- Chung H, Muraoka H, Nakamura M, Han S, Muller O, Son Y. 2013. Experimental warming studies on tree species and forest ecosystems: a literature review. J Plant Res. 126(4):447–460.
- Collatz GJ, Ball JT, Grivet C, Berry JA. 1991. Physiological and environmental regulation of stomatal conductance, photosynthesis, and transpiration: a model that includes a laminar boundary layer. Agric for Meteorol. 54(2–4):107–136.
- Crane JL, Jr., Dickmann DI, Flore JA. 1983. Photosynthesis and transpiration by young Larix kaempferi trees: C3 responses to light and temperature. Physiol Plant. 59(4):635–640.
- Cuesta B, Villar-Salvador P, Puértolas J, Jacobs DF, Rey-Benayas JM. 2010. Why do large, nitrogen rich seedlings better resist stressful transplanting conditions? A physiological analysis in two functionally contrasting Mediterranean forest species. For Ecol Manage. 260(1):71–78.
- Farquhar GD, Sharkey TD. 1982. Stomatal conductance and photosynthesis. Annu Rev Plant Physiol. 33(1):317–345.
- Fisichelli N, Wright A, Rice K, Mau A, Buschena C, Reich PB. 2014. First-year seedlings and climate change: species-specific responses of 15 North American tree species. Oikos. 123(11):1331–1340.
- Flanagan LB, Sharp EJ, Letts MG. 2013. Response of plant biomass and soil respiration to experimental warming and precipitation manipulation in a Northern Great Plains grassland. Agric for Meteorol. 173(15):40–52.
- Guerfel M, Baccouri O, Boujnah D, Chaibi W, Zarrouk M. 2009. Impacts of water stress on gas exchange, water relations, chlorophyll content and leaf structure in the two main Tunisian olive (Olea europaea L.) cultivars. Sci Hortic. 119(3):257–263.
- Han SH, Kim DH, Kim GN, Lee JC, Yun CW. 2012. Changes on initial growth and physiological characteristics of Larix kaempferi and Betula costata seedlings under elevated temperature. Korean J Agric Forest Meteorol. 14(2):63–70.
- Hao P, Zhu J, Gu A, Lv D, Ge P, Chen G, Li X, Yan Y. 2015. An integrative proteome analysis of different seedling organs in tolerant and sensitive wheat cultivars under drought stress and recovery. Proteomics Syst Biol. 15(9):1544–1563.
- He WM, Dong M. 2003. Plasticity in physiology and growth of Salix matsudana in response to simulated atmospheric temperature rise in the Mu Us Sandland. Photosynt. 41(2):297–300.
- IPCC. 2018. Global warming of 1.5 °C. An IPCC Special Report on the impacts of global warming of 1.5 °C above pre-industrial levels and related global greenhouse gas emission pathways, in the context of strengthening the global response to the threat of climate change, sustainable development, and efforts to eradicate poverty. Cambridge (UK): Cambridge University Press; p. 51–54.
- Jo W, Son Y, Chung H, Noh NJ, Yoon TK, Han S, Lee SJ, Lee SK, Yi K, Jin L. 2011. Effect of artificial warming on chlorophyll contents and net photosynthetic rate of Quercus variabilis seedlings in an open-field experiment. J Korean For Soc. 100(4):733–737.
- Khaleghi E, Arzani K, Moallemi N, Barzegar M. 2012. Evaluation of chlorophyll content and chlorophyll fluorescence parameters and relationships between chlorophyll a, b and chlorophyll content index under water stress in Olea europaea cv. Dezful. World Acad Sci Eng Technol. 68:1154–1157.
- Kim GN, Han SH, Park GS. 2014. Difference on growth, photosynthesis and pigment contents of open-pollinated Pinus densiflora families under elevated temperature and drought. Korean Journal of Agricultural and Forest Meteorology. 16(4):285–296.
- Kimball BA, Conley MM, Wang S, Lin X, Luo C, Morgan J, Smith D. 2008. Infrared heater arrays for warming ecosystem field plots. Global Change Biol. 14(2):309–320.
- Korea Forest Service. 2020a. Larch nurturing, it must be different from the forest creation. [accessed 2020 Dec 01]. https://huyang.forest.go.kr/kfsweb/cop/bbs/selectBoardArticle.do?nttId=3149677&bbsId=BBSMSTR_1036&pageUnit=10&pageIndex=1&searchtitle=title&searchcont=&searchkey=&searchwriter=&searchWrd=&ctgryLrcls=&ctgryMdcls=&ctgrySmcls=&ntcStartDt=&ntcEndDt=&mn=NKFS_01_01&orgId=.
- Korea Forest Service. 2020b. [accessed 2021 Jan 06]. Start production of cloned larch seedlings for economic forest development. https://nifos.forest.go.kr/kfsweb/cop/bbs/selectBoardArticle.do;jsessionid=aIMJ8WfBNqtyuhk7ttW6wJsMpyuUB2jDi1CC7LPVOljdWlsq4gG3CpCJOqd9wEs7.frswas02_servlet_engine5?nttId=3145455&bbsId=BBSMSTR_1036&pageUnit=10&pageIndex=1&searchtitle=title&searchcont=&searchkey=&searchwriter=&searchWrd=&ctgryLrcls=CTGRY150&ctgryMdcls=&ctgrySmcls=&ntcStartDt=&ntcEndDt=&mn=UKFR_03_03&orgId=kfri.
- Korea Meteorological Administration. 2020. Climate Information Portal. [accessed 2020 Oct 14]. http://www.climate.go.kr/home/CCS/contents/33_2_areapoint_basic.php.
- Kwak BH, Yoon KE. 2008. Plant Physiology. Seoul, Korea: Hyang Moon Sa. (Korean Literature); p. 333.
- Lee SJ, Han S, Yoon TK, Jo W, Han SH, Jung Y, Son Y. 2013. Changes in chlorophyll contents and net photosynthesis rate of 3-year-old Quercus variabilis seedlings by experimental warming. J Korean For Soc. 102(1):156–160.
- Lewis JD, Lucash M, Olszyk D, Tingey DT. 2001. Seasonal patterns of photosynthesis in Douglas fir seedlings during the third and fourth year of exposure to elevated CO2 and temperature. Plant Cell Environ. 24(5):539–548.
- Li C. 1998. Some aspects of leaf water relations in four provenances of Eucalyptus microtheca seedlings. For Ecol Manage. 111(2–3):303–308.
- Lipiec J, Doussan C, Nosalewicz A, Kondracka K. 2013. Effect of drought and heat stresses on plant growth and yield: a review. Int Agrophy. 27(4):463–477.
- Llorens L, Peñuelas J, Beier C, Emmett B, Estiarte M, Tietema A. 2004. Effects of an experimental increase of temperature and drought on the photosynthetic performance of two ericaceous shrub species along a north-south European gradient. Ecosystems. 7(6):613–624.
- NIFoS (National Institute of Forest Science). 2014. IPCC 5th report on climate change and its lmplications. Seoul: NIFoS (Korean Literature).
- Oh CY, Han SH, Kim YY, Lee JC. 2005. Changes of drought tolerance and photosynthetic characteristics of Populus davidiana dode according to PEG Concentration. Korean J Agricu Forest Meteorol. 7(4):296–302.
- Saxe H, Cannell MGR, Johnsen B, Ryan MG, Vourlitis G. 2001. Tree and forest functioning in response to global warming. New Phytol. 149(3):369–399.
- Vichi M, Saporta G. 2009. Clustering and disjoint principal component analysis. Comput Stat Data Anal. 53(8):3194–3208.
- Wan S, Luo Y, Wallace LL. 2002. Changes in microclimate induced by experimental warming and clipping in tallgrass prairie. Global Change Biol. 8(8):754–768.
- Weih M. 2000. Delayed growth response of Mountain Birch seedlings to a decrease in fertilization and temperature. Funct Ecol. 14(5):566–572.
- Wu Z, Dijkstra P, Koch GW, Peñuelas J, Hungate BA. 2011. Responses of terrestrial ecosystems to temperature and precipitation change: a meta‐analysis of experimental manipulation. Global Change Biol. 17(2):927–942.
- Xu Q, Huang B. 2000. Growth and physiological responses of creeping bentgrass to changes in air and soil temperatures. Crop Sci. 40(5):1363–1368.
- Xu ZF, Hu TX, Zhang YB. 2012. Effects of experimental warming on phenology, growth and gas exchange of treeline birch (Betula utilis) saplings, Eastern Tibetan Plateau, China. Eur J Forest Res. 131(3):811–819.
- Yura H. 1989. Comparative ecophysiology of Larix kaempferi (Lamb.) Carr. and Abies veitchii Lindl. II. Mechanisms of higher drought resistance of seedlings of L. kaempferi as compared with A. veitchii. Ecol Res. 4(3):351–360.
- Zarco-Tejada PJ, Miller JR, Morales A, Berjón A, Agüera J. 2004. Hyperspectral indices and model simulation for chlorophyll estimation in open-canopy tree crops. Remote Sens Environ. 90(4):376–463.
- Zhao C, Liu Q. 2009. Growth and photosynthetic responses of two coniferous species to experimental warming and nitrogen fertilization. Can J for Res. 39(1):1–11.