ABSTRACT
Background: Retrotransposition of long interspersed nuclear element-1 (L1-RTP) is proposed to contribute to central nervous system (CNS) plasticity by inducing mosaicism of neuronal cells. Clinical studies have identified increased L1 copy numbers in the brains of patients with psychiatric disorders. These observations implicate that L1-RTP is important for neurogenesis and that its deregulation represents a risk factor for mental disorders. However, no supportive evidence is available for understanding the importance of L1-RTP in CNS function. Findings: To explore the physiological role of L1-RTP in CNS, we examined the L1 copy number during maturation. Interestingly, the L1 copy number increased after birth in the mouse hippocampus, but not the frontal lobe, with maximal copy numbers found in 8-week-old mice. This age-dependent L1 increase was abolished by administration of a reverse-transcriptase inhibitor, stavudine (d4T), which showed no toxic effects. Notably, the age-dependent L1 increase was attenuated by post-weaning social isolation (SI) stress, a well-known intervention for inducing psychiatric disorders in mice, or deletion of the NR2A gene that encodes a subunit of the glutamate receptor. Moreover, the negative effects of SI stress on L1-RTP were partially restored by environmental enrichment with voluntary running, but not by fluoxetine, a commonly used anti-psychiatric drug. Finally, behavioral experiments revealed that learning memory was defective in d4T-treated mice, which was similarly observed in mice raised under SI stress. Conclusion: We detected the modulation of L1-RTP in the hippocampus during maturation of the CNS. In a recent study, we demonstrated that stimulants such as methamphetamine and cocaine were active in the induction of L1-RTP in neuronal cells, and previous studies have shown that NR2A-deficient mice are susceptible to mental abnormality. Herein, our data support the notion that the age-dependent modulation of L1-RTP is involved in genome differentiation in the hippocampus, and that modulation defects are linked to the development of psychiatric disorders.
Introduction
Long interspersed nuclear element-1 (L1) is an endogenous retroelement, comprising 17% of the human genome.Citation1 It has more than 5 × 105 copies in the human genome, 80–100 of which are active and competent for retrotransposition (L1-RTP).Citation2 L1 is 6 kb in length and encodes 2 proteins named open reading frame 1 and 2 (ORF1 and ORF2), the expressions of which are regulated by 5′ untreated region (UTR).Citation2 Notably, L1 is active in neuronal precursor cells, and L1-RTP insertions provoke the expression of neuron-specific genes when their gene structures are altered by de novo L1 insertion.Citation3–5 Recent studies have identified L1-mediated somatic mosaicism in normal adult brains,Citation6,7 and high copy numbers of L1 were detected in the brain of a schizophrenia patient,Citation8 Okudaira et al. recently reported that stimulants such as methamphetamine and cocaine induced L1-RTP in neuronal cells,Citation9,10 and Moszczynska et al. demonstrated that glutamate activated L1-RTP.Citation10 These observations imply that L1-RTP is physiologically regulated via the axis of glutamate and the glutamate receptor and that normal brain maturation is affected by the deregulation of L1-RTP, which functions as a molecular basis of psychiatric disorders. However, experimental evidence is required to prove the importance of L1-RTP in CNS function.
Post-weaning social isolation (SI) is a commonly used rodent model of environmental stress that causes abnormal behavior or psychiatric disorders.Citation11–16 Generally, SI stress impairs neuronal precursor cells at multiple steps, including the mitotic and survival phases of neurogenesis.Citation17 SI also suppresses the expression of glutamate receptor,Citation18 which functions in learning and memory.Citation19 In contrast, SI-induced abnormalities can be rescued by environmental enrichment,Citation14 and fluoxetine, an antidepressant that selectively inhibits serotonin re-uptake.Citation17,20 Interestingly, environmental enrichment ameliorates SI-induced defects at both the mitotic and post-mitotic phases of hippocampal neurogenesis,Citation21 whereas fluoxetine mainly protects against the SI-induced downregulation of cell proliferation.Citation17
To investigate the physiological roles of L1-RTP in the CNS, we first examined L1 copy numbers in the hippocampus genome of mice at different ages. Interestingly, we found that L1 copy numbers in the hippocampus were higher in 8-week-old mice than in 3-week-old mice. Notably, this age-dependent increase in L1 copy numbers in the hippocampus was attenuated by SI. Moreover, it was absent in knockout mice that were deficient in NR2A, a gene encoding a subunit of N-methyl-D-aspartate receptor (NMDA), which is a glutamate receptor. Taken together with data that blockade of the age-dependent L1-RTP in the hippocampus by d4T caused behavioral abnormalities, these data suggest the importance of age-dependent L1 increases in brain maturation. This is the first study to show the age-related oscillation of L1 in the hippocampus, providing novel insights into the physiological role of L1-RTP in genome differentiation.
Results
Increased copy numbers of L1 in the hippocampus during maturation
We measured L1 copy numbers by amplifying 3 different regions of L1, encompassing ORF2, ORF1, and L1-5′ UTR DNA in brain samples of mice at 3 and 8 weeks after birth. Quantitative polymerase chain reaction (qPCR) revealed that the L1 copy numbers in the hippocampus of 8-week-old mice were 1.6-fold higher than those of 3-week-old mice (Steel–Dwass test, ORF2: P = 0.008, ; Tukey's test, 5′UTR: P = 0.0001, ORF1: P = 0.00003, ). In contrast, no increase in L1 copy numbers was observed in the frontal lobes (). To exclude the effects of contaminated RNA-hybiridsed L1 DNA,Citation22 we re-evaluated copy numbers of L1-ORF2 after treating DNA samples with RNaseH and exonuclease 1, but obtained similar results (Mann–Whitney U test, ORF2 / 5S rDNA: P = 0.02, ORF2 / β-globin: P = 0.01, Figure S1). Additional analysis of the DNA samples of mice at 15, 18, 20, and ∼55 weeks after birth indicated that the L1 copy numbers were maximal at 8 weeks after birth ().
Figure 1. Age-dependent increases in L1 in the mouse hippocampus genome. (A) Age-related variation in the copy numbers in the hippocampus (left panel) and frontal cortex (right panel). Six groups (3-, 8-, 15-, 18-, 20-, and 55-week-old) of C57BL/6J inbred mice were subjected to quantitative polymerase chain reaction (qPCR) analysis. The copy numbers of L1-ORF2 were measured using the 5S ribosomal gene as a reference. We analyzed a total of 42 mice (21 female, 21 male) at 3 (3 female, 3 male), 8 (6 female, 6 male), 15 (3 female, 3 male), 18 (3 female, 3 male), 20 (3 female, 3 male) and 55 (3 female, 3 male) weeks of age. The error bars show the standard error of the mean (SEM) values. The copy numbers in the hippocampus were significantly higher in 8-week-old inbred mice (Steel–Dwass test, 3-week-old vs 8-week-old, P = 0.008; 8-week-old vs 15-week-old, P = 0.4; 8-week-old vs 18-week-old, P = 0.08; 8-week-old vs 20-week-old, P = 0.0001). (B) The copy numbers of L1-5′UTR and -ORF1. The measurement analysis was done using the 5S ribosomal gene as a reference. A total of 18 mice (9 female, 9 male) were analyzed at 3 (3 female, 3 male), 8 (3 female, 3 male), and 20 (3 female, 3 male) weeks of age. Bars show the SEM. The copy numbers in the hippocampus were significantly higher in 8-week-old inbred mice (Tukey's test, 5′UTR; P = 0.0001, ORF1; P = 0.00003). (C) Stavudine (d4T)-induced inhibition of the age-dependent L1 increase in the hippocampus genome. Mice were administered d4T in drinking water beginning 3 weeks after birth and continued for 5 weeks, and then the L1 copy numbers in the hippocampus genome were analyzed. Bars show the SEM. The analysis included 47 control mice (3-week-old; 24 female, 8 male, 8-week-old; 9 female, 6 male) and 12 d4T-treated mice (3 female, 9 male). Of note, d4T treatment inhibited the L1 increase in 8-week-old mice (Steel–Dwass test, P = 0.0000009). (D) Representative results of the immunohistochemical analysis of d4T-treated mice. Ki67 (red; arrowheads), calretinin (green), 5-ethynyl-2-deoxyuridine (EdU) (red) and calretinin-EdU double-positive cells (yellow; arrowheads, inset) were visualised in the dentate gyrus of d4T-treated mice. Nuclei are depicted in blue. Scale bar, 50 µm. (E) Comparison of Ki67-positive cells and calretinin-EdU double-staining cells in mice with or without d4T treatment. Under graph shows calretinine-EdU double-staining cells (blue column) and EdU-positive cells (red column). The analysis included a total of 16 mice (8 female, 8 male); 8 control mice (4 female, 4 male) and 8 d4T-treated mice (4 female, 4 male). Ki67-positive cells and calretinin-EdU double-staining cells were counted in 3 randomly selected sections that were prepared from each mouse.
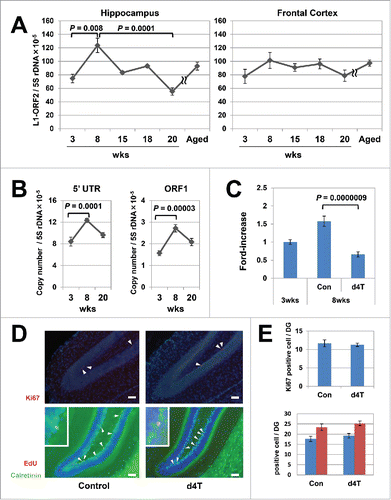
Previously, we observed that d4T, a reverse-transcriptase inhibitor (RTI), reproducibly attenuated L1-RTP both in vitro and in vivo.Citation23,24 To confirm that the increase in L1 copy numbers was dependent on RT activity, we administered d4T to mice in drinking water at a concentration of 25 mM, and performed the same analysis. When d4T was continuously administered from 3 to 8 weeks after birth, the L1 increase in the hippocampus was abolished (Steel–Dwass test, P = 0.0000009; ). No apparent changes in body weight or volume of water intake were observed. To exclude the possibility that d4T has toxic effects on neurogenesis, we carried out 2 different experiments. First, we counted the numbers of Ki67-positive cells in the dentate gyrus of the hippocampus in mice that received d4T from 3 to 6 weeks after birth (). No difference was observed in d4T-treated and control mice, indicating that d4T does not impair proliferation of neuronal precursor cells. Second, we injected 5-ethynyl-2-deoxyuridine (EdU) into 3-week-old mice, administered d4T for 1 week, and sacrificed the mice for analysis. As shown in , we observed no difference in d4T-treated and control mice in the numbers of cells that were double positive for EdU and calretinin signals. Given that calretinin is expressed in immature neuronal cells, these data suggest that d4T did not have toxic effects during the early phase of neuronal cell maturation and cell survival.
Based on these observations, we attributed the age-dependent L1 increase in the hippocampus to the RT activity-dependent induction of L1-RTP.
Post-weaning SI blocked the age-dependent L1 increase, which was partially rescued by environmental enrichment
There is evidence that SI stress induces behavioral abnormalities in mice at various ages.Citation21,25,26 When raised under post-weaning SI, mice also show abnormal behavior, possibly due to impaired brain maturation.Citation27,28 To determine the effects of environmental stress on the age-dependent L1 increase, we reared mice in isolation beginning 3 weeks after birth, and determined the L1 copy numbers at 8 weeks. As shown in , we observed no significant increase in the L1 copy numbers in these mice. Interestingly, however, environmental enrichment restored the SI-induced down-modulation of the L1 copy numbers, whereas fluoxetine treatment did not (Steel–Dwass test, 8-week-olds raised in group housing vs. post-weaning SI P = 0.001; post-weaning SI vs. post-weaning SI with environmental enrichment P = 0.03; ).
Figure 2. Age-dependent L1 increase in the hippocampus genome coupled with brain maturation. (A) Effects of social isolation (SI) and environmental enrichment (EE) on the age-dependent L1 increase. Mice were raised under SI stress for 5 weeks, beginning 3 weeks after birth, and the L1 copy numbers in the hippocampus genome were measured (Steel–Dwass test, group housing vs. SI; P = 0.001). The effects of fluoxetine treatment (Flx) and EE on the L1 copy numbers were analyzed under the same conditions. The analysis included a total of 35 mice (18 female, 17 male); 14 mice (8 female, 6 male) were raised under SI conditions, 11 (5 female, 6 male) under SI + EE conditions, and 10 (5 female, 5 male) under SI+Flx treatment. EE, but not Flx, restored the SI-induced inhibition of the age-dependent L1 increase (Steel–Dwass test, P = 0.03). The average running distance in the EE group was 23.28 km/day. (B) Inhibitory effects of mice lacking the NR2A. A total of 27 mice (13 female, 14 male): 13 wild-type mice (3-week-old; 3 female, 3 male, 8-week-old; 3 female, 4 male), 8 hetero-knockout (8-week-old; 4 female, 4 male) and 6 null mutant (8-week-old; 3 female, 3 male) mice with the same genotypes were raised in the same cages, and the L1 copy numbers in the hippocampus genome were measured at 8 weeks of age (Steel–Dwass test, wild-type vs. null knockout, P = 0.0003, wild-type vs. null hetero-type, P = 0.0008). (C) d4T administration induced abnormalities in passive avoidance. The response latency in the passive avoidance test was significantly shortened in the d4T-treated mice (Steel–Dwass test, P = 0.03). Again, the results were similar to those for the post-weaning SI mice (P = 0.02). (D) d4T administration induced abnormalities in the light/dark transition. The light/dark transition increased significantly in the d4T-treated mice (Tukey's test, P = 0.0001). The results were similar to those for the post-weaning SI mice (P = 0.008). The behavioral assay included a total of 21 mice (6 female, 15 male): 6 control mice (3 female, 3 male), 9 d4T-treated mice (3 female, 6 male), and 6 SI-treated mice (0 female, 6 male).
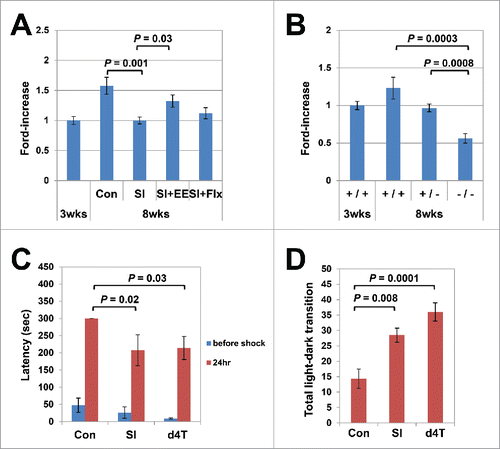
The glutamate receptor gene is required for the age-dependent increase in L1 in the hippocampus
To examine the effects of glutamate receptor on age-dependent increases in L1, quantitative analysis of L1 was performed on the hippocampus genome that was derived from mice lacking the NMDA-type glutamate receptor ε1 (NR2A) subunit.Citation29 NR2B, which is a subunit of the NMDA-type glutamate receptor, is dominantly expressed in the hippocampus of newborns, and is gradually replaced with NR2A during growth to adolescence.Citation30 Knockout of NR2B is neonatal lethal, whereas NR2A−/− mice can be maintained.Citation31 We compared the L1 copy numbers in the hippocampus of 3 groups of 8-week-old mice with different genotypes (wild-type, hetero-knockout, and null knockout litters) and observed that the copy numbers of L1 in knockout mice were significantly lower than in wild-type mice (Steel–Dwass test, wild-type vs. null knockout; P = 0.0003, ). In addition, the copy numbers were also significantly lower in haplo-deficient mice than in wild-type mice (Steel–Dwass test, wild-type vs. null hetero-type, P = 0.0008).
Continuous administration of d4T induced aberrant psychiatric behavior
Consistent with previous reports, we observed that mice raised under post-weaning SI showed abnormal scores on behavioral assays (), implying that the age-dependent L1 increase was linked to physiological brain maturation. To explore this, we conducted behavioral assays using mice that were treated with d4T. Mice were administered d4T in drinking water beginning 3 weeks after birth, and the behavior was assessed at 8 weeks. As shown in , when the passive avoidance test was administered 24 h after a shock, the d4T-treated mice showed a significant reduction in the response latency (Steel's test, P = 0.03). Similar results were observed in mice raised under post-weaning SI stress (P = 0.02). Moreover, the d4T-treated mice showed a significant increase in the frequency of light–dark transitions (Tukey's multiple comparisons test, d4T, P = 0.0001; SI, P = 0.008, ).
Discussion
L1 copy numbers in the mouse hippocampus change with age
We found that the L1 copy numbers in the hippocampus increased up to 8 weeks after birth, and then decreased with aging. It has been proposed that L1 causes neuronal cell multiplication and contributes to CNS plasticity during brain development. Citation3–5,32 Consistent with this hypothesis, Evrony et al. demonstrated L1 insertions during brain development, which enabled cell linage tracing in the brain.Citation6 On the other hand, van Praag et al. reported that more than half of the cells in the adult dentate gyrus of 12-week-old mice do not survive during 4 weeks.Citation33 In addition, the expression of L1 elements was downregulated with aging.Citation34 These observations suggest that the L1 copy numbers in the CNS genome are abundant early in development, and gradually decrease with age. To our surprise, however, the L1 copy numbers in the hippocampus were at maximum levels at 8 weeks after birth. To investigate the mechanism of age-dependent L1-RTP, we compared the expression levels of L1 mRNA between the hippocampuses of 3- and 8-week-old mice. The expression level of L1 mRNA in the 8-week-old mice appeared lower than that of the 3-week-old mice, although the difference was not statistically significant (Figure S2). Considering a recent report by Moszczynska et al. that glutamate functions as a positive factor for the induction of L1-RTP,Citation10 we measured the L1 copy numbers in NR2A knockout mice. Notably, no L1 increase was observed in 8-week-old NR2A knockout mice, suggesting that the age-dependent L1-RTP is dependent upon an intact glutamate receptor. We also observed that L1 copy numbers in the hippocampus decreased after 8 weeks of age. Based on the finding by van Praag et al.,Citation33 it is plausible that mice over 8 weeks of age demonstrate turnover of neuronal cells in the hippocampus, with those cells being less competent for L1-RTP, resulting in decreased L1 copy numbers.
L1 inhibition is linked with psychiatric disorders and blockade of L1-RTP is coupled with behavioral abnormalities
Learning and memory are hampered by gene knockout of the glutamate receptor,Citation35 administration of NMDA receptor inhibitors (MK801)Citation36 and impaired environmental conditions such as post-weaning SI.Citation20 Here, we observed that both SI stress and knockout of a NMDA receptor subunit NR2A gene attenuated the L1 increase in the hippocampus genome, implicating the possible link between age-dependent L1-RTP and normal maturation. In this study, we examined whether suppression of L1-RTP impairs normal brain maturation. Surprisingly, d4T-induced blockage of L1-RTP caused abnormal behaviors similar to those of SI mice. To exclude toxic effects of d4T, we conducted extensive immunohistochemical analysis and observed no apparent difference in the numbers of Ki67-, calretinin- and EdU-positive cells that survived in the hippocampus compared with control mice. Although further studies are required to exclude the possibility of other undefined toxic effects of d4T, our results currently support the idea that the impairment of the age-dependent L1 increase in the hippocampus is linked to behavioral abnormalities.
Interestingly, environmental enrichment significantly restored the L1 copy numbers in post-weaning SI-mice, whereas fluoxetine did not. It has been proposed that SI affects multiple steps in neurogenesis, including the mitotic and post-mitotic phases of neuroprogenitor cells, and that environmental enrichment improves the SI-induced neuronal defects during both phases of neurogenesis.Citation21 Interestingly, however, fluoxetine has been shown to protect against the SI-induced defect mainly during the mitotic phase of neurogenesis,Citation17,37 leading us to speculate that the age-dependent L1 increase is regulated by the post-mitotic step of neurogenesis. Recently, it was reported that stimulants such as methamphetamineCitation9,10 and cocaine,Citation9 or neurotransmitters such as glutamate and dopamineCitation10 reproducibly induced L1-RTP in neuronal cells. These observations imply that multiple environmental factors are capable of inducing L1-RTP in the brain. Further studies are required to identify exogenous compounds affecting L1-RTP that can be used to define the physiological role of L1-RTP in the CNS.
Materials and methods
Animals
C57BL/6J mice were purchased from CLEA Japan (Tokyo, Japan), and bred and maintained in the animal facility at the National Center for Global Health and Medicine. They were housed in standard 25 × 18 × 15 cm cages in a room maintained at 21 ± 2°C with a 12-h light-dark cycle. Food and water were available ad libitum. A total of 107 female and 104 male mice were used in the study. All experiments were conducted in accordance with the guidelines of the Animal Care Committee of the National Center for Global Health and Medicine.
To study chronological changes in the L1-ORF2 copy numbers, 6 groups of mice at various ages (3, 8, 15, 18, 20, and ∼55 weeks) were analyzed. Six mice from each group (3 males and 3 females) and 6 8-week-old mice used for validation (3 males and 3 females) were deeply anaesthetised and perfused transcardially with cold 4% paraformaldehyde in phosphate-buffered saline (PBS), and genomic DNA was extracted from the right and left hippocampi and the prefrontal cortex. In addition, the same hippocampus samples from 3 groups (3-, 8-, and 20-week-old mice) were used for copy number analysis of L1-5′UTR and ORF1. For further validation, the same analysis of L1-ORF2 copy numbers was performed using non-fixed hippocampus samples from 3-week-old (n = 32) and 8-week-old (n = 15) mice in groups of 3 per cage after weaning.
After weaning, 12 3-week-old C57BL/6J mice were treated with 2′,3′-didehydro-3′-deoxythymidine (stavudine, d4T; Tokyo Chemical Industry, Tokyo, Japan) in drinking water (25 μM). The maximum average intake of d4T was 5 μM/kg/day. These mice were reared in groups of 2–3 per cage. Twelve mice were sacrificed and perfused transcardially with cold PBS at 8 weeks of age. Genomic DNA was extracted from the right and left hippocampi. For behavioral assays, we used 9 d4T-treated and 6 untreated control mice; for immunohistochemical analysis of Ki67 as a marker of neurogenesis, we used 4 d4T-treated and 4 untreated control mice; and for EdU cell survival analysis, we used 4 d4T-treated and 4 untreated control mice.
Mutant mice lacking the NR2A subunit of NMDA receptorCitation29 (GluR Epsilon1 KO mouse; RBRC01813) were provided by RIKEN BRC through the National Bio-Resource Project of the MEXT, Japan. Six 3-week-old wild-type mice were used and 7 wild-type, 8 hetero-knockout (+/−) and 6 null (−/−) mutant mice were prepared mainly as littermates and reared in group housing (3–4/cage) to 8 weeks after birth for each genotype.
Quantitative analysis of L1 copy number
Genomic DNA was isolated from formalin-fixed tissue using the ReliaPrep™ FFPE gDNA Miniprep System (Promega, Madison, WI, USA) and from non-fixed tissue using the QuickGene nucleic acid isolation system (Kurabo Industries, Osaka, Japan). To remove the ssDNA or DNA/RNA hybrids in each genomic DNA sample, 1 U RNaseH (New England Biolabs, Ipswich, MA, USA) and 20 U exonuclease I (TaKaRa Bio, Shiga, Japan) were used to treat 10 ng genomic DNA in surplus. mRNA was isolated from non-fixed tissue using RNeasy Mini Kit (Qiagen Hamburg, Germany) and synthesized cDNA was generated using Omniscript Reverse Transcription Kit (Qiagen).
Genomic DNA and cDNA were analyzed by qPCR using TaqMan probe methods with EagleTaq Master Mix (ROX) (Roche Diagnostics, Mannheim, Germany) and SsoAdvanced Universal Probes Supermix (Bio-Rad Laboratories, Hercules, CA, USA). The oligonucleotide probes and primer sets used to detect the L1-ORF1, L1-ORF2 and 5S ribosomal genes were reported by Vitullo et al.Citation38 The TaqMan probe (5′-AGGCGGTCTCCCATCCAA-3′) and primers used for detecting the L1-5'UTR were designed using the nucleotide sequence reported by Muotri et al.Citation3 The probe and primer set for the β-globin gene (NC_000073) were designed by the Oligo@SIGMA-DLP service (Sigma-Aldrich, Tokyo, Japan): forward primer 5′TCTGCTATTGTCTATGCACAAAGG-3′, reverse primer 5′GCTAGATGCCCAAAGGTCTTCA-3′, and TaqMan probe 5′TCCCCTAGAGAAAAACTGTCAATTGTGGGG-3′. The primer set for the β-actin gene (forward primer 5′-GAGGGAAATCGTGCGTGA-3′, reverse primer 5′-AGAAGGAAGGCTGGAAAA-3′) was used in qPCR using SYBR Green methods with SYBR® Premix Ex Taq™ II (TaKaRa Bio, Shiga, Japan). For each qPCR reaction, 2 ng of genomic DNA was used. Standard curves of clone DNA inserting target DNA for L1 and genomic DNA for the 5S rDNA gene and β-globin gene were established. L1 copy numbers were estimated using the 5S rDNA gene and β-globin gene as a reference for the genome, and the β-actin gene was used as a reference for expression analysis.
Effects of environmental enrichment and fluoxetine on the SI-induced down-modulation of L1-RTP
For the SI study, 41 C57BL/6J mice were reared 1 per cage after weaning. To investigate the effects of environmental enrichment and fluoxetine on the SI-induced down-modulation of L1-RTP, 35 mice were studied: 14 mice were raised under SI without any care; 10 mice were isolated with fluoxetine treatment; and 11 mice were isolated with environmental enrichment. In the fluoxetine-treated group (fluoxetine HCl; Tokyo Chemical Industry, Tokyo, Japan) the treatment was initiated at the same time as the SI was started. The concentration of fluoxetine in drinking water was 80 mg/L and the average intake was 16 mg/kg/day. In the environmental enrichment group, SI mice were housed in a cage with a Fast-Trac Amber running disc (K3250; Bio-Serv, Frenchtown, NJ, USA) and Red Mouse Igloo (K3327) for voluntary running. The running distance was measured with an odometer (CC-VT235W; Cateye, Osaka, Japan). For the behavioral assays, 6 SI mice were used as positive controls.
Behavioral assays
The light/dark box test is used to measure anxiety and learning and memory 10 weeks after birth.Citation39 A mouse was trained in dark (20 × 15 cm) and light (30 × 30 cm) chambers connected by a small tunnel with a trap door. Then, the mouse was placed in the dark chamber and the door was opened. The light transition time to the first exit, total light/dark transition, and total time in the light compartment were recorded for 10 min.
The passive avoidance test was used to investigate hippocampus-dependent association memory. A mouse was placed in a light chamber 1 week after training for the light/dark box test. The time to enter before shock was not significantly different among the untreated control mice, d4T-treated mice, and positive controls with abnormal behavior (i.e. post-weaning SI mice). The trap door was closed when a mouse entered the dark chamber, and then a mild electric foot shock (0.15 mA for 2 s) was delivered to the dark chamber using an LE 100-26 electric shock generator system (Panlab; Cornella, Spain). The door was opened immediately, and then the mouse, which escaped from the dark chamber, was returned to a new home cage. After 24 h, the mouse was replaced in the light chamber and then the time latency to enter the dark chamber was recorded. In this test, the control group typically shows a normal response, in which it takes them significantly longer to re-enter the dark chamber. When learning and memory are inhibited, the mouse enters the dark chamber sooner.
Tissue processing, immunohistochemical analysis, and cell survival analysis
Mice were deeply anaesthetised and perfused transcardially with cold 4% paraformaldehyde in PBS. The brains were removed and post-fixed for 4 h and 100-μm-thick coronal sections were cut and processed while free-floating. All washing steps were performed using 0.05% Triton-X in Tris-buffered saline, pH 7.4.
Rabbit anti-Ki67 (1:100; ab15580, Abcam, Cambridge, UK) and Rabbit anti-calretinin (1:250; ab702, Abcam, Cambridge, UK) diluted in 3% bovine serum albumin (BSA) in 0.05% Triton-X in Tris-buffered saline were applied to sections, which were then incubated overnight at 4°C. After washing, anti-rabbit Alexa 555 or 488 (1:1,000; Thermo Fisher Scientific Inc., MA, USA) was applied to the sections for 2 h at room temperature. After washing, the sections were stained with Hoechst 33342 (Thermo Fisher Scientific Inc., MA, USA).
Cell survival analysis was performed by Click-iT® EdU Alexa Fluor® 555 Imaging Kit (Thermo Fisher Scientific Inc., MA, USA). Three-week-old mice were administrated with EdU at 10 mg/kg by intraperitoneal injection. One week after injection, mice were perfused transcardially with cold 4% paraformaldehyde and the brain sections were used for the EdU detection step of Click-iT® to estimate the survival of labeled cells after immunohistochemical staining.
Statistical analysis
Data are shown as the mean ± standard error of the mean (SEM). Statistical analysis of the L1 copy numbers was performed using the statistical software, by Ekuseru-Toukei (2015, Social Survey Research Information Co., Ltd) using a one-way analysis of variance (ANOVA) in conjunction with Tukey's test and Kruskal–Wallis one-way ANOVA in conjunction with the Steel–Dwass test for multiple comparisons. RNaseH and exonuclease I treatment of the genome and expression assays were analyzed by Mann–Whitney U test. In the behavioral assays, data for the passive avoidance test were analyzed using the Steel–Dwass test for multiple comparisons. The data for the light/dark test were analyzed using a multiple-way ANOVA with Tukey's test. P-values < 0.05 were considered statistically significant.
Disclosure of potential conflicts of interest
No potential conflicts of interest were disclosed.
Acknowledgments
We are grateful to Dr. K. Iijima, Dr. N. Okudaira and Y. Saito for their help in the current work.
KMGE_S_1211980.zip
Download Zip (70.2 KB)Funding
This study was supported in part by a Grant-in-Aid from the Tokyo Biochemical Research Foundation and a Grant-in-Aid for Research from the Ministry of Health, Labor, and Welfare of Japan (09156296).
References
- Bannert N, Kurth R. Retroelements and the human genome: new perspectives on an old relation. Proc Natl Acad Sci U S A 2004; 101:14572-9; PMID:15310846
- Goodier JL, Kazazian HH Jr. Retrotransposons revisited: the restraint and rehabilitation of parasites. Cell 2008; 135:23-35; PMID:18854152; http://dx.doi.org/10.1016/j.cell.2008.09.022
- Muotri AR, Chu VT, Marchetto MC, Deng W, Moran JV, Gage FH. Somatic mosaicism in neuronal precursor cells mediated by L1 retrotransposition. Nature 2005; 435:903-10; PMID:15959507
- Coufal NG, Garcia-Perez JL, Peng GE, Yeo GW, Mu Y, Lovci MT, Morell M, O'Shea KS, Moran JV, Gage FH. L1 retrotransposition in human neural progenitor cells. Nature 2009; 460:1127-31; PMID:19657334; http://dx.doi.org/10.1038/nature08248
- Baillie JK, Barnett MW, Upton KR, Gerhardt DJ, Richmond TA, De Sapio F, Brennan PM, Rizzu P, Smith S, Fell M, et al. Somatic retrotransposition alters the genetic landscape of the human brain. Nature 2011; 479:534-7; PMID:22037309; http://dx.doi.org/10.1038/nature10531
- Evrony GD, Lee E, Mehta BK, Benjamini Y, Johnson RM, Cai X, Yang L, Haseley P, Lehmann HS, Park PJ, et al. Cell lineage analysis in human brain using endogenous retroelements. Neuron 2015; 85:49-59. PMID:25569347; http://dx.doi.org/10.1016/j.neuron.2014.12.028
- Poduri A, Evrony GD, Cai X, Walsh CA. Somatic mutation, genomic variation, and neurological disease. Science 2013; 341:1237758; PMID:23828942; http://dx.doi.org/10.1126/science.1237758
- Bundo M, Toyoshima M, Okada Y, Akamatsu W, Ueda J, Nemoto-Miyauchi T, Sunaga F, Toritsuka M, Ikawa D, Kakita A, et al. Increased l1 retrotransposition in the neuronal genome in schizophrenia. Neuron 2014; 81:306-13; PMID:24389010; http://dx.doi.org/10.1016/j.neuron.2013.10.053
- Okudaira N, Ishizaka Y, Nishio H. Retrotransposition of long interspersed element 1 induced by methamphetamine or cocaine. J Biol Chem 2014; 289:25476-85; PMID:25053411; http://dx.doi.org/10.1074/jbc.M114.559419
- Moszczynska A, Flack A, Qiu P, Muotri AR, Killinger BA. Neurotoxic methamphetamine doses increase LINE-1 expression in the neurogenic zones of the adult rat brain. Sci Rep 2015; 5:14356; PMID:26463126; http://dx.doi.org/10.1038/srep14356
- Fone KC, Porkess MV. Behavioural and neurochemical effects of post-weaning social isolation in rodents-relevance to developmental neuropsychiatric disorders. Neurosci Biobehav Rev 2008; 32:1087-102; PMID:18423591;http://dx.doi.org/10.1016/j.neubiorev.2008.03.003
- Okada R, Fujiwara H, Mizuki D, Araki R, Yabe T, Matsumoto K. Involvement of dopaminergic and cholinergic systems in social isolation-induced deficits in social affiliation and conditional fear memory in mice. Neuroscience 2015; 299:134-45; PMID:25943484; http://dx.doi.org/10.1016/j.neuroscience.2015.04.064
- Soga T, Teo CH, Cham KL, Idris MM, Parhar IS. Early-Life Social Isolation Impairs the Gonadotropin-Inhibitory Hormone Neuronal Activity and Serotonergic System in Male Rats. Front Endocrinol 2015; 6:172; PMID:26617573; http://dx.doi.org/10.3389/fendo.2015.00172
- Tanaś Ł, Ostaszewski P, Iwan A. Effects of post-weaning social isolation and environment al enrichment on exploratory behavior and ankiety in Wistar rats. Acta Neurobiol Exp 2015; 75:72-9; PMID:25856524
- Seffer D, Rippberger H, Schwarting RK, Wöhr M. Pro-social 50-kHz ultrasonic communication in rats: post-weaning but not post-adolescent social isolation leads to social impairments-phenotypic rescue by re-socialization. Front Behav Neurosci 2015; 9:102; PMID:25983681; http://dx.doi.org/10.3389/fnbeh.2015.00102
- Silva-Gómez AB, Rojas D, Juárez I, Flores G. Decreased dendritic spine density on prefrontal cortical and hippocampal pyramidal neurons in postweaning social isolation rats. Brain Res 2003; 983:128-36; PMID:12914973
- Ibi D, Takuma K, Koike H, Mizoguchi H, Tsuritani K, Kuwahara Y, Kamei H, Nagai T, Yoneda Y, Nabeshima T, et al. Social isolation rearing-induced impairment of the hippocampal neurogenesis is associated with deficits in spatial memory and emotion-related behaviors in juvenile mice. J Neurochem 2008; 105:921-32; PMID:18182044; http://dx.doi.org/10.1111/j.1471-4159.2007.05207.x
- Hermes G, Li N, Duman C, Duman R. Post-weaning chronic social isolation produces profound behavioral dysregulation with decreases in prefrontal cortex synaptic-associated protein expression in female rats. Physiol Behav 2011; 104:354-9; PMID:21185848; http://dx.doi.org/10.1016/j.physbeh.2010.12.019
- Liu C, Li Y, Edwards TJ, Kurniawan ND, Richards LJ, Jiang T. Altered structural connectome in adolescent socially isolated mice. Neuroimage 2016; 139:259-270; PMID:27338515; http://dx.doi: 10.1016/j.neuroimage.2016.06.037
- Koike H, Ibi D, Mizoguchi H, Nagai T, Nitta A, Takuma K, Nabeshima T, Yoneda Y, Yamada K. Behavioral abnormality and pharmacologic response in social isolation-reared mice. Behav Brain Res 2009; 202:114-21; PMID:19447287; http://dx.doi.org/10.1016/j.bbr.2009.03.028
- Grégoire CA, Bonenfant D, Le Nguyen A, Aumont A, Fernandes KJ. Untangling the influences of voluntary running, environmental complexity, social housing and stress on adult hippocampal neurogenesis. PLoS One 2014; 9:e86237. PMID:2446598; http://dx.doi.org/10.1371/journal.pone.0086237
- Malki S, van der Heijden GW, O'Donnell KA, Martin SL, Bortvin A. A role for retrotransposon LINE-1 in fetal oocyte attrition in mice. Dev Cell 2014; 29:521-33; PMID:24882376; http://dx.doi: 10.1016/j.devcel.2014.04.027
- Iijima K, Okudaira N, Tamura M, Doi A, Saito Y, Shimura M, Goto M, Matsunaga A, Kawamura YI, Otsubo T, et al. Viral protein R of human immunodeficiency virus type-1 induces retrotransposition of long interspersed element-1. Retrovirology 2013; 10:83; PMID:23915234; http://dx.doi.org/10.1186/1742-4690-10-83
- Doi A, Iijima K, Kano S, Ishizaka Y. Viral protein R of HIV type-1 induces retrotransposition and upregulates glutamate synthesis by the signal transducer and activator of transcription 1 signaling pathway. Microbiol Immunol 2015; 59:398-409; PMID:25990091; http://dx.doi.org/10.1111/1348-0421.12266
- Lieberwirth C1, Liu Y, Jia X, Wang Z. Social isolation impairs adult neurogenesis in the limbic system and alters behaviors in female prairie voles. Horm Behav 2012; 62:357-66; PMID:22465453; http://dx.doi.org/10.1016/j.yhbeh.2012.03.005
- Yamada C, Saegusa Y, Nahata M, Sadakane C, Hattori T, Takeda H. Influence of Aging and Gender Differences on Feeding Behavior and Ghrelin-Related Factors during Social Isolation in Mice. PLoS One 2015; 10:e0140094; PMID:26448274; http://dx.doi.org/10.1371/journal.pone.0140094
- Greene JR, Kerkhoff JE, Guiver L, Totterdell S. Structural and functional abnormalities of the hippocampal formation in rats with environmentally induced reductions in prepulse inhibition of acoustic startle. Neuroscience 2001; 103:315-23; PMID:11246147
- Gilabert-Juan J, Belles M, Saez AR, Carceller H, Zamarbide-Fores S, Moltó MD, Nacher J. A “double hit” murine model for schizophrenia shows alterations in the structure and neurochemistry of the medial prefrontal cortex and the hippocampus. Neurobiol Dis 2013; 59:126-40; PMID:23891727; http://dx.doi.org/10.1016/j.nbd.2013.07.008
- Sakimura K, Kutsuwada T, Ito I, Manabe T, Takayama C, Kushiya E, Yagi T, Aizawa S, Inoue Y, Sugiyama H, Mishina M. Reduced hippocampal LTP and spatial learning in mice lacking NMDA receptor epsilon 1 subunit. Nature 1995; 373(6510):151-5. PMID:7816096
- Matta JA1, Ashby MC, Sanz-Clemente A, Roche KW, Isaac JT. mGluR5 and NMDA receptors drive the experience- and activity-dependent NMDA receptor NR2B to NR2A subunit switch. Neuron 2011; 70:339-51. PMID:21521618; http://dx.doi.org/10.1016/j.neuron.2011.02.045
- Kutsuwada T, Sakimura K, Manabe T, Takayama C, Katakura N, Kushiya E, Natsume R, Watanabe M, Inoue Y, Yagi T, et al. Impairment of suckling response, trigeminal neuronal pattern formation, and hippocampal LTD in NMDA receptor epsilon 2 subunit mutant mice. Neuron 1996; 16:333-44
- Singer T1, McConnell MJ, Marchetto MC, Coufal NG, Gage FH. LINE-1 retrotransposons: mediators of somatic variation in neuronal genomes? Trends Neurosci 2010; 33:345-54; PMID:20471112; http://dx.doi.org/10.1016/j.tins.2010.04.001
- van Praag H, Kempermann G, Gage FH. Running increases cell proliferation and neurogenesis in the adult mouse dentate gyrus. Nat Neurosci 1999; 2:266-70. PMID:10195220
- Okamoto M, Inoue K, Iwamura H, Terashima K, Soya H, Asashima M, Kuwabara T. Reduction in paracrine Wnt3 factors during aging causes impaired adult neurogenesis. FASEB J 2011; 25:3570-82; PMID:21746862; http://dx.doi.org/10.1096/fj.11-184697
- Bannerman DM, Niewoehner B, Lyon L, Romberg C, Schmitt WB, Taylor A, Sanderson DJ, Cottam J, Sprengel R, Seeburg PH, et al. NMDA receptor subunit NR2A is required for rapidly acquired spatial working memory but not incremental spatial reference memory. J Neurosci 2008; 28:3623-30. PMID:18385321; http://dx.doi.org/10.1523/JNEUROSCI.3639-07.2008
- Flint RW Jr, Noble LJ, Ulmen AR. NMDA receptor antagonism with MK-801 impairs consolidation and reconsolidation of passive avoidance conditioning in adolescent rats: evidence for a state dependent reconsolidation effect. Neurobiol Learn Mem 2013; 101:114-9. PMID:23391691; http://dx.doi.org/10.1016/j.nlm.2013.01.009
- Tanti A, Westphal WP, Girault V, Brizard B, Devers S, Leguisquet AM, Surget A, Belzung C. Region-dependent and stage-specific effects of stress, environmental enrichment, and antidepressant treatment on hippocampal neurogenesis. Hippocampus 2013; 23:797-811; PMID:23592526; http://dx.doi.org/10.1002/hipo.22134
- Vitullo P, Sciamanna I, Baiocchi M, Sinibaldi-Vallebona P, Spadafora C. LINE-1 retrotransposon copies are amplified during murine early embryo development. Mol Reprod Dev 2012; 79:118-27; PMID:22139884; http://dx.doi.org/10.1002/mrd.22003
- Boyce-Rustay JM, Holmes A. Genetic inactivation of the NMDA receptor NR2A subunit has anxiolytic- and antidepressant-like effects in mice. Neuropsychopharmacology 2006; 31:2405-14; PMID:16482087