Abstract
Elaborate control of gene expression by transcription factors is common to all kingdoms of life. In eukaryotes, transcription factor abundance and activity are often regulated by targeted proteolysis via the ubiquitin-proteasome system (UPS). The yeast MATα2 (α2) cell type regulator has long served as a model for UPS-dependent transcription factor degradation. Proteolysis of α2 is complex: it involves at least 2 ubiquitylation pathways and multiple regions of α2 affect its degradation. Such complexity also exists for the degradation of other UPS substrates. Here I review α2 degradation, most notably our recent identification of 2 novel degradation elements within α2 that overlap corepressor binding sites. I discuss possible implications of these findings and consider how principles of α2 proteolysis may be relevant to the degradation of other UPS substrates.
Cells use multiple mechanisms to control the levels of the thousands of proteins encoded by their genes. The first step of gene expression, transcription, is often the primary mechanism for controlling the levels of a specific protein.Citation1 Protein levels are also frequently regulated by targeted proteolysis, which in eukaryotes is mainly accomplished by the ubiquitin-proteasome system (UPS).Citation1,2 In this system, the small protein ubiquitin is covalently attached to proteins (in a process termed ubiquitylation) destined for degradation by a chambered protease known as the proteasome. Most substrates of the proteasome are modified with multiple ubiquitin molecules, usually in the form of a polyubiquitin chain. Protein ubiquitylation requires 3 enzymes: an ubiquitin-activating enzyme (E1), an ubiquitin-conjugating (Ubc) enzyme (E2), and an ubiquitin ligase (E3), that operate sequentially. Each eukaryotic genome codes for multiple E2s and many E3s, allowing a diverse range of substrates to be ubiquitylated. E3s recognize the substrate to be ubiquitylated, and are the main source of specificity in the UPS.
Among the most common substrates of the UPS are sequence-specific DNA-binding transcriptional activators and repressors—collectively called transcription factors.Citation1 Targeted proteolysis of transcription factors allows rapid changes to gene expression programs, which is important during development and for cellular adaptation to diverse environments. The first example of UPS-mediated transcription factor proteolysis was reported for the yeast cell-type regulator MATα2 (α2).Citation3,4 Subsequent studies of α2 degradation have revealed important principles of protein degradation, some general to the UPS and others specific to the ubiquitylation pathways that target α2.Citation5,6
Multiple degradation pathways for the yeast MATα2 protein
The yeast Saccharomyces cerevisiae can exist as 3 cell types: a non-mating diploid and 2 haploid types (a and α) that can mate to form the diploid. Most wild yeast strains are capable of rapid switching between haploid types.Citation7 Because α2 represses expression of genes for the a mating type (called a-specific genes), its rapid turnover is required for timely mating type switching from α to a cells.Citation8 Efficient proteolysis of α2 involves at least 2 distinct pathways: the Doa10 pathway and the Ubc4 pathway, named for UPS components involved ().Citation9-11 Perturbation of a single pathway results in only modest stabilization of α2, whereas cells lacking both pathways show robust (though not complete) stabilization of α2 and a concomitant block to switching from the α to a cell type.Citation8,9 Many other UPS substrates, including the transcription factors p53 and Myc in mammals, are known to be targeted for degradation by multiple pathways.Citation1,5 Possible reasons and implications for redundancy in UPS-dependent proteolysis have been discussed previously.Citation5
Figure 1. (A) Pathways to MATα2 degradation. Deg1 and Deg2 denote degrons in α2. (B) Degrons, degradation elements, and corepressor binding sites within MATα2. Numbers for each degradation element denote amino acid residues. See text for further details.
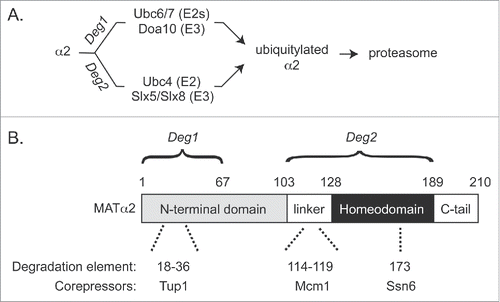
The Doa10 pathway of α2 degradation involves 2 E2 enzymes (Ubc6 and Ubc7) and the E3 Doa10, all of which associate tightly with the endoplasmic reticulum (ER)/nuclear envelope (NE).Citation10,12 As expected for a transcription factor, α2 is primarily localized to the nucleus, and Doa10 localized to the inner nuclear membrane can access nuclear proteinsCitation13,14. The Doa10 pathway received the most attention in early studies of α2 degradation, largely due to the discovery of an N-terminal fragment of α2—called degradation signal 1, or Deg1 (discussed in more detail below)—that allowed identification of Doa10 pathway components ().
The other pathway of α2 degradation, referred to here as the Ubc4 pathway, operates with the E2 Ubc4 and the heterodimeric E3 Slx5/Slx8 (Slx5/8).Citation9,11 However, since cells lacking Slx5/8 do not show stabilization of α2 to the extent observed with lack of Ubc4, this pathway is often named for the E2. An interesting feature of Slx5/8 is its affinity for the small ubiquitin-like modifier (SUMO) protein and its role in ubiquitylating SUMO-modified cellular proteins.Citation15 Because of these properties, Slx5/8 and its functional homologs (including the human proteins RNF4 and RNF111) have been referred to as SUMO-targeted ubiquitin ligases (STUbLs). In addition to targeting transcription factors, STUbLs have conserved roles in genome maintenance and exhibit interesting connections to other components of the SUMO system.Citation16 Surprisingly, despite these known properties, Slx5/8 targets α2 in a SUMO-independent manner.Citation11 In fact, Slx5/8-dependent ubiquitylation of α2 has been reconstituted in vitro with purified proteins that do not include SUMO.Citation6,11
Two other transcription factors—MATa1 and MATα1—regulate yeast mating and are also short-lived substrates of the UPS. MATα1 (α1) functions as a transcriptional activator of α-specific genes in α-type haploid cells and, like α2, is degraded via multiple pathways.Citation17 One of the several E3s that target α1 for degradation is Slx5/8; thus, α2 and α1 degradation may be coordinated via this pathway. MATa1 (a1) is expressed in a-type haploid cells, where it is very short-lived and has no apparent function. However, it functions in a heterodimeric a1/α2 complex to repress haploid-specific genes in diploid cells. Interestingly, in diploid cells a1 and α2 protect each other from proteolysis by direct interaction.Citation18.
Degrons and degradation elements within substrates of the UPS
Studies of UPS substrates have identified regions of these proteins important for their proteolysis.Citation19 I will refer to any region of a protein that contributes to its degradation as a degradation element. These regions have also been called degradation signals—abbreviated as “degrons.” The term degron is defined in different ways in the literature. Here I define a degron as a region of a protein that is both necessary and sufficient to target a protein for proteolysis.Citation19 As such, not all degradation elements—which can theoretically be a single amino acid—meet this definition of a degron, and degrons can often be further characterized to identify degradation elements within them. As defined here, degrons are transferable. That is, the attachment of a degron (usually by genetic engineering) will confer metabolic instability to an otherwise metabolically stable protein.
A degron within a substrate of the UPS requires at least 3 properties, which can be attributed to 3 distinct degradation elements.Citation19,20 The primary function of a UPS degron is to target the protein to a specific ubiquitylation machinery. Thus, degrons have an element or elements that are directly recognized by an E3 (or in some cases by an E3 accessory protein). Degron function also requires an amino acid acceptor (usually lysine) for ubiquitin attachment. Finally, a UPS degron must also contain an unstructured region that will initiate entry into the proteasome. Because UPS-dependent degradation is often spatially controlled, regions of a protein that affect its localization might also satisfy the broadest definition of a degradation element.Citation21
Given the number of proteins that are known or expected substrates of the UPS and the many E3s that recognize UPS substrates—yeast express over 50 E3s and humans have as many as 500—it is not surprising that a diversity of degradation elements exist for UPS targeting.Citation19 Elements recognized by an E3 are either part of the primary sequence of the protein, a post-translational modification (e.g., phosphorylation) to the protein, or a combination of these two.Citation19,20
Multiple degrons and degradation elements within α2
The first natural degron to be identified, Deg1 from α2, is targeted by a single pathway—the Doa10 pathway ().Citation3,10 The essentially complete stabilization observed for Deg1-based proteins in mutants of the Doa10 pathway contrasts with the relatively modest stabilization seen for full-length α2 in such mutants. The ability to isolate the Doa10 pathway of α2 proteolysis via Deg1 was instrumental to multiple studies.Citation12 Mutagenesis of Deg1 identified a hydrophobic face of an amphipathic helix as the most important element for Deg1 proteolysis.Citation18,22 Studies of other Doa10 substrates revealed similar degradation elements.Citation23,24 Given that many long-lived cellular proteins contain amphipathic helices and other ubiquitylation pathways recognize exposed hydrophobicity,Citation25,26 it is predicted that properties of substrates beyond these amphipathic helices are important for targeting by the Doa10 pathway. For Deg1, the aforementioned amphipathic helix (aa 18–36) is not sufficient to drive degradationCitation3 and residues closer to the N-terminus of Deg1 have been implicated in its stability.Citation11,22 It remains unclear whether Doa10 can directly recognize Deg1, and there is evidence for the involvement of chaperone proteins in Doa10-mediated ubiquitylation and degradation.Citation24,27,28 However, how these chaperones function with Doa10 in substrate recognition can only be speculated on at this time (see below). Thus, molecular roles for the degradation elements within the Deg1 region of α2 remain elusive.
Our recent study identified and characterized a second degron within α2—Deg2 ().Citation6 Deg2 is non-overlapping with Deg1 and contains 2 distinct degradation elements. One of the newly characterized degradation elements in Deg2 is part of the Slx5/8 recognition surface within the homeodomain (). The role of the other degradation element in Deg2, a hydrophobic segment, remains unknown. However, our data suggest that this element affects α2 degradation at or before the ubiquitylation step, rather than downstream of ubiquitylation. One possibility is that the linker element is recognized by an E3 other than Slx5/8. Loss of Slx5 or Slx8 does not stabilize α2 to the extent observed in the absence of the E2 Ubc4, suggesting that another Ubc4-dependent E3 might target α2.Citation11 Consistent with these findings, a Deg2 fusion protein is more stable in cells lacking Ubc4 than in cells lacking Slx5.Citation6 Furthermore, the Deg2 fusion is not fully stable in cells lacking Ubc4, suggesting that Deg2 can be targeted by E2s beyond Ubc4.Citation6 It is unclear whether such pathways might also act on full-length α2, but it is noteworthy that α2 is still (slowly) turned over in cells lacking both known ubiquitylation pathways (e.g., in ubc4Δ ubc6Δ cells).Citation9 Thus, it is also possible that an E3 that operates with E2s other than Ubc4, Ubc6, or Ubc7 can ubiquitylate α2. Another possibility is that α2 is targeted by just one E3 other than Slx5/8 and Doa10, but this E3 can operate with Ubc4 and additional E2s besides Ubc6 and Ubc7.
Figure 2. MATα2 degradation is linked to its functional status. (A) Schematic for the structural organization of α2. (B) Schematic showing α2 bound by its cofactors at its target DNA. Cofactor binding to α2 blocks its UPS-mediated degradation. Circles labeled “T” represent Tup1. For simplicity, this schematic shows only one subunit of the α2 dimer bound by cofactors. The exact organization and stoichiometry of the complex is unknown. (C) Schematic depicting α2 recognition by 2 distinct ubiquitylation pathways. INM = inner nuclear membrane. Hypothetical factors and steps in α2 recognition are marked with a (?) symbol. See text for further details.
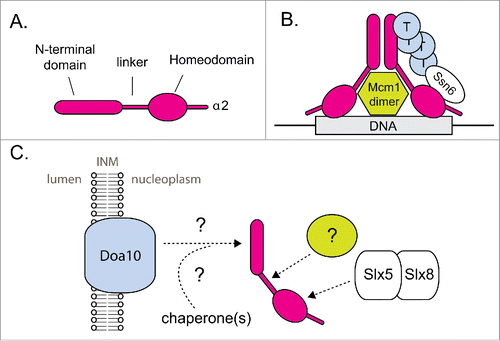
A distinct hypothesis for the mechanistic role of the degradation element within the α2 linker is that it binds a cofactor for Slx5/8 (and potentially any unidentified E3(s) involved in α2 turnover). I favor this hypothesis since Deg2 is fully stabilized by mutation of the linker element,Citation6 suggesting that any E3 that can target Deg2 requires the linker. Since the linker is not required for Slx5/8-dependent ubiquitylation of α2 in our current in vitro assay, I hypothesize that the in vitro reaction would be much more efficient if the hypothetical Slx5/8 cofactor were present. Based on the hydrophobicity of the linker element, I have proposed that the linker binds a chaperone protein that functions with Slx5/8 (see below).Citation6
Other transcription factors with multiple degrons
Like α2, other transcription factors are known or hypothesized to have multiple degrons.Citation1,17 MATα1 contains at least 2 degrons, one that is targeted by Slx5/8 and one that is targeted by a different ligase or ligases.Citation17 The Rpn4 transcriptional activator also has at least 2 degrons.Citation29 Interestingly, one of the degrons in Rpn4 is degraded by the proteasome in an ubiquitin-independent manner, whereas the other degron is clearly ubiquitin-dependent. A few other proteins, all of which have a region of notable intrinsic disorder, are thought to be degraded by the proteasome in an ubiquitin-independent manner.Citation29 As previously mentioned, the need for an unstructured region, usually at protein termini, has been suggested for all proteasome substrates—even those targeted to the proteasome by ubiquitin.Citation2 Thus, studies that employ mutagenesis of short-lived proteins should consider potential effects on proteasomal degradation in addition to effects on ubiquitylation.
The role of corepressors in α2 degradation
The two Ubc4 pathway degradation elements recently identified within α2 overlap binding sites for the α2 corepressors Mcm1 and Ssn6 ().Citation6 The hydrophobic residues within the linker element make intimate contact with Mcm1, as observed in a crystal structure of a ternary complex of α2, Mcm1, and the target operator DNA.Citation30 While these hydrophobic residues are part of a β-strand when bound to Mcm1, it remains unclear how they are structured when not bound to Mcm1. One possibility is that these residues bind another protein—perhaps a chaperone—in the absence of Mcm1. In such a situation, the structure of the linker region may not resemble that observed in the crystal structure of the ternary complex. We recently showed that α2 is still rapidly degraded in the absence of Mcm1.Citation6 In fact, α2 degradation is slightly faster in mcm1Δ yeast, which I hypothesize is due to enhanced exposure of the degradation element in the α2 linker.
A precedent for corepressors blocking the exposure of degradation elements was provided in a previous study of α2 degradation.Citation31 A screen for proteins that when overexpressed stabilize Deg1 fusions yielded the Tup1 protein. Tup1 overexpression also modestly stabilized full-length α2. Furthermore, co-overexpression of both Tup1 and Ssn6 led to robust stabilization of α2. Tup1 and Ssn6 form a corepressor complex that functions with multiple sequence-specific transcription factors.Citation32 Tup1 interacts with the N-terminus of α2, consistent with its ability to block access to Deg1 (). Ssn6 interacts with the homeodomain of α2—in a region recognized by Slx5/8.Citation6,33 Thus, we have proposed a model in which Ssn6 binds to the α2 homeodomain and blocks access by Slx5/8.Citation6 Evidence for a direct competition between Ssn6 and Slx5/8 is lacking, but experiments using the aforementioned in vitro assay with a purified functional fragment of Ssn6 could be attempted in the future.
Developing paradigms for transcription factor degradation
It would be interesting to determine whether the degradation of other transcriptional repressors is affected by their interaction with corepressors, particularly the general corepressors Tup1 and Ssn6. Other short-lived proteins that function with Tup1 and Ssn6 include the repressors Mig1 and Cup9.Citation34,35 Cup9 degradation is mediated by the Ubr1 ubiqutin ligase, and its role in peptide import has been studied extensivelyCitation35-39. Though Ubr1 is best known for its role in degradation of N-end rule substrates, Ubr1 targeting of Cup9 does not depend on the N-terminus of Cup9 but rather a region near its C-terminus.Citation36 How Tup1 and Ssn6 interact with Cup9 remains to be determined.
For both Cup9 and α2, their enhanced stability in UPS mutants leads to repression in circumstances when transcriptional repression should be relieved.Citation8,35 Thus, UPS-mediated turnover of these repressors acts to limit their abundance, allowing rapid changes to the transcriptional programs that they control. This relatively straightforward role for UPS-mediated proteolysis contrasts with a situation observed for many transcriptional activators. For these activators, their UPS-mediated proteolysis actually promotes the transcriptional program under their positive control.Citation1,40 While this counterintuitive phenomenon is not fully understood (as discussed extensively by Geng et al.),Citation1 it is clear that for many transcriptional activators, their degrons closely or completely overlap their transcriptional activation domains.Citation1 In a model that involves phosphorylation of activators, Geng et al. propose that UPS-mediated proteolysis clears spent (no longer active) activators from chromatin, allowing a fresh activator to take its place. Geng et al. also note that a separate mode of activator degradation, when they are not bound to their specific gene sites, plays the more straightforward role of limiting the abundance of the transcription factor. Although UPS-mediated proteolysis has not been observed to enhance repression, this mechanism could theoretically exist for repressors. Nevertheless, the fact that corepressors affect the rate of α2 degradation suggests that cells can link UPS-mediated proteolysis to the functional status of a transcription factor whether it be a repressor or activator.
Hydrophobicity and molecular chaperones in α2 degradation
In addition to corepressors, other proteins that bind α2 may impact its degradation. Given its 2 regions of exposed hydrophobicity—the amphipathic helix in Deg1 and the consecutive hydrophobic amino acids in the linker, both of which are degradation elements—one might expect that α2 would bind chaperone proteins (at least temporarily). Chaperones of the Hsp70 family and their co-chaperones of the Hsp40 family are known to prevent the aggregation of proteins with exposed hydrophobicity—the main characteristic of misfolded proteins.Citation26 These same chaperone families play important roles in several ubiquitylation pathways, as reviewed by Shiber and Ravid.Citation26
For α2 degradation, perhaps the most interesting question is whether chaperones are involved in substrate recognition by Doa10 and/or Slx5/8 (). While potential roles for chaperones in the degradation of full-length α2 have not been investigated, one study provided evidence for the involvement of the Hsp40 chaperone Sis1 in the degradation of a membrane-embedded Deg1-fusion.Citation28 There is additional evidence for Hsp70/Hsp40 chaperone involvement in the degradation of other Doa10 substrates.Citation12,24,27,28,41 As previously mentioned, direct interaction between Doa10 and Deg1 (or α2) has never been demonstrated. Presumably this interaction is transient and ubiquitylation need not be processive—that is, Doa10 might release its substrates between ubiquitin additions. However, there may be mechanisms to keep Doa10 substrates close to the Doa10 machinery on the ER/NE and such mechanisms may be lost after cells are lysed and/or membranes are solubilized in detergent. For proteins bearing the artificial Doa10-dependent degron CL1, the chaperones Ydj1 (Hsp40) and Ssa1 (Hsp70) are proposed to be part of a network of proteins that create a platform for ubiquitylation and degradation on the cytosolic face of the ER membrane.Citation12,27 A subpopulation of Ydj1 is known to acquire a lipidic tail that anchors it in the ER/NE membrane. Another Hsp40 protein, Hlj1, is integral to the ER membrane and has also been implicated in degradation of proteins at the ER, including Doa10 substrates.Citation41,42
For the Ubc4 pathway of α2 degradation, one hypothesis is that a chaperone protein might bind both the α2 linker and Slx5/8 to accelerate the Slx5/8-mediated ubiquitylation of α2 (). This idea can theoretically be tested using the in vitro assay described earlier, with either cell extracts as a potential source of the appropriate chaperone(s) or with purified candidate chaperones. While the ability of chaperones to modulate Slx5/8 activity has never been demonstrated biochemically, Slx5/8 has been genetically linked to one chaperone, the Hsp40-family chaperone Apj1.Citation43
Theoretically, a chaperone that operates with both Doa10 and Slx5/8 could have a large affect on α2 degradation. However, given that yeast—like all eukaryotes—express many chaperones that are functionally redundant,Citation26,43 it is unlikely that a chaperone protein encoded by a single gene would be uniquely responsible for such a function. For this and other reasons, biochemical studies of chaperone function in α2 degradation may be more enlightening than genetic approaches.
Concluding remarks
In-depth analyses of cellular processes using tractable model systems have continually produced discoveries fundamental to our understanding of life that are often relevant to human health and disease. The use of yeast to study proteolysis by the UPS is one of the many great examples of the power of basic biomedical science. The α2 repressor was the first endogenous substrate of the UPS to be characterized and continues to be an important model UPS substrate.
Many questions remain about the UPS and, in particular, transcription factor degradation. Are there cases in which transcriptional repressor degradation enhances repression, similar to what has been observed for activators? Do transcriptional corepressors affect repressor degradation in cases other than α2? With regard to chaperones, what is their exact role in α2 degradation and are these roles more general to the UPS?
A long-term goal of the UPS field is the ability to predict whether a protein is short-lived, and if so, which pathway(s) target it for proteolysis. This is no trivial task. Cells express many E3s—the bulk of which remain uncharacterized—and each E3 has, or is expected to have, multiple substrates. In addition, whether a protein is degraded can be influenced by its subcellular localization, its interaction partners, post-translational modifications to the protein (besides ubiquitin), and the chemical properties of the intracellular environment. Future studies of transcription factor proteolysis are expected to provide further insight into these issues.
Disclosure of potential conflicts of interest
No potential conflicts of interest were disclosed.
Acknowledgments
I thank Mark Hochstrasser (Yale University) for continued support and feedback on the manuscript. I also thank Jason Berk (Yale University) for critical reading of the manuscript.
Funding
My work is supported by NIH grants GM046904 and GM053756 (to M.H.) and previously by a Ruth L. Kirschstein National Research Service Award (F32GM097794).
References
- Geng F, Wenzel S, Tansey WP. Ubiquitin and proteasomes in transcription. Annu Rev Biochem 2012; 81:177-201; PMID:22404630; http://dx.doi.org/10.1146/annurev-biochem-052110-120012
- Inobe T, Matouschek A. Paradigms of protein degradation by the proteasome. Curr Opin Struct Biol 2014; 24:156-64; PMID:24632559; http://dx.doi.org/10.1016/j.sbi.2014.02.002
- Hochstrasser M, Varshavsky A. In vivo degradation of a transcriptional regulator: the yeast alpha 2 repressor. Cell 1990; 61:697-708; PMID:2111732; http://dx.doi.org/10.1016/0092-8674(90)90481-S
- Hochstrasser M, Ellison MJ, Chau V, Varshavsky A. The short-lived MAT alpha 2 transcriptional regulator is ubiquitinated in vivo. Proc Natl Acad Sci U S A 1991; 88:4606-10; PMID:1647011; http://dx.doi.org/10.1073/pnas.88.11.4606
- Rubenstein EM, Hochstrasser M. Redundancy and variation in the ubiquitin-mediated proteolytic targeting of a transcription factor. Cell Cycle 2010; 9:4282-5; PMID:20980825; http://dx.doi.org/10.4161/cc.9.21.13741
- Hickey CM, Hochstrasser M. STUbL-mediated degradation of the transcription factor MATalpha2 requires degradation elements that coincide with corepressor binding sites. Mol Biol Cell 2015; 26:3401-12; PMID:26246605; http://dx.doi.org/10.1091/mbc.E15-06-0436
- Haber JE. Mating-type genes and MAT switching in Saccharomyces cerevisiae. Genetics 2012; 191:33-64; PMID:22555442; http://dx.doi.org/10.1534/genetics.111.134577
- Laney JD, Hochstrasser M. Ubiquitin-dependent degradation of the yeast Mat(alpha)2 repressor enables a switch in developmental state. Genes Dev 2003; 17:2259-70; PMID:12952895; http://dx.doi.org/10.1101/gad.1115703
- Chen P, Johnson P, Sommer T, Jentsch S, Hochstrasser M. Multiple ubiquitin-conjugating enzymes participate in the in vivo degradation of the yeast MAT alpha 2 repressor. Cell 1993; 74:357-69; PMID:8393731; http://dx.doi.org/10.1016/0092-8674(93)90426-Q
- Swanson R, Locher M, Hochstrasser M. A conserved ubiquitin ligase of the nuclear envelope/endoplasmic reticulum that functions in both ER-associated and Matalpha2 repressor degradation. Genes Dev 2001; 15:2660-74; PMID:11641273; http://dx.doi.org/10.1101/gad.933301
- Xie Y, Rubenstein EM, Matt T, Hochstrasser M. SUMO-independent in vivo activity of a SUMO-targeted ubiquitin ligase toward a short-lived transcription factor. Genes Dev 2010; 24:893-903; PMID:20388728; http://dx.doi.org/10.1101/gad.1906510
- Zattas D, Hochstrasser M. Ubiquitin-dependent protein degradation at the yeast endoplasmic reticulum and nuclear envelope. Crit Rev Biochem Mol Biol 2015; 50:1-17; PMID:25231236; http://dx.doi.org/10.3109/10409238.2014.959889
- Hall MN, Craik C, Hiraoka Y. Homeodomain of yeast repressor alpha 2 contains a nuclear localization signal. Proc Natl Acad Sci U S A 1990; 87:6954-8; PMID:1976249; http://dx.doi.org/10.1073/pnas.87.18.6954
- Deng M, Hochstrasser M. Spatially regulated ubiquitin ligation by an ER/nuclear membrane ligase. Nature 2006; 443:827-31; PMID:17051211; http://dx.doi.org/10.1038/nature05170
- Sriramachandran AM, Dohmen RJ. SUMO-targeted ubiquitin ligases. Biochim Biophys Acta 2014; 1843:75-85; PMID:24018209; http://dx.doi.org/10.1016/j.bbamcr.2013.08.022
- Hickey CM, Wilson NR, Hochstrasser M. Function and regulation of SUMO proteases. Nat Rev Mol Cell Biol 2012; 13:755-66; PMID:23175280; http://dx.doi.org/10.1038/nrm3478
- Nixon CE, Wilcox AJ, Laney JD. Degradation of the Saccharomyces cerevisiae mating-type regulator alpha1: genetic dissection of cis-determinants and trans-acting pathways. Genetics 2010; 185:497-511; PMID:20351217; http://dx.doi.org/10.1534/genetics.110.115907
- Johnson PR, Swanson R, Rakhilina L, Hochstrasser M. Degradation signal masking by heterodimerization of MATalpha2 and MATa1 blocks their mutual destruction by the ubiquitin-proteasome pathway. Cell 1998; 94:217-27; PMID:9695950; http://dx.doi.org/10.1016/S0092-8674(00)81421-X
- Ravid T, Hochstrasser M. Diversity of degradation signals in the ubiquitin-proteasome system. Nat Rev Mol Cell Biol 2008; 9:679-90; PMID:18698327; http://dx.doi.org/10.1038/nrm2468
- Guharoy M, Bhowmick P, Sallam M, Tompa P. Tripartite degrons confer diversity and specificity on regulated protein degradation in the ubiquitin-proteasome system. Nat Commun 2016; 7:10239; PMID:26732515; http://dx.doi.org/10.1038/ncomms10239
- Grabbe C, Husnjak K, Dikic I. The spatial and temporal organization of ubiquitin networks. Nat Rev Mol Cell Biol 2011; 12:295-307; PMID:21448225; http://dx.doi.org/10.1038/nrm3099
- Kim I, Miller CR, Young DL, Fields S. High-throughput analysis of in vivo protein stability. Mol Cell Proteomics 2013; 12:3370-8; PMID:23897579; http://dx.doi.org/10.1074/mcp.O113.031708
- Gilon T, Chomsky O, Kulka RG. Degradation signals for ubiquitin system proteolysis in Saccharomyces cerevisiae. EMBO J 1998; 17:2759-66; PMID:9582269; http://dx.doi.org/10.1093/emboj/17.10.2759
- Furth N, Gertman O, Shiber A, Alfassy OS, Cohen I, Rosenberg MM, Doron NK, Friedler A, Ravid T. Exposure of bipartite hydrophobic signal triggers nuclear quality control of Ndc10 at the endoplasmic reticulum/nuclear envelope. Mol Biol Cell 2011; 22:4726-39; PMID:21998200; http://dx.doi.org/10.1091/mbc.E11-05-0463
- Fredrickson EK, Rosenbaum JC, Locke MN, Milac TI, Gardner RG. Exposed hydrophobicity is a key determinant of nuclear quality control degradation. Mol Biol Cell 2011; 22:2384-95; PMID:21551067; http://dx.doi.org/10.1091/mbc.E11-03-0256
- Shiber A, Ravid T. Chaperoning proteins for destruction: diverse roles of Hsp70 chaperones and their co-chaperones in targeting misfolded proteins to the proteasome. Biomolecules 2014; 4:704-24; PMID:25036888; http://dx.doi.org/10.3390/biom4030704
- Metzger MB, Maurer MJ, Dancy BM, Michaelis S. Degradation of a cytosolic protein requires endoplasmic reticulum-associated degradation machinery. J Biol Chem 2008; 283:32302-16; PMID:18812321; http://dx.doi.org/10.1074/jbc.M806424200
- Shiber A, Breuer W, Brandeis M, Ravid T. Ubiquitin conjugation triggers misfolded protein sequestration into quality control foci when Hsp70 chaperone levels are limiting. Mol Biol Cell 2013; 24:2076-87; PMID:23637465; http://dx.doi.org/10.1091/mbc.E13-01-0010
- Erales J, Coffino P. Ubiquitin-independent proteasomal degradation. Biochim Biophys Acta 2014; 1843:216-21; PMID:23684952; http://dx.doi.org/10.1016/j.bbamcr.2013.05.008
- Tan S, Richmond TJ. Crystal structure of the yeast MATalpha2/MCM1/DNA ternary complex. Nature 1998; 391:660-6; PMID:9490409; http://dx.doi.org/10.1038/35563
- Laney JD, Mobley EF, Hochstrasser M. The short-lived Matalpha2 transcriptional repressor is protected from degradation in vivo by interactions with its corepressors Tup1 and Ssn6. Mol Cell Biol 2006; 26:371-80; PMID:16354707; http://dx.doi.org/10.1128/MCB.26.1.371-380.2006
- Malave TM, Dent SY. Transcriptional repression by Tup1-Ssn6. Biochem Cell Biol 2006; 84:437-43; PMID:16936817; http://dx.doi.org/10.1139/o06-073
- Smith RL, Johnson AD. A sequence resembling a peroxisomal targeting sequence directs the interaction between the tetratricopeptide repeats of Ssn6 and the homeodomain of alpha 2. Proc Natl Acad Sci U S A 2000; 97:3901-6; PMID:10759558; http://dx.doi.org/10.1073/pnas.070506797
- Lim MK, Siew WL, Zhao J, Tay YC, Ang E, Lehming N. Galactose induction of the GAL1 gene requires conditional degradation of the Mig2 repressor. Biochem J 2011; 435:641-9; PMID:21323640; http://dx.doi.org/10.1042/BJ20102034
- Xia Z, Turner GC, Hwang CS, Byrd C, Varshavsky A. Amino acids induce peptide uptake via accelerated degradation of CUP9, the transcriptional repressor of the PTR2 peptide transporter. J Biol Chem 2008; 283:28958-68; PMID:18708352; http://dx.doi.org/10.1074/jbc.M803980200
- Xia Z, Webster A, Du F, Piatkov K, Ghislain M, Varshavsky A. Substrate-binding sites of UBR1, the ubiquitin ligase of the N-end rule pathway. J Biol Chem 2008; 283:24011-28; PMID:18566452; http://dx.doi.org/10.1074/jbc.M802583200
- Byrd C, Turner GC, Varshavsky A. The N-end rule pathway controls the import of peptides through degradation of a transcriptional repressor. EMBO J 1998; 17:269-77; PMID:9427760; http://dx.doi.org/10.1093/emboj/17.1.269
- Turner GC, Du F, Varshavsky A. Peptides accelerate their uptake by activating a ubiquitin-dependent proteolytic pathway. Nature 2000; 405:579-83; PMID:10850718; http://dx.doi.org/10.1038/35014629
- Du F, Navarro-Garcia F, Xia Z, Tasaki T, Varshavsky A. Pairs of dipeptides synergistically activate the binding of substrate by ubiquitin ligase through dissociation of its autoinhibitory domain. Proc Natl Acad Sci U S A 2002; 99:14110-5; PMID:12391316; http://dx.doi.org/10.1073/pnas.172527399
- Lipford JR, Smith GT, Chi Y, Deshaies RJ. A putative stimulatory role for activator turnover in gene expression. Nature 2005; 438:113-6; PMID:16267558; http://dx.doi.org/10.1038/nature04098
- Huyer G, Piluek WF, Fansler Z, Kreft SG, Hochstrasser M, Brodsky JL, Michaelis S. Distinct machinery is required in Saccharomyces cerevisiae for the endoplasmic reticulum-associated degradation of a multispanning membrane protein and a soluble luminal protein. J Biol Chem 2004; 279:38369-78; PMID:15252059; http://dx.doi.org/10.1074/jbc.M402468200
- Youker RT, Walsh P, Beilharz T, Lithgow T, Brodsky JL. Distinct roles for the Hsp40 and Hsp90 molecular chaperones during cystic fibrosis transmembrane conductance regulator degradation in yeast. Mol Biol Cell 2004; 15:4787-97; PMID:15342786; http://dx.doi.org/10.1091/mbc.E04-07-0584
- Sahi C, Kominek J, Ziegelhoffer T, Yu HY, Baranowski M, Marszalek J, Craig EA. Sequential duplications of an ancient member of the DnaJ-family expanded the functional chaperone network in the eukaryotic cytosol. Mol Biol Evol 2013; 30:985-98; PMID:23329686; http://dx.doi.org/10.1093/molbev/mst008