ABSTRACT
The ability of cells to direct their movement and growth in response to shallow chemical gradients is essential in the life cycles of all eukaryotic organisms. The signaling mechanisms underlying directional sensing in chemotactic cells have been well studied; however, relatively little is known about how chemotropic cells interpret chemical gradients. Recent studies of chemotropism in budding and fission yeast have revealed 2 quite different mechanisms—biased wandering of the polarity complex, and differential internalization of the receptor and G protein. Each of these mechanisms has been proposed to play a key role in decoding mating pheromone gradients. Here we explore how they may work together as 2 essential components of one gradient sensing machine.
Cells polarize their structures and functions in response to a variety of directional stimuli, including gravity,Citation1 light,Citation2 electrical gradients,Citation3 and chemical gradients.Citation4,5 Cellular responses to chemical gradients have attracted the most attention, as they are essential to the life cycles of all eukaryotic species. The best-known gradient-stimulated cellular outputs, chemotaxis (directed cell movement), and chemotropism (directed cell growth), are required for a wide range of biologic processes. For example, chemotaxis plays a vital role in development, immunity, wound healing, inflammation, and metastasis; chemotropism is integral to axon guidance,Citation6,7 angiogenesis,Citation8-10 pollen tube guidance,Citation11,12 and fungal life cycles.Citation13,14
Although they ultimately exhibit quite different behavior, chemotactic and chemotropic cells face similar challenges. In both cases, the responding cell must sense small differences in chemical concentration across its surface, determine the direction of the gradient source, and then polarize its cytoskeleton toward or away from it. Whereas a great deal has been learned about the mechanisms underlying directional sensing in chemotactic models,Citation15,16 relatively little is known about how chemotropic cells interpret chemical gradients. To date, the best-characterized chemotropic model is the mating response of the budding yeast, Saccharomyces cerevisiae.
Haploid yeast exist as 2 mating types, MATa and MATα, each of which secretes a peptide pheromone that binds to a G protein-coupled receptor (GPCR) on cells of the opposite type. Ligand-bound receptor induces the activation of Gα and consequent dissociation of the heterotrimeric G protein into its Gα and Gβγ subunits. Free Gβγ then activates a MAPK cascade that triggers cell-cycle arrest in G1 and changes in gene expression. Once arrested in G1, pheromone-stimulated cells also form mating projections via actin-cable-directed delivery of membrane vesicles to a focused growth site (for reviews, see referencesCitation17,18). Local activation of the conserved Rho-family GTPase Cdc42 by its guanine nucleotide exchange factor Cdc24 determines the position of actin-cable nucleation, and thus the direction of mating projection growth.Citation19 Cdc24 and Cdc42 are members of a group of interacting proteins collectively known as the polarity complex.
When pheromone-stimulated cells are unable to sense a gradient (e.g., under isotropic or saturating dose conditions), they form mating projections where they would have budded in the next cell cycle.Citation20 This position, marked by Bud1, is known as the default polarity site.Citation21 In mating mixtures, however, proximal cells of opposite mating type orient their mating projections directly toward one another. The projections continue to elongate and narrow until they touch at their tips and fuse.Citation22 Although early observations of yeast mating were suggestive of chemotropism, the first clear demonstration that yeast can align their growth to a gradient was reported by Segall (1993),Citation23 who showed that mating projections grow toward a point source of synthetic pheromone, and that they reorient (track the gradient) when the point source is moved. SegallCitation23 also concluded that a 1% gradient across the diameter of the yeast cell is steep enough to elicit an accurate directional response. Genetic studies in which MATa cells were challenged to discriminate between pheromone-secreting and a large excess of non-secreting partners confirmed that yeast mating is gradient-driven and of remarkably high fidelity.Citation24,25 More recently, gradient-stimulation of yeast in microfluidic devices confirmed Segall's observations,Citation26,27 and time-lapse imaging of mating mixtures has provided definitive examples of yeast chemotropism in situ.Citation28
How do yeast cells accurately sense the direction of the pheromone source and polarize their growth toward it? The discovery that Gβγ interacts with Cdc24 via the Far1 scaffold proteinCitation29,30 suggested that Gβγ links activated receptors to the actin-nucleation machinery, and, in so doing, acts as a positional determinant. In principle, the peak concentration of activated G protein on the membrane should correspond to the peak concentration of activated receptor, and thereby promote the establishment of the chemotropic growth site where the pheromone concentration is highest. Although the communication of receptor status to the actin cytoskeleton via Gβγ-Far1-Cdc24 activation of Cdc42 was an important insight,Citation29 it failed to explain how the cell translates a vanishingly small differential of activated receptors across its surface—with almost as many receptors binding pheromone on the upgradient side as on the downgradient sideCitation23,26,27—into an accurate and robust directional response. Recent advances have revealed unprecedented mechanisms that contribute to this ability.
At the time that the Gβγ-Far1-Cdc24 interaction was discovered, and for many years thereafter, the best explanation for yeast gradient sensing was as follows: the slight excess of Gβγ on the upgradient side of the cell triggered signal amplification mechanisms that were rapidly reinforced by actin-cable-directed secretion, thereby establishing the chemotropic growth site. Although vague, this model did posit 2 key details: (1) Actin-cable-directed secretion is integral to the genesis of the chemotropic site; (2) In gradient-stimulated cells, the polarity proteins that nucleate actin cables are correctly oriented toward the pheromone source when first assembled as a functional complex. The first of these tenets was called into question when pheromone was shown to induce polarized distribution of the receptor in latrunculin A-treated cells and mutant cells unable to target vesicular delivery via actin cables.Citation31,32 The second tenet has been contradicted by 3 recent studies using reporters of the polarity complex in cells responding to pheromone gradients. Results indicated that the reporters do not initially assemble at the chemotropic site; rather, they wander along the cell cortex.Citation33,34,35
In live-cell imaging experiments, Dyer et al.Citation33 and Hegemann et al.Citation34 found that fluorescent reporters of polarity complex constituents at the cell cortex move up a sub-saturating gradient of pheromone. Movement of the polarity complex is biased toward the incipient mating projection site through the interaction of Far1-Bem1-Cdc24 with free Gβγ.Citation33,34 Dyer et al.Citation33 showed that movement of Bem1-GFP, a proxy for the polarity complex, is driven by actin-cable-delivered vesicles, and that although the polarized Bem1-GFP patch arises independently of Bud1 and Gβγ, its wandering is biased by interaction with Bud1 and Gβγ. Constraint of the polarity complex is pheromone dose-dependent—i.e., movement slows as pheromone concentration increases. Importantly, constraint of the polarity complex at the chemotropic site in artificial pheromone gradients depends on Gβγ. Hegemann et al.Citation34 made similar conclusions by following Cdc24, Cdc42-GTP, and Bni1 reporters. Moreover, Bendezu et al.Citation35 reported that a patch of polarity proteins explores the cortex during mating in S. pombe, and that its rate of movement is dependent on pheromone concentration. The picture that emerges from this work is that, in both budding and fission yeast, the polarity complex wanders, but its rate of movement is inversely proportional to the local concentration of pheromone. Thus, position of the polarity complex is biased up the pheromone gradient, and is ultimately stabilized by maximal pheromone signaling at the chemotropic growth site. In S. cerevisiae, it is clear that Gβγ plays the critical role of constraining the polarity complex at the right time and place to orient the cell's axis of polarity toward its mating partner.
This raises the question: how does Gβγ localize to the chemotropic growth site? As pointed out above, the very shallow gradient predicts free Gβγ all over the cell cortex, with only a very slightly greater amount on the upgradient side of the cell. McClure et al.Citation36 present evidence that, as the polarity complex is displaced by vesicle delivery, it leaves newly nucleated actin cables in its wake. These cables direct nascent receptor and G protein to the cortex just behind the moving polarity complex. McClure et al.Citation36 propose that the newly delivered receptor and G protein serve as a “nose” that senses the local pheromone concentration. As pheromone concentration increases, the density of activated receptors and G proteins increases, leading to greater restraint on the traveling complex. In this view, polarization of the receptor and G protein occurs via an “inside out” mechanism. In contrast, Hegemann et al.Citation34 refer to the more traditional view in which receptor and G protein polarity arise in response to the pheromone gradient, although they do not explain how the spatial signal is amplified inside the cell.
Activation of the yeast pheromone receptors induces phosphorylation of their C-terminal cytoplasmic tails by the Yck1 and Yck2 casein kinases, and this triggers receptor internalization. By analogy to mammalian GPCRs, pheromone-induced internalization of the yeast receptors has historically been considered a desensitization mechanism. In the most recent investigation of yeast directional sensing, however, Ismael et al.Citation28 described a novel mechanism by which the receptor and G protein polarize upstream and independently of actin-dependent directed secretion. The authors found that Gβγ interacts with the Yck1 and Yck2 casein kinases in pheromone-treated cells, resulting in local inhibition of Yck-dependent receptor phosphorylation. The protection of the receptor by its cognate G protein, along with the previously known co-internalization of the G protein with the phosphorylated receptor,Citation31 suggested a mechanism for amplifying the spatial signal: the slight gradient of activated receptor across the surface of the cell is expected to induce a corresponding gradient of activated G protein. This leads to differential receptor phosphorylation and receptor/G-protein internalization, with the consequent concentration of the signaling machinery on the upgradient side of the cell. A mathematical model demonstrated the feasibility of driving receptor and G protein polarization toward the pheromone source via this positive feedback mechanism. The effects of various mutant forms of the receptor on the ability of cells to orient toward mating partners in situ supported this hypothesis: Whereas blocking receptor internalization—and thus receptor polarization—conferred a moderate defect in gradient sensing, blocking receptor internalization and phosphorylation entirely eliminated this ability. Additionally, the receptor relocalizes from the default polarity site to the chemotropic growth site ahead of active Cdc42, consistent with its role as the primary sensor of the pheromone gradient.
Taken together, the observations discussed here suggest a unified model of yeast gradient sensing (). Polarity-complex wandering and receptor/G protein polarization at the incipient chemotropic site are not mutually exclusive mechanisms. Rather, they may play 2 distinct roles in a concerted process: While one mechanism propels the polarity complex to move randomly along the cell cortex, the other mechanism slows and stabilizes the complex in the region of highest pheromone concentration. Although this picture represents a breakthrough in our understanding of chemical gradient sensing, many important questions remain to be answered. What are the mechanistic differences, if any, underlying how cells initially orient toward a potential mating partner, and how they reorient in a dynamic gradient? How are receptor and G protein trafficking regulated before the onset of actin-dependent directed secretion? Do anterograde vesicles carrying receptor and G protein preferentially dock and/or fuse where their sister molecules are concentrated on the plasma membrane? Does the Sst2 RGS protein, which catalyzes inactivation of Gα, play a role in establishment of polarity or in gradient tracking? Are there key players in this process that have yet to be identified?
Figure 1. A unified model of yeast gradient sensing. (A) Gβγ constraint of polarity complex wandering. Actin-dependent vesicle docking drives random movement of the Bem1-Cdc24-Cdc42 polarity complex along the PM, but movement of the complex is biased up the pheromone gradient by its interaction with Gβγ. Activated receptor catalyzes the release of Gβγ, which inhibits receptor phosphorylation through its interaction with the receptor kinase, and thereby differentially protects both receptor and G protein from internalization at the incipient mating projection site. (B) Gradient-induced positioning of the chemotropic site. Gβγ inhibition of receptor and G protein internalization initiates a positive feedback loop that concentrates active-unphosphorylated receptor and G protein where the external pheromone concentration is highest. Thus, actin-independent polarization of Gβγ generates a positional cue that aligns the polarity complex with the pheromone gradient prior to cellular morphogenesis.
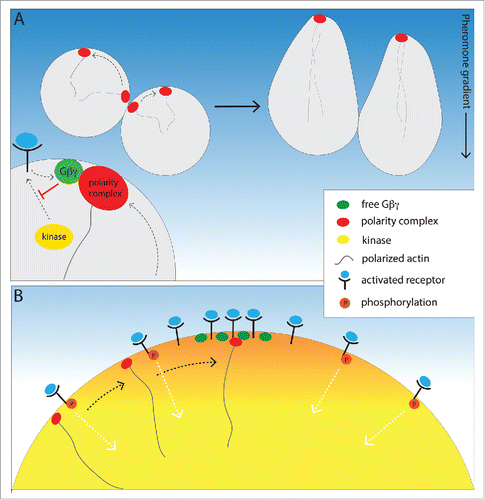
Disclosure of potential conflicts of interest
No potential conflicts of interest were disclosed.
Funding
This work was supported by NSF grant 1415589 (to D.E.S.).
References
- Morita MT. Directional gravity sensing in gravitropism. Annu Rev Plant Biol 2010; 61:705-20; PMID:19152486; http://dx.doi.org/10.1146/annurev.arplant.043008.092042
- Jékely G. Evolution of phototaxis. Philos Trans R Soc Lond B Biol Sci 2009; 364(1531):2795-808; PMID:19720645; http://dx.doi.org/10.1098/rstb.2009.0072
- Cortese B, Ilaria A, Palamà E, D ’amone S, Gigli G. Influence of electrotaxis on cell behaviour. Integr Biol 2014; 6(6):817-30; http://dx.doi.org/10.1039/C4IB00142G
- Cai H, Devreotes PN. Moving in the right direction: How eukaryotic cells migrate along chemical gradients. Semin Cell Dev Biol 2011; 22(8):834-41; PMID:21821139; http://dx.doi.org/10.1016/j.semcdb.2011.07.020
- Arkowitz RA. Chemical gradients and chemotropism in yeast. Cold Spring Harb Perspect Biol 2009; 1(2):1-20; http://dx.doi.org/10.1101/cshperspect.a001958
- Hong K, Nishiyama M. From guidance signals to movement: signaling molecules governing growth cone turning. Neuroscientist 2010; 16(1):65-78; PMID:19700742; http://dx.doi.org/10.1177/1073858409340702
- Tojima T, Hines JH, Henley JR, Kamiguchi H. Second messengers and membrane trafficking direct and organize growth cone steering. Nat Rev Neurosci 2011; 12(4):191-203; PMID:21386859; http://dx.doi.org/10.1038/nrn2996
- Basile JR, Barac A, Zhu T, Guan KL, Gutkind JS. Class IV semaphorins promote angiogenesis by stimulating Rho-initiated pathways through plexin-B. Cancer Res 2004; 64(15):5212-24; PMID:15289326; http://dx.doi.org/10.1158/0008-5472.CAN-04-0126
- English D, Garcia JGN, Brindley DN. Platelet-released phospholipids link haemostasis and angiogenesis. Cardiovasc Res 2001; 49(3):588-99; PMID:11166272; http://dx.doi.org/10.1016/S0008-6363(00)00230-3
- Muñoz-Chápuli R, Quesada AR, Medina MÁ. Angiogenesis and signal transduction in endothelial cells. Cell Mol Life Sci 2004; 61(17):2224-43; PMID:15338053; http://dx.doi.org/10.1007/s00018-004-4070-7
- Kim S, Dong J, Lord EM. Pollen Tube Guidance: The Role of Adhesion and Chemotropic Molecules. Curr Top Dev Biol 2004; 61:61-79; PMID:15350397
- Palanivelu R, Preuss D. Pollen tube targeting and axon guidance: Parallels in tip growth mechanisms. Trends Cell Biol 2000; 10(12):517-24; PMID:11121743; http://dx.doi.org/10.1016/S0962-8924(00)01849-3
- Daniels KJ, Srikantha T, Lockhart SR, Pujol C, Soll DR. Opaque cells signal white cells to form biofilms in Candida albicans. EMBO J 2006; 25(10):2240-52; PMID:16628217; http://dx.doi.org/10.1038/sj.emboj.7601099
- Snetselaar KM, Bolker M, Kahmann R. Ustilago maydis mating hyphae orient their growth toward Pheromone Sources. Fungal Genet Biol 1996; 20(4):299-312; PMID:9045760; http://dx.doi.org/10.1006/fgbi.1996.0044
- King JS, Insall RH. Chemotaxis: finding the way forward with Dictyostelium. Trends Cell Biol 2009; 19(10):523-30; PMID:19733079; http://dx.doi.org/10.1016/j.tcb.2009.07.004
- Van Haastert PJM, Devreotes PN. Chemotaxis: signalling the way forward. Nat Rev Mol Cell Biol 2004; 5(8):626-34; PMID:15366706; http://dx.doi.org/10.1038/nrm1435
- Bardwell L. A walk-through of the yeast mating pheromone response pathway. Peptides 2004; 25(9):1465-76; PMID:15374648; http://dx.doi.org/10.1016/j.peptides.2003.10.022
- Elion EA. Pheromone response, mating and cell biology. Curr Opin Microbiol 2000; 3(6):573-81; PMID:11121776; http://dx.doi.org/10.1016/S1369-5274(00)00143-0
- Pruyne D, Bretscher A. Polarization of cell growth in yeast. J Cell Sci 2000; 113(Pt 4):571-85; PMID:10652251
- Dorer R, Pryciak PM, Hartwell LH. Saccharomyces cerevisiae cells execute a default pathway to select a mate in the absence of pheromone gradients. J Cell Biol 1995; 131(4):845-61; PMID:7490289; http://dx.doi.org/10.1083/jcb.131.4.845
- Park HO, Bi E. Central roles of small GTPases in the development of cell polarity in yeast and beyond. Microbiol Mol Biol Rev 2007; 71(1):48-96; PMID:17347519; http://dx.doi.org/10.1128/MMBR.00028-06
- Merlini L, Dudin O, Martin SG. Mate and fuse: how yeast cells do it. Open Biol 2013; 3(3):130008; PMID:23466674; http://dx.doi.org/10.1098/rsob.130008
- Segall JE. Polarization of yeast cells in spatial gradients of alpha mating factor. Proc Natl Acad Sci U S A 1993; 90(18):8332-6; PMID:8397402; http://dx.doi.org/10.1073/pnas.90.18.8332
- Jackson CL, Hartwell LH. Courtship in S. cerevisiae: Both cell types choose mating partners by responding to the strongest pheromone signal. Cell 1990; 63(5):1039-51; PMID:2257622; http://dx.doi.org/10.1016/0092-8674(90)90507-B
- Schrick K, Garvik B, Hartwell LH. Mating in Saccharomyces cerevisiae: The role of the pheromone signal transduction pathway in the chemotropic response to pheromone. Genetics 1997; 147(1):19-32; PMID:9286665
- Moore TI, Chou CS, Nie Q, Jeon NL, Yi TM. Robust spatial sensing of mating pheromone gradients by yeast cells. PLoS One 2008; 3(12):e3865; PMID:19052645; http://dx.doi.org/10.1371/journal.pone.0003865
- Paliwal S, Iglesias PA, Campbell K, Hilioti Z, Groisman A, Levchenko A. MAPK-mediated bimodal gene expression and adaptive gradient sensing in yeast. Nature 2007; 446(7131):46-51; PMID:17310144; http://dx.doi.org/10.1038/nature05561
- Ismael A, Tian W, Waszczak N, Wang X, Cao Y, Suchkov D, Bar E, Metodiev MV, Liang J, Arkowitz RA, et al. Gβ promotes pheromone receptor polarization and yeast chemotropism by inhibiting receptor phosphorylation. Sci Signal 2016; 9(423):ra38; PMID:27072657; http://dx.doi.org/10.1126/scisignal.aad4376
- Nern A, Arkowitz RA. A Cdc24p-Far1p-G beta gamma protein complex required for yeast orientation during mating. J Cell Biol 1999; 144(6):1187-202; PMID:10087263; http://dx.doi.org/10.1083/jcb.144.6.1187
- Butty AA, Pryciak PM, Huang LS, Herskowitz I, Peter M. The role of Farip in linking G protein to heterotrimeric potarity establishment proteins during yeast mating. Science 2011; 1511-1516.
- Suchkov DV, DeFlorio R, Draper E, Ismael A, Sukumar M, Arkowitz R, Stone DE. Polarization of Yeast Pheromone Receptor Requires Its Internalization but Not Actin-dependent Secretion. Mol Biol Cell 2010; 21(10):1737-52; PMID:20335504; http://dx.doi.org/10.1091/mbc.E09-08-0706
- Ayscough KR, Drubin DG. A role for the yeast actin cytoskeleton in pheromone receptor clustering and signalling. Curr Biol 1998; 8(16):927-30; PMID:9707405; http://dx.doi.org/10.1016/S0960-9822(07)00374-0
- Dyer JM, Savage NS, Jin M, Zyla TR, Elston TC, Lew DJ. Tracking shallow chemical gradients by actin-driven wandering of the polarization site. Curr Biol 2013; 23(1):32-41; PMID:23200992; http://dx.doi.org/10.1016/j.cub.2012.11.014
- Hegemann B, Unger M, Lee SS, Stoffel-Studer I, van den Heuvel J, Pelet S, Koeppl H, Peter M. A cellular system for spatial signal decoding in chemical gradients. Dev Cell 2015; 35(4):458-70; PMID:26585298; http://dx.doi.org/10.1016/j.devcel.2015.10.013
- Bendezú FO, Martin SG. Cdc42 explores the cell periphery for mate selection in fission yeast. Curr Biol 2013; 23(1):42-7; PMID:23200991; http://dx.doi.org/10.1016/j.cub.2012.10.042
- McClure AW, Minakova M, Dyer JM, Zyla TR, Elston TC, Lew DJ. role of polarized G protein signaling in tracking pheromone gradients. Dev Cell 2015; 35(4):471-82; PMID:26609960; http://dx.doi.org/10.1016/j.devcel.2015.10.024