Abstract
Bacteriophages have regained much attention as biocontrol agents against bacterial pathogens. However, with respect to stability, phages are biomolecules and are therefore sensitive to a number of environmental influences. UV-irradiation can readily inactivate phage infectivity, which impedes their potential application in the plant phyllosphere. Therefore, phages for control of Erwinia amylovora, the causative agent of fire blight, need to be protected from UV-damage by adequate measures. We investigated the protective effect of different light-absorbing substances on phage particles exposed to UV-light. For this, natural extracts from carrot, red pepper, and beetroot, casein and soy peptone in solution, and purified substances such as astaxanthin, aromatic amino acids, and Tween 80 were prepared and tested as natural sunscreens for phage. All compounds were found to significantly increase half-life of UV-irradiated phage particles and they did not negatively affect phage viability or infectivity. Altogether, a range of readily available, natural substances are suitable as UV-protectants to prevent phage particles from UV-light damage.
Abbreviations
DMSO | = | Dimethyl sulfoxide |
DNA | = | Deoxyribonucleic acid |
PFU | = | Plaque forming units |
Phe | = | Phenylalanine |
SM | = | Phage buffer containing sodium and magnesium |
Trp | = | Tryptophan |
Tyr | = | Tyrosine |
UV | = | Ultraviolet. |
Introduction
Erwinia amylovora is the causative agent of fire blight, a devastating plant disease affecting Rosaceae species, and a major threat to pome fruit production causing high economic losses every year.Citation1 To date, the most effective control option is the prophylactic application of antibiotics, e.g., streptomycin, during the flowering period. The use is limited due to regulatory restrictions, pathogen resistance development, and consumers' demands.Citation2
Biocontrol of bacterial plant pathogens using bacteriophages (phages) has gained increased attention in agriculture as an alternative treatment,Citation3,4 and several studies have demonstrated the potential of phages to control fire blight. Phage-treatment significantly reduced the number of viable E. amylovora and symptom development in vitro,Citation5,6 on immature pear fruit,Citation7,8 on detached flowers,Citation8,9 and on apple trees.Citation9,10 Furthermore, “Erwiphage,” a bacteriophage cocktail consisting of 2 phages, was recently introduced and is commercially available.Citation11
However, unfavorable conditions, which prevail in the phyllosphere, can inactivate the phage, i.e., variation of temperature and pH, rain, desiccation, and sunlight irradiation, with the latter being the most detrimental factor for phage persistence.Citation12-14 Light of a wide spectrum, i.e., 254 nm, UVB (280-320 nm), and > 320 nm, was shown to have a negative impact on phage DNA.Citation15-21 Sunlight-mediated damage of phage particles is characterized by the formation of pyrimidine (mostly thymine) dimers in DNA caused by direct absorption of UV-light.Citation22 The decay of infective phage is in direct correlation with the received irradiation dose.Citation15,17 Given that the number of infective phage is proportional to the outcome of the treatment, phage persistence must be guaranteed by adequate measures.Citation13 A feasible option is the avoidance of exposure to sunlight by applying the phage in the evening, as shown in experiments on tomato leaves.Citation14,23 Another option to improve phage persistence in the phyllosphere is the application of an avirulent bacterial strain, which supports phage replication. This strategy was successfully applied with phages of E. amylovora (carrier: Pantoea agglomerans) on apple treesCitation9 and on tomato leaves using an attenuated Xanthomonas perforans strain as host bacterium.Citation24 Phages can also be protected from inactivation by the addition of substances, which absorb and prevent phages from UV-light. Balogh et al.Citation23 developed 3 different formulations including (i) pregelatinized corn flour (PCF) and sucrose; (ii) casecrete, sucrose, and PCF; and (iii) skim milk and sucrose (M+S). Phage-longevity was significantly increased and a reduction of disease severity was achieved. In a subsequent study, the formulation with M+S was found to successfully prevent the decline of infective phages caused by temperature, desiccation, fluorescent light, and copper.Citation14
In general, any substance absorbing detrimental light offers potential as a protectant. Plant compounds such as pigments (e.g., carotenoids or betalaines) or polyphenols absorb light of a wide spectral range and could reduce the inactivation of phages by sunlight irradiation. They are readily available, natural, and safe substances demonstrated by their use as colorants and antioxidants in the food-industry.Citation25-27 Alternatively, peptides or aromatic amino acids could have a similar effect. As a proof of concept study, we investigated the efficacy of juices from vegetables (carrot, red pepper, and beetroot), astaxanthin, a pure carotenoid from the freshwater chlorophyte algae Haematococcus pluvialis,Citation28 aromatic amino acids, casein, peptone, and Tween 80 to prevent E. amylovora phage Y2 (vB_EamM-Y2), a virulent myovirus,Citation5 from UV-damage.
Materials and Methods
Preparation of plant extracts and UV absorbing substances
A series of substances were tested for their abilities to protect Y2 from UV-inactivation. These included juice of beetroot, red pepper, and carrot, casein from bovine milk, soy peptone, astaxanthin, aromatic amino acids (Phe, Trp, Tyr), and Tween 80. Carrot juice, red peppers and beetroots were bought in a local grocery store. The juice from the peppers was extracted using a commercial juice squeezer. Beetroots were cut into pieces and homogenized using an Ultra-Turrax high speed blender (IKA®-Werke GmbH & Co. KG) for 5 min. Solid particles were removed from all juices by centrifugation (1000 ×g, 5 min) and serial passages through filters. The final filter had a pore size of 0.2 μm. The juices were diluted in SM buffer (50 mM Tris, 100 mM NaCl, 8 mM MgSO4, pH 7.4) to a stock-concentration of 20% ([vol/vol], carrot and beetroot) or 10% ([vol/vol], red pepper) and stored at 4 °C. Casein from bovine milk (Sigma-Aldrich) was dissolved in 1 M NaOH (final concentration: 20 mg/ml), soy peptone in ddH2O (100 mg/ml), astaxanthin (Sigma-Aldrich) in DMSO (6 mg/ml), aromatic amino acids (Sigma-Aldrich) in ddH2O (100 mM each), and Tween 80 (Sigma-Aldrich) in ddH2O (10%, [vol/vol]).
UV sensitivity assays
In wells of a 96 multiwell plate (Nunc GmbH & Co. KG), 100 μl aliquots containing phage Y2 (2 × 108 PFU/ml) in SM buffer were mixed with 100 μl of the UV-absorbing substance or a dilution thereof. A UV lamp (λ = 254 nm; Camag 29200), switched on 15 min prior to use, was placed 6 cm above the plate assuring equal radiation of every well (an estimated 1 mW/cm2 was applied). The wavelength of 254 nm was used in the present work since it is near the peak absorption of DNA and it was the wavelength of choice for a vast number of studies on UV-inactivation due to its detrimental impact on DNA.Citation29 After 0 (no irradiation), 30, 60, 120, and 300 s of exposure to UV-light, the remainder of infective phage particles was determined by the soft agar overlay methodCitation30 using E. amylovora strain 4/82 as host as described previously.Citation5 Each experiment was carried out in triplicates and independently repeated twice.
In addition, phages were mixed with the UV-absorbing substances without being irradiated to investigate if the UV-protectants influence the number of infective phage. The concentrations of phage were determined with the same soft agar overlay method at the same time points as above.
Statistical analysis
Statistical significance of the effects observed after 5 min of irradiation was evaluated by analysis of variance (ANOVA).
Results
The inactivation of phage Y2 by UV-irradiation could be minimized by addition of different substances to the phage suspension. Yet, the UV-protective effects differed depending on the substance and the concentration applied. Importantly, phage Y2 was not adversely affected by the UV-protectants, i.e., the number of PFU mixed with any substance remained constant over 5 min (data not shown). In contrast, the number of infective particles not supplemented with a UV-protectant was rapidly reduced by UV-light. This demonstrates the instability of phages exposed to UV-irradiation. In average, only 0.21% of the initial number of PFU remained infective after being irradiated with UV-light for 5 min.
When treated with carrot (10%) or red pepper juice (5%), 28% and 34%, respectively, of the original number of PFU remained infective after 5 min of UV-irradiation. The number of infective particles was approximately 2 logs higher compared to the untreated controls (). These protective effects were found to be highly significant (P < 0.01). Beetroot juice also showed a concentration-dependent efficacy. With the concentrations applied, a lower protection was achieved compared to the juices of carrot and red pepper. Only 8% of the UV-irradiated phages treated with 10% beetroot juice remained infective after 5 min. Nevertheless, beetroot juice also provided a highly significant protection compared to the untreated control (P < 0.01) ().
Figure 1. Effect of carrot, red pepper, and beetroot juice on the time-dependent decay of UV-irradiated phage particles. Values indicate means of 3 independent experiments ± standard deviations. (A): Carrot juice, circles: control without protectant, squares: 1% carrot juice, triangles: 10% carrot juice. (B): Red pepper juice, circles: control without protectant, triangles: 5% red pepper juice. (C): Beetroot juice, circles: control without protectant, squares: 1% beetroot juice, triangles: 10% beetroot juice.
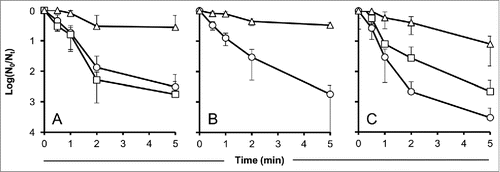
A mixture of the aromatic amino acids phenylalanine, tryptophan, and tyrosine also provided a UV-protection to phage Y2. After 5 min of exposure to UV-light, the remainder of infective phage was as high as 30% (5 mM of each amino acid at a ratio of 1:1:1) and 38% (50 mM of each amino acid at a ratio of 1:1:1), respectively (). As expected, soy peptone and casein also protected Y2 from degradation. Phage-inactivation could be reduced by >2 logs (50 mg/ml peptone) and approximately 1 log (10 mg/ml casein) (). The protection activities of the amino acids, soy peptone, and casein were found to be highly significant (P < 0.01).
Figure 2. Effect of pure aromatic amino acids (Tyr, Trp, Phe) and peptides (peptone and casein) on the time-dependent decay of UV-irradiated phage particles. Values indicate means of 3 independent experiments ± standard deviations. (A): amino acids, circles: control without protectant, squares: 5 mM amino acids, triangles: 50 mM amino acids. (B): Peptone, circles: control without protectant, triangles: 50 mg/ml peptone. (C): Casein, circles: control without protectant, squares: 5 mg/ml casein, triangles: 10 mg/ml casein.
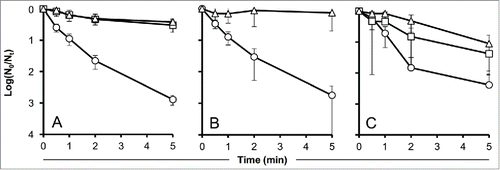
Finally, 24% of the original number of PFU were still infective after UV treatment when protected by the pure carotenoid astaxanthin (3 mg/ml), which was also found to be highly significant (P < 0.01). UV-protection by Tween 80 was weak in our experimental setup. Only 0.5% of the number of phage at t0 formed plaques after 5 min of UV-irradiation. Still, the effect was found to be significant (P < 0.05) ().
Figure 3. Effect of astaxanthin and Tween 80 on the time-dependent decay of UV-irradiated phage particles. Values indicate means of 3 independent experiments ± standard deviations. (A): Astaxanthin, circles: control without protectant, triangles: 3 mg/ml astaxanthin. (B): Tween 80, circles: control without protectant, triangles: 5 % Tween 80.
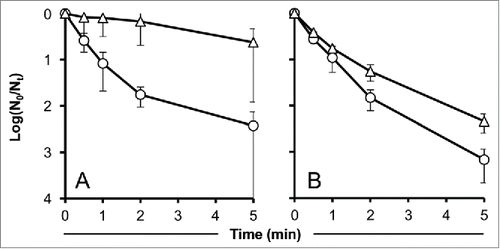
Discussion
As a proof of concept, we could show the UV-protective activity of various substances. All tested substances significantly reduced the inactivation of phage Y2 by UV-light.
The UV-protecting activity of the carrot, red pepper and beetroot extracts could be attributed to plant pigments. The major pigments of carrots are α- and β-carotenesCitation31 with an absorption maximum (λmax) of approximately 450 nm.Citation25 Capsanthin and capsorubin are the predominant pigments of Capsicum spp., e.g., red pepper. Compared to α- and β-carotenes, their absorption is slightly shifted toward longer wavelengths with a maximum at around 480 nm.Citation31 Carotenes, capsanthin, and capsorubin are carotenoids and composed of a polyene backbone with a variable number of conjugated double bonds and specific end-groups. They fulfill an important role in photosynthesis due to their potential to absorb light and are known as antioxidants. Their absorption spectra and maxima are defined by the number of conjugated double bonds.Citation25,32 Members of the Caryophyllales (e.g., beetroot) typically produce betalaines. The red pigment betanin is characterized by a λmax of approximately 540 nm and is abundant in beetroot.Citation26,33
It is striking that the absorption maxima of the carotenoids and betalaines and the wavelength used in this study for phage-inactivation differ considerably. Thus, other substances present in the juices, e.g., polyphenols, might be responsible for the protective effects. Carrots,Citation34,35 peppers,Citation36,37 and beetrootsCitation38 are known to contain various phenolic compounds. Their absorption maxima typically lie in the range of 200 - 400 nm,Citation39,40 which corresponds to the applied wavelength of 254 nm. Thus, phenolic compounds are likely involved in UV protection by direct absorption.
Interestingly, the pure carotenoid astaxanthin, produced by the chlorophyte algae H. pluvialis, also attenuated the detrimental effect of UV-light on phage. Astaxanthin is a close relative of β-carotene with an absorption maximum at approximately 480 nm.Citation28,31 This finding indicates that carotenoids might also exhibit a UV-protective effect. Hence, plant pigments could protect phages from UV-irradiation by either absorption or by prevention from oxidative stress. However, although pigments are known as antioxidants,Citation25,28 oxidative damage was found to play a minor role in the inactivation of phage M13 by UVC-irradiation.Citation17 Instead, extensive pyrimidine dimer formation caused by light of 254 nm as a consequence of direct absorption, was reported for phages M13, T7, and T4.Citation16,17,41
The amino acids phenylalanine, tryptophan, and tyrosine were also found to protect Y2 from inactivation. They share an aromatic ring, which enables them to absorb light at longer wavelengths than other amino acids. Their absorption maxima lie at around 260 nm (Phe) and 280 nm (Trp, Tyr),Citation42 i.e., in the range of the applied UV-light. Therefore, direct absorption might be the mechanism by which phage Y2 was protected.
The efficacy of soy peptone and casein is probably owing to the protein and peptide compounds similar to the protective ability of casecrete and skim milk.Citation23 Tween 80 was the substance with the lowest protective activity, although it does absorb in the critical range. This non-ionic detergent is a complex mixture of oligomers and absorbs at 200-300 nm, with a peak at approximately 235 nm.Citation43,44 The concentration used might have been too low.
In conclusion, a selection of different natural substances was found to reduce UV-mediated inactivation of phage particles. In addition, preliminary data indicate that the results presented in this study may be translated to other phages from the family of Myoviridae and Podoviridae. The efficacy was dependent on the concentration, i.e., our observations were in agreement with the Beer-Lambert law. Yet, we cannot draw clear conclusions regarding the nature of the protective ingredients in the plant extracts. Neither can we identify the UV-protectant with the highest efficacy since the concentrations of the absorbing substances cannot be directly compared. Therefore, for practical applications, defined substances (e.g., astaxanthin or amino acids) should be preferred over complex compositions (e.g., vegetable extracts or peptone). The efficacy of these substances needs to be assessed under in vivo conditions, where phages and protectants are confronted with the natural spectrum of UV-light over longer periods of exposure.
Disclosure of Potential Conflicts of Interest
No potential conflicts of interest were disclosed.
Acknowledgments
We thank Mira Hochstrasser and Janine Marazzi for excellent technical assistance.
Funding
This work was funded by the Swiss Federal Office for Agriculture (BLW Fire Blight Project – Biocontrol) and was conducted within the European Science Foundation COST Action 864 and Swiss ProfiCrops research networks.
References
- Bonn WG, van der Zwet T. Distribution and economic importance of fire blight. In: Vanneste JL, ed. Fire Blight: The Disease and its Causative Agent, Erwinia amylovora. Wallingford: CAB International, 2000; 37-53
- Stockwell VO, Duffy B. Use of antibiotics in plant agriculture. Rev Sci Tech 2012; 31:199-210; PMID:22849276
- Żaczek M, Weber-Dąbrowska B, Górski A. Phages in the global fruit and vegetable industry. J Appl Microbiol 2015; 118:537-56; PMID:25410419; http://dx.doi.org/10.1111/jam.12700
- Balogh B, Jones JB, Iriarte FB, Momol MT. Phage therapy for plant disease control. Curr Pharm Biotechnol 2010; 11:48-57; PMID:20214607; http://dx.doi.org/10.2174/138920110790725302
- Born Y, Fieseler L, Marazzi J, Lurz R, Duffy B, Loessner MJ. Novel virulent and broad-host-range Erwinia amylovora bacteriophages reveal a high degree of mosaicism and a relationship to Enterobacteriaceae phages. Appl Environ Microbiol 2011; 77:5945-54; PMID:21764969; http://dx.doi.org/10.1128/AEM.03022-10
- Schnabel EL, Jones AL. Isolation and characterization of five Erwinia amylovora bacteriophages and assessment of phage resistance in strains of Erwinia amylovora. Appl Environ Microbiol 2001; 67:59-64; PMID:11133428; http://dx.doi.org/10.1128/AEM.67.1.59-64.2001
- Erskine JM. Characteristics of Erwinia amylovora bacteriophage and its possible role in the epidemiology of fire blight. Can J Microbiol 1973; 19:837-45; PMID:4125539; http://dx.doi.org/10.1139/m73-134
- Müller I, Lurz R, Kube M, Quedenau C, Jelkmann W, Geider K. Molecular and physiological properties of bacteriophages from North America and Germany affecting the fire blight pathogen Erwinia amylovora. Microb Biotechnol 2011; 4:735-45; PMID:21791029; http://dx.doi.org/10.1111/j.1751-7915.2011.00272.x
- Boulé J, Sholberg PL, Lehman SM, O'Gorman DT, Svircev AM. Isolation and characterization of eight bacteriophages infecting Erwinia amylovora and their potential as biological control agents in British Columbia, Canada. Can J Plant Pathol 2011; 33:308-17; PMID:Can't; http://dx.doi.org/10.1080/07060661.2011.588250
- Schnabel EL, Fernando WGD, Meyer MP, Jones AL, Jackson LE. Bacteriophage of Erwinia amylovora and their potential for biocontrol. ISHS Acta Hortic 1999; 489:649-53
- Meczker K, Dömötör D, Vass J, Rákhely G, Schneider G, Kovács T. The genome of the Erwinia amylovora phage PhiEaH1 reveals greater diversity and broadens the applicability of phages for the treatment of fire blight. FEMS Microbiol Lett 2014; 350:25-7; PMID:24551880; http://dx.doi.org/10.1111/1574-6968.12319
- Jones JB, Jackson LE, Balogh B, Obradovic A, Iriarte FB, Momol MT. Bacteriophages for plant disease control. Annu Rev Phytopathol 2007; 45:245-62; PMID:17386003; http://dx.doi.org/10.1146/annurev.phyto.45.062806.094411
- Svircev AM, Castle AJ, Lehman SM. Bacteriophages for control of phytopathogens in food production systems. In: Sabour PM, Griffiths MW, eds. Bacteriophages in the Control of Food- and Waterborne Pathogens. Washington, DC: ASM Press, 2010; 79-96.
- Iriarte FB, Balogh B, Momol MT, Smith LM, Wilson M, Jones JB. Factors affecting survival of bacteriophage on tomato leaf surfaces. Appl Environ Microbiol 2007; 73:1704-11; PMID:17259361; http://dx.doi.org/10.1128/AEM.02118-06
- Suttle CA, Chen F. Mechanisms and rates of decay of marine viruses in seawater. Appl Environ Microbiol 1992; 58:3721-9; PMID:16348812
- Tyrrell RM. Repair of near (365 nm)- and far (254 nm)- UV damage to bacteriophage of Escherichia coli. Photochem Photobiol 1979; 29:963-70; PMID:538092; http://dx.doi.org/10.1111/j.1751-1097.1979.tb07799.x
- Kurosaki Y, Abe H, Morioka H, Hirayama J, Ikebuchi K, Kamo N, Nikaido O, Azuma H, Ikeda H. Pyrimidine dimer formation and oxidative damage in M13 bacteriophage inactivation by ultraviolet C irradiation. Photochem Photobiol 2003; 78:349-54; PMID:14626662; http://dx.doi.org/10.1562/0031-8655(2003)078%3c0349:PDFAOD%3e2.0.CO;2
- Diston D, Ebdon JE, Taylor HD. The effect of UV-C radiation (254 nm) on candidate microbial source tracking phages infecting a human-specific strain of Bacteroides fragilis (GB-124). J Water Health 2012; 10:262-70; PMID:22717751; http://dx.doi.org/10.2166/wh.2012.173
- Diston D, Ebdon JE, Taylor HD. Inactivation of bacteriophage infecting Bacteroides strain GB124 using UV-B radiation. Photochem Photobiol 2014; 90:622-7; PMID:24329482; http://dx.doi.org/10.1111/php.12223
- Sommer R. Inactivation of bacteriophages in water by means of non-ionizing (UV-253.7nm) and ionizing (gamma) radiation: a comparative approach. Water Res 2001; 35:3109-16; PMID:11487107; http://dx.doi.org/10.1016/S0043-1354(01)00030-6
- Rodriguez RA, Bounty S, Beck S, Chan C, McGuire C, Linden KG. Photoreactivation of bacteriophages after UV disinfection: role of genome structure and impacts of UV source. Water Res 2014; 55:143-9; PMID:24607520; http://dx.doi.org/10.1016/j.watres.2014.01.065
- Ravanat JL, Douki T, Cadet J. Direct and indirect effects of UV radiation on DNA and its components. J Photochem Photobiol B Biol 2001; 63:88-102; http://dx.doi.org/10.1016/S1011-1344(01)00206-8
- Balogh B, Jones JB, Momol MT, Olson SM, Obradovic A, King P, Jackson LE. Improved efficacy of newly formulated bacteriophages for management of bacterial spot on tomato. Plant Dis 2003; 87:949-54; http://dx.doi.org/10.1094/PDIS.2003.87.8.949
- Iriarte FB, Obradović A, Wernsing MH, Jackson LE, Balogh B, Hong JA, Momol MT, Jones JB, Vallad GE. Soil-based systemic delivery and phyllosphere in vivo propagation of bacteriophages: Two possible strategies for improving bacteriophage persistence for plant disease control. Bacteriophage 2012; 2:215-24; PMID:23532156; http://dx.doi.org/10.4161/bact.23530
- Namitha KK, Negi PS. Chemistry and biotechnology of carotenoids. Crit Rev Food Sci Nutr 2010; 50:728-60; PMID:20830634; http://dx.doi.org/10.1080/10408398.2010.499811
- Strack D, Vogt T, Schliemann W. Recent advances in betalain research. Phytochemistry 2003; 62:247-69; PMID:12620337; http://dx.doi.org/10.1016/S0031-9422(02)00564-2
- Manach C, Scalbert A, Morand C, Remesy C, Jimenez L. Polyphenols: food sources and bioavailability. Am J Clin Nutr 2004; 79:727-47; PMID:15113710
- Guerin M, Huntley ME, Olaizola M. Haematococcus astaxanthin: applications for human health and nutrition. Trends Biotechnol 2003; 21:210-6; PMID:12727382; http://dx.doi.org/10.1016/S0167-7799(03)00078-7
- Coohill TP, Sagripanti JL. Overview of the inactivation by 254 nm ultraviolet radiation of bacteria with particular relevance to biodefense. Photochem Photobiol 2008; 84:1084-90; PMID:18627518
- Adams MH. Bacteriophages. New York: Interscience Publishers Inc., 1959.
- Rodriguez-Amaya DB. A guide to carotenoid analysis in foods. Washington DC: ILSI Press, 2001.
- Khoo HE, Prasad KN, Kong KW, Jiang YM, Ismail A. Carotenoids and their isomers: color pigments in fruits and vegetables. Molecules 2011; 16:1710-38; PMID:21336241; http://dx.doi.org/10.3390/molecules16021710
- Harmer RA. Occurrence, chemistry and application of betanin. Food Chem 1980; 5:81-90; http://dx.doi.org/10.1016/0308-8146(80)90066-7
- Quitão-Teixeira LJ, Odriozola-Serrano I, Soliva-Fortuny R, Mota-Ramos A, Martín-Belloso O. Comparative study on antioxidant properties of carrot juice stabilised by high-intensity pulsed electric fields or heat treatments. J Sci Food Agric 2009; 89:2636-42; http://dx.doi.org/10.1002/jsfa.3767
- Ma T, Tian C, Luo J, Zhou R, Sun X, Ma J. Influence of technical processing units on polyphenols and antioxidant capacity of carrot (Daucus carrot L.) juice. Food Chem 2013; 141:1637-44; PMID:23870871; http://dx.doi.org/10.1016/j.foodchem.2013.04.121
- Jeong WY, Jin JS, Cho YA, Lee JH, Park S, Jeong SW, Kim YH, Lim CS, Abd El-Aty AM, Kim GS, et al. Determination of polyphenols in three Capsicum annuum L. (bell pepper) varieties using high-performance liquid chromatography-tandem mass spectrometry: their contribution to overall antioxidant and anticancer activity. J Sep Sci 2011; 34:2967-74; PMID:21898818; http://dx.doi.org/10.1002/jssc.201100524
- Sun T, Xu Z, Wu CT, Janes M, Prinyawiwatkul W, No HK. Antioxidant activities of different colored sweet bell peppers (Capsicum annuum L.). J Food Sci 2007; 72:98-102; http://dx.doi.org/10.1111/j.1750-3841.2006.00245.x
- Kujala TS, Vienola MS, Klika KD, Loponen JM, Pihlaja K. Betalain and phenolic compositions of four beetroot (Beta vulgaris) cultivars. Eur Food Res Technol 2002; 214:505-10; http://dx.doi.org/10.1007/s00217-001-0478-6
- Tsimogiannis D, Samiotaki M, Panayotou G, Oreopoulou V. Characterization of flavonoid subgroups and hydroxy substitution by HPLC-MS/MS. Molecules 2007; 12:593-606; PMID:17851414; http://dx.doi.org/10.3390/12030593
- Robbins RJ. Phenolic acids in foods: an overview of analytical methodology. J Agric Food Chem 2003; 51:2866-87; PMID:12720366; http://dx.doi.org/10.1021/jf026182t
- Fekete A, Vink AA, Gaspar S, Modos K, Berces A, Ronto G, Roza L. Influence of phage proteins on formation of specific UV DNA photoproducts in phage T7. Photochem Photobiol 1999; 69:545-52; PMID:10333760
- Sprenger GA. Aromatic amino acids. In: Wendisch VF, ed. Amino acid biosynthesis. Berlin: Springer-Verlag, 2007; 93-127.
- Wuelfing WP, Kosuda K, Templeton AC, Harman A, Mowery MD, Reed RA. Polysorbate 80 UV/vis spectral and chromatographic characteristics - defining boundary conditions for use of the surfactant in dissolution analysis. J Pharm Biomed Anal; Internet 2006; 41:774-82
- Ayorinde FO, Gelain S V, Johnson JH, Wan LW. Analysis of some commercial polysorbate formulations using matrix-assisted laser desorption/ionization time-of-flight mass spectrometry. Rapid Commun Mass Spectrom 2000; 14:2116-24; PMID:11114018; http://dx.doi.org/10.1002/1097-0231(20001130)14:22%3c2116::AID-RCM142%3e3.0.CO;2-1