ABSTRACT
The occurrence of antibiotic-resistant bacterial pathogens is on the rise because antibiotics exert selection pressure that kills only the antibiotic-sensitive pathogens. Sanitation and cleansing of hospital surfaces and the skin of medical personnel do not counteract this selective pressure, but rather indiscriminately reduce total pathogens on treated surfaces. Here, we discuss two recently introduced genetic strategies, based on temperate bacteriophages as DNA-delivery vehicles, that aim to sensitize bacteria to antibiotics and selectively kill the antibiotic-resistant ones. Outlooks for rendering one such approach more efficient and applicable are proposed. We believe that using an end product designed according to the provided principles on hospital surfaces and in hand-sanitizers will facilitate substitution of antibiotic-resistant pathogens with sensitive ones.
Bacterial pathogen resistance to antibiotics is a major health threat worldwide. Infection rates by antibiotic-resistant pathogens continue to rise, and some pathogens are resistant to virtually all first- and second-line antibiotics.Citation1 Although this puts the entire population at risk, particularly susceptible are the hospitalized immunocompromised patients who are undergoing chemotherapy, dialysis or surgery (especially organ transplants), and are thus more prone to secondary infections.Citation1 Eliminating resistant pathogens residing on hospital surfaces, devices, and hands of medical personnel should therefore significantly improve the prospects of these patients to survive.
Bacterial pathogens evolve to overcome the therapeutic effect of antibiotics simply because antibiotics kill bacteria that are not resistant to them. This selective pressure, which kills sensitive bacteria but not resistant ones, is applied with each use of antibiotics. Various approaches to reducing the rapid emergence of antibiotic-resistant pathogens have been proposed. Refraining from use of a specific antibiotic has been shown to increase the ratio of bacteria that are sensitive to that antibiotic, but this effect disappears rapidly upon its reuse.Citation2 Antibiotics are misused by unnecessary prescriptions issued by physicians and by patients who take them at incorrect doses or duration, as well as by prophylactic or overuse, mostly in animal husbandry and food production.Citation1 Prudent use of antibiotics is advised to reduce the rate of resistance formation.Citation3 However, such use merely delays the evolution of antibiotic resistance without actively reducing it. Disinfection protocols for hospital surfaces as well as frequent hand disinfection by medical personnel indiscriminately eradicate both antibiotic-sensitive and antibiotic-resistant pathogens, without counteracting the selection pressure for resistant bacteria. On the contrary, disinfection agents applied to hospital surfaces and used for hand sanitization exert selection pressure for pathogens to become resistant to these agents. Lytic bacteriophages (phages) are also used as disinfection agents on consumed food. For example, a cocktail of six naturally occurring lytic phages targeting Listeria was approved by the United States Food and Drug Administration (US FDA) for specific decontamination of Listeria present on food.Citation4,5 While this product demonstrates that phages are both safe and effective at targeting environmental pathogens, the product does not aim to specifically target antibiotic-resistant pathogens. None of the above approaches exerts selection pressure that counteracts that created by antibiotics, i.e., a selection pressure that changes the ratio in favor of antibiotic-sensitive pathogens over antibiotic-resistant ones. Applying such a selection pressure might actively reverse the ratio of antibiotic-sensitive bacteria, thereby treating the core problem rather than its symptoms.
We previously reported a proof-of-principle system that sensitizes resistant bacteria and applies selective pressure against the remaining resistant bacteriaCitation6 (see comments in refs.Citation7-9). This system uses temperate phages to transfer sensitizing genes into bacteria. The sensitizing genes are then integrated into the bacterial genome by lysogenization of the temperate phage. The integrated DNA, encoding the dominant sensitive genes rpsL and gyrA, dominantly conferred sensitivity to two antibiotics, streptomycin and nalidixic acid. These sensitizing genes were linked to a gene conferring resistance to the toxic compound tellurite. This allowed using tellurite to target the remaining antibiotic-resistant bacteria that were sensitive to this compound, without killing the sensitive bacteria. The approach thus selected for antibiotic-sensitive bacteria by conferring a selective advantage through resistance to a toxic compound, while simultaneously sensitizing the bacteria to antibiotics ().
Figure 1. Different schemes for sensitizing bacteria to antibiotics. (A) A dominant sensitive gene, conferring sensitivity to antibiotics despite the presence of a resistance allele in the bacterium, is cloned with a tellurite resistance gene, conferring resistance to tellurite. This cassette is delivered by a lysogen into resistant bacteria, rendering them antibiotic sensitive, as well as tellurite-resistant. Addition of tellurite results in selection of antibiotic sensitive bacteria.Citation6 (B) A CRISPR-Cas cassette targets resistance genes and protects against lytic phages. The cassette is delivered by a lysogen into resistant bacteria, rendering them antibiotic sensitive, as well as phage-resistant. Lytic phages select antibiotic sensitive bacteria.Citation10
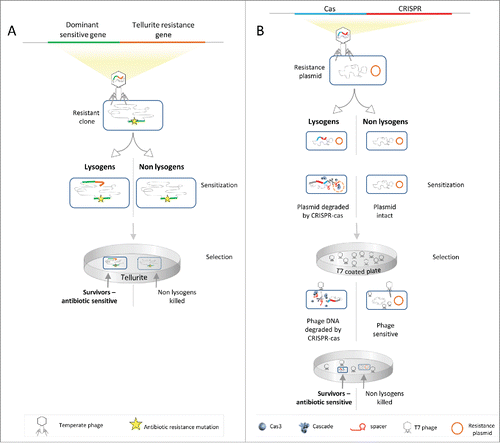
The above approach had several major shortcomings: (i) it reversed resistance to only two antibiotics, streptomycin and nalidixic acid; (ii) it did not prevent the spread of horizontally transferred resistance genes; (iii) it relied on prior sensitivity of the bacteria to tellurite, which could vanish upon extended usage; (iv) the host range of the temperate phages was narrow and therefore some resistant bacteria could escape treatment.
In a recently published study,Citation10 we made significant improvements that overcame the first three of these shortcomings. We used temperate phages that are capable of transferring DNA as a tool to deliver a CRISPR-Cas system into bacteria. The delivered CRISPR-Cas system was programmed to cleave antibiotic-resistant plasmids. In addition, the system, which originally evolved as a defense mechanism against phages,Citation11-13 was programmed to protect against specific lytic phages. This allowed us to genetically link phage protection to antibiotic sensitization. By using temperate phages to transfer the above CRISPR-Cas system and adding lethal phages (against which the sensitized bacteria were now protected), we changed the selective pressure in the treated samples such that sensitive bacteria were favored over resistant ones (bacteria that do not encode a protective CRISPR-Cas system) ().
Two recent studies also used phage delivery of CRISPR-Cas into bacteria to eliminate antibiotic-resistance genes or to specifically target a subpopulation of bacteria.Citation14,15 In contrast to those studies, our study showed, for the first time, the combination of selective lytic phages in the treatment to facilitate selection of treated bacteria that were sensitized to antibiotics. As opposed to studies that use the CRISPR-Cas system, lytic properties of phages, or other disinfection strategies, our aim was not to kill bacteria but rather to sensitize them. In counteracting the antibiotic's selective pressure, our strategy was expected to shift the ratio of pathogens toward antibiotic sensitivity.
The improved approach allows targeting various antibiotic-resistance plasmids, as the CRISPR-Cas can be programmed to cleave any DNA sequence.Citation16 In addition, the CRISPR-Cas system can be programmed to recognize many such determinants by extending the CRISPR array with appropriate sequences. Moreover, protection against lytic phages can be designed against multiple phages, thus allowing the use of phage cocktails to reduce the probability of resistance to all of them simultaneously. In addition to these improvements, the CRISPR-Cas system also prevents horizontal transfer of the targeted resistance genes. Finally, the lytic phages were engineered to have short DNA sequences that are identical to those targeted in the DNA conferring antibiotic resistance. Thus, phage protection is linked to antibiotic sensitization, as loss of the sensitizing CRISPR segment will result in loss of protection from the phage as well.
While the above improvements constitute a significant step toward applying the strategy to hospital surfaces and hand cleaners, some obstacles still need to be overcome. First, thus far we have only carried out proof-of-principle studies on laboratory strains of Escherichia coli, and not on pathogenic bacteria. To make the strategy operational, it must be tested on pathogenic bacteria. To this end, appropriate temperate and lytic phages should be collected for each targeted species. Fortunately, the lion's share of infections that are evolving antibiotic resistance at a dangerous rate are limited to six main pathogenic species, collectively termed the ESKAPE pathogens: Enterococcus faecium, Staphylococcus aureus, Klebsiella pneumoniae, Acinetobacter baumannii, Pseudomonas aeruginosa, and Enterobacter spp.Citation1 This means that finding cocktails of phages that are specific to one or more of these species will significantly reduce the occurrence of antibiotic-resistant infections. However, we foresee some technical problems in choosing suitable combinations of temperate phages that will deliver the DNA into these pathogens, and suitable combinations of lytic phages that will select for the sensitized pathogens. Therefore, a more general solution would be a universal platform for efficient DNA delivery to all pathogens.
As an end-product, we envision a spray or liquid composed of a cocktail of lytic phages and of a universal DNA-delivery vehicle. This product would be added to hand cleansers, washing detergents, and even as a stand-alone spray for surfaces. The fact that naturally occurring phages for human consumption have been approved by the US FDACitation4 suggests that genetically modified phages could also be approved for similar uses, as it is hard to imagine the harmful consequences of these controlled genetic modifications. On the contrary, the modifications of the lytic phages to be targeted by the engineered CRISPR-Cas system result in their targeting with no effect on the susceptibility of the pathogen to naturally occurring phages. We believe that the end product applied to pathogens on hospital surfaces or the hands of medical personnel could favorably change the ratio of treatable bacterial infections. In fact, as opposed to antibiotics, the more these products are used, the more sensitized the bacterial populations will become.
Disclosure of potential conflicts of interest
No potential conflicts of interest were disclosed.
References
- Pendleton JN, Gorman SP, Gilmore BF. Clinical relevance of the ESKAPE pathogens. Exp Rev Anti-Infect Ther 2013; 11:297-308; PMID:23458769; http://dx.doi.org/10.1586/eri.13.12
- Willemsen I, Cooper B, van Buitenen C, Winters M, Andriesse G, Kluytmans J. Improving quinolone use in hospitals by using a bundle of interventions in an interrupted time series analysis. Antimicrob Agents Chemother 2010; 54:3763-9; PMID:20585135; http://dx.doi.org/10.1128/AAC.01581-09
- Levy SB, O'Brien TF, Alliance for the Prudent Use of A. Global antimicrobial resistance alerts and implications. Clin Infect Dis 2005; 41 Suppl 4:S219-20; PMID:16032554; http://dx.doi.org/10.1086/432443
- Lang LH. FDA approves use of bacteriophages to be added to meat and poultry products. Gastroenterology 2006; 131:1370; PMID:17067600
- Abuladze T, Li M, Menetrez MY, Dean T, Senecal A, Sulakvelidze A. Bacteriophages reduce experimental contamination of hard surfaces, tomato, spinach, broccoli, and ground beef by Escherichia coli O157:H7. Appl Environ Microbiol 2008; 74:6230-8; PMID:18723643; http://dx.doi.org/10.1128/AEM.01465-08
- Edgar R, Friedman N, Molshanski-Mor S, Qimron U. Reversing Bacterial Resistance to Antibiotics by Phage-Mediated Delivery of Dominant Sensitive Genes. Appl Environ Microbiol 2012 Feb; 78(3):744-51; PMID:22113912
- Lukits A. A New Weapon in the Fight Against Superbugs. Wall Street J 2012; Retrieved from http://www.wsj.com/articles/SB10001424052970204331304577144853241744454.
- Gravitz L. Turning a new phage. Nat Med 2012; 18:1318-20; PMID:22961150; http://dx.doi.org/10.1038/nm0912-1318
- Jermy A. Antimicrobials: Reversing resistance with phage. Nat Rev Microbiol 2011; 10:83; PMID:22203376
- Yosef I, Manor M, Kiro R, Qimron U. Temperate and lytic bacteriophages programmed to sensitize and kill antibiotic-resistant bacteria. Proc Natl Acad Sci U S A 2015; 112:7267-72; PMID:26060300; http://dx.doi.org/10.1073/pnas.1500107112
- Marraffini LA, Sontheimer EJ. CRISPR interference: RNA-directed adaptive immunity in bacteria and archaea. Nat Rev Genet 2010; 11:181-90; PMID:20125085; http://dx.doi.org/10.1038/nrg2749
- Barrangou R, Fremaux C, Deveau H, Richards M, Boyaval P, Moineau S, Romero DA, Horvath P. CRISPR provides acquired resistance against viruses in prokaryotes. Science 2007; 315:1709-12; PMID:17379808; http://dx.doi.org/10.1126/science.1138140
- Hale CR, Zhao P, Olson S, Duff MO, Graveley BR, Wells L, Terns RM, Terns MP. RNA-guided RNA cleavage by a CRISPR RNA-Cas protein complex. Cell 2009; 139:945-56; PMID:19945378; http://dx.doi.org/10.1016/j.cell.2009.07.040
- Bikard D, Euler CW, Jiang W, Nussenzweig PM, Goldberg GW, Duportet X, Fischetti VA, Marraffini LA. Exploiting CRISPR-Cas nucleases to produce sequence-specific antimicrobials. Nat Biotechnol 2014; 32:1146-50; PMID:25282355; http://dx.doi.org/10.1038/nbt.3043
- Citorik RJ, Mimee M, Lu TK. Sequence-specific antimicrobials using efficiently delivered RNA-guided nucleases. Nat Biotechnol 2014; 32(11):1141-5; PMID:25240928
- Marraffini LA, Sontheimer EJ. CRISPR inteference limits horizontal gene transfer in staphylococci by targeting DNA. Science 2008; 322:1843-5; PMID:19095942; http://dx.doi.org/10.1126/science.1165771