Abstract
A novel and efficient route to thieno[3,4-c]pyrrole-4,6-dione (TPD) facilitating late-stage alkylation is presented. Four copolymers with alkylated bithiophene (2T) are synthesised, with different TPD N−alkyl side-chain branching points. The effect of steric bulk on solid-state properties is investigated (UV–VIS, differential scanning calorimetry, X-ray diffraction) and C1-branching found to increase crystallinity and solid-state packing in TPD-2T.
1. Introduction
Organic semiconducting materials have potential as large-scale, thin, flexible, lightweight and crucially low-cost electronic devices, fabricated by printing techniques. Currently organic field-effect transistors and bulk heterojunction (BHJ) solar cells routinely utilise π-conjugated polymers with donor–acceptor (D–A) hybridisation due to their readily tunable optical and electronic properties, with alkyl side-chains to afford the required solubility and processability.[Citation1–6]
However, the exact structure of the solubilising chain is often overshadowed by energetic contributions that influence the frontier orbital band gap, despite evidence that considerations such as side-chain spacing, branching and length can prove vital.[Citation7–12] The size of the solubilising chain is a key factor to consider during polymer synthesis: with a longer alkyl chain, solubility is generally gained.[Citation13] However, this can come at the detriment of other factors such as packing, blend morphology and fullerene miscibility.[Citation14,Citation15] To improve device microstructure attention has also been paid to the comparison of branched and linear alkyl chains, evaluating their effect on device performance, with the former preferred for organic photovoltaic applications and the latter for transistors.[Citation16,Citation17] More recently, a number of studies have begun to elucidate the effect of precision alkyl-chain engineering by shifting the branching point position, which has resulted in both improved photovoltaic efficiency and charge carrier mobility in field-effect transistors.[Citation18–20] However, the preferred side-chain is largely dependent on the exact polymer system. Our approach herein is to conduct a systematic study of the alkyl side-chain branching point position, investigating the effects of overall steric bulk on solid-state properties in a promising class of semiconducting polymers.
The thieno[3,4-c]pyrrole-4,6-dione (TPD) unit is a commonly used N−alkylated acceptor. The electron-deficient nature of the TPD unit lowers the HOMO levels of D–A copolymers and promotes intramolecular charge transfer, while the thiophene–maleimide structure takes a quinoidal form in the exicted state, lowering the bandgap. In addition,{} the planarity and ability to hydrogen-bond encourages intermolecular interactions such as π–π stacking. The relatively low cost of TPD further increases its potential for commercial use.
When copolymerised with electronically coupled electron-rich bithiophene (2T) – which also features rigid, aromatic rings with a coplanar structure – crystalline morphologies and enhanced charge transport are achieved. Use of a bi(alkyl)thiophene aids solubility, though the tail-to-tail configuration of the alkyl chains is vital in preserving crystallinity.[Citation21] Such alkylated TPD-2T polymers have achieved efficiencies as high as 7.3% in BHJ photovoltaic cells and field-effect transistors exhibit hole mobilities as high as 0.6 cm2/V s.[Citation22–27] In addition, these polymers have recently been reported with both economic and green syntheses.[Citation28,Citation29]
Here, we report a novel and efficient synthesis of the TPD unit facilitating late-stage alkylation by haloalkanes and direct C-H activation polymerisation. Four novel TPD-2T polymers with different branching points in the N−alkyl side-chain are prepared () and their optical (UV–VIS) and thermal (differential scanning calorimetry (DSC)) properties, molecular models and X-ray diffraction (XRD) data presented. The impact on solid-state (thin-film) morphology is then analysed to derive a structure–property relationship.
2. Results and discussion
Synthesis. The dibromo-TPD monomers were prepared in four simple steps from 3,4-thiophene dicarboxylic acid with late-stage alkylation and bromination (). Initially thiophene anhydride 1 is prepared from the dicarboxylic acid according to the literature procedure.[Citation30] Typically a ring-opening condensation with an alkyl-amine and subsequent ring-closure then furnishes the N−alkylated TPD heterocycle. Whilst Leclerc et al. have recently reported an efficient one-step synthesis of TPD this requires the use of alkyl-amines (RNH2), which are not always readily available.[Citation31] We were interested in removing the necessity of alkyl amines from the synthesis of TPD. This would allow access to a greater number of alkyl chains which have been shown to have a significant effect on device performance. To this end we subjected the thiophene anhydride to refluxing formamide, isolating the unalkylated TPD heterocycle 2 from a one-pot reaction in good yield.[Citation32] This novel route does not require prior bromination, which means that alternative polymerisations conditions, such as direct heteroarylations can also be performed.[Citation28,Citation33] Here we proceeded with bromination, albeit non-facile, and subsequent alkylation of amide 3 with haloalkanes.[Citation34] The use of a C1-branched alkyl chain (from 3-bromopentane) results in a lower yield, most likely as a result of increased steric hindrance on alkylation. The C3-branched alkyl chain was installed from 3-ethyl-iodoheptane (6) (), the key step in preparation of which was the one-pot formation of a Grignard reagent from 2-ethyl-bromohexane and subsequent addition to formaldehyde, the latter being prepared in situ by decomposition of paraformaldehyde.
The four TPD-2T polymers were synthesised under standard microwave Stille coupling conditions in chlorobenzene. The polymers were purified by precipitation from methanol followed by Soxhlet extraction using acetone, hexane and finally chloroform. The latter fraction was heated and stirred vigorously with aqueous sodium diethyldithiocarbamate to remove residual catalytic metal impurities. Stille polymerisation whilst versatile and reliable affords number-average molecular weights (Mn) below 10 kDa (), as has been observed with those reported for similar copolymers.[Citation26,Citation29] It may be possible to achieve higher molecular weights by direct heteroarylation polymerisations.[Citation28]
Table 1. Properties of the TPD-2T copolymers.
Optical properties. UV–VIS absorption spectra of the TPD-2T polymers are shown in and key properties summarised in . Dilute solutions were in chlorobenzene and thin-films spin-coated from ∼5 mg/mL chlorobenzene solutions on glass substrates. The polymers all exhibited absorption maximum over 530 nm in thin-films, being assigned to internal charge transfer between the TPD and bithiophene units, while the vibronic shoulders at ∼630 nm imply an ordered arrangement in the solid state. The thin-film maxima are red-shifted by ∼70 nm in comparison with their solutions due to increased π orbital overlap in the planar, conjugated polymers, which reduces the bandgap. The small difference in each of the UV–VIS spectra could be explained by a different morphology in the solid state and/or the different molecular weights. However, the red-shift of the P3 thin-film absoption maximum could be attributed to the reduction in steric bulk of the overall shorter alkyl chain, reducing any distortion of bonds in the pyrrolidone moiety and enhancing any planarising interaction between the carbonyls and neighbouring sulphur atoms of the bithiophene units.
Figure 1. Normalised UV–VIS absorption spectra of the four thieno[3,4-c]pyrrole-4,6-dione-based (TPD-2T) copolymers as (left) solutions in dilute chlorobenzene and (right) thin-films spin-coated on glass substrates from 5 mg/mL hot chlorobenzene solutions.
![Figure 1. Normalised UV–VIS absorption spectra of the four thieno[3,4-c]pyrrole-4,6-dione-based (TPD-2T) copolymers as (left) solutions in dilute chlorobenzene and (right) thin-films spin-coated on glass substrates from 5 mg/mL hot chlorobenzene solutions.](/cms/asset/010306aa-f49e-44a1-9c39-912fb7b97a80/tjos_a_829393_f0001_b.gif)
Energy levels. Ionisation potentials are summarised in , having been measured by Photo Electron Spectroscopy in air using the same polymer thin-film samples as for optical analysis. The TPD-2T polymers all exhibited Highest Occupied Molecular Orbital (HOMO) energy levels of −5.2 eV, confirming the minimal impact of the alkyl side-chain on ionisation potential. In addition, these reasonably low HOMO energy levels (below −5 eV) could lead to a high open-circuit voltage (Voc) in devices and indicate good stability towards unintentional doping by atmospheric oxidants. The TPD-2T polymers all exhibited optical bandgaps of 1.8 eV, with the exception of P3 which was 0.1 eV lower (1.7 eV), attributable to the reduction in overall steric bulk aiding planarisation, as aforementioned, resulting in increased π orbital overlap and delocalisation of electrons stabilising the LUMO; the LUMO levels were found by adding the optical bandgap to the PESA-determined HOMO of the same sample. An optimised structure of the TPD-2T polymer backbone reveals this to be effectively fully planar, possibly as a result of planarising intra-molecular interactions between the carbonyl on the pyrroledione and sulphur on the thiophene moieties[Citation35] (Figure S1, Supplementary Information) (modelled at the B3LYP/6-31G* level, for an N−methyl substituted tetramer). Notably the HOMO and LUMO densities are extensively delocalised along the polymer chain, as opposed to being localised on the D–A parts, respectively, which could facilitate enhanced charge transport.
Thermal properties. The DSC thermograms exhibit well-defined melt and crystallisation peaks () (under a nitrogen atmosphere with a heating rate of 10°C/min). There is a steady increase in melt and crystallisation temperatures as the branch-point of the alkyl side-chain is moved from C3 to C2 to C1. While this could be attributed in part to molecular weight effects, the higher melting points are more indicative of better stability in the crystals as alkyl side-chain bulk is reduced. As expected, P4 which has a linear octyl side-chain – being isomeric with the branched side-chain of P2, and containing one more carbon than that of P3 – sits between the two, demonstrating the presence of both side-chain size and shape effect on polymer properties.
Figure 2. DSC scans of the TPD-2T copolymers exhibit an increase in crystallisation and melt temperatures with a reduction in steric bulk of the alkyl side-chain; (0−350°C temperature range; heating at 10°C/min under a nitrogen atmosphere).
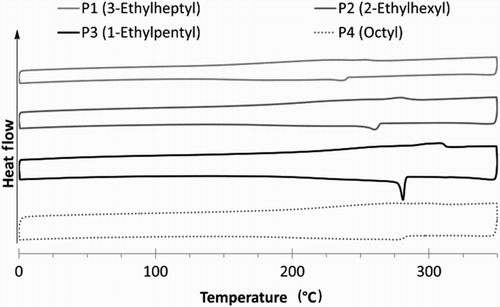
Morphology. Thin-films of the TPD-2T copolymers were investigated by XRD ( and ). Thin-films were drop-cast from polymer solution (10 mg/mL hot chlorobenzene) onto Si substrates and allowed to dry in air. While the P1 and P2 films exhibited an out-of-plane primary reflection peak at 2, this was slightly larger for P3 being
. The low angle diffraction peaks are characteristic of lamellar-type crystallinity in π stacked conjugated polymers, being associable with the interlayer d-spacing distance, calculated to be ∼24 Å for P1 and P2 and ∼22 Å for P3. The reduced lamellar spacing (∼2 Å) for P3 may be attributed to the reduction in steric bulk of the alky side-chains allowing the polymer chains to pack closer together. In addition, P3 appears to exhibit increased crystallinity with higher intensities of the reflection peaks and a discernible quaternary reflection peak, while the tertiary is the highest order reflection peak readily observed for P1 and P2. In common with previous studies, the (100), (200) and (300) diffraction peaks dominate the spectra making indefinite any (010) peak, associable with facial π–π stacking between polymeric backbones.[Citation29] The difference in XRD patterns mean the polymers are organised differently in the solid state and as such the differences in optical absorption spectra and DSC thermograms are unlikely to be attributed to variations in molecular weight.
Figure 3. XRD diagrams of the TPD-2T copolymers exhibit a higher degree of crystallinity with a reduction in steric bulk of the alkyl side-chain; (polymers as drop cast thin-films on Si substrates from 10 mg/mL hot chlorobenzene solutions).
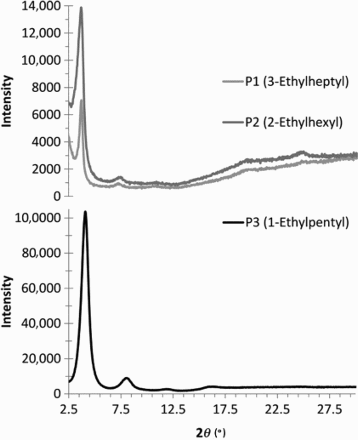
Table 2. XRD data for the TPD-2T copolymers.
The increase in solid-state crystallinity for P3 may be attributed to a reduction in side-chain steric bulk (as opposed to length), promoting crystallisation of the polymer. This has been shown to improve current in devices by strengthening polymer/PCBM interactions and as such the synthesis and device performance of high molecular weight N-1-ethylpentyl alkylated TPD-2T will be of interest.[Citation14,Citation16]
3. Conclusions
In summary, we have described a novel and efficient route to thieno[3,4-c]pyrrole-4,6-dione (TPD) allowing for late-stage alkylation and direct C-H polymerisation. A series of four copolymers with alkylated bithiophene are synthesised, in this case by Stille polymerisation, with different TPD N−alkyl side-chain branching points. The nature of an alkyl side-chain has a minimal effect on the energetics of the polymer, exhibiting greater influence over the morphology. The exact position of a side-chain branch-point (if any) and the length of the chain are two independent factors, with the overall steric bulk induced at the side-chain being responsible for changes in the properties of a polymer series. The use of a C1-branched side-chain promotes increased crystallinity and solid-state packing effects in TPD-2T polymers. To conclude, high molecular weight N-1-ethylpentyl alkylated TPD-2T polymers may exhibit enhanced device performance in organic field-effect transistors and BHJ solar cells.
SupInfo TPD-2T Revised.docx
Download ()Acknowledgements
This work was carried out with funding from Solvay, BASF, SUPERGEN, EC FP7 Project X10D; the Centre for Plastic Electronics at Imperial College London, Doctoral Training Centre EP/G037515/1; the International Collaborative Research Programme of Gyeonggi-do, Korea; EPSRC EP/I002936 and NSF CHE 1026664. The authors declare no competing financial interest.
References
- Pagliaro M, Palmisano G, Ciriminna, R. Flexible solar cells. Weinheim: Wiley-VCH; 2008.
- Zhou H, Yang L, You, W. Rational design of high performance conjugated polymers for organic solar cells. Macromolecules. 2012;45:607–632. doi: 10.1021/ma201648t
- Facchetti, A. π-Conjugated polymers for organic electronics and photovoltaic cell applications. Chem Mater. 2011;23:733–758. doi: 10.1021/cm102419z
- Beaujuge PM, Frechet JMJ. Molecular design and ordering effects in π-functional materials for transistor and solar cell applications. J Am Chem Soc. 2011;133:20009–20029. doi: 10.1021/ja2073643
- Yan H, Chen ZH, Zheng Y, Newman C, Quinn JR, Dotz F, Kastler M, Facchetti A. A high-mobility electron-transporting polymer for printed transistors. Nature. 2009;457:679–686. doi: 10.1038/nature07727
- Skotheim TA, Reynolds JR, editors. Handbook of conducting polymers. 3rd ed. Boca Raton, FL: CRC Press; 2007.
- Lei T, Wang J-Y, Pei J. Roles of flexible chains in organic semiconducting materials. Chem Mater., in press. doi: 10.1021/cm4018776.
- Mayer AC, Toney MF, Scully SR, Rivnay J, Brabec CJ, Scharber M, Koppe M, Heeney M, McCulloch I, McGehee MD. Bimolecular crystals of fullerenes in conjugated polymers and the implications of molecular mixing for solar cells. Adv Funct Mater. 2009;19:1173–1179. doi: 10.1002/adfm.200801684
- Savage RC, Orgiu E, Mativetsky JM, Pisula W, Schnitzler T, Eversloh CL, Li C, Mullen K, Samori, P. Charge transport in fibre-based perylene-diimide transistors: effect of the alkyl substitution and processing technique. Nanoscale. 2012;4:2387–2393. doi: 10.1039/c2nr30088e
- Tsao HN, Cho DM, Park I, Hansen MR, Mavrinkskiy A, Yoon DY, Graf R, Pisula W, Spiess HW, Mullen KJ. Ultrahigh mobility in polymer field-effect transistors by design. J Am Chem Soc. 2011;133:2605–2612. doi: 10.1021/ja108861q
- Kline RJ, Delongchamp DM, Fischer DA, Lin EK, Richter LJ, Chabinyc M, Toney MF, Heeney M, McCulloch I. Critical role of side-chain attachment density on the order and device performance of polythiophenes. Macromolecules. 2007;40:7960–7965. doi: 10.1021/ma0709001
- Cabanetos C, El Labban A, Bartelt JA, Douglas JD, Mateker WR, Frechet JMJ, McGehee MD, Beaujuge PM. Linear side chains in benzo[1,2-b:4,5-b′]dithiophene–thieno[3,4-c]pyrrole-4,6-dione polymers direct self-assembly and solar cell performance. J Am Chem Soc. 2013;135:4656–4659. doi: 10.1021/ja400365b
- Meager I, Ashraf RS, Rossbauer S, Bronstein H, Donaghey JE, Marshall J, Schroeder BC, Heeney M, Anthopoulos TD, McCulloch, I. Alkyl chain extension as a route to novel thieno[3,2-b]thiophene flanked diketopyrrolopyrrole polymers for use in organic solar cells and field effect transistors. Macromolecules, in press, doi: 10.1021/ma401128s.
- Yang LQ, Zhou HX, You WJ. Quantitatively analyzing the influence of side chains on photovoltaic properties of polymer-fullerene solar cells. Phys Chem C. 2010;114:16793–16800. doi: 10.1021/jp106640r
- Bronstein H, Leem DS, Hamilton R, Woebkenberg P, King S, Zhang W, Ashraf RS, Heeney M, Anthopoulos TD, de Mello J, McCulloch I. Indacenodithiophene-co-benzothiadiazole copolymers for high performance solar cells or transistors via alkyl chain optimization. Macromolecules. 2011;44:6649–6652. doi: 10.1021/ma201158d
- Piliego C, Holcombe TW, Douglas JD, Woo CH, Beaujuge PM, Frechet JMJ. Synthetic control of structural order in N-alkylthieno[3,4-c]pyrrole-4,6-dione-based polymers for efficient solar cells. J Am Chem Soc. 2010;132:7595–7597. doi: 10.1021/ja103275u
- Yiu AT, Beaujuge PM, Lee OP, Woo CH, Toney MF, Frechet JMJ. Side-chain tunability of furan-containing low-band-gap polymers provides control of structural order in efficient solar cells. J Am Chem Soc. 2011;134:2180–2185. doi: 10.1021/ja2089662
- Meager I, Ashraf RS, Mollinger S, Schroeder BC, Bronstein H, Beatrup D, Vezie MS, Kirchartz T, Salleo A, Nelson J, McCulloch I. Photocurrent enhancement from diketopyrrolopyrrole polymer solar cells through alkyl-chain branching point manipulation. J Am Chem Soc., in press, doi: 10.1021/ja406934j.
- Zhang F, Hu Y, Schuettfort T, Di C, Gao X, McNeill CR, Thomsen L, Mannsfeld SCB, Yuan W, Sirringhaus H, Zhu D. Critical role of alkyl chain branching of organic semiconductors in enabling solution-processed N-channel organic thin-film transistors with mobility of up to 3.50 cm2 V−1 s−1. J Am Chem Soc. 2013;135:2338–2349. doi: 10.1021/ja311469y
- Lei T, Dou JH, Pei J. Influence of alkyl chain branching positions on the hole mobilities of polymer thin-film transistors. Adv Mater. 2012;24:6457–6461. doi: 10.1002/adma.201202689
- Guo X, Xin H, Kim FS, Liyanage ADT, Jenekhe SA, Watson MD. Thieno[3,4-c]pyrrole-4,6-dione-based donor–acceptor conjugated polymers for solar cells. Macromolecules. 2011;44:269–277. doi: 10.1021/ma101878w
- Zhang QT, Tour JM. Alternating donor/acceptor repeat units in polythiophenes. Intramolecular charge transfer for reducing band gaps in fully substituted conjugated polymers. J Am Chem Soc. 1998;120:5355–5362. doi: 10.1021/ja972373e
- Pomerantz M, Amarasekara AS. Studies of planar poly(3,4-disubstituted-thiophenes). Synth Met. 2003;135–136:257–258. doi: 10.1016/S0379-6779(02)00670-7
- Nielsen CB, Bjørnholm T. New regiosymmetrical dioxopyr-rolo- and dihydropyrrolo-functionalized polythiophenes. Org Lett. 2004;6:3381–3384. doi: 10.1021/ol048659n
- Pron A, Berrouard P, Leclerc, M. Thieno[3,4-c]pyrrole-4,6-dione-based polymers for optoelectronic applications. Macromol Chem Phys. 2013;214:7–16. doi: 10.1002/macp.201200549
- Su M-S, Kuo C-Y, Yuan M-C, Jeng U-S, Su C-J, Wei K-H. Improving device efficiency of polymer/fullerene bulk heterojunction solar cells through enhanced crystallinity and reduced grain boundaries induced by solvent additives. Adv Mater. 2011;23:3315–3319. doi: 10.1002/adma.201101274
- Guo X, Ortiz RP, Zheng Y, Kim M-G, Zhang S, Hu Y, Lu G, Fachetti A, Marks TJ. Thieno[3,4-c]pyrrole-4,6-dione-based polymer semiconductors: toward high-performance, air-stable organic thin-film transistors. J Am Chem Soc. 2011;133:13685–13697. doi: 10.1021/ja205398u
- Berrouard P, Najari A, Pron A, Gendron D, Morin P-O, Pouliot J-R, Veilleux J, Leclerc M. Synthesis of 5-alkyl[3,4-c]thienopyrrole-4,6-dione-based polymers by direct heteroarylation. Angew Chem Int Ed. 2012;51:2068–2071.
- Yuan M-C, Chiu M-Y, Liu S-P, Chen C-M, Wei K-H. A thieno[3,4-c]pyrrole-4,6-dione-based donor–acceptor polymer exhibiting high crystallinity for photovoltaic applications. Macromolecules. 2010;43:6936–6939. doi: 10.1021/ma101523a
- Cornelis D, Peeters H, Zrig S, Andrioletti B, Rose E, Verbiest T, Koeckelberghs, G. A chiroptical study of chiral Λ- and X- type oligothiophenes toward modelling the interchain interactions of chiral conjugated polymers. Chem Mater. 2008;20:2133–2143. doi: 10.1021/cm702875p
- Berrouard P, Dufresne S, Pron A, Veilleux J, Leclerc M. Low-cost synthesis and physical characterization of thieno[3,4-c]pyrrole-4,6-dione-based polymers. J Org Chem. 2012;77: 8167–8173. doi: 10.1021/jo301512e
- Peng Y, Song G, Qian X. Imidation of cyclic carboxylic anhydrides under microwave irradiation. Synth Comm. 2001;31:1927–1931. doi: 10.1081/SCC-100104345
- Facchetti A, Vaccaro L, Marrocchi A. Semiconducting polymers prepared by direct arylation polycondensation. Angew Chem Int Ed. 2012;51:3520–3523.
- Griffini G, Douglas JD, Piliego C, Holcombe TW, Turri S, Frechet JMJ, Mynar JL. Long-term thermal stability of high-efficiency polymer solar cells based on photocrosslinkable donor-acceptor conjugated polymers. Adv Mater. 2011;23:1660–1664. doi: 10.1002/adma.201004743
- Guo X, Zhou N, Lou SJ, Hennek JW, Ortiz RP, Butler MR, Boudreault P-LT, Strzalka J, Morin P-O, Leclerc M, Navarrete JTL, Ratner MA, Chen LX, Chang RPH, Facchetti A, Marks TJ. Bithiopheneimide–dithienosilole/ dithienogermole copolymers for efficient solar cells: information from structure–property–device performance correlations and comparison to thieno[3,4-c]pyrrole-4,6-dione analogues. J Am Chem Soc. 2012;134:18427–18439. doi: 10.1021/ja3081583