ABSTRACT
Pollution and increasing water demand, especially for agriculture, put severe stress on freshwater sources, and as a result, there is progressive deficit in the global water supply and severe water scarcity is projected in the coming decades. Discharges from domestic, industrial and agricultural activities are potential sources of water pollution, impacting human and environmental health. In the face of growing water scarcity and droughts, coupled with the increasing water demand for irrigation, integration of high water-volume and nutrient-rich industrial effluents, into the existing water management plans for agriculture, could play an important role in tackling the problem of water scarcity. However, there is a gap in knowledge about integration of industrial effluents to sewage treatments and the reuse potential of biologically treated mixed industrial and domestic wastewater in agriculture. This study, therefore, provides a critical review on biological treatment of industrial effluents, including petroleum, textile and pharmaceutical wastewater to better understand the capability of bioprocesses and conditions for efficient degradation of pollutants. The effectiveness of activated sludge-based processes, for the treatment of mixed industrial and domestic wastewater, was critically examined, and biomass acclimation plays a vital role in enhanced biodegradation performance. Finally, the reuse potential of mixed industrial and domestic wastewaters for crop irrigation was assessed by studying the reuse outcomes in different cases where industrial effluents were utilized for crop production. Management practices, such as cultivation of salt- and metal-tolerant crops, blending and dilution of industrial wastewater with freshwater and sewage, could make industrial effluents valuable for irrigation.
1. Introduction
Global water resources are under increasing pressure from pollution, rapidly growing demands and climate change. With the overwhelming population growth, rapid industrial development, haphazard urbanization and agricultural expansion, freshwater resources are severely impacted, leading to serious deterioration in quality and global water scarcity [Citation1,Citation2]. The release of untreated industrial and municipal wastewaters, from expanding anthropogenic activities into the environment, not only generates physical, chemical and biological pollution that poses ecological and health risks, but also creates serious global freshwater scarcity. Water pollution and scarcity are among the leading challenges facing mankind that require urgent solutions. Wastewater treatment and reuse, particularly for irrigated agriculture, which is rated the largest consumer of available freshwater provides a long-term sustainable solution to water scarcity and water pollution issues across the globe.
The reuse of wastewater in irrigated agriculture is gaining huge popularity as an alternative source of water and nutrients for food production, and it is expected to increase significantly especially in water-drought regions and countries where access to freshwater is declining due to pollution. In the records of wastewater application in agriculture, the majority of the reuse water originates from domestic and municipal sources, while industrial effluents are classified unsuitable for irrigation due to their known chemical origin which unfairly undermines their water-volume and nutrient-rich potential for agriculture. Cost of treatment and public health concerns are constraints for the adaptation of industrial wastewater to irrigated agriculture. To implement industrial wastewater reuse in agriculture, adequate and effective treatment is required to adjust the composition of industrial effluents to obtain approved water quality for irrigation. Mixing of industrial wastewater with domestic sewage dilutes the concentration of recalcitrant and toxic compounds in industrial effluents and enhances their biodegradability amenable to resource-efficient and cost-effective microbial degradation. However, in the developing countries where only 8% of wastewater generated receives some form of treatment before discharge [Citation3] due to serious lack of sewerage facilities and treatment infrastructures, unethical disposal of untreated domestic and industrial wastewaters into the environment has remained the norm, thus creating a complex wastewater matrix. Industrial wastewater mixtures of common occurrence in the developing countries could consist of, but not limited to, effluents from oil refinery and petrochemical industry, textile mill and pharmaceutical operation.
In the face of growing water scarcity, treatment and reuse of mixed industrial effluents and domestic sewage, which represents the scenario in the developing countries, is an emerging area with high research interest. The physico-chemical treatment methods, such as the advanced oxidation processes (AOPs) [Citation4], carbon adsorption and membrane filtration [Citation5], are efficient for industrial wastewater reclamation for reuse purposes; however, they are generally cost prohibitive owing to the relatively high energy, chemical and material requirements. Biological processes, utilizing the biodegradation capability of different microbial strains, are robust, highly efficient, relatively economical and eco-friendly [Citation6]. Activated sludge-based processes, otherwise referred to as suspended growth biological processes (SGBPs), have been extensively studied for the treatment of industrial effluents and degradation of recalcitrant industrial compounds, and by adequate biomass acclimation, promising results have been reported [Citation7,Citation8]. However, there is a lack of knowledge about the effectiveness of activated sludge-based techniques for the treatment of mixture of industrial effluents with domestic sewage. This is because most studies are focused on biodegradation of individual industrial effluents rather than in complex mixtures, and as a result, there is limited literature on this topic. For this study, three industrial effluents, including petroleum refinery, textile and pharmaceutical, were considered to depict a complex wastewater mixture as would be obtainable in the developing countries. Considering the pressure on water resources from wastewater discharge issues in developing countries and increasing water demand which are likely to intensify, reusing mixed industrial and domestic sewage for agricultural irrigation could potentially contribute considerably to improve the sustainability of irrigated agriculture especially in developing countries and water-drought regions, and enhanced access to freshwater supplies.
Therefore, this structured review paper aims to provide a critical review of the potential of mixture of industrial effluents with domestic sewage for agricultural irrigation. Due to the limited literature on the topic, individual industrial effluents, including petroleum refinery, textile mill and pharmaceutical effluents, were first reviewed with respect to water consumption, effluent generation and characteristics to provide a glimpse on the complexity of industrial effluents mixtures, followed by a review on the characteristics and quality of mixed industrial wastewaters from the available literature. The treatment of the individual industrial effluents and domestic/municipal sewage, by activated sludge-based techniques, was critically examined to identify and better understand process conditions and factors that are critical for efficient biodegradation of pollutants in the respective wastewaters. Finally, the potential for reuse of mixed industrial effluents and domestic sewage in irrigated agriculture after biological treatments was assessed by critical review of the literature where activated sludge-based processes were used to treat individual/mixed industrial and complex wastewaters and where such treated wastewaters were reused for irrigation.
2. Mixed wastewater: wastewater generation and characteristic
Mixed wastewater is a heterogeneous mixture of waste streams emanating from various human activities, including industrial operations, domestic activities, and agricultural operations. Most of these liquid wastes, especially the industrial discharges, contain substances that are characterized as toxic and bio-recalcitrant, leading to their classification as persistent organic compounds (POCs) of environmental concern. As industries continue to proliferate rapidly along with population growth, the release of hazardous chemicals and refractory organic compounds into the environment is increasing substantially with alarming threat to public health and aquatic ecosystems. Some of the compounds and elements with priority concern for their potential public health threat include hydrocarbons, phenolic compounds and heavy metals [Citation9], pesticides [Citation10], chlorinated organic compounds and dyes with aromatic ring structures [Citation11,Citation12]. Industries, identified as potential sources of POCs and emerging pollutants, include but not limited to, oil refinery and petrochemicals, textile mill, metallurgical, rubber and plastics, pharmaceutical, tanning industry, pulp and paper board, chemical and paint facilities [Citation13–15].
While there are many industries, whose effluents present serious threats to the public and environment, this review focuses on petroleum refinery effluent, textile mill wastewater and pharmaceutical stream discharge. Knowing that there are very little publications on mixed wastewater in general, and perhaps with none addressing the specific mixed wastewater scenario considered for this study, the individual industries are briefly discussed in relation to water consumption, effluent generation and wastewater characteristics to provide a glimpse about the complexity of their mixture. Giving the limited publications on this topic, industrial wastewater mixtures, containing at least one of petroleum, textile and pharmaceutical effluents and outside the three industries, are considered for the mixed wastewater scenario.
2.1. Petroleum refinery effluent
The petroleum refinery industry consumes a huge amount of water in crude oil refining and other oil-related operations, and consequently, a large volume of liquid wastes is generated. Specifically, crude oil refining consumes nearly 100 gallons of water in processing a barrel of crude oil, and generates about 1.6 times the volume of crude refined as liquid wastes [Citation16,Citation17]. Effluent from oil refinery has several origins, including streams from desalting operation, distillation, thermal cracking, catalytic and hydro-treatment processes, and cooling units [Citation18]. The chemical composition of petroleum refinery effluent may vary considerably depending on crude oil characteristics, plant configuration and process designs, chemical treatments applied, and which units are in operation at any given time [Citation18]. However, in general petroleum refinery effluent is characterized by high organic loads ranging between 3600 and 5300 mgCOD/L [Citation16], and this is as a result of its enrichment in hydrocarbons, phenolic compounds, emulsified oil and grease, sulphides, ammonia, cyanides and other dissolved substances [Citation16,Citation19]. Effluents from oil refinery operations also contain a substantial amount of heavy metals (HMs), adding to the complexity of the waste streams. The characteristics and composition of a typical petroleum refinery effluent are presented in .
Table 1. Characteristics and composition of petroleum refinery, textile, pharmaceutical and domestic wastewaters.
2.2. Textile mill effluent
Textile manufacturing industry uses large amounts of dyes, water and a range of chemicals in the production of different fabrics; and about 20% of the dyestuffs that remained unfixed are washed into the effluent [Citation35]. On global scale, nearly 3.0 × 105 tons of textile dyes are discharged in textile industrial wastewater yearly [Citation36]. Different processes, such as sizing, desizing, bleaching, mercerizing, dyeing and printing, are involved in the production of textile products, and each stage consumes a large volume of water, and different chemicals, such as acids, alkalis, surfactants, hydrogen peroxides, dispersing agents, are utilized [Citation37]. An estimated 0.2 m3 of water is consumed in processing one kg of fabric in the textile manufacturing industry [Citation37]. Consequently, textile manufacturing generates a large volume of liquid wastes characterized as complex mixtures of dyes and a wide range of chemicals, organic compounds and HMs, which are potentially toxic to the environment and humans [Citation37]. The characteristics of textile wastewater, reported in the scientific literature, are presented in . Most of the dyestuffs used in textile mills today are synthetic as they offer brighter shades and a wider range of colours. These synthetic dyes have aromatic structures, and as a result, they are biologically stable, and tend to bioaccumulate in the environment [Citation12]. Some of the chemical compounds in textile effluents with potential health hazards include amines (from azo dyes), sulphur, chromium, naphthol, acids, hypochlorite, formaldehyde, organic solvents, heavy metals and auxiliary chemicals [Citation38].
2.3. Pharmaceutical effluent
Pharmaceutical industries are engaged in the production of a wide range of pharmaceuticals and personal care products (PPCPs) formulated for the treatment or prevention of diseases and illness for general well-being of humans and animals. Similarly, the sector consumes a huge amount of water in their various operations, including production, formulation, processing of PPCPs and washing of manufacturing equipment and general laboratory cleaning. Consequently, a huge volume of wastewater, containing a wide spectrum of pharmaceutical products, is generated [Citation28], and the products end up in the environment due to their bio-resistant properties. The frequent detection and ubiquity of PPCPs in natural and built water environments, including surface water, groundwater, and wastewater treatment plants [Citation39,Citation40] adds up to their characteristic as biologically active organic micropollutants. Pharmaceutical wastewaters are characterized by high organic loads, nutrients, solids, oil and grease, and residual pharmaceutical products and micropollutants [Citation27]. presents the characteristics of a typical pharmaceutical wastewater, as reported in different scientific literature.
2.4. Domestic sewage
Domestic wastewater (sewage) is household-generated liquid wastes, which include blackwater (mainly urine and faeces) and greywater (kitchen, bath and laundry) [Citation41]. It is characterized by high levels of organic compounds, nutrients, solid materials, and fat and oil, as presented in . Household wastewater may contain some trace metals, such as cadmium, lead, chromium, copper, zinc, and iron [Citation32], and PPCPs. Domestic wastewater, discharged into the environment via drainage systems or septic tanks, reaches the water environment directly or diffusely, and by seepage, thus resulting in eutrophication of receiving water bodies, oxygen depletion, water quality impairment and groundwater pollution.
2.5. Mixed industrial effluents and domestic sewage
Considering the characteristics of the individual industrial effluents presented in , a mixture of industrial waste streams with domestic sewage, although it will allow dilution of active and toxic substances of industrial wastewaters, will generate a complex matrix with treatment difficulties for biodegradation [Citation42,Citation43]. Mixed wastewater generation is more of a characteristic of the developing countries where sewerage facilities and wastewater treatment infrastructures are in serious lack due to financial constraints. However, the detection of toxic organic compounds of industrial origin, such as polycyclic aromatic hydrocarbons (PAHs), polychlorinated biphenyls (PCBs) and pesticides in sewage and sewage sludges generated in the United Kingdom [Citation44] and USA [Citation45], is an indication of industrial wastewater infiltration into municipal sewers, thus highlighting the extend mixed wastewater generation is practised across the globe. According to [Citation13], about half of 10,200 m3/d industrial wastewater generated in Jorden is released to public sewers and the industries, including food industries, textile, metal and chemical enterprises, oil refinery and power plant produce toxic substances that have serious hazardous implications on the environment [Citation13]. Similarly, the expansion of industries in Eastern China has put enormous pressure on municipal wastewater treatment plants initially designed to treat organic and nutrients-laden domestic wastewater, to begin to receive effluents from industries, such as paper making, tanning, printing and dying that have the potential to upset the biological process [Citation42]. Although this shift in practice allows only pre-treated wastewater, the influents are generally poorly biodegradable with less than 0.1 biodegradability.
Although mixing industrial wastewaters with domestic sewage may provide some degree of dilution to the toxic compounds in industrial streams, treatments by biodegradation will be difficult because of the complexity of potentially toxic compounds that can interfere with the effectiveness of the treatment system. A mixed industrial wastewater with domestic sewage may be characterized as totally recalcitrant and non-biodegradable or poorly biodegradable, depending on the composition and proportion of domestic and industrial components in the matrix. The composition and characteristics of mixed industrial effluents and domestic sewage are presented in . The table reveals that mixed wastewaters are characterized by nutrients and a remarkable number of hazardous materials, including complex organic compounds, heavy metals, salts, and oil and grease, which vary depending on industrial wastewater sources. In general, a greater percentage of industrial chemical compounds is biologically stable and they tend to bioaccumulate in the environment and exert hazardous impacts. Liquid discharges from heavy industrial facilities are characterized by HMs, hazardous chemicals and organic compounds, such as PAHs, phenols, PCBs, polybrominated diphenyl ethers (PBDEs), phthalate esters (PAEs), pesticides, etc. [Citation46,Citation47]. These compounds are toxic, carcinogenic and mutagenic, and have been classified as priority pollutants by the United States Environmental Protection Agency and European Union, and their discharge into publicly operated treatment works is restricted [Citation46,Citation47]. A mixture of industrial waste streams with domestic sewage presents complex composition that poses grave threats to public health and aquatic ecosystems if it is not adequately treated. The release of nutrient-rich wastewater into the environment is the principal cause of algal bloom and eutrophication in rivers, and which adversely impact aquatic habitants [Citation48]. Similarly, discharge of organic-rich wastewater into the environment results in drastic depletion of dissolved oxygen concentration in receiving water bodies with aquatic kill and ecosystem imbalance as the consequences.
Table 2. Characteristics and composition of mixed industrial effluents and domestic sewage.
3. Treatment of mixed industrial effluents and domestic sewage
Technologies available for mixed wastewater treatment include physical and chemical processes, AOPs, biological processes, and integrated systems. Each treatment method offers different pros and cons, thereby leading to a trade-off between efficiency and cost. AOPs have shown good reputation in treating complex wastewater mixtures, but they are both chemical and energy intensive, and as a result, they are economically unattractive. Biological treatments especially the SGBPs are the most preferred and widely used wastewater treatments because they are resource-efficient, robust and cost-effective at least compared with chemical methods [Citation6].
The SGBP can be installed in different variants or application modes, such as sequencing batch reactors (SBRs), continuous stirred-tank reactor (CSTR), step-feed reactors, granular sludge reactors, etc. The mechanism involves the cultivation of diverse microbial populations in aerated and continuously mixed reactors, which, in turn, utilize the organic compounds and pollutants present in wastewater as a source of carbon for growth and cell maintenance; and afterwards are physically separated from the treated wastewater by filtration or clarification. In general, the microorganisms transform both biodegradable and non-biodegradable (by hydrolysis reaction) organic matters as well as nutrients into low energy level and stable by-products, including carbon dioxide, nitrogen, water, and new biomass. By virtue of aeration and mixing, the oxygen requirements for microbial respiration and growth are supplied, adequate dispersion of food is maintained, and organisms are kept in suspension [Citation58]. A schematic illustration of SGBP, employed for wastewater treatment, is presented in . Although the SGBP was originally established and operated for the removal of carbonaceous compounds and nutrients in domestic and municipal wastewaters [Citation59], the system has equally shown great potential for the removal of persistent and recalcitrant industrial pollutants by metabolic and co-metabolic degradation pathways [Citation60,Citation61] and adequate biomass acclimation [Citation7]. However, with the very few literature available, there is gap in knowledge regarding the effectiveness of SGBPs for the treatment of mixture of industrial effluents with domestic sewage. To better understand the process conditions favourable for the efficient removal of recalcitrant industrial compounds and critically appraise the SGBPs’ potential for the treatment of wastewater mixtures intended for reuse in agriculture, the performance of SGBPs in treating the individual industrial effluents, including petroleum refinery wastewater, textile mill wastewater and pharmaceutical effluents (for which extensive studies have been conducted) and domestic sewage are critically reviewed and discussed.
3.1. Application of SGBPs in petroleum refinery wastewater treatment
Biological degradation of hydrocarbon compounds in petroleum wastewater has been particularly successful by the synergistic actions of diverse microbial populations. A specific example was a mixed bacterial strains belonging to Pseudomonas, Flavobacterium, Comamonas, Cytophaga, Acidovorax, Sphingomonas, Bacillus and Acinetobacter genera which jointly degraded hydrocarbons in real petroleum wastewater up to 80 and 89% for total hydrocarbons and COD removal, respectively [Citation6]. The record-high degradation efficiencies could be accredited to joint enzymatic activities of the different bacterial cells involved in the process. Biodegradation of hydrocarbons and other substances, in petroleum refinery wastewater with different microbial strains, has been extensively investigated and provides a summary of the findings from few selected studies on biological treatments of petroleum refinery wastewater containing diesel fuel.
Table 3. Performance of SGBPs with specific microbial strains and operating conditions for the treatment of Industrial (petroleum refinery, textile and pharmaceutical) effluents.
The effectiveness of most biological treatment processes, in degrading/removing pollutants in petroleum refinery wastewater, depends on the ability of the microorganisms to withstand both hydraulic and toxic shocks of bio-refractory compounds, such as phenols, benzene, toluene, ethylbenzene, xylenes (BTEX) and other organic and inorganic constituents. Microbial response to shocks was studied using chromium (VI) and low HRT to simulate toxic load and hydraulic shock, respectively, and the results showed a drastic reduction in microbial activity which was accompanied by poor effluent quality [Citation80]. The reduced number of microbial populations was a manifestation of toxicity effect of chromium compound on bacterial cells which suppressed their activity. Secondly, at short hydraulic retention times wash-out of active microbial cells was almost inevitable.
The low biodegradability to non-biodegradability of petroleum hydrocarbons has to do with properties such as solubility and hydrophobicity. Hydrocarbons are generally hydrophobic and less soluble in water, and as a result, they are poorly available for biodegradation by microorganisms [Citation81]. Different approaches amongst them include the use of surfactants that have been employed to increase hydrocarbon-bacteria interactions which could ultimately lead to the availability of more substrates and biodegradation enhancement. Surfactants, commonly cited in the literature for their potency to increase the solubility and biodegradability of total petroleum hydrocarbons (TPH) in wastewater, include rhamnolipid [Citation82], triton X – 100 [Citation65], Igepal CO-630 [Citation81] and saponins [Citation64]. It was added that surfactants have both hydrophobic and hydrophilic properties and are capable of solubilizing hydrophobic compounds and enhance their biodegradability. In a laboratory-scale study by [Citation82], bio-surfactant rhamnolipid was found to enhance the microbial degradation of diesel fuel hydrocarbons. In like manner, triton X–100 not only enhanced the biodegradability of diesel oil, but also catalyzed the biodegradation rate by 10 days earlier, achieving nearly 70% removal against 53% obtained in triton X–100 free conditions [Citation65]. While the authors presumed the surfactants were active emulsifying agents with the characteristics of producing polymeric substances which have the capacity to entrap biomass and enlarge, and subsequently settle rapidly under gravity, surfactants could also reduce the surface tension on hydrocarbons and increase their solubility for possible degradation by microorganisms.
In aerobic biological degradation of hydrocarbons in petroleum wastewater, different factors, such as microbial species and acclimation, available nutrients, dissolved oxygen level, pH, temperature, salinity, surfactants, concentration of contaminant [Citation83], and HRT [Citation84], have been identified to play a crucial role. In the investigation by [Citation84] high HRT resulted in low microbial growth rate and accumulation of old cells, thus, HRT of 20-h was recommended as optimum for biodegradation of petroleum hydrocarbons in wastewater.
3.2. Application of SGBPs in textile dyes’ wastewater treatment
Microbial degradation process is an attractive and resource-efficient alternative for decolourization and breakdown of dyes and recalcitrant compound in textile wastewater, achieved through two principal mechanisms: enzymatic activity and microbial biosorption. Enzymatic degradation has high industrial application for oxidation of a wide range of industrial pollutants and this has led to research to elucidate their capabilities for degradation of dyes and bio-recalcitrant substances in textile wastewater. A variety of active extracellular and intracellular enzymes, produced by diverse categories of microorganisms under favourable environmental conditions, have shown great potential to decolourize and degrade the textile dyes and those commonly cited in the literature include azoreductases, laccases, peroxidases [Citation36,Citation37]. Biosorption on the other hand, which involves uptake of pollutants by microorganisms and inactive biomass, has been explored extensively using biological and microbial systems, chiefly bacteria, fungi and microalgal for the removal of dyes in industrial effluents, and promising results have been reported [Citation85]. In-depth studies on the potential of biological processes, for degradation of dyes and organic constituents in textile wastewater using specific microbial strains, have been conducted and findings from selected literature, especially on methylene blue dye textile wastewater degradation, are summarized in .
Biological treatment of textile effluent has been challenging particularly with wastewater, containing azo dyes known to possess highly stable complex structures, which are resistant to microbial degradation and have toxic properties. While biodegradation of textile dyes depends on their molecular structures, microbial populations and the secreted enzymes as well as other factors, the biodegradation process generally involves two phases: anaerobic and aerobic reaction stages, in sequential order. In the first phase, under anaerobic conditions, cleavage and decolourization of azo dye is realized through reduction and pyrolysis reactions mainly by azo-reductase enzymes, but toxic aromatic amines are produced [Citation86,Citation87]. Under aerobic conditions, the intermediate aromatic amines, which are resistant to anaerobic biodegradation, are further mineralized either completely or partially into inorganic molecules, such as CO2, H2O and NH3, principally by oxidase enzymes secreted by aerobic microbes [Citation86,Citation87].
Partial/incomplete degradation of aromatic amines, under aerobic conditions observed in some studies [Citation88,Citation89], was due to lack of adequate microbial populations with the capability to mineralize intermediate aromatic amines [Citation90]. Besides, considering the toxicity properties of aromatic amines, as confirmed by [Citation89], the activities of aerobic bacteria could be suppressed considerably under the influence of these azo dye intermediates, thus leading to their partial mineralization. However, complete degradation of aromatic amines could be realized by the use of well-acclimated aerobic biomass [Citation88].
Other factors, identified to influence the degradation and decolourization of textile dyes in biological treatment processes, include salinity level, pH values, oxygen transfer rate, dye concentration, structure of dye and solution temperature [Citation37]. High concentration of dyes inhibits microbial activity, enzymatic functions, and biomass growth. The pH and temperature of aqueous solution play a vital role in the affixation of dyes onto textile fibres, thus, understanding their influence in biodegradation of dyes is crucial for efficient decolouration and degradation of dye molecules and recalcitrant chemicals in textile wastewater. Effects of pH and temperature on microbial decolourization of textile dyes have been well examined and the pH range of 3–10 [Citation37] and temperature range of 30–40°C [Citation37,Citation91] have been proposed for efficient bio-decolourization of dyes.
3.3. Application of SGBPs in pharmaceutical wastewater treatment
Removal of pharmaceutical compounds, from wastewater in activated sludge-based processes, can be done by biodegradation, sorption and volatilization. Removal by air stripping or volatilization is almost negligible since the Henry constant for most pharmaceuticals is less than 0.005 [Citation75,Citation92]. The removal pathway is largely a function of the polarity of the compound, implying that highly polar pharmaceuticals are preferentially removed through biodegradation mechanisms (transformation and mineralization) [Citation93], while sorption is the dominant pathway for the removal of non-polar pharmaceutical compounds [Citation92]. Hydrophobic compounds, such as diclofenac, triclocarban, and carbamazepine, have high sorption potential toward the microorganisms, hence their removal in SGBPs is primarily by biosorption, whereas hydrophilic compounds are governed by biodegradation [Citation94]. Several studies have been conducted to assess the potential of SGBPs for the degradation of micropollutants in pharmaceutical wastewater and promising results have been reported especially with respect to compounds, such as analgesic ibuprofen and naproxen, antibiotic Penicillin G, lipid regulator bezafibrate [Citation77,Citation78]. provides a summary of selected literature on the removal efficiency of PPCPs (diclofenac, carbamazepine and penicillin G) in activated sludge-based processes and the operating conditions for their removal.
Biodegradation is the most plausible mechanism for the removal of a vast majority of micropollutants and their metabolites that does not constitute a secondary pollution [Citation95]. Microbial degradation process can lead to complete or partial mineralization of pharmaceutical compounds depending on the microbial communities present, availability of substrates, redox and operating conditions of the treatment system and characteristics of target compounds [Citation96]. Due to the recalcitrant properties of some pharmaceutical compounds, direct metabolization may be very limited and in such conditions, microorganisms tend to utilize the co-metabolization mechanisms in the premise of growth substrates to degrade the persistent trace organic compounds [Citation97]. For instance, bio-persistent compounds, such as carbamazepine and diclofenac, have shown a substantial degree of biodegradability when supplemented with organic substrates [Citation97] such as acetate and glucose. Operating conditions of activated sludge-based processes found to have an important role in biodegradation of micropollutants, including sludge retention time, hydraulic retention time, biomass concentration, redox conditions, pH and temperature of medium [Citation92,Citation95].
Sorption mechanism relates directly to hydrophobicity of the compound which is a measure of octanol water partition coefficient (Kow) having three classifications, namely; Log Kow < 2.5 implies low sorption potential, Log Kow > 2.5 < 4.0 indicates medium sorption potential, and Log Kow > 4.0 represents a high sorption propensity [Citation96]. Similarly, sorption is achieved by electrostatic interactions between the positively charged compounds and the negatively charged surfaces of the biomass [Citation60,Citation92]. Other useful properties for prediction of sorptivity of organic micropollutants in activated sludge-based processes include acid dissociation constant (pKa) and solid-water distribution coefficient (Kd).
Sorptivity of compounds with Log Kd < 2.48 is relatively insignificant, while compounds having Log Kd > 3.2 are readily available for sorption onto biomass [Citation96]. Acidic pharmaceuticals that are negatively charged at neutral pH or that have chlorine atoms like diclofenac and compounds with Kd less than 500 L/kg [Citation92] are not potential candidates for sorption; therefore, biodegradation could be the major removal pathway. Understanding the sorption mechanisms of micropollutants in SGBPs and factors influencing their behaviour is imperative for optimization of wastewater treatment systems.
3.4. Application of SGBPs in domestic/municipal wastewater treatment
Domestic wastewater is characterized by high levels of organic compounds, nutrients, and solids as well as trace amounts of PPCPs, hence, its discharge into the environment without adequate treatments can lead to serious eutrophication in rivers, high organic pollution in receiving water bodies and water quality impairment, thus limiting downstream water uses. Nutrients and organic matters are the primary pollutants of concern in domestic/municipal wastewater and their removal in activated sludge-based processes has been studied quite extensively.
Removal of organic compounds in wastewater is a task for heterotrophic microbes (mainly bacteria and fungi) which utilize the pollutants as source of carbon for energy production (catabolism) and cell synthesis and growth (anabolism) while decomposing/oxidizing the organic materials into carbon dioxide, water and inorganic nutrients [Citation98]. Mineralization of organic material in activated sludge-based processes assumes two distinct pathways: aerobic (principal mechanism) and anaerobic processes. Aerobic processes are commonly employed where higher wastewater treatment efficiency and effluent quality are required. In aerobic conditions, heterotrophic aerobes utilize the free or dissolved oxygen in the oxidation of organic materials to CO2, H2O and biomass while under anaerobic environments (without oxygen) organic pollutants are metabolized into methane, CO2 and H2O via hydrolysis, acidogenesis and methanogenesis [Citation99].
Nutrient (nitrogen and phosphorus) removal in wastewater is pivotal to prevent eutrophication in receiving water bodies and minimize nutrients load to downstream water bodies. Nitrification and denitrification are two sequential conventional processes by which nitrogen is removed from wastewater and released into the atmosphere as nitrogen gas, with a fraction settled in bio-solids. In the first step (nitrification), aerobic autotrophs consume soluble ammonia and carbon in the presence of free or dissolved oxygen to generate nitrate and more nitrifying biomass. Occasionally, nitrification involves two metabolic processes: first is ammonium oxidation to nitrite and second is nitrite oxidation to nitrate, and these processes are catalyzed mainly by ammonium-oxidizing bacteria (AOB) and nitrite-oxidizing bacteria (NOB), respectively. In the second step (denitrification), the heterotrophic microbes under anoxic conditions utilize the nitrates in the solution as source of oxygen (electron acceptor) to produce extra biomass and nitrogen gas [Citation100].
Alternative to the conventional nitrification–denitrification pathway for biological nitrogen removal is anaerobic ammonium oxidation (anammox) process which converts ammonium and nitrite directly to nitrogen gas by anammox bacteria. There are two-step reactions involved in anammox-based processes: partial oxidation of ammonium to nitrite with low DO by AOB, and subsequent oxidation of nitrite to nitrogen gas under anoxic conditions by anammox bacteria such as Planctomycetales [Citation101]. Anammox process has received considerable attention in recent years as an innovative and resource-efficient process considering particularly its relatively minimal oxygen requirements and less sludge production. The process allows a short-cut for nitrogen removal through partial nitrification to nitrite (nitritation) which is then used for anammox activity. In more specific terms, the stoichiometric values for oxygen and carbon requirements and sludge production in anammox process given as 3.43 g-O2/g-N, 1.71 g-COD/g-N and 0.73 g-VSS/g-N, respectively are substantially lower than the corresponding values 4.57 g-O2/g-N, 2.86 g-COD/g-N and 1.19 g-VSS/g-N for conventional nitrification–denitrification pathway [Citation102], adding that anammox process reduces power requirements by 64%, COD demand by 100% and sludge production by 80–90% [Citation102]. For stability and efficiency of anammox process in biological nitrogen removal, conditions that promote nitritation over nitrification, such as enrichment of AOB over NOB, suppression of nitrite oxidation, etc. need to be applied. Like the traditional nitrification–denitrification process, the effectiveness of anammox process depends on operational factors, such as dissolved oxygen level, organic carbon content, substrate concentration, nitrogen loading, temperature and pH condition and sludge retention time [Citation103,Citation104]. Although the conventional biological nitrogen removal was originally developed to take place in two-stage reactors, studies have shown that both the nitrification–denitrification process and anammox process could be operated efficiently in a single-stage reactor by the proper management of dissolve oxygen concentration and selection of appropriate operational conditions [Citation101,Citation105].
Biological phosphorus removal (BPR) is undertaken by bacteria that accumulate phosphorus in excess of their normal metabolic requirements, and these bacteria are generally referred to as polyphosphate accumulating organisms (PAOs). Cycling of microbial biomass and influent wastewater between anaerobic and aerobic reactor zones allows for the proliferation of PAOs with the capacity to accumulate polyphosphates intracellularly in aerobic conditions [Citation106]. Under anaerobic conditions, PAOs take up short chain volatile fatty acids (VFAs) like acetates and store them intracellularly as carbon polymer such as poly-B-hydroxybutyrates (PHAs) to be synthesized in aerobic conditions, and by cleavage of the stored polyphosphate the energy required for the biotransformation is generated and phosphate is released into the bulk liquor [Citation106]. Under aerobic conditions, the PHA is metabolized to drive biomass growth and provide the energy required for uptake of all available orthophosphate and storage of polyphosphate, and excess phosphorus is removed from the bulk liquid by wasting polyphosphate-rich sludge [Citation106,Citation107]. Outside the anaerobic–aerobic operation that serves as the major pathway for the removal of phosphorus in enhanced biological phosphorus removal (EBPR), some PAOs are capable of utilizing nitrate or nitrite as electron acceptor instead of oxygen for uptake of phosphorus from the bulk liquid, thus allowing for simultaneous phosphorus removal and denitrification [Citation108]. Like the nitrogen, phosphorus removal can be achieved in a single-stage reactor by providing the enabling environment for efficient operation of specialized microbial populations [Citation106,Citation108].
3.5. Treatment of mixed industrial effluents and domestic sewage by SGBPs
Treatment of mixture of industrial effluents and domestic sewage is an interesting area with high research need, yet it has remained sparsely studied over the years. Therefore, there is relatively very little literature available on the treatment of mixed wastewater by SGBPs. While there are challenges with mixed wastewater treatment practice, some studies have reported considerable breakthrough.
Industrial wastewaters, especially those from chemical-based operations, contain compounds that are toxic and inhibitory to microbial activity [Citation8], and amongst them include chromium, sulphides, phenolics, PAHs, cyanides, etc. Mixing industrial effluents together creates a more complex composition that can easily hamper the oxidation process of biological treatment units. Industrial contaminants interact in complex ways that could drastically change the pH of wastewater to extreme acidic or alkaline conditions making it incompatible with sewage treatment [Citation109,Citation110]. To ascertain treatment compatibility and avoid undue upset of sewage treatment plants, a comprehensive analysis of the industrial wastewater is strongly required. However, of particular concern is the temporal and spatial variability of industrial wastewater, as regards to flow and chemical composition, due to changes in manufacturing products, transitory operation of industrial plants, cleaning and washing, etc., which cause great fluctuations in the characteristics of inlet (influent) wastewater to sewage treatment unit, and consequently may result in abrupt failure of biological process [Citation111]. The high degree of uncertainties, pH fluctuation and variabilities in industrial wastewater characteristics can potentially lead to loss of genetic ability of competent microbial communities already developed through biomass acclimation [Citation112], thus, leading to inefficient biodegradation. However, unit operations, such as equalization and neutralization, are of huge practical importance in industrial wastewater treatment by biological process. The toxicants in industrial wastewaters have negative impact on biomass population, particularly the nitrifying bacteria, which are naturally slow growers that require a longer solids retention time (SRT) over their heterotrophic counterparts [Citation8]. Depending on the origin, industrial effluents unleash acute toxicity and/or lethal effects on microbial communities, which can potentially lead to loss microbial diversity, loss of nitrification capacity, and impair the functioning of biological treatment process.
However, studies have shown that biomass, including nitrifying bacteria, could be engineered/nurtured to develop resistance to toxic substances through microbial adaptations, and consolidate their oxidation reactions/activities for the degradation of recalcitrant organic compounds and nitrification [Citation7,Citation8,Citation109]. Specifically, in the recent study by Zou and co-workers, it emerged that after acclimation to phenol, the biomass became enriched with bacterial populations having phenol-degrading traits and good nitrification activity, thus, achieving high phenol removal and nitrification capacity when utilized in phenol biodegradation studies [Citation7]. It was further highlighted that the adaptation mechanism not only enhanced phenol degradation/removal, but also increased the rate of degradation with total phenol removal in 1.2-h, and by this strategy, nitrifying bacteria were shielded from toxic inhibition [Citation7]. Noteworthy, phenol degradation by the acclimatized biomass was accredited to Burkholderiaceae, Anaerolineaceae, Rhodocyclaceae and Cyanobacteria bacterial strains [Citation7]. In addition to direct phenol impact on nitrifying biomass, [Citation109] highlighted that full-scale nitrification can be inhibited through indirect mechanism of oxygen depletion due to the fast growth of heterotrophic bacteria with phenol degrading traits. Similarly, the crucial role of biomass adaptation in industrial/complex wastewater treatment by biological process was also confirmed in the work by [Citation8] where pharmaceutical/chemical wastewater mixed with municipal sewage was treated effectively with stable nitrification activity up to 40% industrial contribution to treatment plant inflow. Increasing the industrial flow share resulted in nitrification breakdown and which, however, was recovered after prolonged adaptation of nitrifying bacteria. Furthermore, in a recent study by [Citation113] diversified bacteria populations, sourced from municipal wastewater treatment plant, were assessed for the potential to treat simulated mixed industrial and domestic wastewater. Findings indicate that the mechanism of long-term biomass adaptation played a crucial role in development and multiplication of competent bacteria populations and acquisition of new genetic properties capable of degrading complex organic compounds of petroleum, textile and pharmaceutical origins in the mixed wastewater up to 92.1% reduction measured as COD, while maintaining stable nitrification (about 65%) [Citation114]. Considering the high degree of treatment efficiencies achieved through the adoption of certain process conditions that enhance the metabolization of degradation-resistant organics and huge economic benefits with biological treatment technologies, SGBPs could serve as potential treatments for the mixture of industrial effluents and domestic sewage.
In other studies, SGBPs showed promise for the treatment of various complex wastewater mixtures including mixture of industrial effluents and domestic sewage. In the mid-1980s, Nesaratnam and co-worker investigated the effectiveness of activated sludge system for the treatment of combined industrial effluents originating from oil refinery and petrochemical plants and domestic wastewater [Citation55]. According to the study, activated sludge system has a great potential for simultaneous carbon and ammonium removal as well as removal of the industrial pollutants such as chromium, hydrogen sulphide, phenol, and cyanide [Citation55]. It is important to note the toxic compounds only exerted temporal inhibition on nitrifying biomass, but over time the microbial populations recovered and became fully adapted to the pollutants and consequently, a stable nitrification activity was sustained. That is not all, the authors also cited a number of studies conducted before the mid-1980s where activated sludge process was successfully employed for the treatment of mixed industrial and domestic wastewater through strict control of the proportion of toxic compounds discharged into the sewers [Citation55]. SBR, operated under periodic exposure of biomass to set process conditions, was used effectively to treat complex mixture of wastewater from industries producing a variety of chemicals, drugs, pharmaceuticals and pesticides [Citation111]. Authors laid particular emphasis on the loading rate of industrial complexes as a key factor upon which the biological oxidation process depends, and that biodegradation performance was actively maintained at organic loading of 1.7 kgCOD/m3/day but became inhibited at 3.5 kgCOD/m3/day loading of complex organics [Citation111]. The results clearly indicate that microbial populations once fully adapted to set environmental conditions they develop a sort of immunity for the selected pressures and become capable of metabolizing the bio-resistant compounds already adapted to providing their stress history is not exceeded considerably.
Addition of domestic effluents and/or wastes from food manufacturing to industrial wastewaters provides the heterotrophic organisms a background rich in assimilable carbon sources to sustain and support their growth and metabolic functions and which, in extension, enhances the biodegradability of recalcitrant and/or inhibitory organic pollutants in industrial waste streams [Citation115–117]. Industrial effluents, in particular, those originating from chemical-based industries are rich in degradation-resistant organics, and their mixture with domestic (carbonaceous-rich) wastewater for treatment by activated sludge-based processes is a promising approach to efficiently and economically treat complex industrial liquid discharges that may otherwise attract huge treatment costs [Citation8,Citation116]. However, emphasis was made on volume ratio of the mixture as it plays a crucial role in toxic compound assimilation in domestic sewage [Citation54,Citation110]. The appropriate volume ratio of industrial and domestic wastewater in a single mixture for efficient biodegradation varies, mainly due to the characteristics and composition of the industrial streams. There are a number of cases where this treatment practice has been carried out with successful outcomes. In Singapore, mixed sewage at a volume ratio of 60% industrial waste to 40% domestic waste was treated efficiently by activated sludge process [Citation56]. Similarly, in Pistoia, Italy, a municipal wastewater treatment plant with configuration: primary grit and oil removal, chemically enhanced primary sedimentation and a conventional nitrification–denitrification activated-sludge process collects and efficiently treats mixed industrial and domestic wastewater at a ratio of 70% textile wastewater to 30% domestic sewage (Gori et al., [Citation118]). A volume ratio of 1:2 petroleum wastewater to brewery wastewater was revealed to provide the perfect mixture for efficient treatment in fluidized bed reactor [Citation116]. A volume mixture of 40% industrial (pharmaceutical and chemical) effluent to 60% municipal wastewater was efficiently treated using a pilot-scale MBR with good nitrification performance [Citation8]. The complex organic constituents of industrial wastewater mixture from petrochemical and cosmetic manufacturing industries with >80% microbial inhibition were effectively degraded in SBR utilizing acclimated sludge biomass (activated sludge) at a dilution ratio of 1:5 to urban wastewater [Citation119]. With the integration of hydrolysis acidification process to SBR, a higher proportion (>60%) of mixed industrial effluents from papermaking, tanning, printing and dyeing industries was efficiently treated in a mixture with domestic sewage [Citation42]. The hydrolysis acidification process provided conditions for anaerobic digestion of inhibitory organics which improved the biodegradation kinetics of bio-recalcitrant compounds in the industrial effluents [Citation42]. From the various literature reviewed, treatment of complex industrial wastewater mixtures is practically feasible by adopting the mechanisms of biomass adaptation and dilution with urban sewage at a ratio that allows for good assimilation for microbial degradation. The dilution ratio is majorly dependent on the composition and concentration of toxicants in industrial wastewater.
Given that removal of bio-recalcitrant pollutants in biological treatments is not necessarily an indicative of toxicity abatement [Citation112], the performance of biological treatments needs to be assessed on the basis of possible generation of harmful by-products during treatment by performing relevant toxicity tests tailored to treatment goal. In this view, a genotoxicity test, conducted on Salmonella using raw and biologically treated mixed complex wastewater (hospital, industrial and domestic), revealed that as much as the genotoxicity risks confirmed in the raw wastewater, none was identified under the treated wastewater assay [Citation120]. While the efficient treatment performance may not represent various wastewater conditions, the biomass acclimation mechanism has proved to be an integral operating condition in the biological treatment of complex wastewaters. The few available literature, specifically on biological treatment of real mixed industrial effluents and domestic/nutrients-rich, wastewater are presented in . Based on the treatment outcomes, it could be affirmed that prior acclimation of biomass to bio-recalcitrant pollutants in complex wastewater mixtures presents promising process condition and strategy for biodegradation of toxic and refractory organic pollutants in industrial and complex wastewater mixtures [Citation7]. From both the specific applications of biological processes in mixed industrial and domestic wastewater as well as the individual industrial effluents, there is positive indication that SGBPs might have the potential and capability to improve the quality of complex mixed wastewater considerably. Meanwhile, considering the relatively short-term biomass acclimation conditions and a rather rapid biodegradation assay is commonly used/adopted for the estimation of treatment capacity of suspended growth processes, there is a likelihood of underestimation of the real potential of biological processes for the treatment of industrial effluents. Hence, longer biomass adaptation is most appropriate for activated sludge-based processes receiving industrial wastewater for efficient degradation of bio-recalcitrant industrial compounds.
Table 4. Performance of SGBPs for the treatment of real mixed industrial and domestic wastewater.
4. Wastewater reuse in agriculture
Water scarcity is a widespread problem that attracts great concern. Agriculture is identified as the major culprit of this problem as it accounts for 70% of all freshwater withdrawals globally [Citation121]. According to [Citation121], agricultural operations have increased by more than 100% in the past three decades in response to economic and demographic growths, and about 60% increase in food demand is predicted by 2050 with 10% increase in freshwater demand for irrigation. Moreover, with the estimated 5 cubic metres of water required in production of per head daily food coupled with the current pace in population growth and industrial expansion, freshwater scarcity is bound to attain alarming thresholds, especially in regions with low annual rainfall [Citation1]. Wastewater reuse in agriculture is a water resources management measure which is not limited to addressing water scarcity and quality deterioration problems but serves as supplemental nutrient source for increased and sustainable food production for the teeming population at reduced costs.
For the safe use of wastewater in agriculture, guidelines have been established at international, regional and national levels to ensure proper management and administration of reclaimed water and gain public acceptance of the produce. While some of the water reuse criteria like the California guidelines are stricter, the United States Environmental Protection Agency (US EPA) and the World Health Organisation (WHO) standards were established to accommodate possible water reuse in low-income countries, and are used as references by many countries. In general, these criteria, especially the WHO guidelines, focused mainly on the microbial risks of the reuse water, distinguishing between different classes of water reuse in agriculture with particular reference to crop types, effluent quality, applied treatment technology and irrigation methods [Citation122]. The WHO water reuse guidelines have been adapted to specific regions with local epidemiological, sociocultural and environmental factors taken into account. The most commonly used guidelines, for water reuse in agriculture and water quality for irrigation, are presented in .
Table 5. Agricultural water reuse criteria and guidelines for the interpretation of water quality for irrigation.
Wastewater reuse in agriculture is gaining significant traction and the well-established guidelines that exist particularly in developed countries are critical for regulation of the reuse practice. The non-existence of guidelines or lax regulations on water reuse in most developing countries hampers the key objectives of water reuse. However, to the best of current knowledge, there are no guidelines for emerging contaminants such as endocrine disrupting compounds (EDCs), PPCPs, PAHs, etc. With the current global water scarcity crises coupled with freshwater resources pollution, extensive reuse of both domestic and industrial wastewater in agriculture could serve as a sustainable solution to the freshwater problems. The mixing of industrial liquid wastes with municipal effluents which is prevailing in many cities especially in developing countries would amount to definite application of industrial wastewater in crop irrigation as water reuse in agriculture is implemented across the globe. Therefore, future integration of industrial wastewater into water reuse in agriculture is an area with huge interest, and for safe, extensive and sustainable water reuse practice in agriculture, future inclusion of emerging contaminants in water reuse criteria is highly recommended.
5. Potential of industrial wastewater for reuse in irrigated agriculture
Wastewater reuse in agricultural irrigation (especially of food crops) merits high consideration due to the increasing water demand from the sector. Agriculture accounts for nearly 70% of all freshwater withdrawals worldwide [Citation121], and 15% additional increase is projected by 2050 [Citation125]. Moreover, the presence of beneficial nutrients and elements in wastewater makes its reuse for crop irrigation lucrative and attractive. However, the majority of the wastewater used in irrigated agriculture is sourced from domestic and municipal waste streams, and a very rare consideration is paid to industrial effluents whose chemical origin always overshadows its nutrient-rich and water-volume potential. Consequently, the possibility of applying industrial wastewaters in agriculture has not been extensively studied and only a few literature are available on this topic. To ascertain the reuse potential of this resource in irrigated agriculture, the experiences and outcomes of irrigation with industrial effluents in various case studies need to be critically evaluated. presents laboratory, greenhouse and field-scale experiments on the use of mixtures of industrial effluents and domestic sewage (where indicated) for irrigation, and provides crucial information relating to the wastewater treatment type applied and quality of irrigation water used. The information in further helps to identify specific challenges faced when industrial wastewater is used for irrigation, best management practices (BMPs) to be adopted for successful reuse practice and assess the potential of biological treatments in improving the quality of mixed industrial and domestic sewage for agriculture.
Table 6. Cases of irrigation of crops with mixed and individual industrial effluents and in a mixture with domestic sewage.
Exploring the possibility and extent of utilization of industrial effluents in agriculture has led to the utilization of different water conditions including untreated, diluted and treated wastewaters for studies on both food and non-food crops. Overall, the studies revealed that with adequate treatments, industrial effluents could serve as alternative source of irrigation water and potential source of plant nutrient (in irrigated agriculture) for sustainable food production, while preserving the environment and water sources. Looking into the specific studies on reuse potential of industrial wastewater for irrigation, [Citation126] found that winter wheat (Triticum aestivum L.) irrigated with biologically treated mixed industrial, municipal and household effluents had better morphological development and produced greater yields than counterparts grown under freshwater irrigation. This is clearly due to the quality of water utilized, which according to the available data, met the FAO water quality criteria for irrigation except for trace metal Cu (). The better growth performance and high yields in crops that received the treated wastewater over the freshwater irrigation could be a function of nutrients’ availability in the reused water which supported the plants for better growth and development. Similarly, textile mill effluent, treated by anoxic-aerobic photobioreactor, was used for irrigation of radish and the results indicate higher dry weight, leaf number and leaf area by 49.0, 19.2 and 62.0%, respectively compared to those that received freshwater irrigation [Citation127]. Again, water quality and nutrient content of the treated wastewater are responsible for the higher biomass yields and growth outcomes in radish. In the study by [Citation128], it was revealed that the growth and yield parameters (such as above and belowground biomass, photosynthetic pigments, leaf area, plant height, leaf number, etc.) in Zea mays irrigated with biologically (UASB – upflow anaerobic sludge blanket) treated mixed textile and domestic wastewater were comparable to the outcomes in control treatment irrigated with freshwater. The authors added that dilution of the treated mixed wastewater further enhanced its productivity for the growth of maize crop.
Dilution of industrial wastewaters, intended for reuse in irrigated agriculture with freshwater, is identified as a good water resource management practice to reduce the prohibitive costs of treatment and health risks in wastewater reuse for irrigation. Blending industrial effluent with freshwater dilutes the concentrations of hazardous substances, heavy metals, salts and decreases their phytotoxic effects to plants [Citation135,Citation147].
Sequential anaerobic and aerobic biological process showed to be the effective treatment for pulp and paper industrial effluent to meet agricultural reuse quality [Citation145].
The reuse potential of the treated wastewater was confirmed following a phytotoxicity assessment performed on mustard plant which had higher germination rate (90%) and produced longer shoot and root elongations under treated wastewater irrigation over tap water treatments [Citation145]. Similarly, it was affirmed in another experimental study [Citation118] that mixed industrial (textile) and domestic could serve as a suitable source of irrigation water for ornament plants following the plants’ excellent growth performance and outcomes, although the reuse effluent was further treated by sand filtration and disinfection. Recently, biologically treated reactive textile wastewater was applied for irrigation of wheat crop and the yield and overall outcomes was excellent and high nutritional values with respect to protein, carbohydrates, and energy were recorded in the grains [Citation143]. Paper and pulp industrial wastewater, known to contain organic halides and chlorinated organic compounds, served as a source of irrigation after biological pre-treatment in Jordan [Citation13]. In the review conducted by [Citation148], it was gathered that wastewater from food processing industries was reused for crop irrigation with no compromise on crop quality, higher yields were obtained, and no accumulation of hazardous elements was found in the soil. At 25% effluent concentration, electroplating wastewater was successfully reused for the growth of Hordeum vulgare, and could serve as a potential source of micro-nutrients that are beneficial to plants [Citation133]. Overall, the reuse outcomes of the respective studies were largely dependent on the irrigation water quality, plant tolerance to substances in water and micro- and macro-nutrients availability in the reused water. Based on the available information, the treated mixed wastewater did meet certain criteria in terms of parameters stipulated by the FAO () for the interpretation of water quality for irrigation.
Majority of the literature consulted indicate there is indeed positive indication for the reuse of treated industrial/mixed wastewater in agriculture; however, issues, such as salinity problems, plant uptake of heavy metals and toxicity, were reported in some cases. Salinity is a common problem in irrigated agriculture, especially where wastewater is reused. Depending on the source, industrial effluents contain certain salts in certain amounts. Irrigation (long term) with water containing considerable amount of salts can lead to soil salinity and sodicity to levels that are detrimental to crop and soil health, even on a short term [Citation149]. Similarly, industrial wastewaters contain varying concentrations of heavy metals which accumulate in the soil and eventually migrate into plants and could potentially end up in the food chain, posing a threat to public health. Like salts, heavy metals are not biodegradable, and as a result, biological treatments are not suitable for their removal in wastewater, hence, specialized treatments are required to get rid of them. Growing of crops with high resistance to metal contamination and salt tolerance are recommended BMPs to address the specific problems of salinity and heavy metal accumulation in industrial wastewater irrigated agriculture [Citation144].
The risk of pathogenic contamination of farm products is another troubling concern in wastewater irrigated agriculture. The detection of faecal coliforms, faecal enterococci and total heterotrophic bacteria counts in tomatoes and broccoli irrigated with biologically treated agro-industrial effluents [Citation132] is a clear indication of serious food contamination with the likelihood of human exposure to the risk of pathogenic diseases, thus highlighting the need for inactivation of pathogenic bacteria and helminths in biologically treated wastewater prior to irrigation. For this particular reason, WHO recommends a high degree of disinfection be applied after efficient biological stabilization of wastewater to inactivate faecal coliforms, pathogenic bacteria and enteric viruses [Citation150]. However, the proposed treatment is originally applicable to domestic/municipal sewage presumed to be free of organic and inorganic chemicals, recalcitrant organic compounds and heavy metals that are potentially hazardous to human health and the environment. The mode of water application is critical for the safe reuse of wastewater in agriculture, and drip irrigation method is recommended to ensure proper administration of the water to crops while avoiding its direct contact with the edible parts of the crop to eliminate possible route of microbial contamination [Citation132].
The overall assessment of treatment and reuse potential of mixed industrial effluents and domestic sewage treated by activated sludge-based processes is presented in .
Figure 2. Potential of suspended growth biological processes (SGBPs) for the treatment of individual wastewaters for reuse in irrigated agriculture. TPH: total petroleum hydrocarbon; PPCPs: pharmaceuticals and personal care products; MB: methylene blue; LR: low range; PAHs: polycyclic aromatic hydrocarbons.
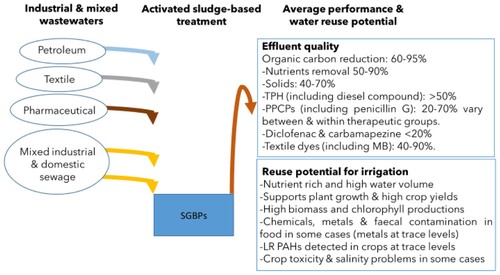
6. Research gaps and future directions: adapting industrial effluents to agriculture or irrigation
As water scarcity continues to intensify across the globe as a result of accelerated population growth, industrial development, agricultural expansion and climate change, the need for a targeted integrated wastewater reuse in agriculture becomes central. Industrial effluent is a valuable resource for irrigated agriculture considering its high water-volume and nutrients-rich properties which have been unfairly undermined by its chemical origin. As a result, industrial wastewater has not been given the needed consideration for reuse in agriculture; therefore, its potential in irrigated agriculture remains unexplored. Reuse or not, industrial wastewater needs to be treated for safe environmental disposal, therefore, adapting industrial wastewater to agriculture will require a little more additional measures in the form of treatment and management practices.
Amongst other factors, cost of treatment and concerns for public health safety are the major constrains for the implementation of industrial wastewater reuse in agriculture, especially in low-income countries. Biological treatments are more economical and resource efficient than physicochemical alternatives and they have shown a great deal of potential for improving the quality of industrial wastewater mixtures. However, some constituents, such as HMs, salts, pharmaceuticals, PAHs, phenolics, amines in industrial wastewater, are generally not biodegradable, hence, irrigation, especially on a long term with water containing these compounds/elements, can lead to soil salinity and sodicity problems, changes in soil structure, loss of soil fertility, problems of toxicity to crops [Citation123,Citation149,Citation151]. Therefore, in order to use industrial wastewater for irrigation sustainably, the quality has to meet the reuse criteria stipulated by local and international regulating organizations such as USEPA, WHO and FAO.
Blending industrial effluents with domestic/municipal is a direction that can break the gridlock surrounding industrial wastewater reuse in agriculture. Mixing with municipal sewage will bring about a substantial degree of dilution of chemicals and toxic substances in industrial wastewater and equally enhance the biodegradability of recalcitrant organic compounds amenable to biological treatments [Citation115]. Another innovative path to adapting industrial wastewater to irrigation already cited in the literature [Citation128] is by dilution of biologically treated effluents with freshwater to generate a more improved water quality that can be used sustainably for irrigation to maintain agricultural production, while water sources and environmental qualities are preserved. However, considering the high salt loads and concentration of heavy metals, some mixed industrial and domestic wastewater cannot be sustainably used for irrigation, and for such scenario, BMPs, such as cultivation of salt-tolerant plants, crops with low capacity for heavy metal uptake, application of soil amendments, etc. are the principal solutions to addressing the problems [Citation149]. Further treatment with efficient and cost-effective systems is another recommended option to ensure a safe reuse of wastewater in agriculture. AOPs are among the alternative treatment technologies that have been proposed for effective treatment of industrial wastewater intended for reuse. Among the AOPs, great attention has been given to ozone oxidation process. Considering the negative implications of using industrial and mixed wastewater for crop irrigation due to water quality issues already discussed, potential treatment options for possible reclamation and reuse of mixed industrial effluents and domestic sewage in agriculture are conceptualized and presented in . The capability of each treatment option to produce effluents that meet water quality parameters categorized into physical, chemical, organic compounds and microbial parameters is illustrated by binary words – yes and no ().
Figure 3. Conceptualization of mixed wastewater treatment options with possible water quality attainments and level of reuse applications for irrigation. SGBPs: suspended growth biological processes; Cl: chlorination; AOPs: advanced oxidation processes. (Water quality parameters, as indicated in : P – physical; C – chemical; O – organic compounds; M – microbial).
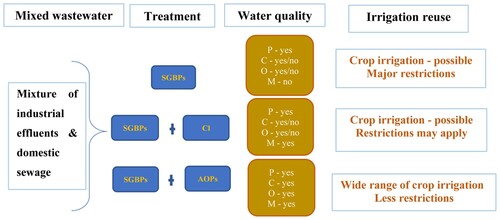
7. Conclusions
Wastewater reused in agriculture is sourced mainly from domestic/municipal sewage, while the industrial streams are unfairly disfranchised due to their chemical origin. Although industrial effluents, depending on the source, may contain hazardous substances, they could be resourceful in irrigated agriculture considering their high water-volume and nutrient-rich potential. Cost of reclamation of industrial effluents and public health concerns are the major constrains for the implementation of industrial wastewater reuse in agriculture. Biological treatment processes are generally resource-efficient and relatively cost-effective for wastewater decontamination. If adequately operated, and by adoption of dilution mechanism and full biomass adaptation, SGBPs have the capability to improve the quality of mixed industrial effluents and domestic sewage for adaptation to irrigated agriculture. Integration of industrial effluents into domestic sewage treatments allows some degree of dilution of toxic substances and provides the biomass the assimilable food and enabling environment required for metabolization and co-metabolization of degradation-resistant industrial pollutants. The volume ratio of domestic/municipal sewage to industrial effluents, which depends primarily on characteristics and composition of industrial streams, is particularly critical in the development of blended mixtures that allow assimilation of toxic and recalcitrant compounds for microbial degradation. Biodegradability of refractory industrial pollutants in activated sludge-based treatments is enhanced through biomass acclimation, allowing for the development and multiplication of diverse competent microbial populations with genetic traits capable of degrading target pollutants.
Based on the reuse outcomes in agriculture and quality of the irrigation water summarized in this paper, SGBPs have the potential to improve the quality of mixed industrial effluents and domestic sewage for application in agriculture. Irrigation with biologically treated mixed industrial effluents and domestic sewage presented some problems, such as plant toxicity, accumulation of heavy metals in plants and agricultural soils and soil salinity; however, cultivation of heavy metal and salt-resistant crops is a recommended management practice to ensure safe and sustainable adaptation of industrial effluents to irrigated agriculture. Blending the biologically treated mixed industrial and domestic wastewater with freshwater could potentially remove the bottlenecks for the extended use of industrial wastewater for irrigation. Integration of supplementary treatments, such as AOPs, into activated sludge-based processes will allow the recovery of high-quality water for wider irrigation reuse applications. With the evidence collected in this review, it can be concluded that SGBPs, if operated optimally, could address the problem of wastewater treatment quality for agricultural reuse or in the very least improve the quality of mixed wastewater considerably for further treatment to meet irrigation reuse quality. However, the level of current knowledge and research data are limited’ therefore, further integrated studies are necessary to fully explore this area and understand the sustainability of irrigation with mixed industrial effluents and domestic sewage.
Acknowledgements
The first author sincerely thanks Petroleum Technology Development Fund (PTDF), Nigeria for providing full financial sponsorship for PhD research at the University of Surrey, Surrey, United Kingdom.
Disclosure statement
No potential conflict of interest was reported by the author(s).
Correction Statement
This article has been republished with minor changes. These changes do not impact the academic content of the article.
Additional information
Funding
Notes on contributors
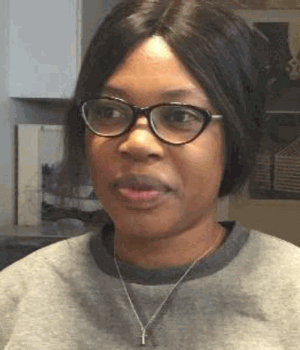
Precious N. Egbuikwem
Precious N. Egbuikwem (PhD Candidate) is Lecturer at the department of Agricultural and Bio-Environmental Engineering, Imo State Polytechnic Umuagwo, Nigeria. She is currently a postgraduate researcher in the Centre for Environmental Health and Engineering (CEHE), department of Civil and Environmental Engineering, University of Surrey, United Kingdom. She is a PhD Scholar of the Petroleum Technology Development Fund (PTDF) Nigeria. Her PhD research is in Environmental Engineering with particular focus on mixed industrial and domestic wastewater treatment using biological, chemical and physical processes; sustainable treatment and reclamation of mixed wastewater and reuse of treated wastewater for crop irrigation under hydroponic conditions.

Gregory C. Obiechefu
Dr. Gregory C. Obiechefu is Deputy Rector (Academics) and Dean, School of Engineering Technology, Imo State Polytechnic Umuagwo, Nigeria. He is a faculty member Agricultural and Bio-environmental Engineering of the Polytechnic delivering quality lectures in Soil and Water Conservation specialization. He is the Chairperson Committee of Deans of Engineering of Polytechnics and Colleges of Technology (COMPODET) of Nigeria. His work involves Soil and Water Engineering, Erosion studies, Irrigation, Evapotranspiration and Wastewater Treatment and Management.

Faisal I. Hai
Hai, Faisal I is Professor in the Faculty of Engineering and Information Sciences, University of Wollongong Australia. He is the director of the Strategic Water Infrastructure Lab (SWIL). He carries out a number of research studies, teaching and governance leadership roles across the Faculty of Engineering and Information Sciences (EIS). He is Head of Postgraduate Studies of the School of Civil, Mining and Environmental Engineering (CME) where he coordinates and supports the research of around 80 higher degree research (HDR) students (PhD, Masters). He is the coordinator of the ‘Environmental Engineering and Water Resources’ Powerhouse of the Faculty of EIS. He also coordinates the ‘Water and Bioresource Technologies’ strength of the Simulation, Modelling, Analysis, Research and Teaching (SMART) Research Facility at UOW. As a member of the Faculty Research Committee (FRC) and the Faculty International Committee (FIC) leadership teams, Professor Hai participates in the review and implementation of the Faculty Research and International Partnership/Outreach programmes.
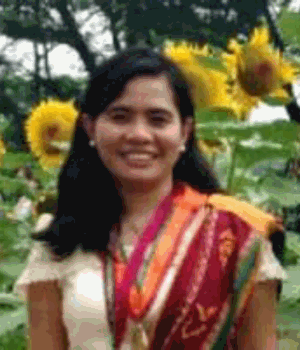
Ma. Catriona E. Devanadera
Ma. Catriona Devanadera is a faculty member and researcher at the Department of Community and Environmental Resources Planning, College of Human Ecology, University of the Philippines Los Banos, Philippines. She is an Environmental Engineer/Specialist in projects related to water and wastewater treatment, constructed wetlands treatment system, watershed management plan, sustainability planning, wetland management and conservation

Devendra P. Saroj
Devendra P. Saroj Dr (PhD, CEnv, MSOE, FHEA) is Senior Lecturer at the department of Civil and Environmental Engineering, University of Surrey United Kingdom. He is Head of Centre for Environmental Health and Engineering (CEHE) and Programme Director – MSc Water and Environmental Engineering. His work covers a wide area of water and wastewater treatment systems and processes. His recent works include nutrient pollution of freshwater bodies, natural wastewater treatment systems (wetlands) membrane systems for organic micro-pollutant removal, membrane-assisted bioreactors for domestic and industrial wastewater treatment and recycling, membrane technology assessment for urban water reuse scenarios, decision support in water supply, reuse and sanitation, adaptation of sewage treatment works for biological nutrient removal, removal of organic micro-pollutants, biofilm biotechnology for advanced wastewater treatment, microbial communities in bioreactors for wastewater treatment, recovery of phosphorus from sewage and anaerobic biodegradation of organic waste materials.
References
- WWAP (United Nations World Water Assessment Programme). The United Nations World water development Report 2015: water for a sustainable World. Paris: UNESCO; 2015.
- UN-Water. (2015). Wastewater management: A UN-Water Analytical Brief, United Nations Water.
- WWAP (United Nations World Water Assessment Programme). The United Nations World water development Report 2017: wastewater The Untapped resource. Paris: UNESCO; 2017.
- Bustillo-Lecompte CF, Kakar D, Mehrvar M. Photochemical treatment of benzene, toluene, ethylbenzene, and xylenes (BTEX) in aqueous solutions using advanced oxidation processes: towards a cleaner production in the petroleum refining and petrochemical industries. J Clean Prod. 2018;186:609–617. doi:https://doi.org/10.1016/J.JCLEPRO.2018.03.135.
- Shahmansouri A, Bellona C. Nanofiltration technology in water treatment and reuse: applications and costs. Water Sci Technol. 2015;71:309–319.
- Shokrollahzadeh S, Azizmohseni F, Golmohammad F, et al. Biodegradation potential and bacterial diversity of a petrochemical wastewater treatment plant in Iran. Bioresour Technol. 2008;99:6127–6133. doi:https://doi.org/10.1016/j.biortech.2007.12.034.
- Zou S, Yan N, Zhang C, et al. Acclimation of nitrifying biomass to phenol leads to persistent resistance to inhibition. Sci. Total Environ. 2019;693:133622. doi:https://doi.org/10.1016/j.scitotenv.2019.133622.
- Dvořák L, Svojitka J, Wanner J, et al. Nitrification performance in a membrane bioreactor treating industrial wastewater. Water Res. 2013;47:4412–4421. doi:https://doi.org/10.1016/j.watres.2013.03.053.
- Iqbal M, Nisar J, Adil M, et al. Mutagenicity and cytotoxicity evaluation of photo-catalytically treated petroleum refinery wastewater using an array of bioassays. Chemosphere. 2017;168:590–598. doi:https://doi.org/10.1016/j.chemosphere.2016.11.021.
- GA T. Residues analysis of organochlorine pesticides in fish, sediment and water samples from Tekeze Dam, Tigray, Ethiopia. J Environ Anal Toxicol. 2016;6:1–6.
- Choi YY, Baek SR, Kim JI, et al. Characteristics and biodegradability of wastewater organic matter in municipal wastewater treatment plants collecting domestic wastewater and industrial discharge. Water (Basel). 2017;9:1–12. doi:https://doi.org/10.3390/w9060409.
- Singh K, Arora S. Removal of synthetic textile dyes from wastewaters: a critical review on present treatment technologies. Crit Rev Environ Sci Technol. 2011;41:807–878.
- Mohsen MS, Jaber JO. Potential of industrial wastewater reuse. Desalination. 2003;152:281–289. doi:https://doi.org/10.1016/S0011-9164(02)01075-5.
- Javed T, Qureshi RM, Ahmad S, et al. An overview of environmental pollution status and waste treatment technology used in Pakistan. Int At Energy Agency. 1998;29:521–530.
- West Central Africa. (1995). Nigeria strategic options for redressing industrial pollution. doi: 287401468333530717.
- El-Naas MH, Alhaija MA, Al-Zuhair S. Evaluation of a three-step process for the treatment of petroleum refinery wastewater. J Environ Chem Eng. 2014;2:56–62. doi:https://doi.org/10.1016/j.jece.2013.11.024.
- Ishak S, Malakahmad A, Isa MH. Refinery wastewater biological treatment: a short review. J Sci Ind Res. 2012;71:251–256.
- Kuyukina MS, Krivoruchko AV, Ivshina IB. Advanced bioreactor treatments of hydrocarbon-containing wastewater. Appl Sci. 2020;10:1–19.
- Diya’Uddeen BH, Daud WMAW, Abdul Aziz AR. Treatment technologies for petroleum refinery effluents: A review. Process Saf Environ Prot. 2011;89:95–105. doi:https://doi.org/10.1016/j.psep.2010.11.003.
- Shabir G, Afzal M, Tahseen R, et al. Treatment of oil refinery wastewater using pilot scale fed batch reactor followed by coagulation and sand filtration. Am J Environ Prot. 2013;1:10–13.
- Gasim HA, Kutty SRM, Isa MH. Anaerobic treatment of petroleum refinery wastewater. World Acad Sci Eng Technol. 2012;6:512–515.
- Coelho A, Castro AV, Dezotti M, et al. Treatment of petroleum refinery sourwater by advanced oxidation processes. J Hazard Mater. 2006;137:178–184.
- Kamal AKI, Ahmed F, Hassan M, et al. Characterization of textile effluents from Dhaka Export processing Zone (DEPZ) area in Dhaka, Bangladesh. Sci Environ. 2016;2:141–151.
- Lim S-L, Chu W-L, Phang S-M. Use of Chlorella vulgaris for bioremediation of textile wastewater. Bioresour Technol. 2010;101:7314–7322.
- Sahinkaya E, Uzal N, Yetis U, et al. Biological treatment and nanofiltration of denim textile wastewater for reuse. J Hazard Mater. 2008;153:1142–1148.
- Yusuff RO, Sonibare JA. Characterization of textile industries ‘ effluents in Kaduna, Nigeria and pollution implications. Glob Nest Int J. 2004;6:212–221.
- Pal P. Treatment and disposal of pharmaceutical wastewater: toward the sustainable strategy. Sep Purif Rev. 2018;473:179–198.
- Gadipelly C, Peez-Gonzaíez A, Yadav GD, et al. Pharmaceutical industry wastewater: review of the technologies for water treatment and reuse. Ind Eng Chem Res. 2014;53:11571–11592.
- James OO, Oluwaleye SE, Saka KL, et al. Physico-chemical characteristics of pharmaceutical effluents from Sango industrial area. Nigeria Bull Env Pharmacol Life Sci. 2014;3:78–81.
- Lefebvre O, Shi X, Wu CH, et al. Biological treatment of pharmaceutical wastewater from the antibiotics industry. Water Sci Technol. 2014;69:855–861.
- Rajendran S M, Sekaran V. Generation, characteristics and treatment of municipal wastewater in Madurai City. Int J Civ Eng Technol. 2015;6:58–70.
- Dash AK. Characterization of domestic wastewater at Bhubaneswar, Odisha, India. The Ecoscan. 2013;Special issue Vol. III:297–305.
- Mahmoud M, Tawfik A, El-Gohary F. Use of down-flow hanging sponge (DHS) reactor as a promising post-treatment system for municipal wastewater. Chem Eng J. 2011;168:535–543. doi:https://doi.org/10.1016/j.cej.2011.01.019.
- Elmitwalli TA, Oahn KLT, Zeeman G, et al. Treatment of domestic sewage in a two-step anaerobic filter/anaerobic hybrid system at low temperature. Water Res. 2002;36:2225–2232. doi:https://doi.org/10.1016/S0043-1354(01)00438-9.
- Punzi M, Anbalagan A, Aragão Börner R, et al. Degradation of a textile azo dye using biological treatment followed by photo-Fenton oxidation: evaluation of toxicity and microbial community structure. Chem Eng J. 2015;270:290–299. doi:https://doi.org/10.1016/j.cej.2015.02.042.
- Singh RL, Singh PK, Singh RP. Enzymatic decolorization and degradation of azo dyes – a review. Int Biodeterior Biodegradation. 2015;104:21–31.
- Holkar CR, Jadhav AJ, Pinjari DV, et al. A critical review on textile wastewater treatments: possible approaches. J Environ Manage. 2016;182:351–366. doi:https://doi.org/10.1016/j.jenvman.2016.07.090.
- Kant R. Textile dyeing industry an environmental hazard. Nat Sci. 2012;4:22–26. doi:https://doi.org/10.4236/ns.2012.41004.
- Patel M, Kumar R, Kishor K, et al. Pharmaceuticals of emerging concern in aquatic systems: chemistry, occurrence, effects, and removal methods. Chem Rev. 2019;119:3510–3673. doi:https://doi.org/10.1021/acs.chemrev.8b00299.
- Koné M, Cologgi DL, Lu W, et al. Pharmaceuticals in Canadian sewage treatment plant effluents and surface waters: occurrence and environmental risk assessment. Environ Technol Rev. 2013;2:17–27. doi:https://doi.org/10.1080/21622515.2013.865793.
- Boutin C, Eme C. (2016). Domestic wastewater characterization by emission source, in: 13eme Congres Specialise IWA on small water and wastewater systems. Athenes, Greece, p. 8.
- Bai J, Xu H, Zhang Y, et al. Combined industrial and domestic wastewater treatment by periodic allocating water hybrid hydrolysis acidification reactor followed by SBR. Biochem Eng J. 2013;70:115–119. doi:https://doi.org/10.1016/j.bej.2012.10.009.
- El-Bestawy E. Treatment of mixed domestic-industrial wastewater using cyanobacteria. J Ind Microbiol Biotechnol. 2008;35:1503–1516.
- Stevens JL, Northcott GL, Stern GA, et al. PAHs, PCBs, PCNs, organochlorine pesticides, synthetic musks, and polychlorinated n-alkanes in U.K. sewage sludge: survey results and implications. Environ Sci Technol. 2003;37:462–467. doi:https://doi.org/10.1021/es020161y.
- Harrison EZ, Oakes SR, Hysell M, et al. Organic chemicals in sewage sludges. Sci Total Environ. 2006;367:481–497. doi:https://doi.org/10.1016/j.scitotenv.2006.04.002.
- Bennett GF. Impact of toxic chemicals on local wastewater treatment plant and the environment. Environ Geol Water Sci. 1989;13:201–212. doi:https://doi.org/10.1007/BF01665370.
- Chen C-F, Ju Y-R, Lim YC, et al. Determination of polycyclic aromatic hydrocarbons in sludge from water and wastewater treatment plants by GC-MS. Int J Environ Res Public Health. 2019;16; doi:https://doi.org/10.3390/ijerph16142604.
- Sharpley aN, Daniel T, Sims T, et al. Agricultural phosphorus and eutrophication second edition. United States Dep Agric ARS. 2003;149:1–43.
- Badawy MI, Ali MEM. Fenton’s peroxidation and coagulation processes for the treatment of combined industrial and domestic wastewater. J Hazard Mater. 2006;136:961–966. doi:https://doi.org/10.1016/j.jhazmat.2006.01.042.
- Nousheen R, Batool A, Rehman MSU, et al. Fenton-biological coupled biochemical oxidation of mixed wastewater for color and COD reduction. J Taiwan Inst Chem Eng. 2014;45:1661–1665.
- Tawfik A, Sobhey M, Badawy M. Treatment of a combined dairy and domestic wastewater in an up-flow anaerobic sludge blanket (UASB) reactor followed by activated sludge (AS system). Desalination. 2008;227:167–177. doi:https://doi.org/10.1016/j.desal.2007.06.023.
- Houda B, Dorra G, Chafai A, et al. Impact of a mixed “industrial and domestic” wastewater effluent on the southern coastal sediments of Sfax (Tunisia) in the Mediterranean Sea. Int J Environ Res. 2011;5:691–704.
- Ileri R, Sengil IA, Kulac S, et al. Treatment of mixed pharmaceutical industry and domestic wastewater by sequencing batch reactor. J Environ Sci Heal – Part A Toxic/Hazardous Subst Environ Eng. 2003;38:2101–2111. doi:https://doi.org/10.1081/ESE-120023336.
- El-Gohary FA, Abo-Elela SI. Management of wastewater from soap and food industries: a case study. Sci Total Environ. 1987;66:203–212.
- Nesaratnam ST, Ghobrial FH. Biological treatment of mixed industrial and sanitary wastewaters. Conserv Recycl. 1985;8:135–142.
- Qin JJ, Wai MN, Tao G, et al. Membrane bioreactor study for reclamation of mixed sewage mostly from industrial sources. Sep Purif Technol. 2007;53:296–300. doi:https://doi.org/10.1016/j.seppur.2006.08.002.
- Popat A, Nidheesh PV, Anantha Singh TS, et al. Mixed industrial wastewater treatment by combined electrochemical advanced oxidation and biological processes. Chemosphere. 2019;237:124419. doi:https://doi.org/10.1016/j.chemosphere.2019.124419.
- Scholz M. Activated sludge processes, in: wetlands for water pollution control. Elsevier. 2016: 91–105. doi:https://doi.org/10.1016/B978-0-444-63607-2.00015-0.
- Davis ML. (2010). Secondary treatment by suspended growth biological processes, Chapter (McGraw-Hill Professional, 2010), access Engineering, in: Water and wastewater engineering: Design principles and practice. The McGraw-Hill Companies, Inc., pp. 23-1-23–115.
- Alvarino T, Suarez S, Lema J, et al. Understanding the sorption and biotransformation of organic micropollutants in innovative biological wastewater treatment technologies. Sci Total Environ. 2018;615:297–306.
- Shi J, Han Y, Xu C, et al. Biological coupling process for treatment of toxic and refractory compounds in coal gasification wastewater. Rev Environ Sci Biotechnol. 2018;17:765–790. doi:https://doi.org/10.1007/s11157-018-9481-2.
- Lohi A, Alvarez Cuenca M, Anania G, et al. Biodegradation of diesel fuel-contaminated wastewater using a three-phase fluidized bed reactor. J Hazard Mater. 2008;154:105–111. doi:https://doi.org/10.1016/j.jhazmat.2007.10.001.
- Chen Y, Lin J, Chen Z. Remediation of water contaminated with diesel oil using a coupled process: biological degradation followed by heterogeneous Fenton-like oxidation. Chemosphere. 2017;183:286–293. doi:https://doi.org/10.1016/j.chemosphere.2017.05.120.
- Zdarta A, Smułek W, Pietraszak E, et al. Hydrocarbons biodegradation by activated sludge bacteria in the presence of natural and synthetic surfactants. J Environ Sci Heal Part A. 2016;51:1262–1268. doi:https://doi.org/10.1080/10934529.2016.1215194.
- Mohanty G, Mukherji S. Effect of an emulsifying surfactant on diesel degradation by cultures exhibiting inducible cell surface hydrophobicity. J Chem Technol Biotechnol. 2007;82:1004–1011.
- Capodici M, Cosenza A, Di Trapani D, et al. Treatment of oily wastewater with membrane bioreactor systems. Water. 2017;9:412. doi:https://doi.org/10.3390/w9060412.
- Mannina G, Cosenza A, Di Trapani D, et al. Membrane bioreactors for treatment of saline wastewater contaminated by hydrocarbons (diesel fuel): an experimental pilot plant case study. Chem Eng J. 2016;291:269–278. doi:https://doi.org/10.1016/j.cej.2016.01.107.
- Tong K, Zhang Y, Liu G, et al. Treatment of heavy oil wastewater by a conventional activated sludge process coupled with an immobilized biological filter. Int Biodeterior Biodegrad. 2013;84:65–71. doi:https://doi.org/10.1016/j.ibiod.2013.06.002.
- Ma DY, Wang XH, Song C, et al. Aerobic granulation for methylene blue biodegradation in a sequencing batch reactor. Desalination. 2011;276:233–238.
- Farooqi IH, Basheer F, Tiwari P. Biodegradation of methylene blue Dye by sequential treatment using anaerobic hybrid reactor and submerged aerobic Fixed Film bioreactor. J Inst Eng Ser A. 2017;98:397–403. doi:https://doi.org/10.1007/s40030-017-0251-x.
- Malachova K, Rybkova Z, Sezimova H, et al. Biodegradation and detoxification potential of rotating biological contactor (RBC) with Irpex lacteus for remediation of dye-containing wastewater. Water Res. 2013;47:7143–7148. doi:https://doi.org/10.1016/j.watres.2013.07.050.
- Ogunlaja A, Nwankwo IN, Omaliko ME, et al. Biodegradation of methylene blue as an evidence of synthetic dyes mineralization during textile effluent biotreatment by Acinetobacter pittii. Environ Process. 2020;7:931–947. doi:https://doi.org/10.1007/s40710-020-00443-6.
- Li D, Yang M, Hu J, et al. Determination of penicillin G and its degradation products in a penicillin production wastewater treatment plant and the receiving river. Water Res. 2008;42:307–317.
- Gulkowska A, Leung H, So M, et al. Removal of antibiotics from wastewater by sewage treatment facilities in. Water Res. 2008;42:395–403. doi:https://doi.org/10.1016/j.watres.2007.07.031.
- Zhang Y, Geißen SU, Gal C. (2008). Carbamazepine and diclofenac: removal in wastewater treatment plants and occurrence in water bodies. Chemosphere. doi: https://doi.org/10.1016/j.chemosphere.2008.07.086.
- Zhang Y, Geißen SU. Elimination of carbamazepine in a non-sterile fungal bioreactor. Bioresour. Technol. 2012;112:221–227. doi:https://doi.org/10.1016/j.biortech.2012.02.073.
- Alvarino T, Suarez S, Lema JM, et al. Understanding the removal mechanisms of PPCPs and the influence of main technological parameters in anaerobic UASB and aerobic CAS reactors. J. Hazard. Mater. 2014;278:506–513.
- Clara M, Kreuzinger N, Strenn B, et al. The solids retention time – a suitable design parameter to evaluate the capacity of wastewater treatment plants to remove micropollutants. Water Res. 2005;39:97–106. doi:https://doi.org/10.1016/j.watres.2004.08.036.
- Kruglova A, Ahlgren P, Korhonen N, et al. Biodegradation of ibuprofen, diclofenac and carbamazepine in nitrifying activated sludge under 12°C temperature conditions. Sci Total Environ. 2014;499:394–401. doi:https://doi.org/10.1016/j.scitotenv.2014.08.069.
- Mizzouri NS, Shaaban MG. Individual and combined effects of organic, toxic, and hydraulic shocks on sequencing batch reactor in treating petroleum refinery wastewater. J Hazard Mater. 2013;250–251:333–344. doi:https://doi.org/10.1016/j.jhazmat.2013.01.082.
- Van Hamme JD, Ward OP. Influence of chemical surfactants on the biodegradation of crude oil by a mixed bacterial culture. Can J Microbiol. 1999;45:130–137. doi:https://doi.org/10.1139/w98-209.
- Chrzanowski L, Owsianiak M, Szulc A, et al. Interactions between rhamnolipid biosurfactants and toxic chlorinated phenols enhance biodegradation of a model hydrocarbon-rich effluent. Int Biodeterior Biodegrad. 2011;65:605–611. doi:https://doi.org/10.1016/j.ibiod.2010.10.015.
- Gargouri B, Karray F, Mhiri N, et al. Application of a continuously stirred tank bioreactor (CSTR) for bioremediation of hydrocarbon-rich industrial wastewater effluents. J Hazard Mater. 2011;189:427–434. doi:https://doi.org/10.1016/j.jhazmat.2011.02.057.
- Thakur C, Srivastava VC, Mall ID. Aerobic degradation of petroleum refinery wastewater in sequential batch reactor. J Environ Sci Heal Part A. 2014;49:1436–1444. doi:https://doi.org/10.1080/10934529.2014.928557.
- Saeed A, Iqbal M, Zafar SI. Immobilization of Trichoderma viride for enhanced methylene blue biosorption: batch and column studies. J Hazard Mater. 2009;168:406–415. doi:https://doi.org/10.1016/j.jhazmat.2009.02.058.
- Tomei MC, Pascual JS, Angelucci DM. Analysing performance of real textile wastewater bio-decolourization under different reaction environments. J Clean Prod. 2016;129:468–477.
- Işik M, Sponza DT. Decolorization of Azo dyes under batch anaerobic and sequential anaerobic/aerobic conditions. J Environ Sci Heal – Part A Toxic/Hazardous Subst Environ Eng. 2004;39:1107–1127. doi:https://doi.org/10.1081/ESE-120028417.
- Zhou LJ, Rong ZY, Gu W, et al. Integrated fate assessment of aromatic amines in aerobic sewage treatment plants. Environ Monit Assess. 2020;192. doi:https://doi.org/10.1007/s10661-020-8111-y.
- Lourenco ND, Franca RDG, Moreira MA, et al. Comparing aerobic granular sludge and flocculent sequencing batch reactor technologies for textile wastewater treatment. Biochem Eng J. 2015;104:57–63.
- Franca RDG, Vieira A, Mata AMT, et al. Effect of an azo dye on the performance of an aerobic granular sludge sequencing batch reactor treating a simulated textile wastewater. Water Res. 2015;85:327–336.
- Lalnunhlimi S, Krishnaswamy V. Decolorization of azo dyes (direct blue 151 and direct Red 31) by moderately alkaliphilic bacterial consortium. Brazilian J Microbiol. 2016;47:39–46.
- Besha AT, Gebreyohannes AY, Tufa RA, et al. Removal of emerging micropollutants by activated sludge process and membrane bioreactors and the effects of micropollutants on membrane fouling: a review. J Environ Chem Eng. 2017;5:2395–2414. doi:https://doi.org/10.1016/j.jece.2017.04.027.
- Ahmed MB, Zhou JL, Ngo HH, et al. Progress in the biological and chemical treatment technologies for emerging contaminant removal from wastewater: a critical review. J Hazard Mater. 2017;323:274–298. doi:https://doi.org/10.1016/j.jhazmat.2016.04.045.
- Tadkaew N, Hai FI, McDonald JA, et al. Removal of trace organics by MBR treatment: the role of molecular properties. Water Res. 2011;45:2439–2451. doi:https://doi.org/10.1016/j.watres.2011.01.023.
- Cecconet D, Molognoni D, Callegari A, et al. Biological combination processes for efficient removal of pharmaceutically active compounds from wastewater: a review and future perspectives. J Environ Chem Eng. 2017. doi:https://doi.org/10.1016/j.jece.2017.07.020
- Luo Y, Guo W, Hao Ngo H, et al. A review on the occurrence of micropollutants in the aquatic environment and their fate and removal during wastewater treatment. Sci Total Environmen. 2014;473–474:619–641.
- Tran NH, Urase T, Kusakabe O. The characteristics of enriched nitrifier culture in the degradation of selected pharmaceutically active compounds. J Hazard Mater. 2009;171:1051–1057. doi:https://doi.org/10.1016/j.jhazmat.2009.06.114.
- Kirchman DL. Degradation of organic material, in: processes in microbial Ecology. Oxford: Oxford University Press; 2013.
- Chan YJ, Chong MF, Law CL, et al. A review on anaerobic-aerobic treatment of industrial and municipal wastewater. Chem Eng J. 2009. doi:https://doi.org/10.1016/j.cej.2009.06.041
- Zhao HW, Mavinic DS, Oldham WK, et al. Controlling factors for simultaneous nitrification and denitrification in a two-stage intermittent aeration process treating domestic sewage. Water Res. 1999;33:961–970. doi:https://doi.org/10.1016/S0043-1354(98)00292-9.
- Van Der Star WRL, Abma WR, Blommers D, et al. Startup of reactors for anoxic ammonium oxidation: experiences from the first full-scale anammox reactor in Rotterdam. Water Res. 2007;41:4149–4163. doi:https://doi.org/10.1016/j.watres.2007.03.044.
- Terada A, Zhou S, Hosomi M. Presence and detection of anaerobic ammonium-oxidizing (anammox) bacteria and appraisal of anammox process for high-strength nitrogenous wastewater treatment: A review. Clean Technol Environ Policy. 2011;13:759–781. doi:https://doi.org/10.1007/s10098-011-0355-3.
- Cho S, Kambey C, Nguyen V. Performance of anammox processes for wastewater treatment: A critical review on effects of operational conditions and environmental stresses. Water (Basel). 2019;12:20. doi:https://doi.org/10.3390/w12010020.
- Ma B, Wang S, Cao S, et al. Biological nitrogen removal from sewage via anammox: recent advances. Bioresour Technol. 2016;200:981–990. doi:https://doi.org/10.1016/j.biortech.2015.10.074.
- Lochmatter S, Gonzalez-Gil G, Holliger C. Optimized aeration strategies for nitrogen and phosphorus removal with aerobic granular sludge. Water Res. 2013;47:6187–6197. doi:https://doi.org/10.1016/j.watres.2013.07.030.
- Broughton A, Pratt S, Shilton A. Enhanced biological phosphorus removal for high-strength wastewater with a low rbCOD:P ratio. Bioresour Technol. 2008;99:1236–1241. doi:https://doi.org/10.1016/j.biortech.2007.02.013.
- Oehmen A, Lemos PC, Carvalho G, et al. Advances in enhanced biological phosphorus removal: from micro to macro scale. Water Res. 2007. doi:https://doi.org/10.1016/j.watres.2007.02.030
- Hu JY, Ong SL, Ng WJ, et al. A new method for characterizing denitrifying phosphorus removal bacteria by using three different types of electron acceptors. Water Res. 2003;37:3463–3471. doi:https://doi.org/10.1016/S0043-1354(03)00205-7.
- Kim YM, Park D, Lee DS, et al. Inhibitory effects of toxic compounds on nitrification process for cokes wastewater treatment. J Hazard Mater. 2008;152:915–921. doi:https://doi.org/10.1016/j.jhazmat.2007.07.065.
- Nemerow NL. Joint treatment of raw industrial waste with domestic sewage. In: Nemerow NL, editor. Industrial waste treatment. Oxford: Butterworth-Heinemann; 2007. p. 175–244. doi:https://doi.org/10.1016/b978-012372493-9/50045-4.
- Mohan SV, Rao NC, Prasad KK, et al. Treatment of complex chemical wastewater in a sequencing batch reactor (SBR) with an aerobic suspended growth configuration. Process Biochem. 2005;40:1501–1508. doi:https://doi.org/10.1016/j.procbio.2003.02.001.
- Guieysse B, Norvill ZN. Sequential chemical-biological processes for the treatment of industrial wastewaters: review of recent progresses and critical assessment. J Hazard Mater. 2014. doi:https://doi.org/10.1016/j.jhazmat.2013.12.016
- Egbuikwem PN, Naz I, Saroj DP. Appraisal of suspended growth process for treatment of mixture of simulated petroleum, textile, domestic, agriculture and pharmaceutical wastewater. Environ Technol. 2019. doi:https://doi.org/10.1080/09593330.2019.1609097
- Egbuikwem PN, Mierzwa JC, Saroj DP. Evaluation of aerobic biological process with post-ozonation for treatment of mixed industrial and domestic wastewater for potential reuse in agriculture. Bioresour Technol. 2020a;318:124200. doi:https://doi.org/10.1016/j.biortech.2020.124200.
- Kutty SRM, Gasim HA, Khamaruddin PF, et al. Biological treatability study for refinery wastewater using bench scale sequencing batch reactor systems. Water Resour Manag. VI. 2011;145:691–699. doi:https://doi.org/10.2495/WRM110621.
- Ochieng A, Odiyo JO, Mutsago M. Biological treatment of mixed industrial wastewaters in a fluidised bed reactor. J Hazard Mater. 2003;96:79–90. doi:https://doi.org/10.1016/S0304-3894(02)00166-8.
- The City of Dallas, O. (1971). Combined treatment of domestic and industrial wastes by activated sludge.
- Gori R, Lubello C, Ferrini F, et al. Reuse of industrial wastewater for the irrigation of ornamental plants. Water Sci Technol. 2008a;57:883–889. doi:https://doi.org/10.2166/wst.2008.185.
- Chávez AM, Gimeno O, Rey A, et al. Treatment of highly polluted industrial wastewater by means of sequential aerobic biological oxidation-ozone based AOPs. Chem Eng J. 2019;361:89–98. doi:https://doi.org/10.1016/j.cej.2018.12.064.
- Jolibois B, Guerbet M. Evaluation of industrial, hospital and domestic wastewater genotoxicity with the Salmonella fluctuation test and the SOS chromotest. Mutat Res. 2005;565:151–162. doi:https://doi.org/10.1016/j.mrgentox.2004.10.006.
- FAO. (2017). Water for sustainable food and agriculture: a report produced for the G20 Presidency of Germany [WWW Document]. Food Agric Organ. http://www.fao.org/3/a-i7959e.pdf.
- WHO. (2006). WHO Guidelines for the safe use of wastewater, excreta and greywater. Vol. II: Geneva: Wastewater Use in Agriculture.
- US-EPA. (2012). Guidelines for water reuse. EPA/600/R-12/618. https://www3.epa.gov/region1/npdes/merrimackstation/pdfs/ar/AR-1530.pdf.
- Alcalde-Sanz L, Gawlik BM. Minimum quality requirements for water reuse in agricultural irrigation and aquifer recharge - Towards a legal instrument on water reuse at EU level, EUR 28962 EN. Luxembourg: Publications Office of the European Union. 2017.
- World Bank. (2017). World development indicator. Water Resources Management [WWW Document]. URL https://www.worldbank.org/en/topic/waterresourcesmanagement (accessed 8.11.18).
- Rezapour S, Atashpaz B, Moghaddam SS, et al. Heavy metal bioavailability and accumulation in winter wheat (Triticum aestivum L.) irrigated with treated wastewater in calcareous soils. Sci Total Environ. 2019;656:261–269. doi:https://doi.org/10.1016/j.scitotenv.2018.11.288.
- Dhaouefi Z, Toledo-Cervantes A, García D, et al. Assessing textile wastewater treatment in an anoxic-aerobic photobioreactor and the potential of the treated water for irrigation. Algal Res. 2018;29:170–178. doi:https://doi.org/10.1016/j.algal.2017.11.032.
- Younas S, Rizvi H, Ali S, et al. Irrigation of Zea mays with UASB-treated textile wastewater; effect on early irrigation of Zea mays with UASB-treated textile wastewater; effect on early growth and physiology. Environ Sci Pollut Res. 2020;27:15305–15324. doi:https://doi.org/10.1007/s11356-020-07948-5.
- Hashem HA, Hassanein RA, El-Deep MH, et al. Irrigation with industrial wastewater activates antioxidant system and osmoprotectant accumulation in lettuce, turnip and tomato plants. Ecotoxicol Environ Saf. 2013;95:144–152. doi:https://doi.org/10.1016/j.ecoenv.2013.05.030.
- Chow YN, Lee LK, Zakaria NA, et al. Insight into the chromium-enriched industrial wastewater irrigation practice on Lablab purpureus. J Environ Eng. 2020;146. doi:https://doi.org/10.1061/(ASCE)EE.1943-7870.0001742.
- Ali F, Ullah H, Khan I. Heavy metals accumulation in vegetables irrigated with industrial influents and possible impact of such vegetables on human health. Sarhad J Agric. 2017;33; doi:https://doi.org/10.17582/journal.sja/2017/33.3.489.500.
- Libutti A, Gatta G, Gagliardi A, et al. Agro-industrial wastewater reuse for irrigation of a vegetable crop succession under Mediterranean conditions. Agric Water Manag. 2018;196:1–14. doi:https://doi.org/10.1016/J.AGWAT.2017.10.015.
- Bhateria R, Dhaka R. Impact of electroplating effluent on growth of Triticum aestivum and Hordeum vulgare. Environ Technol Innov. 2017;8:389–398. doi:https://doi.org/10.1016/j.eti.2017.09.005.
- Kaushik P, Garg VK, Singh B. Effect of textile effluents on growth performance of wheat cultivars. Bioresour Technol. 2005;96:1189–1193. doi:https://doi.org/10.1016/j.biortech.2004.09.020.
- Ramana S, Biswas AK, Kundu S, et al. Effect of distillery effluent on seed germination in some vegetable crops. Bioresour Technol. 2002;82:273–275. doi:https://doi.org/10.1016/S0960-8524(01)00184-5.
- Al-Absi KM, Al-Nasir FM, Mahadeen AY. Mineral content of three olive cultivars irrigated with treated industrial wastewater. Agric Water Manag. 2009;96:616–626. doi:https://doi.org/10.1016/j.agwat.2008.09.026.
- Rivoira L, Castiglioni M, Kettab A, et al. Impact of effluents from wastewater treatments reused for irrigation: strawberry as case study. Environ Eng Manag J. 2019;18:2133–2143.
- Pandey R, Singh J. Effect of industrial wastewater irrigation on productivity of wheat crop effect of industrial wastewater irrigation on productivity of wheat crop. Int J Environ Sci. 2015;6:402–409. doi:https://doi.org/10.6088/ijes.6045.
- Sedlacko EM, Jahn CE, Heuberger AL, et al. Potential for beneficial reuse of oil and gas-derived produced water in agriculture: physiological and morphological responses in Spring wheat (Triticum aestivum). Environ Toxicol Chem. 2019;38:1756–1769. doi:https://doi.org/10.1002/etc.4449.
- Tiwari KK, Singh NK, Patel MP, et al. Metal contamination of soil and translocation in vegetables growing under industrial wastewater irrigated agricultural field of Vadodara, Gujarat, India. Ecotoxicol Environ Saf. 2011;74:1670–1677. doi:https://doi.org/10.1016/j.ecoenv.2011.04.029.
- Ivy N, Hossain MK, Hossain ML. Effects of industrial effluents on germination and early growth of selected agricultural crops. J Agron. 2015;14:43–48.
- Alemu T, Mekonnen A, Leta S. Integrated tannery wastewater treatment for effluent reuse for irrigation: Encouraging water efficiency and sustainable development in developing countries. J Water Process Eng. 2019;30:100514. doi:https://doi.org/10.1016/j.jwpe.2017.10.014.
- Sen SK, Patra P, Das CR, et al. Pilot-scale evaluation of bio-decolorization and biodegradation of reactive textile wastewater: an impact on its use in irrigation of wheat crop. Water Resour Ind. 2019;21:100106. doi:https://doi.org/10.1016/j.wri.2019.100106.
- Mabasa NC, Jones CLW, Laing M. The use of treated brewery effluent for salt tolerant crop irrigation. Agric Water Manag. 2021;245:106590. doi:https://doi.org/10.1016/j.agwat.2020.106590.
- Mazhar S, Ditta A, Bulgariu L, et al. Sequential treatment of paper and pulp industrial wastewater: prediction of water quality parameters by Mamdani Fuzzy Logic model and phytotoxicity assessment. Chemosphere. 2019;227:256–268. doi:https://doi.org/10.1016/j.chemosphere.2019.04.022.
- Egbuikwem PN, Mierzwa JC, Saroj DP. Assessment of suspended growth biological process for treatment and reuse of mixed wastewater for irrigation of edible crops under hydroponic conditions. Agric Water Manag. 2020b;231:106034. doi:https://doi.org/10.1016/j.agwat.2020.106034.
- Hajihashemi S, Noedoost F, Hedayatzadeh F. Characterization of Brassica napus responses to diluted and undiluted industrial wastewater. Physiol Mol Biol Plants. 2019;25:1469–1482. doi:https://doi.org/10.1007/s12298-019-00717-w.
- Kretschmer N, Ribbe L, Gaese H. Wastewater reuse for agriculture. Technol Ressour Manag Dev Sci Contrib Sustain Dev. 2004;2:37–64.
- Echchelh A, Hess T, Sakrabani R. Reusing oil and gas produced water for irrigation of food crops in drylands. Agric Water Manag. 2018. doi:https://doi.org/10.1016/j.agwat.2018.05.006
- WHO. (1973). Reuse of effluents: methods of wastewater treatment and health safeguards. Report of a WHO meeting of experts. World Health Organization – Technical Report Series, No. 517. Geneva.
- Khan S, Aijun L, Zhang S, et al. Accumulation of polycyclic aromatic hydrocarbons and heavy metals in lettuce grown in the soils contaminated with long-term wastewater irrigation. J Hazard Mater. 2008;152:506–515. doi:https://doi.org/10.1016/J.JHAZMAT.2007.07.014.