Abstract
The extracellular matrix (ECM) plays important roles in maintaining adequate adipose tissue function and in metabolic regulation. Here we have examined the organization of a relatively unexplored adipose tissue ECM component, elastin and its response to diet induced obesity in mice. Additionally, we have explored the regulation and requirement of macrophage metalloelastase, MMP-12, in adipose tissue ECM remodeling in obesity. In visceral fat depots, elastin fibers form a mesh-like net that becomes denser with diet-induced obesity. In contrast, the elastin fibers in subcutaneous adipose depots are more linear in organization, and are tightly associated with adipose tissue macrophages (ATMs). We found that Mmp12 is produced predominantly by ATMs and can be induced with both short- and long-term high fat diet challenge and rapid remodeling induced by lipolysis. This contrasts with Mmp14 and Timp1 which are further induced only after chronic obesity in non-ATM populations. We examined obese transgenic Mmp12−/− mice and found an increase in gene expression of ECM genes with diet-induced obesity, but showed few significant differences in metabolic parameters, elastin matrix density, or in adipose tissue inflammation. Together, these studies reveal the architecture and diet-induced regulation of the elastin matrix and suggest that MMP-12 is not required for elastin matrix remodeling or for the metabolic dysfunction that occurs with obesity.
Introduction
The proper function of adipocytes is dependent on stromal cells in adipose tissue, as well as the organization and composition of the extracellular matrix (ECM). The adipose tissue ECM is composed of a diverse network of proteins that include collagens (Type I, III, V, and VI), fibronectin, and elastin.Citation1 The healthy physiologic expansion of adipose tissue requires a tightly regulated balance of ECM deposition and degradation.Citation2,3 Obesity promotes ECM accumulation in adipose tissue, which can contribute to fibrosis and ultimately impair its function as an efficient nutrient storage organ.Citation4 Associations between adipose tissue fibrosis and metabolic disease has led to a considerable interest in understanding the mechanisms that regulate adipose tissue ECM remodeling.Citation4
Elastin is an ECM protein found in almost all tissues, but there is a paucity of information regarding its distribution and regulation in adipose tissue. Elastin is a heavily cross-linked protein that enables tissues to stretch and return back to its original conformation.Citation5,6 In human adipose tissue, the density of the elastin network was higher in tissues from lean individuals compared to obese individuals, suggesting remodeling of the elastin network with increased adiposity.Citation7 An interaction between collagen and elastin networks in adipose tissue has been suggested, as elastin expression is decreased in Collagen VI-deficient mice, which have a less dense collagen matrix that is permissive to adipocyte hypertrophy.Citation8 Inhibition of lysyl oxidase, an enzyme that crosslinks both collagen and elastin fibers, can improve metabolic parameters in obese animals.Citation9,10 In addition to this structural role, elastin-derived peptides have been shown to contribute to insulin resistance by blocking glucose uptake in muscle and adipose tissue.Citation11
The degradation of the ECM proteins such as collagen and elastin is controlled by proteinases that include matrix metalloproteinases (MMPs) and their endogenous inhibitors such as tissue inhibitors of metalloproteinases (TIMPs).Citation12-14 Of these, MMP-12 is a metalloelastase that has the capacity to degrade elastin and plays important roles in tissue fibrosis and injury.Citation15-17 Mmp12 expression is highly induced in adipose tissue of mice with diet-induced obesity, and its expression has been reported in both mature adipocytes as well as in adipose tissue macrophages (ATMs).Citation13,14,18,19 The importance of Mmp12 in obesity-induced metabolic dysfunction remains unclear as one report failed to identify any metabolic phenotype in Mmp12−/− mice despite a decrease in ATMs and another found that Mmp12 deficiency provided protection from metabolic dysfunction and obesity-induced adipose tissue inflammation.Citation20
In this report, we describe an extensive network of elastin fibers in adipose tissue that are regulated by diet-induced obesity. Elastin deposition is increased in visceral adipose tissue depots with obesity and there are significant qualitative differences in the cross linking of elastin fibers between depots. Since we observe a strong physical association between ATMs and elastin we have examined the response to dietary obesity in Mmp12−/− mice and observe changes in ECM gene expression but no significant change in metabolic measures with Mmp12 deficiency.
Results
Adipose tissue depot specific differences in the organization of the elastin matrix
While the collagen network is relatively well characterized in adipose tissue, little is known about the distribution of the adipose tissue elastin network and how this changes with obesity. We assessed elastin composition in adipose tissue using several complementary methods. First, Verhoeff's stain, previously used to identify elastin in other tissues, was performed on section from visceral (epididymal; eWAT) and subcutaneous (inguinal; iWAT) white adipose tissue depots from lean (16wks ND) and obese (16wks HFD) male C57BL/6 mice. In lean mice, Verhoeff+ regions were more prominent in iWAT compared to eWAT (). With obesity, Verhoeff+ areas increased in eWAT and were concentrated around crown-like structures (CLSs) – regions of adipocyte death and ATM accumulation. In contrast, iWAT from obese mice had reduced Verhoeff+ regions relative to lean tissues. To verify the specificity of Verhoeff's stain for elastin, we performed immunohistochemistry (IHC) for elastin on eWAT and iWAT from lean and obese (). In eWAT, elastin+ regions were increased with HFD and recapitulated the observations with Verhoeff stain. Less elastin+ staining was observed in iWAT compared to eWAT and there were no significant changes in elastin positivity in iWAT with HFD. The iWAT staining did not match the Verhoeff's reagent and suggests non-specific staining in iWAT may limit the use of the Verhoeff's reagent.
Figure 1. High fat diet induced obesity modifies the adipose tissue elastin matrix. Paraffin embedded sections were stained with (A) Verhoeff Van Gieson's elastin stain and (B) Immunohistochemical staining with anti-elastin antibodies. Samples were obtained from lean (ND) and obese (HFD) mouse epididymal (eWAT) and subcutaneous inguinal (iWAT) tissues. Representative images shown from one of 5 replicate samples with similar results. (scale bar = 100 μM).
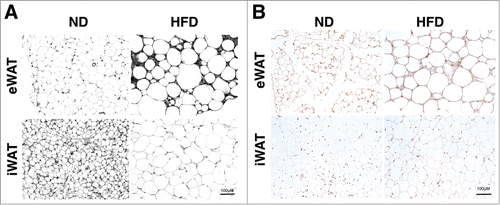
As a third approach, elastin was assessed in fixed whole mount adipose tissue samples by immunofluorescence microscopy. Elastin fibers in eWAT of ND mice formed a highly cross-linked mesh-like network around adipocytes (). In stark contrast, elastin fibers in iWAT were organized in individual fiber bundles with less cross-linking compared to eWAT (). After 16 weeks of HFD, the elastin network in eWAT showed an increase in the density of elastin fibers and with more intersecting fibers (). The iWAT elastin network in obese mice also demonstrated a qualitative increase in intersecting fibers (). Quantification of elastin+ regions confirmed the increase in elastin fibers in eWAT, but not iWAT with HFD. () The mean linear intercept (MLI) method was utilized to quantify reticulation (increased branching is associated with a decrease in MLI).Citation21,22 This demonstrated a significant increase in elastin branching in eWAT compared to iWAT and an increase in branching with HFD feeding (decrease in MLI) (). No significant changes in elastin (Eln) gene expression were seen in eWAT or iWAT suggesting that post-transcriptional regulation of elastin degradation/formation may be a critically regulated step ().
Figure 2. Elastin matrix architecture differences between visceral and subcutaneous tissues. Immunofluorescence imaging of elastin (red), resident ATMs (MGL1; green), and blood vessels (Isolectin; Blue). (A, B) Representation of whole mount tissues from lean (ND) eWAT and iWAT and (C, D) obese (HFD) eWAT and iWAT. (scale bar = 50 μM). (E) Graphical representation of percent elastin positive (%Eln+ area) and (F) reticulation by Mean Linear Intercept (MLI). (G) Gene expression analysis of Elastin from eWAT from ND and HFD animals. (H) Representative image of ATMs bound to Elastin matrix (scale bar = 100 μM). (mean ± SEM; n = 2-4 per group ,*P < 0.05).
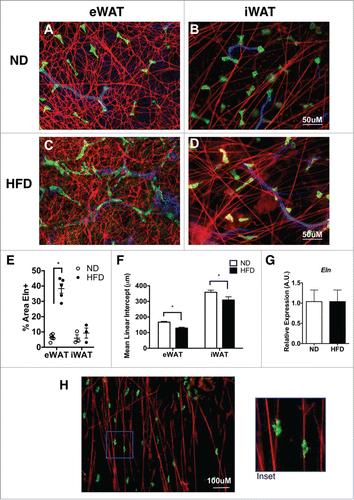
Mmp12 expression is induced in ATMs with obesity
MGL1+ resident ATMs are found in all adipose tissue depots and are the dominant macrophage population in lean mice. Citation23 In iWAT, MGL1+ ATMs were observed to be tightly associated with elastin fibers (). The close physical association between ATMs and elastin fibers led us to explore the hypothesis that macrophages may participate in the remodeling of the elastin matrix via the macrophage metalloelastase MMP-12.
We examined Mmp12 expression in 4 adipose tissue depots (eWAT, iWAT, peri-renal adipose tissue (rWAT) and mesenteric adipose tissue (mWAT)) after a short, 4-week HFD challenge to induce rapid fat expansion. This short term HFD treatment induced significant adipose tissue expansion without accumulation of CD11c+ ATMs (data not shown). Gene expression analysis revealed that Mmp12 was induced at least 80 fold in all 4 depots after HFD challenge when compared to lean age-matched ND-fed controls (). In contrast, the expression of Timp1, an endogenous inhibitor of MMP-12, was not altered by obesity in eWAT or iWAT, but was induced in mWAT and rWAT depots (). Expression of Mmp14, a pericellular collagenase, decreased in eWAT with short-term HFD, but did not change in other depots (). Long-term HFD (16wks) induced both Mmp12 and Timp1 expression in eWAT (). Mmp14 increased in eWAT after HFD ().
Figure 3. Mmp12 dynamically regulated in adipose tissue with short-term and long-term diet induced obesity. Analysis of (A) Mmp12, (B) Timp1, and (C) Mmp14 gene expression in major fat pad depots (mWAT=mesenteric, rWAT=perirenal) from lean (ND) and obese (HFD) mice after 4 weeks (n=4). (D, E, G) eWAT gene expression analysis for (D) Mmp12, (E) Timp1, and (F) Mmp14 from animals on ND or HFD for 16 weeks. (mean ± SEM; n=4-6 per group, *P < 0.05, **< 0.01***0.001, ****0.0001).
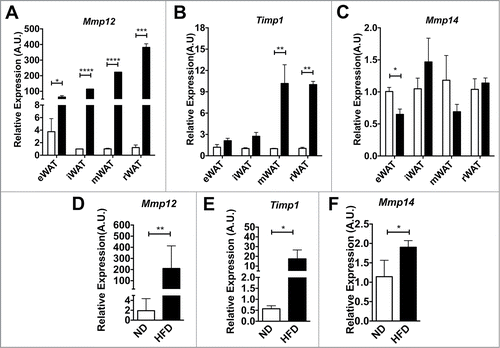
Based on the range of reports in the literature, we next set out to clarify the source of Mmp12 expression in adipose tissue after diet-induced obesity. We analyzed Mmp12 gene expression from total adipose tissue or from adipose tissues that had been fractionated to separate mature adipocytes and the stromal vascular fraction (SVF). Consistent with previous reports, the SVF was identified as the major site of Mmp12 induction (). FACS cell sorting was then used to separate ATMs (F4/80+CD11b+) and Non-ATMs from the SVF. Mmp12 expression was enriched in the ATM sub-fraction from both lean and obese groups and was induced by HFD only in the ATM sub-fraction. () In contrast, the expression of Timp1 and Mmp14 were more prominently expressed in the non-ATM sub-fraction, where they were both induced with HFD (). Overall, our data demonstrate a rapid induction of Mmp12 in ATMs with fat expansion that precedes other genes involved in ECM remodeling.
Figure 4. Mmp12 is primarily induced in adipose tissue macrophages with HFD. (A) Expression of Mmp12 from total eWAT, dissociated adipocyte fraction (Adipo), and the stromal vascular fraction (SVF) normalized to total ND eWAT levels. Expression analysis of (B) Mmp12, (C) Timp1, and (D) Mmp14 from FACS sorted eWAT SVF. (ATMs= Black bars, F4/80+ CD11b+, Non-ATMs = White bars, F4/80−CD11b−). (mean ± SEM; n = 4 per group, *P < 0.05, **< 0.01***0.001, ****0.0001).
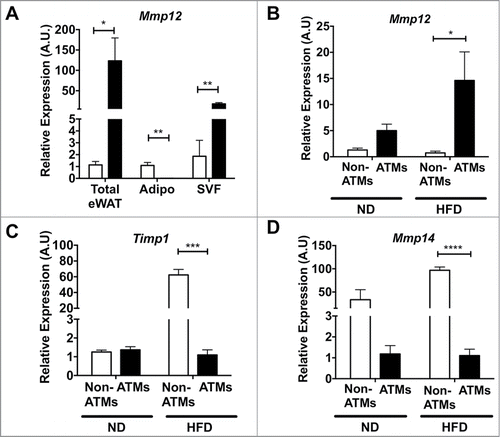
Lipolytic remodeling activates Mmp12 expression
To observe rapid adipose tissue remodeling in a different context, we employed the use of an acute lipolysis model.Citation24 To examine the regulation of Mmp12 gene expression in this setting, we assessed age-matched lean (ND) and obese (16-week HFD) mice after 3 days of treatment with a β3 adrenergic receptor agonist, CL−316243 (CL). Compared to lean vehicle-treated controls, Mmp12 was induced by CL compound (). In obese mice, Mmp12 was highly expressed compared to lean controls, however CL did not induce further Mmp12 expression in obese mice. CL had no significant effects on Timp1 expression or Mmp14 expression in lean or obese mice ().
Mmp12 deficiency does not alter weight or metabolism with high fat diet feeding
From our observations, we hypothesized that MMP-12 would play a critical role in modifying the elastin matrix to permit adipocyte hypertrophy in response to high calorie diets and obesity. Therefore, we tested the impact of Mmp12 deficiency on adiposity and measures of metabolic dysfunction and inflammation in adipose tissue. To assess this, Mmp12−/−, Mmp12+/−, and Mmp12+/+ littermates were fed ND or HFD for 10 weeks. In adult lean mice, there were no significant differences in body weight or glucose metabolism as seen by fasting glucose measurements (data not shown). In the HFD fed group, Mmp12−/− and Mmp12+/− mice had higher body weights compared to Mmp12+/+ during the later stages of HFD feeding. () However, body composition analysis showed no significant differences in percent lean or fat mass. To evaluate glucose regulation, we measured fasting glucose and insulin levels, and performed glucose and insulin tolerance tests and observed no significant differences between genotypes (). Tissue weights of the liver, eWAT, iWAT, and rWAT fat depots did not differ between genotypes, indicating that adipose tissue expansion was not dependent upon Mmp12 (). Adipocyte size () and density (# of adipocytes per gram, not shown) did not differ between genotypes, nor did expression of the markers of adiposity Lep or AdipoQ ().
Figure 6. Mmp12 deficiency does not influence body composition or metabolism. (A) Body weights were measured in male Mmp12−/−, Mmp12+/−, and Mmp12+/+ mice fed HFD for 10 weeks, body composition was measured at 8 weeks of HFD. (B) Glucose Tolerance Tests (GTT), Insulin tolerance tests (ITT), and fasting serum insulin concentrations were examined. (C) eWAT weights were recorded after sacrifice, and (D) a graphical representation of adipocyte number by size was recorded. (E) AdipoQ and Lep gene expression was examined. (F) Verhoeff's stain and (G) immunofluorescence stain for Mac-2+ ATMs in HFD-fed Mmp12−/− mice and wild type controls shown in gray scale. (scale bar = 100 μm) (mean ± SEM; n = 4-10).
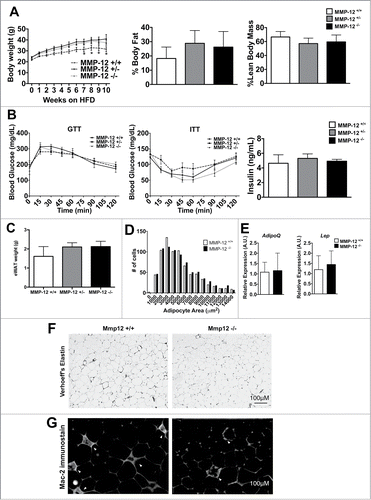
To evaluate if Mmp12 plays a role in chronic obesity and metabolism, we fed Mmp12−/− and Mmp12+/+ HFD for 25–27 weeks. In contrast to short term feeding, no significant differences in body weight, body composition, fat pad weights, or glucose tolerance were observed between genotypes (data not shown). Together, these data suggest that an Mmp12 deficiency does not play a significant role in adipose tissue expansion, metabolism, or chronic obesity.
Mmp12 deficiency does not influence ECM deposition or ATM accumulation
We assessed long term HFD fed Mmp12−/− and Mmp12+/+ mice for adipose tissue ECM and did not observe significant differences in the deposition of total collagen as measured by Picrosirius collagen stain (data not shown). Similarly, we did not see differences in the organization of the elastin matrix in eWAT as measured by Verhoeff's stain (). Because Mmp12 may impact ATM function, we examined CLS formation and observed a mild qualitative decrease in the number of Mac-2+ CLS in eWAT of Mmp12−/− animals similar to other reports ().Citation20 However, flow cytometry analysis failed to detect any differences in the quantity of CD11c+ ATMs and CD11c− resident ATMs in long-term HFD fed Mmp12+/+ and Mmp12−/− mice (data not shown).
Mmp12 deficient animals express higher levels of ECM genes
Finally, we assessed if Mmp12 deficiency influences ECM gene expression in adipose tissue. From the eWAT from HFD- fed Mmp12−/− and Mmp12+/+ mice, small but statistically significant increases in the expression of Col1a, Col5a, Col6a3, and Tgfb1 in eWAT of Mmp12−/− mice were observed (). We did not observe differences in expression of genes involved in crosslinking ECM components and ECM processing (). Evaluation of genes related to macrophage migration and inflammation in adipose tissue showed an increase in Csf1, but a decrease in Mrc1 gene expression (a marker of alternatively activated macrophages) (). No significant differences in expression of chemokine genes were observed ().
Figure 7. Gene expression analysis of markers of extracellular matrix remodeling in Mmp12−/− mice. Mmp12+/+ data shown in white bars, Mmp12−/− in black bars. (A, B) Gene expression analyses of ECM remodeling-related genes and markers of ECM maturity and crosslinking, (C, D) inflammatory cytokines and chemokines are shown (mean ± SEM; n = 4-8, *P < 0.05, **< 0.01***0.001).
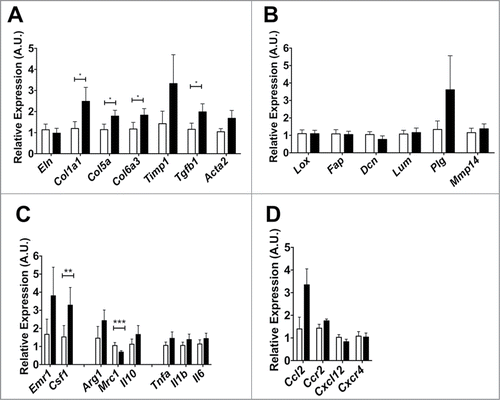
Discussion
The makeup of the adipose tissue ECM has the potential to influence whole-body metabolism by modifying the ability of adipocytes to store nutrients. Of the ECM components, adipose tissue elastin is one of the least understood. Elastin has unique mechanical properties that provide tissue flexibility and resilience to deformation.Citation25 Our findings demonstrate 1) a distinct depot-specific architecture of the elastin network in adipose tissue with more a dense network of elastin fibers in visceral compared to subcutaneous adipose tissues, 2) the use of elastin fibers perhaps as a scaffold by ATMs, 3) ATMs are the main source of the metalloelastase Mmp12 that is induced with lipolysis, short-term, and chronic HFD challenge, and 4) obese Mmp12−/− mice have increase expression of ECM genes, but do not demonstrate significant differences in glucose metabolism or adipose tissue inflammation compared to WT mice.
In several tissue fibrosis settings, the density of elastin has been shown to increase after injury.Citation26,27 Our results match a study reporting the pericellular localization of heterogenic elastin fibers around adipocytes.Citation25 Our results add further detail about its organization and changes with obesity and suggest the importance of post-translational regulation of elastin stability and fiber organization in adipose tissue. The stark differences in elastin organization and mesh density between the visceral and subcutaneous depots may shed some light into why the expansion and inflammatory potential of these 2 fat pads are different. We posit that the organization of elastin fibers may be responsible for the metabolic and inflammatory differences between visceral the subcutaneous adipose depots.
Our immunostaining revealed the surprising observation that many resident ATMs are bound to elastin fibers, and was more clearly observed in subcutaneous depots. This may fall in line with observations that elastin and its fragments can stimulate macrophage chemotaxis.Citation28,29 ATMs may be using elastin fibers as an organizing scaffold or alternatively located there poised to degrade or remodel the elastin network.
To further study the role of MMP-12 in adipose tissue, we challenged Mmp12−/− mice to short term and long term HFD. We observed a small increase in total body weight in the Mmp12−/− mice compared to WT controls with the short-term treatment. This effect was not observed with a longer HFD challenge. Our short term diet challenge results are comparable to the study by Lee et al. however our studies differ because they demonstrated a profound increase in adipose tissue mass which we did not observe.Citation30 It is unclear why the body weight differences did not persist with chronic feeding in our studies. In both cohorts however, we did not observe significant metabolic differences related to Mmp12 deficiency. This observation concurs with Bauters et al. which show no metabolic phenotype despite an decrease in ATMs, and conflicts with Lee et al. who suggest that Mmp12 plays a role in aggravating insulin resistance.Citation20,30 Differences in experimental design and husbandry conditions (e.g. microbiome) may explain our differing results. Bauters et al. used a mouse strain on a mixed genetic background. Lee et al. used Mmp12−/− animals on a C57BL/6 background, yet it is unclear if littermate controls were used. We feel that the use of littermate and heterozygous controls is appropriate, and in our hands, these data fail to demonstrate significant effects of Mmp12 on metabolism.
The observation that Mmp12 is robustly and rapidly induced with lipolytic and obesogenic stimuli in iWAT may be consistent with a requirement for elastin to be remodeled to permit adipocyte morphological changes such as with hypertrophy. However, from our mouse studies, it appears that overall Mmp12 is dispensable in the overall regulation of the elastin network. Our findings further emphasize the importance of overlapping and redundant function of MMPs and other ECM remodeling enzymes. It suggests that other elastases such as cathepsins and neutrophil elastases which have been implicated in obesity and metabolic disease may be play a more prominent role in adipose tissue.Citation31,32 Overall, while we have added further detail in one of the components of the adipose tissue extracellular matrix, our data suggests that MMP-12 does not play a significant role in regulating the elastin matrix, metabolism, or in modifying adipose tissue macrophage recruitment or phenotypes in mice.
Methods/Materials
Mice
C57BL/6J and B6.129X-Mmp12tm1Sds/J (Mmp12−/−) mice were obtained from The Jackson Laboratories. Male C57 mice, (6 weeks of age) were fed a normal diet (ND, 4.5% fat Research Diets, New Brunswick, NJ), or High fat diet (HFD, 60% fat from lard; Research Diets). For Mmp12−/− experiments, littermate males were fed a HFD ad libitum starting at 8 weeks of age. For lipolysis experiments, mice fed a ND or HFD for 16 weeks were injected with 1 mg/kg of CL316243 or PBS-vehicle intraperitonealy (IP) for 3 consecutive days. All procedures were approved by the University of Michigan University Committee on Use of Care of Animals and were in compliance with the Institute of Laboratory Animal Research Guide for the Care and Use of Laboratory Animals.
Quantitative real-time PCR
Tissues were frozen in liquid nitrogen and stored at −80°C. Total RNA was isolated from adipose tissues, SVCs, or FACS-sorted cells using the RNeasy Lipid Tissue Kit or QIAzol lysis reagent (Qiagen, Valencia, CA) followed by DNAse digestion according to manufacturer's instructions. cDNA was generated from 0.5-1 ug of RNA using the High Capacity cDNA Reverse Transcription Kits (Applied Biosystems, Carlsbad, CA). Real-time PCR analysis was performed using SYBR Green PCR Master Mix (Applied Biosystems) and StepOnePlus thermocycler (Applied Biosystems). Relative expression was performed in duplicate samples and determined using the 2−ΔΔCT method after normalizing to Arbp. Mmp12 to Timp1 ratios calculated by dividing Mmp12 relative expression levels by Timp1 relative expression levels.
Microscopy and histology
Tissues used for sectioning were fixed and stored in 10% Buffered Formalin. Anti-Elastin antibodies for immunofluorescence staining were kindly provided by Dr. Robert Mecham (Washington University in St. Louis). Macrophages were stained with anti-MGL-1 (AbCam) or anti-Mac-2 (eBiosciences) antibodies. Whole mount immunofluorescence microscopy was performed as describedCitation33. Images were captured using an Olympus inverted microscope fitted with an Olympus DP72 camera. ImageJ software (NIH) was used measure adipocyte size and to assemble composite images. Mean Linear Intercept (MLI) was used to measure the mean free distance between elastin matrix fibers, was performed by point counting method as described.Citation34 Elastin positive area (%Eln+ area) calculated with ImageJ software.
Analysis of adipose tissue stromal vascular fraction (SVF)
SVF isolation and flow cytometry were performed as described.Citation35 Antibodies used were: CD45-eFluor405, CD64-PE, and CD11c-APCCy7, F480-APC, CD11b-APCCy7, and propidium iodide viability stain. Flow Cytometry was performed using a FACS CANTO II Flow Cytometer (BD Biosciences) and sorting on a FACSAria III. Analysis with FlowJo software (Tree Star, Ashland, OR).
Metabolic studies
Glucose and Insulin tolerance tests were performed as described.Citation36 Plasma insulin levels were measured using a mouse insulin ELISA kit (Crystal Chem, Downers Grove, IL). Body composition analysis (body fat, lean mass, free fluid) was performed by the Animal Phenotyping Core at the University of Michigan's Nutrition and Obesity Research Center using the NMR-based analyzer (Minispec LF90II, Bruker Optics).
Statistical analysis
Data are expressed as the standard error of the mean (SEM). GraphPad Prism software (GraphPad Software, La Jolla, CA) was used for statistical analyses. Differences between groups were determined using unpaired 2-tailed Student's t-test, where a P ≤ 0.05 was considered significant.
Disclosure of Potential Conflicts of Interest
No potential conflicts of interest were disclosed.
Table_S1.docx
Download MS Word (120.7 KB)Acknowledgment
We thank Dr. Robert Mecham (Washington University in St. Louis) for providing elastin antibodies.
Funding
This work was carried out with support from grants from the NIH (DK090262 and DK092873) and the American Diabetes Association to C.N.L and an NIH Diversity Supplement for DK090262-S1 to GM-S. This work utilized Core Services from the Michigan Nutrition and Obesity Research Center supported by grant DK089503 of NIH to the University of Michigan. This work utilized the Microscopy Core of the Michigan Diabetes Research Center funded by NIH 2P30-DK20572 from the National Institute of Diabetes & Digestive & Kidney Diseases.
References
- Divoux A, Tordjman J, Lacasa D, Veyrie N, Hugol D, Aissat A, Basdevant A, Guerre-Millo M, Poitou C, Zucker JD, et al. Fibrosis in human adipose tissue: composition, distribution, and link with lipid metabolism and fat mass loss. Diabetes 2010; 59:2817-25; PMID:20713683; http://dx.doi.org/10.2337/db10-0585
- Chun TH. Peri-adipocyte ECM remodeling in obesity and adipose tissue fibrosis. Adipocyte 2012; 1:89-95; PMID:23700517; http://dx.doi.org/10.4161/adip.19752
- Chun TH, Hotary KB, Sabeh F, Saltiel AR, Allen ED, Weiss SJ. A pericellular collagenase directs the 3-dimensional development of white adipose tissue. Cell 2006; 125:577-91; PMID:16678100; http://dx.doi.org/10.1016/j.cell.2006.02.050
- Sun K, Tordjman J, Clement K, Scherer PE. Fibrosis and adipose tissue dysfunction. Cell Metab 2013; 18:470-7; PMID:23954640; http://dx.doi.org/10.1016/j.cmet.2013.06.016
- Kielty CM, Sherratt MJ, Shuttleworth CA. Elastic fibres. J Cell Sci 2002; 115:2817-28; PMID:12082143
- Holst J, Watson S, Lord MS, Eamegdool SS, Bax DV, Nivison-Smith LB, Kondyurin A, Ma L, Oberhauser AF, Weiss AS, et al. Substrate elasticity provides mechanical signals for the expansion of hemopoietic stem and progenitor cells. Nat Biotechnol 2010; 28:1123-8; PMID:20890282; http://dx.doi.org/10.1038/nbt.1687
- Spencer M, Unal R, Zhu B, Rasouli N, McGehee RE Jr, Peterson CA, Kern PA. Adipose tissue extracellular matrix and vascular abnormalities in obesity and insulin resistance. J Clin Endocrinol Metab 2011; 96:E1990-8; PMID:21994960; http://dx.doi.org/10.1210/jc.2011-1567
- Khan T, Muise ES, Iyengar P, Wang ZV, Chandalia M, Abate N, Zhang BB, Bonaldo P, Chua S, Scherer PE. Metabolic dysregulation and adipose tissue fibrosis: role of collagen VI. Mol Cell Biol 2009; 29:1575-91; PMID:19114551; http://dx.doi.org/10.1128/MCB.01300-08
- Maki JM, Sormunen R, Lippo S, Kaarteenaho-Wiik R, Soininen R, Myllyharju J. Lysyl oxidase is essential for normal development and function of the respiratory system and for the integrity of elastic and collagen fibers in various tissues. Am J Pathol 2005; 167:927-36; PMID:16192629; http://dx.doi.org/10.1016/S0002-9440(10)61183-2
- Halberg N, Khan T, Trujillo ME, Wernstedt-Asterholm I, Attie AD, Sherwani S, Wang ZV, Landskroner-Eiger S, Dineen S, Magalang UJ, et al. Hypoxia-inducible factor 1alpha induces fibrosis and insulin resistance in white adipose tissue. Mol Cell Biol 2009; 29:4467-83; PMID:19546236; http://dx.doi.org/10.1128/MCB.00192-09
- Blaise S, Romier B, Kawecki C, Ghirardi M, Rabenoelina F, Baud S, Duca L, Maurice P, Heinz A, Schmelzer CE, et al. Elastin-derived peptides, new regulators of insulin resistance development in mice. Diabetes 2013; PMID:23919962
- Lijnen HR, Maquoi E, Hansen LB, Van Hoef B, Frederix L, Collen D. Matrix metalloproteinase inhibition impairs adipose tissue development in mice. Atertio Thromb Vasc Biol 2002; 22:374-9; http://dx.doi.org/10.1161/hq0302.104522
- Maquoi E, Munaut C, Colige A, Collen D, Lijnen HR. Modulation of adipose tissue expression of murine matrix metalloproteinases and their tissue inhibitors with obesity. Diabetes 2002; 51:1093-101; PMID:11916931; http://dx.doi.org/10.2337/diabetes.51.4.1093
- Chavey C, Mari B, Monthouel MN, Bonnafous S, Anglard P, Van Obberghen E, Tartare-Deckert S. Matrix metalloproteinases are differentially expressed in adipose tissue during obesity and modulate adipocyte differentiation. J Biol Chem 2003; 278:11888-96; PMID:12529376; http://dx.doi.org/10.1074/jbc.M209196200
- Werb Z, Gordon S. Elastase secretion by stimulated macrophages. Characterization and regulation. J Exp Med 1975; 142:361-77; PMID:167096; http://dx.doi.org/10.1084/jem.142.2.361
- Hautamaki RD, Kobayashi DK, Senior RM, Shapiro SD. Requirement for macrophage elastase for cigarette smoke-induced emphysema in mice. Science 1997; 277:2002-4; PMID:9302297; http://dx.doi.org/10.1126/science.277.5334.2002
- Hunninghake GM, Cho MH, Tesfaigzi Y, Soto-Quiros ME, Avila L, Lasky-Su J, Stidley C, Melen E, Soderhall C, Hallberg J, et al. MMP12, lung function, and COPD in high-risk populations. N Engl J Med 2009; 361:2599-608; PMID:20018959; http://dx.doi.org/10.1056/NEJMoa0904006
- Shaul ME, Bennett G, Strissel KJ, Greenberg AS, Obin MS. Dynamic, M2-like remodeling phenotypes of CD11c+ adipose tissue macrophages during high-fat diet–induced obesity in mice. Diabetes 2010; 59:1171-81; PMID:20185806; http://dx.doi.org/10.2337/db09-1402
- Huber J, Loffler M, Bilban M, Reimers M, Kadl A, Todoric J, Zeyda M, Geyeregger R, Schreiner M, Weichhart T, et al. Prevention of high-fat diet-induced adipose tissue remodeling in obese diabetic mice by n-3 polyunsaturated fatty acids. Int J Obes (Lond) 2007; 31:1004-13; PMID:17130847; http://dx.doi.org/10.1038/sj.ijo.0803511
- Bauters D, Van Hul M, Lijnen HR. Macrophage elastase (MMP-12) in expanding murine adipose tissue. Biochim Biophys Acta 2013; 1830:2954-9; PMID:23295969; http://dx.doi.org/10.1016/j.bbagen.2012.12.024
- Anyanwu AC, Bentley JK, Popova AP, Malas O, Alghanem H, Goldsmith AM, Hershenson MB, Pinsky DJ. Suppression of inflammatory cell trafficking and alveolar simplification by the heme oxygenase-1 product carbon monoxide. Am J Physiol Lung Cell Mol Physiol 2014; 306:L749-63; PMID:24532288; http://dx.doi.org/10.1152/ajplung.00236.2013
- Lumeng CN, Liu J, Geletka L, Delaney C, Delproposto J, Desai A, Oatmen K, Martinez-Santibanez G, Julius A, Garg S, et al. Aging is associated with an increase in T cells and inflammatory macrophages in visceral adipose tissue. J Immunol 2011; 187:6208-16; PMID:22075699; http://dx.doi.org/10.4049/jimmunol.1102188
- Lumeng CN, Bodzin JL, Saltiel AR. Obesity induces a phenotypic switch in adipose tissue macrophage polarization. J Clin Invest 2007; 117:175-84; PMID:17200717; http://dx.doi.org/10.1172/JCI29881
- Kosteli A, Sugaru E, Haemmerle G, Martin JF, Lei J, Zechner R, Ferrante AW Jr. Weight loss and lipolysis promote a dynamic immune response in murine adipose tissue. J Clin Invest 2010; 120:3466-79; PMID:20877011; http://dx.doi.org/10.1172/JCI42845
- Alkhouli N, Mansfield J, Green E, Bell J, Knight B, Liversedge N, Tham JC, Welbourn R, Shore AC, Kos K, et al. The mechanical properties of human adipose tissues and their relationships to the structure and composition of the extracellular matrix. Am J Physiol Endocrinol Metab 2013; 305:E1427-35; PMID:24105412; http://dx.doi.org/10.1152/ajpendo.00111.2013
- Blaauboer ME, Boeijen FR, Emson CL, Turner SM, Zandieh-Doulabi B, Hanemaaijer R, Smit TH, Stoop R, Everts V. Extracellular matrix proteins: a positive feedback loop in lung fibrosis? Matrix Biol 2014; 34:170-8; PMID:24291458; http://dx.doi.org/10.1016/j.matbio.2013.11.002
- Wagenseil JE, Mecham RP. Elastin in large artery stiffness and hypertension. J Cardiovasc Transl Res 2012; 5:264-73; PMID:22290157; http://dx.doi.org/10.1007/s12265-012-9349-8
- Kamisato S, Uemura Y, Takami N, Okamoto K. Involvement of intracellular cyclic GMP and cyclic GMP-dependent protein kinase in alpha-elastin-induced macrophage chemotaxis. J Biochem 1997; 121:862-7; PMID:9192726; http://dx.doi.org/10.1093/oxfordjournals.jbchem.a021666
- Hunninghake GW, Davidson JM, Rennard S, Szapiel S, Gadek JE, Crystal RG. Elastin fragments attract macrophage precursors to diseased sites in pulmonary emphysema. Science 1981; 212:925-7; PMID:7233186; http://dx.doi.org/10.1126/science.7233186
- Lee JT, Pamir N, Liu NC, Kirk EA, Averill MM, Becker L, Larson I, Hagman DK, Foster-Schubert KE, van Yserloo B, et al. Macrophage metalloelastase (MMP12) regulates adipose tissue expansion, insulin sensitivity, and expression of inducible nitric oxide synthase. Endocrinology 2014; 155:3409-20; PMID:24914938; http://dx.doi.org/10.1210/en.2014-1037
- Talukdar S, Oh da Y, Bandyopadhyay G, Li D, Xu J, McNelis J, Lu M, Li P, Yan Q, Zhu Y, et al. Neutrophils mediate insulin resistance in mice fed a high-fat diet through secreted elastase. Nat Med 2012; 18:1407-12; PMID:22863787; http://dx.doi.org/10.1038/nm.2885
- Yang M, Sun J, Zhang T, Liu J, Zhang J, Shi MA, Darakhshan F, Guerre-Millo M, Clement K, Gelb BD, et al. Deficiency and inhibition of cathepsin K reduce body weight gain and increase glucose metabolism in mice. Arterioscler Thromb Vac Biol 2008; 28:2202-8; http://dx.doi.org/10.1161/ATVBAHA.108.172320
- Lumeng CN, DelProposto JB, Westcott DJ, Saltiel AR. Phenotypic switching of adipose tissue macrophages with obesity is generated by spatiotemporal differences in macrophage subtypes. Diabetes 2008; 57:3239-46; PMID:18829989; http://dx.doi.org/10.2337/db08-0872
- Knudsen L, Weibel ER, Gundersen HJ, Weinstein FV, Ochs M. Assessment of air space size characteristics by intercept (chord) measurement: an accurate and efficient stereological approach. J Appl Physiol (1985) 2010; 108:412-21; PMID:19959763; http://dx.doi.org/10.1152/japplphysiol.01100.2009
- Morris DL, Oatmen KE, Wang T, DelProposto JL, Lumeng CN. CX3CR1 deficiency does not influence trafficking of adipose tissue macrophages in mice with diet-induced obesity. Obesity 2012; 20:1189-99; PMID:22252034; http://dx.doi.org/10.1038/oby.2012.7
- Morris DL, Cho KW, Delproposto JL, Oatmen KE, Geletka LM, Martinez-Santibanez G, Singer K, Lumeng CN. Adipose tissue macrophages function as antigen presenting cells and regulate adipose tissue CD4+ T cells in mice. Diabetes 2013; 62:2762-72; PMID:23493569; http://dx.doi.org/10.2337/db12-1404