Abstract
The circadian clock is an essential time-keeping mechanism that entrains internal physiology to environmental cues. Despite the well-established link between the molecular clock and metabolic homeostasis, an intimate interplay between the clock machinery and the metabolically active brown adipose tissue (BAT) is only emerging. Recently, we came to appreciate that the formation and metabolic functions of BAT, a key organ for body temperature maintenance, are under an orchestrated circadian clock regulation. Two complementary studies from our group uncover that the cell-intrinsic clock machinery exerts concerted control of brown adipogenesis with consequent impacts on adaptive thermogenesis, which adds a previously unappreciated temporal dimension to the regulatory mechanisms governing BAT development and function. The essential clock transcriptional activator, Bmal1, suppresses adipocyte lineage commitment and differentiation, whereas the clock repressor, Rev-erbα, promotes these processes. This newly discovered temporal mechanism in fine-tuning BAT thermogenic capacity may enable energy utilization and body temperature regulation in accordance with external timing signals during development and functional recruitment. Given the important role of BAT in whole-body metabolic homeostasis, pharmacological interventions targeting the BAT-modulatory activities of the clock circuit may offer new avenues for the prevention and treatment of metabolic disorders, particularly those associated with circadian dysregulation.
Introduction
The circadian clock is the overt ∼24 hour daily rhythm in physiology and behavior that evolved to respond to Earth's rotation. This evolutionarily-conserved mechanism that synchronizes diverse internal biological processes with environmental timing cues ensures organismal adaptation, fitness and survival.Citation1-3
The circadian clock system is comprised of a hierarchal structure.Citation1-3 The central clock, residing in the suprachiasmatic nuclei (SCN) in hypothalamus, receives light input originating from retina through the retino-hypothalamic tract and entrains peripheral tissue clocks. Peripheral clocks are self-sustaining clock mechanisms that exist in nearly all tissue/cell types that are outside of and driven by SCN. In both central and peripheral tissues, the ∼24 hour clock oscillatory cycle is generated by interlocking transcriptional regulatory loops coupled with translational control. Brain and muscle Arnt-like 1 (BMAL1), a basic helix-loop-helix transcription factor, is an essential positive regulator of the core molecular clock loop.Citation2 Bmal1 forms a heterodimer with CLOCK (Circadian Locomotor Output Cycles Kaput), which recognizes conserved E-box (CACGTG) or tandem E1-E2 elements to elicit target gene transcription.Citation4 BMAL1/CLOCK direct targets include negative regulators of the core clock circuit, the Period genes (Per1, 2 and 3) and the Cryptochromes (Cry1 and 2). These factors inhibit the transcriptional activity of BMAL1/CLOCK, which, coupled with temporally-controlled proteosome-mediated degradation mechanisms, generates the daily oscillations of the molecular clock. In addition, an intricate BMAL1-Rev-erbα/β transcriptional negative feedback loop forms a reinforcing mechanism that safeguards the robustness of the clock circuitry.Citation5 Rev-erbα/β are both direct target genes, and potent repressors of Bmal1 that participates its negative feedback regulation. Together with positive transcriptional regulation by retinoid acid receptor-related orphan receptor (RORα,β,γ), Rev-erbs/RORs generates the rhythmic oscillation of Bmal1 gene transcription.Citation6
BAT is a specialized thermogenic organ, owning to its unique ability to generate heat through the action of uncoupling protein-1 (UCP-1).Citation7 In addition to its key role in maintaining body temperature under cold-stress, the metabolic functions of BAT are increasingly recognized as important contributors to global energy balance, insulin sensitivity and lipid metabolism.Citation8-10 With the alarming rate of obesity and associated metabolic disorders in our society, harnessing the ability of BAT to augment energy consumption and metabolic substrate oxidation represents an attractive target for metabolic disease therapy. Hence, recent years have witnessed rapid progresses in our understanding of the regulatory mechanisms concerning the development and functions of BAT. However, how brown fat-intrinsic clock machinery may function in this highly metabolically active tissue is only beginning to be appreciated.
Circadian clock control of metabolic homeostasis
The clock confers critical temporal control on metabolism, which enables adaptation of nutrient utilization and energy homeostasis with circadian timing.Citation11 The circadian regulation of rate-limiting steps of key metabolic pathways ranges from TCA cycle, mitochondrial function to glucose and fatty acid synthesis and oxidation,Citation12 which leads to diurnal oscillations of various metabolites.Citation13 Due to the wide range of temporal regulation involved in metabolic control, numerous animal models,Citation14-16 epidemiologicalCitation17,18 and clinical studiesCitation19 demonstrate that disruption of this mechanism leads to a myriad of metabolic abnormalities. Furthermore, examination of specific tissue-resident peripheral clocks in metabolic organs, such as the liver, adipose tissue, and the islet, reveal that they are key mediators of diurnal nutrient partitioning and systemic metabolic homeostasis.Citation20-22 Particularly striking is the tight link between circadian disruption and the development of obesity and insulin resistance. Although neural and humoral control through the central clock is a significant component of the overall metabolic dysregulation observed,Citation15 clock regulators, including Bmal1,Citation21 Per2,Citation23 and Rev-erbαCitation24 can directly interact with the adipogenic cascade to modulate adipogenesis. These accumulating evidence indicate that compromised temporal coordination hinders the body's ability to maintain energy balance.
Circadian misalignment frequently occurs in people on a shift work schedule or experience trans-meridian travel. However, in the modern society upon the advent of artificial lighting, dys-synchronization of activity cycles with the natural day-night cycles are ever increasingly prevalent.Citation25 This wide-spread “social jet-lag,” conflict between the natural circadian cycles and activity cycles, could be a significant yet currently under-appreciated environmental etiology of metabolic diseases based on compelling epidemiological evidence. Experimental settings mimicking shift-work schedule or artificial light exposure reveal a range of metabolic dysregulations in animal models and clinical cohorts.Citation17,26-29 Metabolic consequences of circadian misalignment could, therefore, contribute to the current epidemics of metabolic disorders and pose significant public health risks.
As circadian disruption in humans involves the central clock as well as tissue-resident peripheral clocks, the obesogenic effects of circadian misalignment likely originates from metabolic perturbations in all metabolic tissues involved. The current challenge, therefore, is to pinpoint the specific contributions of individual tissue clocks to the global metabolic perturbations in order to devise targeted interventions. Hence, an important aspect is to ascertain clock functions in the metabolically active BAT, and define its specific contribution to metabolic disease processes including obesity and diabetes.
Intricate interplays among circadian clock, body temperature regulation and BAT activity
Accumulating findings indicate intricate interplays among the circadian clock machinery, body temperature regulation system, and the thermogenic functions of brown fat. The known interactions between these components are illustrated in . As a mechanism evolved to anticipate and adapt to environmental timing cues, such as seasonal changes in light and temperature, the clock machinery is conceivably intertwined with body temperature regulation. Other than light as a prevailing entrainment signal on earth, temperature is a universal cue for clock phase resetting.Citation30,31 Interestingly, although peripheral clocks are sensitive to temperature re-setting within the physiological range, the central clock in SCN is completely resistant to temperature entrainment, which allows the ability of the SCN in mammals to drive body temperature rhythms.Citation30
Figure 1. Intricate interplay between circadian clock, brown fat and body temperature homeostatic regulation. Temperature is a universal clock resetting signal. The clock, through central clock-driven neuronal innervation to brown fat or the brown fat intrinsic clock regulations, feeds into body temperature homeostatic control.
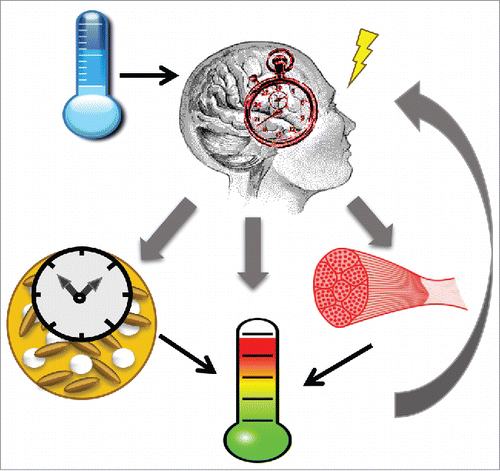
The influence of the clock system on temperature homeostatic regulation has also been explored. In homeothermic mammals, body temperature fluctuates within a physiological range, 36°C to 38.5°C in mice, through intricate mechanisms involving the thermoregulatory center in the brain stem, temperature-sensing thermoreceptors in various tissues, and effector organs involved in heat generation, conservation or dissipation.Citation32 Remarkably, despite the tight homeostatic control, body temperature displays a daily rhythmicity that rises at the active phase and falls during resting.Citation33 Through adaptive thermogenesis, BAT is a major thermo-effector organ for heat production to maintain constant body temperature.Citation7 Recently, a diurnal rhythm of BAT activity was detected by Fluoro-18-deoxyglucose (FDG) uptake, which coincides with body temperature fluctuations peaking prior to the active period and dropping to lowest level before start of the resting period.Citation34 Therefore, it is conceivable that the observed oscillatory BAT activity may, at least in part, contribute to the circadian rhythmicity of physiological body temperature fluctuation.
A number of studies to date indicate that circadian clock machinery is present and actively participate in brown adipose tissue function. On one hand, the retinohypothalamic tract, which conveys the light input signal from the retina to the central clock SCN, can stimulate the thermogenic activity of BAT through its neuronal projections,Citation35,36 a potential component linking central clock and BAT function. On the other, circadian clock gene oscillations have been characterized in BAT and white adipose tissues (WAT).Citation37 Interestingly, thermogenic inducers regulate clock in BAT but not WAT.Citation38,39 Per2 gene was found to co-activate PPARα to induce Ucp1 and Fabp3 upon cold challenge in BAT.Citation39 Upon 4°C cold stimulation, clock genes, including Bmal1, Clock, and Cry1, are significantly up-regualted, whereas Rev-erbα and Rev-erbβ are suppressed.Citation38 In contrast, these genes fail to respond to cold stimuli in WAT. A similarly differential response of clock in BAT vs. WAT depots to β-adrenergic agents were also observed. This distinct clock regulation by thermogenic stimuli could be attributable to the presence of tissue-specific co-regulators that modulate circadian transcription factor gene regulation, or difference in adrenergic signaling transduction in brown vs. white adipocytes. The responsiveness of BAT clock to thermogenic stimuli suggests that it may facilitate to achieve overt body temperature rhythm through time-dependent heat production. In aggregate, current evidence indicates that the BAT-resident clock could be a critical link between clock and body temperature regulation. It is also intriguing to postulate that, based on the evolutionary role of the clock in adaptation to environmental cues, a temperature-entrained clock in BAT may enable animals living in the wild to adjust to wild seasonal fluctuations in external temperature.
Clock regulation of brown fat function
The aforementioned study using FDG uptake PET-CT scan offers direct evidence of the circadian control of BAT metabolic function.Citation34 However, as BAT activity is under the control of sympathetic nervous system and the observed diurnal rhythm coincides with sympathetic drive, this phenomenon could be driven, at least in part, by central clock-regulated neural output in mice. Thus, the precise contribution of an intrinsic clock to oscillatory BAT function remains to be further defined. Interestingly, photoperiod length, which directly entrains the circadian clock, has an appreciable effect on thermogenic activities.Citation40,41 A seasonal variation of detectable BAT activity in patients was also reported, and its correlation with photoperiod length is stronger than with environmental temperature.Citation42 Thus, given that clock components of BAT are capable of responding to β-adrenergic stimuli and cold challenge,Citation38 the BAT clock may integrate environmental cues with its functional activation.
Recent studies of genetic animal models provide direct proof that clock regulators, including Bmal1, Period2, and Rev-erbα, participate in the thermogenic functions of BAT.Citation39,43,44 Our study of Bmal1 reveals that it suppresses brown adipogenesis and loss of this function promotes BAT formation, consequently leading to enhanced BAT thermogenic activity and resistance against cold challenge.Citation44 Interestingly, the Period 2 (Per2) gene, which inhibits BMAL1/CLOCK transcriptional activity, can transcriptionally activate UCP1 expression, thereby promotes thermogenesis. Thus, loss of this UCP1-induction mechanism significantly attenuates cold tolerance in animals lacking Per2.Citation39 As Per2 suppresses Bmal1 transcriptional activity, the opposite effects observed between Bmal1 and Per2 on thermogenic response are in line with their opposing regulations in the molecular clock, suggesting that the thermogenic capacity of BAT is under a concerted control of individual molecular clock components.
Rev-erbα, a Bmal1 gene transcription repressor, also participates in the circadian control of BAT function. Gerhart-Hines et al. recently reported its role in suppressing thermogenic functions of BAT through transcriptional inhibition of UCP-143. Interestingly, we found that Rev-erbα promotes brown adipogenesis and BAT development.Citation45 These studies likely uncover distinct, yet related aspects of Rev-erbα function in BAT, one concerning the developmental control of brown fat formationCitation45 and the other of modulating its responsiveness to external stimuli.Citation43 As both contribute to the total thermogenic capacity, these layers of Rev-erbα action in BAT may reflect the complexity of long-term vs. acute regulatory roles of a temporal control mechanism in this thermogenic organ. However, due to the global ablation nature of Rev-erbα mutant, we found that these mice display sympathetic overstimulation as a result of severe lack of white adipose tissue, which may cause secondary stimulation of BAT activity. Therefore, definitive assessment of the physiological role of Rev-erbα in BAT functional regulation will require further investigations using BAT-selective ablation models.
Given these evidence of clock involvement in BAT function, dysregulation of this mechanism may affect thermogenic response and overall metabolic homeostasis. Indeed, prolonged light exposure that mimics “light pollution” attenuates the ability of the brown fat for triglyceride and glucose uptake along with diminished sympathetic signaling in this tissue,Citation28 which could account for, at least in part, the development of obesity under this setting. As many types of circadian manipulations lead to a common metabolic consequence of obesity and insulin resistance,Citation15,27 detrimental effects of circadian dys-synchrony on brown fat function could be a significant component of these metabolic disorders.
Recently, additional endocrine, lipid metabolic and inflammation-suppressing activities of the BAT have been identified.Citation46 In particular, BAT secrets cytokines such as Fgf21, Nrg4, IL-6, etc. to influence metabolic processes in other tissues. Therefore, it remains to be seen whether the clock temporal control encompass the full range of BAT activities and how these additional mechanisms may contribute to development of obesity or diabetes.
Clock regulation of brown adipocyte development
One of the direct mechanisms that link circadian disruption and obesity is the interaction of molecular clock components with adipogenic factors.Citation16,21,47 In contrast, few studies so far have examined the specific role of the molecular clock in brown adipopocyte formation. Two recent studies from our group provide initial insights into how the clock machinery exerts concerted temporal control in fine-tuning the development, and hence, the total thermogenic capacity of BAT.Citation44,45
BAT precursors share a common mesodermal origin with white adipose tissue and skeletal muscle. Based on previous findings of Bmal1 in white adipocyte and myogenic lineage differentiation,Citation21,48 we tested whether the cell-intrinsic molecular clock participates in brown adipocyte development. Given Bmal1 as an essential activator and Rev-erbα as a key repressor of the clock circuit, we chose to interrogate whether these Yin-Yang components of the clock exerts opposing actions on brown adipogenesis. Indeed, while Bmal1 suppresses brown adipocyte lineage allocation and terminal differentiation, Rev-erbα promotes these processes. Mechanistically, these functions are achieved through Bmal1 and Rev-erbα respective positive and negative transcriptional control of key components of an important inhibitory signal of brown adipocyte development, the TGF-β signaling cascade.Citation49,50 The regulation of TGF-β pathway by Bmal1 and Rev-erbα leads to suppression and promotion, respectively, of mesenchymal commitment to the brown lineage and its subsequent mature differentiation to mature adipocyte ().
Figure 2. Molecular clock control of the TGFβ signaling cascade modulates brown adipogenesis. The opposing transcriptional regulators of the molecular clock circuit, Bmal1 and Rev-erbα, exerts positive and negative transcriptional regulations of key components of the TGFβ signaling pathway, through their specific E-box and RORE elements, respectively. This regulation of TGFβ pathway, a key inhibitory mechanism of brown adipocyte development, leads to suppression and promotion of mesenchymal commitment to the brown lineage and its subsequent mature differentiation to mature adipocyte by Bmal1 and Rev-erbα, respectively.
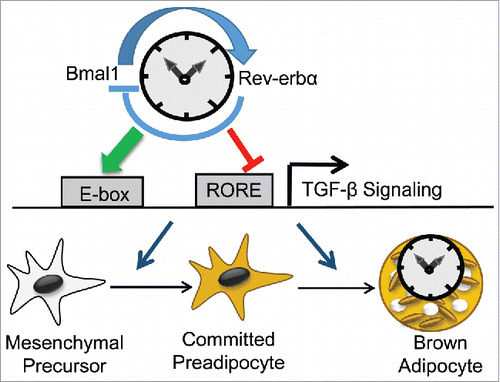
Together with previous findings,Citation21 our investigations indicate that clock machinery employs distinct developmental signaling pathways to modulate white and brown adipogenesis. In classical white adipose tissue, Bmal1 primarily regulates the canonical Wnt cascade, whereas in brown fat, clock regulation of the TGF-β pathway is predominant. Circadian regulatory elements have been identified in many developmental signaling pathways in epidermal stem cells, including Wnt, Notch, Sonic hedgehog and TGF-β/BMP.Citation51 It is postulated that temporal control of these signals synchronizes stem cell activation behavior in accordance with cyclic tissue-remodeling stimuli. Our related studies of how clock modulates muscle satellite cells proliferation and differentiation to promote skeletal muscle regeneration supports this notion,48,52 and clock regulation of stem cell properties has also been explored in diverse populations such as the skin, the hair follicle and intestine.Citation51-53 These studies, in aggregate, suggest that the clock may provide critical timing cues to orchestrate stem cell processes involved in development or tissue remodeling. The distinct signals required for transmitting temporal cues in a given tissue may thus reflect its specific developmental and functional needs.
The observed effects of clock genes in adipogenic precursors, including mesenchymal stem cells and committed preadipocytes, imply that they may function in the BAT stem/progenitor compartment and may participate in tissue remodeling involved in thermogenic recruitment by β-adrenergic and cold activation. Along this line, our recent findings implicate that the clock may play a role in the formation and functional activation of a recently identified new type of highly recruitable thermogenic fat, the subcutaneous beige fat. In this regard, generation of temporally-controlled, adipose depot-selective genetic targeting models would be required to dissect the specific roles of clock regulators in brown or beige fat formation vs. functional regulation.
BAT is increasingly recognized as a promising target for anti-obesity therapy. Thus, pharmacological targeting of the clock network in BAT could be tested for potential therapeutic applications. As a ligand-dependent nuclear receptor, Rev-erbα represents a readily “druggable” target for pharmacological intervention. Currently available Rev-erbα agonists, SR9011 and SR9009, demonstrates strong anti-obesity efficacy in mice.Citation54 In brown adipocytes, we have tested the effects of pharmacological activation and inhibition of Rev-erbα by small molecule modulators.Citation55 In agreement with findings from genetic manipulation, Rev-erbα agonist SR9011 significantly promotes brown adipocyte differentiation, whereas the antagonist SR8278 exhibits the opposite effect. Considering the beneficial effect of SR9011 on lowering lipids and protecting against obesity,Citation54 its regulation of BAT metabolism could be part of the global beneficial effect, which warrants further investigation. Further, based on the high bioavailability of synthetic Rev-erbα ligands, these agents are not only useful chemical tools for interrogation of Rev-erbα function in vivo, they also represent prototype molecules for targeted clock therapies in the future.
Future perspectives
The past few years yield a wealth of knowledge regarding the complex regulatory mechanisms governing BAT development and function. Based on the current progress and our recent studies, future investigation of how the clock circuit functions to confer a timing cue to modulate BAT function, and how these actions may feed into thermoregulatory control and energy balance are intriguing new avenues to pursue. One important aspect is to identify upstream signals responsible for transmitting temperature, particularly cold cues, to the molecular clock in BAT. Cold-induced sympathetic activation is transmitted in brown adipocytes through the PKA-cAMP-CREB pathway.Citation56 It is thus conceivable that the adrenergic-cAMP cascade may feed into the clock network to facilitate a temporally coordinated thermogenic response. Characterization of the precise nature of upstream activation pathways of BAT clock may yield novel targets for future therapeutic interventions.
Another area of great interest is to further dissect the molecular links that are responsible for the well-recognized association between circadian disruption and development of obesity. Based on insights gleaned from recent studies, dysfunctions in circadianly-controlled brown fat activity may contribute to this phenomenon, and thus, the clock mechanism in BAT may be pharmacologically targeted to counteract obesity. Based on its “druggable” nature, Rev-erbα represents an entry point of the clock circuit for therapeutic interventions, although additional factors may also prove to be amenable to small-molecule regulations. Given the demonstrated strong anti-obesity efficacies of Rev-erbα agonists,Citation54 therapeutic targeting of clock regulators has enormous potentials for the prevention or treatment of metabolic diseases.
Disclosure of potential conflicts of interest
No potential conflicts of interest were disclosed.
Funding
This work was supported by American Heart Association grant 12SDG12080076, American Diabetes Association grant 1-13-BS-118 to KM, and American Diabetes Association grant 7-12-BS-210 and National Institute of Health grant DK097160-01 to VY.
References
- Dibner C, Schibler U, Albrecht U. The mammalian circadian timing system: organization and coordination of central and peripheral clocks. Annu Rev Physiol 2010; 72:517-49; PMID:20148687; http://dx.doi.org/10.1146/annurev-physiol-021909-135821
- Reppert SM, Weaver DR. Coordination of circadian timing in mammals. Nature 2002; 418:935-41; PMID:12198538; http://dx.doi.org/10.1038/nature00965
- Schibler U, Sassone-Corsi P. A web of circadian pacemakers. Cell 2002; 111:919-22; PMID:12507418; http://dx.doi.org/10.1016/S0092-8674(02)01225-4
- Rey G, Cesbron F, Rougemont J, Reinke H, Brunner M, Naef F. Genome-Wide and Phase-Specific DNA-Binding Rhythms of BMAL1 Control Circadian Output Functions in Mouse Liver. PLoS Biol 2011; 9:e1000595; PMID:21364973; http://dx.doi.org/10.1371/journal.pbio.1000595
- Stratmann M, Schibler U. REV-ERBs: more than the sum of the individual parts. Cell Metab 2012; 15:791-3; PMID:22682217; http://dx.doi.org/10.1016/j.cmet.2012.05.006
- Preitner N, Damiola F, Lopez-Molina L, Zakany J, Duboule D, Albrecht U, Schibler U. The orphan nuclear receptor REV-ERBalpha controls circadian transcription within the positive limb of the mammalian circadian oscillator. Cell 2002; 110:251-60; PMID:12150932; http://dx.doi.org/10.1016/S0092-8674(02)00825-5
- Cannon B, Nedergaard J. Brown adipose tissue: function and physiological significance. Physiol Rev 2004; 84:277-359; PMID:14715917; http://dx.doi.org/10.1152/physrev.00015.2003
- Yoneshiro T, Aita S, Matsushita M, Kayahara T, Kameya T, Kawai Y, Iwanaga T, Saito M. Recruited brown adipose tissue as an antiobesity agent in humans. J Clin Invest 2013; 123:3404-8; PMID:23867622; http://dx.doi.org/10.1172/JCI67803
- Stanford KI, Middelbeek RJ, Townsend KL, An D, Nygaard EB, Hitchcox KM, Markan KR, Nakano K, Hirshman MF, Tseng YH, et al. Brown adipose tissue regulates glucose homeostasis and insulin sensitivity. J Clin Invest 2013; 123:215-23; PMID:23221344; http://dx.doi.org/10.1172/JCI62308
- Bartelt A, Bruns OT, Reimer R, Hohenberg H, Ittrich H, Peldschus K, Kaul MG, Tromsdorf UI, Weller H, Waurisch C, et al. Brown adipose tissue activity controls triglyceride clearance. Nat Med 2011; 17:200-5; PMID:21258337; http://dx.doi.org/10.1038/nm.2297
- Bass J, Takahashi JS. Circadian integration of metabolism and energetics. Science 2010; 330:1349-54; PMID:21127246; http://dx.doi.org/10.1126/science.1195027
- Panda S, Antoch MP, Miller BH, Su AI, Schook AB, Straume M, Schultz PG, Kay SA, Takahashi JS, Hogenesch JB. Coordinated transcription of key pathways in the mouse by the circadian clock. Cell 2002; 109:307-20; PMID:12015981; http://dx.doi.org/10.1016/S0092-8674(02)00722-5
- Eckel-Mahan KL, Patel VR, Mohney RP, Vignola KS, Baldi P, Sassone-Corsi P. Coordination of the transcriptome and metabolome by the circadian clock. Proc Natl Acad Sci U S A 2012; 109:5541-6; PMID:22431615; http://dx.doi.org/10.1073/pnas.1118726109
- Turek FW, Joshu C, Kohsaka A, Lin E, Ivanova G, McDearmon E, Laposky A, Losee-Olson S, Easton A, Jensen DR, et al. Obesity and metabolic syndrome in circadian Clock mutant mice. Science 2005; 308:1043-5; PMID:15845877; http://dx.doi.org/10.1126/science.1108750
- Karatsoreos IN, Bhagat S, Bloss EB, Morrison JH, McEwen BS. Disruption of circadian clocks has ramifications for metabolism, brain, and behavior. Proc Natl Acad Sci U S A 2011; 108:1657-62; PMID:21220317; http://dx.doi.org/10.1073/pnas.1018375108
- Grimaldi B, Bellet MM, Katada S, Astarita G, Hirayama J, Amin RH, Granneman JG, Piomelli D, Leff T, Sassone-Corsi P. PER2 controls lipid metabolism by direct regulation of PPARgamma. Cell Metab 2010; 12:509-20; PMID:21035761; http://dx.doi.org/10.1016/j.cmet.2010.10.005
- Pan A, Schernhammer ES, Sun Q, Hu FB. Rotating night shift work and risk of type 2 diabetes: two prospective cohort studies in women. PLoS Med 2011; 8:e1001141; PMID:22162955; http://dx.doi.org/10.1371/journal.pmed.1001141
- Parkes KR. Shift work and age as interactive predictors of body mass index among offshore workers. Scand J Work Environ Health 2002; 28:64-71; PMID:11871855; http://dx.doi.org/10.5271/sjweh.648
- Scheer FA, Hilton MF, Mantzoros CS, Shea SA. Adverse metabolic and cardiovascular consequences of circadian misalignment. Proc Natl Acad Sci U S A 2009; 106:4453-8; PMID:19255424; http://dx.doi.org/10.1073/pnas.0808180106
- Lamia KA, Storch KF, Weitz CJ. Physiological significance of a peripheral tissue circadian clock. Proc Natl Acad Sci U S A 2008; 105:15172-7; PMID:18779586; http://dx.doi.org/10.1073/pnas.0806717105
- Guo B, Chatterjee S, Li L, Kim JM, Lee J, Yechoor VK, Minze LJ, Hsueh W, Ma K. The clock gene, brain and muscle Arnt-like 1, regulates adipogenesis via Wnt signaling pathway. FASEB J 2012; 26:3453-63; PMID:22611086; http://dx.doi.org/10.1096/fj.12-205781
- Lee J, Kim MS, Li R, Liu VY, Fu L, Moore DD, Ma K, Yechoor VK. Loss of Bmal1 leads to uncoupling and impaired glucose-stimulated insulin secretion in β-cells. Islets 2011; 3:381-8; PMID:22045262; http://dx.doi.org/10.4161/isl.3.6.18157
- Goumidi L, Grechez A, Dumont J, Cottel D, Kafatos A, Moreno LA, Molnar D, Moschonis G, Gottrand F, Huybrechts I, et al. Impact of REV-ERB α gene polymorphisms on obesity phenotypes in adult and adolescent samples. Int J Obes (Lond) 2014; 37:666-72; http://dx.doi.org/10.1038/ijo.2012.117
- Fontaine C, Dubois G, Duguay Y, Helledie T, Vu-Dac N, Gervois P, Soncin F, Mandrup S, Fruchart JC, Fruchart-Najib J, et al. The orphan nuclear receptor Rev-Erbalpha is a peroxisome proliferator-activated receptor (PPAR) gamma target gene and promotes PPARgamma-induced adipocyte differentiation. J Biol Chem 2003; 278:37672-80; PMID:12821652; http://dx.doi.org/10.1074/jbc.M304664200
- Roenneberg T, Allebrandt KV, Merrow M, Vetter C. Social jetlag and obesity. Curr Biol 2012; 22:939-43; PMID:22578422; http://dx.doi.org/10.1016/j.cub.2012.03.038
- Coomans CP, van den Berg SA, Houben T, van Klinken JB, van den Berg R, Pronk AC, Havekes LM, Romijn JA, van Dijk KW, Biermasz NR, et al. Detrimental effects of constant light exposure and high-fat diet on circadian energy metabolism and insulin sensitivity. FASEB J 2013; 27:1721-32; PMID:23303208; http://dx.doi.org/10.1096/fj.12-210898
- Fonken LK, Aubrecht TG, Melendez-Fernandez OH, Weil ZM, Nelson RJ. Dim light at night disrupts molecular circadian rhythms and increases body weight. J Biol Rhythms 2013; 28:262-71; PMID:23929553; http://dx.doi.org/10.1177/0748730413493862
- Kooijman S, van den Berg R, Ramkisoensing A, Boon MR, Kuipers EN, Loef M, Zonneveld TC, Lucassen EA, Sips HC, Chatzispyrou IA, et al. Prolonged daily light exposure increases body fat mass through attenuation of brown adipose tissue activity. Proc Natl Acad Sci U S A 2015; 112: 6748-53; PMID:25964318; http://dx.doi.org/10.1073/pnas.1504239112.
- Herrero L, Valcarcel L, da Silva CA, Albert N, Diez-Noguera A, Cambras T, Serra D. Altered circadian rhythm and metabolic gene profile in rats subjected to advanced light phase shifts. PLoS One 2015; 10:e0122570; PMID:25837425; http://dx.doi.org/10.1371/journal.pone.0122570
- Millar AJ. Input signals to the plant circadian clock. J Exp Bot 2004; 55:277-83; PMID:14695902; http://dx.doi.org/10.1093/jxb/erh034
- Buhr ED, Yoo SH, Takahashi JS. Temperature as a universal resetting cue for mammalian circadian oscillators. Science 2010; 330:379-85; PMID:20947768; http://dx.doi.org/10.1126/science.1195262
- Romanovsky AA. Thermoregulation: some concepts have changed. Functional architecture of the thermoregulatory system. Am J Physiol Regul Integr Comp Physiol 2007; 292:R37-46; PMID:17008453; http://dx.doi.org/10.1152/ajpregu.00668.2006
- Refinetti R. The circadian rhythm of body temperature. Front Biosci (Landmark Ed) 2010; 15:564-94; PMID:20036834; http://dx.doi.org/10.2741/3634
- van der Veen DR, Shao J, Chapman S, Leevy WM, Duffield GE. A Diurnal Rhythm in Glucose Uptake in Brown Adipose Tissue Revealed by In Vivo PET-FDG Imaging. Obesity (Silver Spring) 2012; 20:1527-9; PMID:22447290
- Bartness TJ, Song CK, Demas GE. SCN efferents to peripheral tissues: implications for biological rhythms. J Biol Rhythms 2001; 16:196-204; PMID:11407779; http://dx.doi.org/10.1177/074873040101600302
- Amir S. Retinohypothalamic tract stimulation activates thermogenesis in brown adipose tissue in the rat. Brain Res 1989; 503:163-6; PMID:2611651; http://dx.doi.org/10.1016/0006-8993(89)91720-4
- Zvonic S, Ptitsyn AA, Conrad SA, Scott LK, Floyd ZE, Kilroy G, Wu X, Goh BC, Mynatt RL, Gimble JM. Characterization of peripheral circadian clocks in adipose tissues. Diabetes 2006; 55:962-70; PMID:16567517; http://dx.doi.org/10.2337/diabetes.55.04.06.db05-0873
- Li S, Yu Q, Wang GX, Lin JD. The biological clock is regulated by adrenergic signaling in brown fat but is dispensable for cold-induced thermogenesis. PLoS One 2013; 8:e70109; PMID:23990898; http://dx.doi.org/10.1371/journal.pone.0070109
- Chappuis S, Ripperger JA, Schnell A, Rando G, Jud C, Wahli W, Albrecht U. Role of the circadian clock gene Per2 in adaptation to cold temperature. Mol Metab 2013; 2:184-93; PMID:24049733; http://dx.doi.org/10.1016/j.molmet.2013.05.002
- Vesala L, Salminen TS, Kankare M, Hoikkala A. Photoperiodic regulation of cold tolerance and expression levels of regucalcin gene in Drosophila montana. J Insect Physiol 2012; 58:704-9; PMID:22360999; http://dx.doi.org/10.1016/j.jinsphys.2012.02.004
- Demas GE, Bowers RR, Bartness TJ, Gettys TW. Photoperiodic regulation of gene expression in brown and white adipose tissue of Siberian hamsters (Phodopus sungorus). Am J Physiol Regul Integr Comp Physiol 2002; 282:R114-121; PMID:11742829
- Au-Yong IT, Thorn N, Ganatra R, Perkins AC, Symonds ME. Brown adipose tissue and seasonal variation in humans. Diabetes 2009; 58:2583-7; PMID:19696186; http://dx.doi.org/10.2337/db09-0833
- Gerhart-Hines Z, Feng D, Emmett MJ, Everett LJ, Loro E, Briggs ER, Bugge A, Hou C, Ferrara C, Seale P, et al. The nuclear receptor Rev-erbalpha controls circadian thermogenic plasticity. Nature 2013; 503:410-3; PMID:24162845; http://dx.doi.org/10.1038/nature12642
- Nam D, Guo B, Chatterjee S, Chen MH, Nelson D, Yechoor VK, Ma K. The adipocyte clock controls brown adipogenesis through the TGF-β and BMP signaling pathways. J Cell Sci 2015; 128:1835-47; PMID:25749863; http://dx.doi.org/10.1242/jcs.167643
- Nam D, Chatterjee S, Yin H, Liu R, Lee J, Yechoor VK, Ma K. Novel Function of Rev-erbalpha in Promoting Brown Adipogenesis. Sci Rep 2015; 5:11239; PMID:26058812; http://dx.doi.org/10.1038/srep11239
- Wang GX, Zhao XY, Lin JD. The brown fat secretome: metabolic functions beyond thermogenesis. Trends Endocrinol Metab 2015; 26:231-7; PMID:25843910; http://dx.doi.org/10.1016/j.tem.2015.03.002
- Marcheva B, Ramsey KM, Buhr ED, Kobayashi Y, Su H, Ko CH, Ivanova G, Omura C, Mo S, Vitaterna MH, et al. Disruption of the clock components CLOCK and BMAL1 leads to hypoinsulinaemia and diabetes. Nature 2010; 466:627-31; PMID:20562852; http://dx.doi.org/10.1038/nature09253
- Chatterjee S, Nam D, Guo B, Kim JM, Winnier GE, Lee J, Berdeaux R, Yechoor VK, Ma K. Brain and muscle Arnt-like 1 is a key regulator of myogenesis. J Cell Sci 2013; 126:2213-24; PMID:23525013; http://dx.doi.org/10.1242/jcs.120519
- Koncarevic A, Kajimura S, Cornwall-Brady M, Andreucci A, Pullen A, Sako D, Kumar R, Grinberg AV, Liharska K, Ucran JA, et al. A novel therapeutic approach to treating obesity through modulation of TGFbeta signaling. Endocrinology 2012; 153:3133-46; PMID:22549226; http://dx.doi.org/10.1210/en.2012-1016
- Yadav H, Quijano C, Kamaraju AK, Gavrilova O, Malek R, Chen W, Zerfas P, Zhigang D, Wright EC, Stuelten C, et al. Protection from obesity and diabetes by blockade of TGF-β/Smad3 signaling. Cell Metab 2011; 14:67-79; PMID:21723505; http://dx.doi.org/10.1016/j.cmet.2011.04.013
- Janich P, Pascual G, Merlos-Suarez A, Batlle E, Ripperger J, Albrecht U, Obrietan K, Di Croce L, Benitah SA. The circadian molecular clock creates epidermal stem cell heterogeneity. Nature 2011; 480: 209-14.
- Chatterjee S, Yin H, Nam D, Li Y, Ma K. Brain and muscle Arnt-like 1 promotes skeletal muscle regeneration through satellite cell expansion. Exp Cell Res 2015; 331:200-10; PMID:25218946; http://dx.doi.org/10.1016/j.yexcr.2014.08.041
- Karpowicz P, Zhang Y, Hogenesch JB, Emery P, Perrimon N. The circadian clock gates the intestinal stem cell regenerative state. Cell Rep 2013; 3:996-1004; PMID:23583176; http://dx.doi.org/10.1016/j.celrep.2013.03.016
- Plikus MV, Vollmers C, de la Cruz D, Chaix A, Ramos R, Panda S, Chuong CM. Local circadian clock gates cell cycle progression of transient amplifying cells during regenerative hair cycling. Proc Natl Acad Sci U S A 2013; 110:E2106-15; PMID:23690597; http://dx.doi.org/10.1073/pnas.1215935110
- Solt LA, Wang Y, Banerjee S, Hughes T, Kojetin DJ, Lundasen T, Shin Y, Liu J, Cameron MD, Noel R, et al. Regulation of circadian behaviour and metabolism by synthetic REV-ERB agonists. Nature 2012; 485:62-8; PMID:22460951; http://dx.doi.org/10.1038/nature11030
- Robidoux J, Martin TL, Collins S. Beta-adrenergic receptors and regulation of energy expenditure: a family affair. Annu Rev Pharmacol Toxicol 2004; 44:297-323; PMID:14744248; http://dx.doi.org/10.1146/annurev.pharmtox.44.101802.121659