abstract
The notion that the obesogenic potential of high fat diets in rodents is attenuated when the protein:carbohydrate ratio is increased is largely based on studies using casein or whey as the protein source. We fed C57BL/6J mice high fat-high protein diets using casein, soy, cod, beef, chicken or pork as protein sources. Casein stood out as the most efficient in preventing weight gain and accretion of adipose mass. By contrast, mice fed diets based on pork or chicken, and to a lesser extent mice fed cod or beef protein, had increased adipose tissue mass gain relative to casein fed mice. Decreasing the protein:carbohydrate ratio in diets with casein or pork as protein sources led to accentuated fat mass accumulation. Pork fed mice were more obese than casein fed mice, and relative to casein, the pork-based feed induced substantial accumulation of fat in classic interscapular brown adipose tissue accompanied by decreased UCP1 expression. Furthermore, intake of a low fat diet with casein, but not pork, as a protein source reversed diet-induced obesity. Compared to pork, casein seems unique in maintaining the classical brown morphology in interscapular brown adipose tissue with high UCP1 expression. This was accompanied by increased expression of genes involved in a futile cycling of fatty acids. Our results demonstrate that intake of high protein diets based on other protein sources may not have similar effects, and hence, the obesity protective effect of high protein diets is clearly modulated by protein source.
Background
High protein diets have become increasingly popular as a mean to prevent obesity development and lose weight.Citation1–4 Yet, solid evidence as to what extent different protein sources differ in their ability to counteract obesity development is still lacking.Citation5 However, a few studies where rodents were fed diets with standard levels (i.e 18–20 energy %) of proteins indicated that different protein sources have different obesogenic potential.Citation6–12 Nevertheless, the general notion that high protein diets attenuate obesity in rodents is almost exclusively based on studies where milk proteins are used as protein sources.Citation13–22 However, protein sources for human consumption would normally comprise fish and meat. Compared with proteins from fish and meat, casein and whey have a high content of branched chain amino acids (BCAAs), valine, leucine and isoleucine. In particular, leucine is recognized as a nutrient signal proposed to mediate, at least in part, the effect of high protein diets on metabolism.Citation23–26 Thus, experiments with a high dietary protein:carbohydrate ratio using whey and casein may not be representative for high protein diets in general.
There are several mechanisms by which diets with a high content of casein or whey may prevent high fat diet-induced obesity. A high intake of proteins is in general known to have high satiating effect, thereby reducing energy intake,Citation1,3,4 but importantly, pair-feeding experiments have demonstrated that diets with a high content of casein also reduce feed efficiency and obesity development independently of feed intake.Citation18,19,21 The reduced energy efficiency may relate to the higher thermic effect of proteins (20–30%) compared with carbohydrates (5–10%).Citation27 Furthermore, high protein intake is associated with augmented energy expenditure due to an increase in ATP-consuming processes such as protein degradation and re-synthesis, as well as synthesis of urea. Moreover, if the dietary carbohydrate level is low, ATP is required for gluconeogenesis.Citation28,29 Mitochondrial ATP synthesis may be impaired by the uncoupling protein 1 (UCP1) expressed in brown or brite (brown-in-white) adipocytes, also known as beige adipocytes that can be found together with white adipocytes in several fat depots.Citation30 As UCP1 allows energy to be dissipated in the form of heat, its expression is positively correlated with metabolic inefficiency.Citation31 The number of UCP1-expressing adipocytes in different adipose depots varies between mouse strains and may account for their different propensity for diet-induced obesity.Citation32,33 Furthermore, Ucp1 expression is upregulated in both mice and rats by several pharmacological and nutritional agents,Citation34 including high protein diets.Citation18,19,21,35 Of note, milk based protein sources were used in all these experiments.
A more brown phenotype of typically white adipose tissue with a concomitant resistance to diet-induced obesity can also be obtained by transgenic expression of UCP1 itself Citation36 or by modulation of several key molecules involved in brown adipocyte differentiation.Citation37 For instance, the lean phenotype of mice lacking NRIP1 is accompanied by increased Ucp1 expression in white adipose tissue.Citation38 These mice are of particular interest, as loss of NRIP1 not only leads to dissipation of energy via UCP1-dependent uncoupled respiration, but also leads to energy loss by increasing expression of genes involved in a futile cycle of triacylglycerol breakdown and synthesis.Citation39 Activation of this futile cycle was earlier reported to be activated by thiazolinediones and requires induction of glycerol kinase (Gyk).Citation40 Interestingly, this futile cycle may be activated without a concomitant upregulation of UCP1, and may play a key role in regulation of the metabolic flexibility of both adipocytes and the whole organism.Citation41 It is not yet known if high protein diets can modulate such a futile cycle.
Since (i) the protein source in diets with standard protein levels may influence both Ucp1 expression and obesity development, and (ii) the perception that high protein diets protect against obesity development almost exclusively is based on studies using milk proteins as a protein source, we here aimed to evaluate the development of obesity in mice fed high fat diets with a high protein:carbohydrate ratio using different protein sources.
Results
High fat high protein diets based on cod, beef, chicken and pork are obesogenic
In order to investigate whether a high protein:carbohydrate ratio attenuates high fat diet-induced obesity when feed based on isolated casein powder is replaced by feed based on other protein sources, we prepared diets using a soy protein isolate or freezed dried muscle fillets from cod, beef, chicken or pork as the protein sources. Before preparing the diets we measured the levels of fat and protein, and determined the amino acid compositions of the different protein sources ( and ). As the protein content varied between the different protein sources, the amino acid compositions are presented as % of total amino acids to facilitate comparison. As expected, the levels of BCAAs were higher in isolated casein than in the other protein sources (). Moreover, proteins from cod or meat also had a lower level of phenylalanine than casein. Furthermore casein had lower levels of arginine, glycine and cysteine, but higher levels of glutamic acid + glutamine, proline and tyrosine than the other protein sources (). As expected, the soy-isolate contained less methionine and lysine than the other sources (), but the sulfur-amino acid requirement for rodentsCitation42 was met
Table 1. Compositions of the different protein sources.
Table 2. Composition of the high fat high sucrose diets and the high fat high protein diets with different protein sources.
Given the varying content of nitrogen in the different protein sources, different amounts of these were required to prepare isonitrogenous diets (). Furthermore, as also the levels of endogenous fat in the different protein sources varied, adjustment of the fat content was required to prepare isoenergetic diets (). Accordingly, we measured the fatty acid compositions in the diets (). Since beef contained more endogenous fat than the other protein sources, less corn oil was used in the preparation of the beef-containing diet (). Thus, the beef-based diet contained more saturated fat and less n-6 polyunsaturated fatty acid (PUFA) linoleic acid than the other diets (). As expected, the marine n-3 PUFAs, eicosapentaenoic acid (EPA) and docosahexaenoic acid (DHA) were present in the cod based diet, but although at lower levels, they were also present in the diets prepared using beef, chicken and pork ().
Table 3. Fatty acid compositions of the high fat high protein diets with different protein sources.
In agreement with our earlier studies,Citation18,19,21 the mice fed the high fat, high casein diet did not gain more body mass than mice fed a low fat reference diet (). Mice fed a high proportion of casein protein had significantly lower eWAT and iWAT masses than mice fed the casein-based HF/HS reference diet (). Fat masses in mice fed a high proportion of soy and cod did not differ significantly from those fed the casein-based HF/HS diet (). Strikingly, compared with mice fed the casein-based HF/HS diet, mice fed diets with high content of proteins from beef, chicken and pork had significantly higher fat masses ().
Figure 1. Effect of high fat high protein diets with different protein sources on body mass, body composition and digestibility. Male C57BL/6J mice were fed a high fat diet with a low protein:carbohydrate ratio (HF/HS) using casein as the protein source or high fat diets with a high protein:carbohydrate ratio (HF/HP) using different protein sources for 11 weeks. Mice fed a low fat regular diet (RD) were used as a reference. (A), Body mass development was recorded during the first 8 weeks. B-C , After 11 weeks epididymal white adipose tissue (eWAT) (B) and inguinal white adipose tissue (iWAT) (C) were dissected out and their masses recorded. D-E, Energy intake (D) and feed efficiency (E) were calculated based on data collected during the first 8 weeks of feeding. F-G, Apparent fat (F) and nitrogen (G) digestibility were calculated based on feed intake and feces collection during the 7th week of feeding. Data represent mean ± SEM (n = 9). Different small letters denote significant differences between the groups (p < 0 .05).
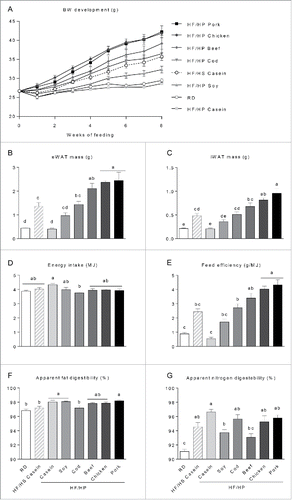
Replacement of casein with cod, but not any other protein sources, led to a reduced feed intake (). Consequently, compared with casein, feed efficiency was higher with all other protein sources tested, with the exception of soy (). Based on intake and excretion, the apparent digestibility of fat in the cod containing diet was lower than in the casein based diet (), and the apparent nitrogen digestibility of soy and beef protein was lower than of casein (). Importantly however, the high obesogenic potential of the pork- and chicken-based diets did not correlate with increased fat or nitrogen digestibility.
Protein source and amount modulate the obesogenic effect of high fat diets
To further analyze the influence of protein intake and source, we performed a second experiment using a factorial design with protein:carbohydrate ratio and protein source as categorical predictors. Mice fed casein and pork represented the extremes in experiment 1, and therefore casein and pork were chosen as protein sources. As expected, body weight gain and fat mass, as determined by nuclear magnetic resonance (NMR), in mice fed a high proportion of casein were comparable to mice fed the low fat reference diet after 8 weeks of feeding (), also verified by dissection of different adipose tissue depots (not shown). Both protein level and source influenced on body weight gain and fat mass (), whereas lean masses were comparable (). Casein fed mice were less obese than pork fed mice, but increasing the protein:carbohydrate ratio attenuated obesity development irrespectively of the protein source (). As feed intake was similar in all 4 groups (data not shown), calculation of feed efficiency mirrored body weight gain (). Feed efficiency was, however, not directly linked to digestibility, as fat digestibility was higher following intake of the casein-based feed with a high protein:carbohydrate ratio compared to intake of a feed with low protein:carbohydrate ratio (). No differences in fat digestibility were observed comparing mice fed the pork-based feed (). Furthermore, apparent nitrogen digestibility was also higher in mice fed a high protein:carbohydrate ratio diet, independent of protein source (). Thus, feed efficiency was not directly related to digestibility of neither fat nor nitrogen.
Figure 2. Effect of high fat diets with high and low protein:carbohydrate ratios on body mass gain, body composition and digestibility. Male C57BL/6J mice were fed a high fat diet with a low protein:carbohydrate ratio (HF/HS) or a high protein:carbohydrate ratio (HF/HP) using casein or pork as protein sources for 11 weeks. The mice were feed-deprived 4 h before blood collection and termination. (A), Body mass development was recorded and body weight (BW) gain calculated during the first 8 weeks of the trial. B-C, Fat mass (B) and lean mass (C) were measured after 8 weeks of feeding. D, Feed efficiency was calculated based on data collected during the first 8 weeks of feeding. E-F, Apparent fat (E) and nitrogen (F) digestibility were calculated based on feed intake and feces collection during the 7th week of feeding. G-I, Plasma levels of urea (G), hydroxybutyrate (H) and free fatty acids (FFAs) (I) were measured in plasma collected at termination. Data represent mean ± SEM (n = 9). Significant differences (p < 0 .05) between the protein sources are presented with different letters and differences between high and low protein:carbohydrate ratio with*.
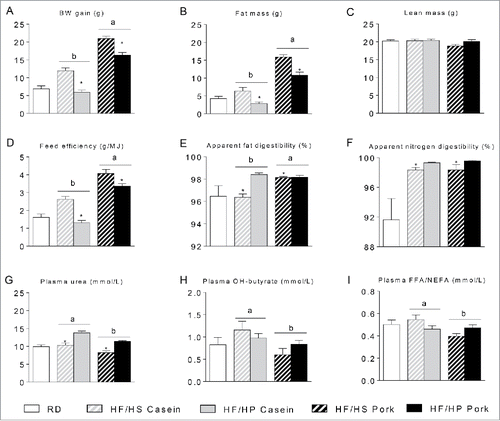
Glucose tolerance and insulin sensitivity mirrored fat mass in the mice (Fig. S1). Thus, decreasing the protein:carbohydrate ratio impaired insulin sensitivity and glucose tolerance. However, mice fed pork-based diets exhibited a more pronounced impairment in insulin sensitivity and glucose tolerance than mice fed the casein-based diets (Fig. S1). The observed chronic elevated levels of BCAAs in mice with disrupted mitochondrial branched chain aminotransferase, BCATm-KO mice, have been associated with increased energy expenditure, protection against diet-induced obesity and improved insulin sensitivity.Citation43 Compared with pork protein, the levels of BCAAs in casein are higher (). Interestingly, analysis of the amino acid profile in plasma collected from mice that were feed deprived for 4 h revealed that the circulating levels of Val, Leu and Ile were indeed higher in casein than in pork fed mice ().
Table 4. Plasma concentrations of amino acids in mice fed high fat high sucrose (HF/HS) or high fat high protein (HF/HP) diets with casein or pork as the protein source.
Plasma levels of urea and hydroxybutyrate were higher in mice fed casein than in mice fed pork, suggesting that catabolism of both protein and fat were higher in casein than in pork fed mice (). Moreover, plasma levels of free fatty acids (FFAs) were higher in casein than in pork fed mice, suggesting increased lipolysis (). The protein:carbohydrate ratio did not modulate plasma levels of FFAs or hydroxybutyrate, but as expected, plasma levels of urea were higher in mice fed a high proportion of proteins ().
We utilized indirect calorimetry to evaluate diet-induced differences in metabolism between HF/HS or HF/HP diets using casein or pork as protein sources. To determine changes independent of those associated with weight gain, these analyses were performed during the period of 24–72h after the shift to the experimental HF/HS or HF/HP diets. Body weight, calorimetry and activity measurements of the mice on low fat reference diet before the shift to experimental diets showed no differences between groups (Fig. S2). After the shift to experimental diets, we observed a tendency toward increased total activity in the mice fed casein compared to mice fed pork (p = 0.072) over the 48 h period (). Energy expenditure (EE), illustrated as EC50 of the percent relative cumulative frequency (PRCF), showed comparable values between the 4 groups (). However, an effect of protein source was observed on the EE hill slope, indicating a larger EE range for the casein-fed mice (). As expected mice fed the HF/HP diets had lower RER compared to those fed HF/HS diets (), hence less carbohydrates were used as energy source. In addition, the protein source had significant effects on the RER hill slope, indicating higher metabolic flexibility in the casein-fed mice ().
Figure 3. Effect of high fat diets with high and low protein:carbohydrate ratios on activity, energy expenditure (EE) and respiratory exchange ratio (RER). Male C57BL/6J mice were fed high fat diets with a low protein:carbohydrate ratio (HF/HS) or a high protein:carbohydrate ratio (HF/HP) using casein or pork as protein sources. 48 hours measurements of activity (A) with total number of beam breaks (B). PRCF analysis of EE (C) and RER (F) over 48 hours with graphs illustrating the mean value. Based on the PRCF data EC50 (D,G) and hill slope (E,H) were calculated. Data represent mean ± SEM (n = 4). Significant difference (p < 0 .05) between the protein sources are presented with different letters and differences between high and low protein:carbohydrate ratio with *.
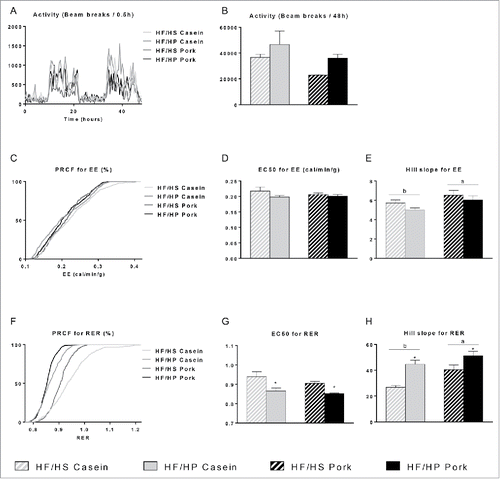
Protein source and protein:carbohydrate ratio modulate iBAT morphology and expression of genes involved in futile cycling of fatty acids
To investigate whether an increased protein:carbohydrate ratio leads to an increased number of brite adipocytes in iWAT, histological examinations were performed. Increasing the protein:carbohydrate ratio led to smaller adipocytes in iWAT in mice fed either the casein-based or the pork-based HF/HP diets compared to mice fed the corresponding HF/HS diets (). Of note, the adipocytes in iWAT of casein fed mice were smaller than adipocytes from pork fed mice comparing either the HF/HS or the HF/HP diets (). Expression levels of marker genes for brown/brite adipocytes in iWAT were not significantly altered by either the type or amount of dietary protein (). UCP1 was not detectable by neither immunohistochemistry nor Western blotting in iWAT (not shown).
Figure 4. Effect of high fat diets with high and low protein:carbohydrate ratios on histological appearance and gene expression in inguinal white adipose tissue (iWAT). Male C57BL/6J mice were fed a high fat diet with a low protein:carbohydrate ratio (HF/HS) or a high protein:carbohydrate ratio (HF/HP) using casein or pork as protein sources for 11 weeks. The mice were feed-deprived 4h before termination. (A) Sections of iWAT (n = 4) were stained with eosin and hematoxylin, scalebar = 100 μm and (B) mean diameter of the adipocytes was measured and data represent mean ± SEM. (C) Expression levels of uncoupling protein 1 (Ucp1), deiodinase, iodothyronine, type II (Dio2), peroxisome proliferator-activated receptor-γ coactivator 1α (Ppargc1a) and cell death-inducing DFFA-like effector (Cidea) were measured and expression levels were normalized to TATA-box binding protein (Tbp). Gene-expression data represent mean ± SEM (n = 9). Significant difference (p < 0 .05) between the protein sources are presented with different letters and differences between high and low protein:carbohydrate ratio with *.
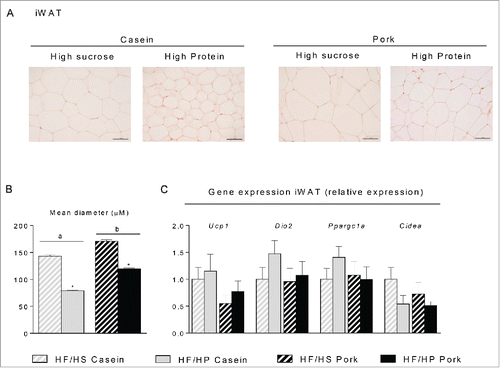
Histological examination of iBAT revealed that only adipocytes from mice fed a high proportion of casein maintained a classic brown phenotype with multilocular lipid droplets (). Mice fed a low protein:carbohydrate ratio, in particular those fed pork, had large “white-like” adipocytes with a single large lipid droplet in the iBAT depot (). At the protein level, immunohistochemical analyses demonstrated that a higher proportion of adipocytes in casein compared to pork fed mice stained positive for UCP1 (). This was verified by Western blotting (). Thus, our data indicate that maintenance of a classical brown morphology of adipocytes in the interscapular region by a high proportion of casein may contribute to the observed protection against diet-induced obesity. Contrasting the differences in UCP1 protein levels, we observed no significant differences in the levels of RNAs encoding UCP1 or other markers of enhanced thermogenesis in relation to protein source and amount in iBAT ( and ) suggesting that regulation of UCP1 levels in this setting may reflect differences in posttranscriptional processes.
Figure 5. Effect of high fat diets with high and low protein:carbohydrate ratios on histological appearance, protein and gene expression in interscapular brown adipose tissue (iBAT). Male C57BL/6J mice were fed a high fat diet with a low protein:carbohydrate ratio (HF/HS) or a high protein:carbohydrate ratio (HF/HP) using casein or pork as protein sources for 11 weeks. The mice were feed-deprived 4 h before termination. (A) Sections of iBAT (n = 4) were stained with eosin and hematoxylin (H&E), scalebar = 100 μm and (B) Sections of iBAT (n = 4) were immunohistologically stained with an UCP1-antibody and (C) the area quantified, scalebar = 200μm. (D) UCP1 was detected by Western-blotting (n = 6) and the expression levels were quantified. (E) Expressions of uncoupling protein 1 (Ucp1) and Leptin were measured in addition to genes involved in fatty acid oxidation, carnitine palmitoyltransferase 1b (Cpt1b), acyl-Coenzyme A dehydrogenase, medium chain (Acadm) and acyl-Coenzyme A oxidase 1 (Acox1), fatty acid synthesis, fatty acid synthase (Fasn), stearoyl-Coenzyme A desaturase 1 (Scd1) and elongation of very long chain fatty acids (Elovl3) and triacylglycerol synthesis- and breakdown, microsomal CoA:glycerol-3-phosphate acyltransferase 4 (Gpat4), diacylglycerol acyltransferase 1 (Dgat 1), glycerol kinase (Gyk) glycerol-3-phosphate dehydrogenase 1 (Gpd1) and nuclear receptor interacting protein 1 (Nrip1). Expression levels were normalized to TATA-box binding protein (Tbp). Gene expression data represent mean ± SEM (n = 9). Significant difference (p < 0 .05) between the protein sources are presented with different letters and differences between high and low protein:carbohydrate ratio with *.
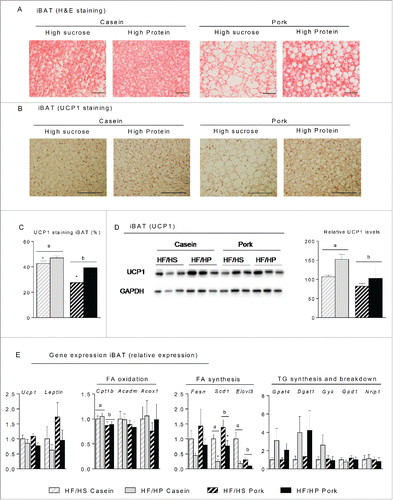
To further investigate possible molecular mechanisms that might contribute to the reduced feed efficiency of mice fed casein compared to mice fed pork, we performed systematic qPCR analyses of expression of genes with a potential to modulate metabolism in iBAT. Expression of Cpt1b in iBAT was higher in mice fed casein than in mice fed pork, but expression of other genes involved in fatty acid oxidation, Acadm and Acox1 did not vary in response to protein amount or source (). Expression in iBAT of genes involved in fatty acid synthesis, Scd1 and Elovl3, appeared to be modulated by both protein amount and source, and was in general highest in mice fed pork with a high proportion of sucrose (). In particular, expression of Scd1 was higher in mice fed the diets based on pork compared with mice fed the casein based diets, indicating a that pork fed mice might have a higher potential for fatty acid synthesis in iBAT (). In keeping with the effects of increased intake of carbohydrate relative to protein, expression of 6-phosphogluconate dehydrogenase (Pgd), involved in conversion of glucose to ribose-5 phosphate to generate NADPH required for fatty acids synthesis was higher in iBAT from mice fed diets with a high carbohydrate:protein ratio (Fig. S3). Expression of genes involved in glucose uptake, glucose transporter type 4 (Glut4), and glycolysis, glucose-6-phosphate isomerase (Gpi1), and aldolase A/fructose-bisphosphate aldolase (Aldoa) was not significantly modulated by neither protein source nor amount. Furthermore, mRNA expression of the genes encoding lactate dehydrogenase (LDHA) and pyruvate carboxylase (PCX) converting pyruvate into oxaloacetate did not change significantly in response to protein source or amount (Fig. S3). Expression of genes involved in glycogen storage and hydrolysis, glycogen synthase 2 (Gys2) and glycogen phosphorylase (Pygl1), respectively, was lower in mice fed the high protein diets irrespective of protein source (Fig. S3). Unexpectedly, expression of genes involved in triacylglycerol synthesis and reesterification, microsomal CoA:glycerol-3-phosphate acyltransferase 4 (Gpat4) and diacylglycerol acyltransferase (Dgat1) tended to be higher in iBAT from mice fed a high proportion of HF/HP compared to mice fed HF/HS (p = 0.077 and 0.094, respectively) (). Of note, expression of glycerol kinase (Gyk) tended to be higher in mice fed the casein-based HF/HP diet only. In conclusion, intake of a high protein diet based on casein resulted in the maintenance of a classical brown morphology of iBAT with higher expression of UCP1 than that observed in mice fed pork-based diets. Furthermore, high protein diets reciprocally modulated expression of genes involved in fatty acid synthesis and triacylglycerol synthesis in a manner suggesting the possible involvement of a futile cycling of fatty acids, which would increase energy expenditure and decrease feed efficiency.
To further investigate possible differences in metabolism of iBAT in reponse to intake of feed based on pork or casein as protein sources in connection with weight loss, we fed mice a very high fat diet (VHF) for 11 weeks to induce obesity and adipocytes with a white-like phenotype in iBAT. To facilitate weight loss, the mice were subsequently fed low fat diets with casein or pork as protein sources at standard levels (20 g/100 g feed). Mice fed casein-based diets lost significantly more body- and adipose tissue mass than mice fed pork (). In fact, body- and adipose tissue mass did not decrease in mice fed the pork-based low fat diet during the 6 weeks of feeding. Histological examination of iBAT revealed that only adipocytes from mice fed the low fat diet based on casein regained a classic brown phenotype (), and immunohistochemical analyses demonstrated that a higher proportion of adipocytes in casein compared to pork fed mice stained positive for UCP1 (). In this experiment increased UCP1 immunoreactivity was accompanied by increased Ucp1 mRNA expression (), but expression levels of other markers such as Dio2, Ppargc1a, Cidea did not differ between mice fed casein or pork-based diets (Fig. S4). The level of Nrip1 mRNA was significantly lower in the pork fed mice than in the casein fed mice (). NRIP1 has been shown to negatively regulate expression of UCP1Citation40 and hence, a lower expression of NRIP1 in mice fed casein compared to mice fed pork might contribute to the increased expression of UCP1 and the enhanced weight loss observed in the casein fed mice. Finally, whereas expression of genes involved in fatty acid oxidation and synthesis was similar in iBAT of casein and pork fed mice, expression of Gpat4 and Gyk, mRNAs involved in triacylglycerol synthesis and reesterification of fatty acids released from triacylglycerols, was significantly higher in casein than in pork fed mice (). Thus it is possible that intake of casein enhanced energy loss through a futile cycling of fatty acids.
Figure 6. Effect of low fat (LF) diets with casein or pork as protein source on body and fat mass reduction, histological appearance and gene expression in interscapular brown adipose tissue (iBAT). Male C57BL/6J mice were fed a very high fat (VHF) diet for 11 weeks to induce obesity development and were then switched to low fat (LF) diets (n = 10) containing either casein or pork as protein source for 6 additional weeks. One group marked with a scattered line continued eating the VHF diet. (A) body weight change of the mice after 6 weeks on the LF diets. The mice were terminated and (B) the epididymal white adipose tissue (eWAT) mass and (C) the inguinal white adipose tissue (iWAT) mass were recorded. (D) sections of iBAT (n = 4) were stained with eosin and hematoxylin (H&E), scalebar = 100 μm (E) sections of iBAT (n = 4) were immunohistologically stained with an UCP1-antibody and the area quantified, scalebar = 200 μm. (F) expression level of uncoupling protein 1 (Ucp1) and Leptin in addition to genes involved in fatty acid oxidation, carnitine palmitoyltransferase 1b (Cpt1b), acyl-Coenzyme A dehydrogenase, medium chain (Acadm) and acyl-Coenzyme A oxidase 1 (Acox1), fatty acid synthesis, fatty acid synthase (Fasn), stearoyl-Coenzyme A desaturase 1 (Scd1) and elongation of very long chain fatty acids (Elovl3) and triacylglycerol synthesis- and breakdown, microsomal CoA:glycerol-3-phosphate acyltransferase 4 (Gpat4), diacylglycerol acyltransferase 1 (Dgat 1), glycerol kinase (Gyk) glycerol-3-phosphate dehydrogenase 1 (Gpd1) and nuclear receptor interacting protein 1 (Nrip1). Expression levels were normalized to TATA-box binding protein (Tbp). Expression of individual genes in mice fed the LF diet is expressed relative to the expression in the mice fed the VHF (scattered line) diet. Significant differences (p < 0 .05) between the protein sources are presented with*.
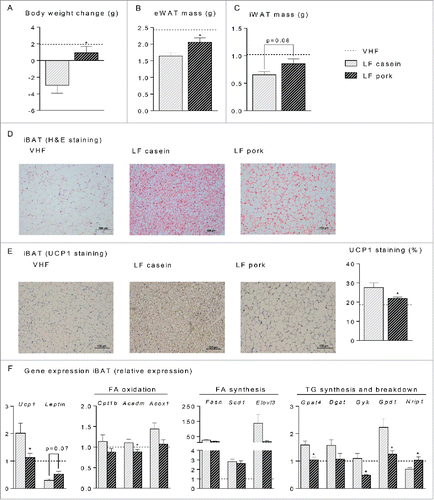
Discussion
A number of studies performed with casein or whey as protein sources has led to the general notion that intake of high protein diets protects against obesity. However, in this study we observed striking differences in relation to obesity development following intake of high protein diets based on different protein sources. Of the protein sources tested, casein was the only protein source that was able to fully prevent high fat diet-induced obesity, whereas feed based on the other protein sources to varying degree resulted in increased accretion of adipose tissue mass. Furthermore, intake of a low fat diet with casein, but not pork, as a protein source reversed diet-induced obesity. Compared to pork, casein seems superior in maintaining the classical brown morphology in interscapular brown adipose tissue with high UCP1 expression. This was accompanied by increased expression of genes involved in a futile cycling of fatty acids.
Casein, whey and soy are the most commonly used protein sources in commercially available rodent diets. However, protein sources for human consumption would normally comprise fish and meat. Freeze dried fish and meat will necessarily contain nutrients, including micronutrients, in addition to proteins. The amounts of carbohydrates in cod, beef, chicken and pork are negligible, but although lean muscle fillets were used as the protein source in the present study, they contained different types and amounts of endogenous fat. The total content of fat in the diets was balanced using corn oil, and since the the fatty acid composition varied between the different types of fillets, the fatty acid compositions in the experimental diets were not completely identical. In particular, as freeze-dried beef contained 17.6 g % fat, the fatty acid composition of the beef-based diet contained more saturated fat and less PUFA. However, the obesogenic effect of the various diets did not follow the different amounts of saturated fat. Also the n-6:n-3 PUFA ratio was influenced by the protein source. As the highest n-6:n-3 PUFA ratios were measured in the casein, soy and pork containing diets, it is not likely that the obesogenic effects were determined by this factor. However, given the well documented anti-obesity effect in rodents of n-3 PUFAs, in particular when they originate from phospholipids,Citation44,45 we can not exclude the possibility that an altered fatty acid composition, as well as different levels of micronutrients, influence the obesogenic potential of the diets. Elucidating the potential role of the slight differences in fatty acid composition and the possible effects of micronutrients will require further investigations, but still, our results emphasize the strikingly different effects of intake of different protein sources.
Our results clearly indicate that earlier studies using feed with a high proportion of casein are not representative for high protein diets in general. In the present study only mice receiving a feed with a high proportion of casein were as lean as mice fed the regular low fat diet. Furthermore, obesity was reversed with a low fat diet when casein, but not pork was used as a protein source. The anti-obesogenic effect of casein may relate to the high content of BCAAs in the casein-based diets, as BCAA supplementation of a high fat diet protects against development of obesity.Citation24 In fact, dietary supplementation of leucine alone is able to partially mimic the effect of high protein diets.Citation25,26 The circulating levels of BCAA were indeed higher in casein-fed than pork-fed mice. Accordingly, our results are in agreement with the notion that chronic elevated levels of BCAAs in mice with disrupted mitochondrial branched chain aminotransferase (BCATm−/− mice) are associated with increased energy expenditure and a lean phenotype.Citation43 The role of BCAA in insulin resistance is less clear, and in particular conflicting results of leucine supplementation on insulin resistance have been reported.Citation26 Increased circulating levels of BCAA have been associated with insulin resistance in humans, and the attenuated obesity development in rats fed a high fat diet supplemented with BCAA was accompanied with insulin resistance.Citation24 In our experiment, both insulin sensitivity and glucose tolerance mirrored adipose tissue mass. Thus, similar to BCATm−/− mice, the lean phenotype in casein fed mice was accompanied by increased circulating levels of BCAA and insulin sensitivity. The plasma levels of glycine and proline were higher in casein fed mice than in mice fed the other protein sources. An anti-obesogenic effect of glycine is reported in rats Citation46 and a high content of dietary proline may activate the nutrient-sensing lipolytic adipose tissue triglyceride lipase.Citation47 The latter may thereby contribute to the observed higher levels of free fatty acids in casein fed mice. Thus, future studies supplementing the other protein sources with BCAA, glycine and proline will be of interest to determine whether the higher levels of these amino acids contribute to the obesity protective effects of casein-based feed.
We utilized indirect calorimetry to evaluate diet-induced changes in energy metabolism. As both body mass and composition are strong determinants for gas exchange,Citation48 it was crucial to subject the mice to indirect calorimetric measurements before onset of obesity at the transition from a low fat reference diet to the experimental diets. However, we were unable to detect any significant differences in energy expenditure that could explain the difference in feed efficiency or adiposity. Gas exchange was quantified at intervals (i.e.1.9 min every 30 min). Thus, we cannot exclude the possibility that changes in energy expenditure, too small to be detected within the 48h indirect calorimetry measurements occurred. Furthermore, compared with mice fed casein, mice fed diets containing pork had a tendency toward lower activity level which over time may have impact on feed efficiency and obesity development. We can therefore not exclude the possibility that accumulated differences in locomotor activity elicited changes in EE that could over time result in altered weight gain and fat mass accretion.Citation49–51
RER measurements demonstrated lower metabolic flexibility in the mice fed pork- compared to casein-based diets. In addition, extented feeding with the diets resulted in impaired insulin sensitivity and glucose tolerance. Taken together this suggests a potential protein source-dependent effect on energy homeostasis. Intake of feed with a high proportion of protein reduced RER, indicating a lower utilization of carbohydrates and a higher utilization of fat and/or proteins. In line with this, we observed a higher plasma level of urea in mice fed diets with a high proportion of proteins for 11 weeks. Whether this reflects higher utilization of amino acids at the expense of carbohydrates remains to be determined. However, loss of energy in the form of ATP used in syntheses of urea and by the required conversion of amino acids to glucose Citation28,29 most likely contributes to the reduced feed efficiency in mice fed diets with high protein:carbohydrate ratios.
Energy may also be lost by conversion to heat by UCP1-dependent uncoupled mitochondrial respiration in brown adipocytes. White and brown (or brown-like) adipocytes are found together in most fat depots forming a plastic organ.Citation30 In reponse to aging or obesity and when mice are kept under thermoneutral conditions a “whitening” of the brown adipose organ can be observed.Citation52 Both protein amount and protein source influenced on the morphology and abundance of UCP1 immunoreactive cells in the interscapular region. In particular, a large proportion of the adipocytes in iBAT from the obese mice fed pork protein in combination with a low protein:carbohydrate ratio was unilocular and not UCP1-immunoreactive. By contrast, a large proportion of adipocytes from the lean mice fed the HF/HP diet based on casein was multilocular and stained positive for UCP1. Thus, maintenance of a brown phenotype of adipocytes in the interscapular region by a diet with a high content of casein may protect against obesity development.
Finally, energy may be lost by futile substrate cycling. Low activity of glycerol kinase would normally prevent a futile cycle of triacylglycerol breakdown and resynthesis from glycerol and free fatty acids. Thus, in the weight loss experiment, the higher level of Gyk expression in combination with higher expression of Gpat4 (and a tendency to higher expression of Dgat1) in iBAT of the casein fed mice is compatible with loss of energy via a futile cycling of fatty acids, suggesting that loss of energy may also relate to formation of fatty-acyl-CoA. Additionally, during weight loss, decreased expression of Nrip1 in the casein fed mice may further contribute to loss of energy by allowing increased expression of Ucp1 and Gyk supporting enhanced uncoupled respiration and futile cycling of triacylglycerol breakdown and resynthesis, respectively, in iBAT.
Conclusions
The protein source exerts a pronounced effect on the metabolic consequences by intake of high protein diets. A high protein diet based on casein effectively protects against high fat diet-induced obesity. Other tested protein sources are far less effective, and high protein diets based on chicken or pork are even more obesogenic than a traditional casein based high fat/high sucrose diet. While the detailed molecular background for the different ability of various protein sources to counteract obesity remains to be established, our results demonstrate that high protein diets based on casein are particularly effective in preventing whitening of interscapular brown adipose tissue and maintaining UCP1 expression. This was accompanied by increased expression of genes involved in a futile cycling of fatty acids, thereby preserving the possibility for increased energy expenditure in interscapular brown adipocytes. Given the popularity of high protein diets for weight management in humans, this warrants further investigations.
Methods
Ethical statement
The animal experiments were performed in accordance with the guidelines of the Norwegian Animal Research Authority (FOTS id.nr 3750). We observed no adverse effects during the trials.
Mouse diets
We prepared high fat/high sucrose (HF/HS) diets, high fat/high protein (HF/HP) diets and low fat (LF) diets using different protein sources according to and . We used casein (Sigma, C-8654), soy powder (Ssniff Spezialdiäten, Soest, Germany), cod fillet powder (Seagarden AS), beef tenderloin (H. Bragstad A/S, Bergen), chicken breast fillet (Prior, Norway) or pork sirloin (H. Bragstad A/S, Bergen) as protein sources. The cod fillet was freeze dried and pulverized by the provider (Seagarden AS), whereas beef, chicken and pork filets were freeze-dried and pulverized at NIFES. All protein sources were subsequently analyzed for amino acid composition, nitrogen and total fat content () as described previouslyCitation11 in order to balance the diets with respect to total protein and fat content. The diets () were mixed using a Crypto Peerless EF20 blender, kept at -20 °C, and analyzed for gross energy () by bomb calometry (Parr Instruments, Moline, IL, USA) and fatty acid composition () using a capillary gas chromatograph (GLC Trance GC 200, Thermo Scientific, USA) with a flame ionization detector (Thermo Scientific) as described.Citation11 In addition, a standard very high fat (VHF) diet obtained from Ssniff (acc. D12492 Ssniff, Spezialdiäten, Soest, Germany) was used to induce obesity in experiment 4, see below. A regular casein-based low-fat diet was used as a reference diet (RD) (ssniff EF R/M control (E15000–04), Germany).
Animals
The data in this paper are based on the results from 4 separate animal studies using male C57BL/6JBomTac mice (Taconic), 8 weeks of age at the start of the study, housed in individual cages at thermoneutrality (28–30°C) with a 12 h light/dark cycle. In experiment 1 and 2, the mice were assigned to experimental groups (n = 9) by body weight after 5 d acclimatization. The mice were weighed once a week and fed ad libitum 3 times a week. In experiment 1, the mice were fed RD, HF/HS using casein as the protein source or HF/HP using casein, soy, cod, beef, chicken or pork as a protein source. In experiment 2, the mice were fed casein-based RD, or HF/HS and HF/HP using either casein or pork as the protein source. In experiment 2, body composition was determined after 8 weeks of feeding using nuclear magnetic resonance (Minispec mq 7.5, NMR analyzer, Bruker, Germany). Furthermore, after 9 and 10 weeks, respectively, intraperitoneal glucose and insulin tolerance tests (ITT and GTT) were performed as described earlier using 2g glucose and 0.75 units of insulin (Actrapid, Novo Nordisk, Denmark) pr kg body mass, respectively.Citation53 As GTT and ITT may influence on feed intake, data prior to testing (first 8 weeks) were used to calculate feed efficiency. Apparent digestibility was measured as described Citation11 during the 7th week of feeding. After 11 weeks of feeding the mice in both experiment 1 and 2 were fasted for 4 h before they were sacrificed by cardiac puncture under Isoflurane anesthesia (Isoba-vet, Schering-Plough, Denmark). Plasma was prepared from blood and stored at −80°C before further analyses using commercial available kits as previously described.Citation10 Liver, muscle and adipose tissue were dissected out, weighed, snap-frozen in liquid nitrogen and stored at -80°C. A portion of each adipose depot was fixed for histology (see below). A third cohort (experiment 3) of C57BL/6J mice was used for indirect calorimetry measurements (see below). In experiment 3, the mice were fed HF/HS and HF/HP using casein or pork as the protein sources. In experiment 4, 30 mice were fed a VHF diet for 11 weeks before they were assigned to experimental groups (n = 10) and fed LF diets containing either casein or pork as protein source for 6 weeks (). An additional group of mice were continuously fed the VHF diet.
Real-time qPCR and Western blotting
Using tissues from experiment 2 and 3, RNA purification, reverse transcription and RT-qPCR were performed as described Citation22 and mRNA expression was normalized to that of the housekeeping gene TATA box binding protein (Tbp). Western blotting was performed essentially as described earlier Citation54 using antibodies against glyceraldehyde 3-phosphate dehydrogenase (Ab8245, Abcam) and UCP1 (Ab10983, Abcam).
Indirect calorimetric measurements
As body mass and body composition are strong determinants for both O2-utilization and CO2-production,Citation48 we used a new set of mice (Experiment 3) to record diet-induced metabolic changes. The mice were placed in the metabolic cages and fed RD for 72 h. Gas exchange and beam breaks, as a proxy for activity, were recorded during the first 48 h. The mice (n = 4) were subsequently fed the experimental diets for another 72 h and gas exchange and activity were recorded for the last 48 h. Diet-induced changes for each individual mouse were calculated. Respiratory exchange ratio (RER) was calculated from VO2 and VCO2 and spontaneous locomotor activity was defined as total counts of light-beam breaks. VO2 and VCO2 were measured in open-circuit indirect calorimetry cages as described previously Citation22 using CaloCages (Phenomaster, TSE Systems), equipped with infrared light-beam frames (ActiMot2). Energy expenditure (EE) was calculated using the following equation: 3.9 cal/L x L VO2 + 1.1cal/L x L VCO2.Citation55 EE and RER were analyzed by generating percent relative cumulative frequency (PRCF) curves as described by Riachi et al.Citation56 EE data were normalized to body weight.
Histology
Sections of different adipose depots were fixed in 4 % formaldehyde in 0.1 M phosphate buffer overnight, dehydrated, embedded and stained with eosin and hematoxylin.Citation57 Adipocyte cell size was determined and immunohistological detection of UCP1-positive cells was performed by an avidin-biotin peroxidase method as described earlier.Citation53
Statistical analyses
All data are presented as mean ± SEM. The data from experiment 1 was analyzed using 1-way ANOVA followed by Tukey's multiple comparison, group means were considered statistically different at P < 0.05. Growth, GTT and ITT were analyzed by repeated measurements ANOVA followed by Tukey's post hoc test. In experiment 2 and 3 we used a factorial ANOVA design with protein:carbohydrate ratio and protein source as categorical predictors (Statistica 9.0, StatSoft Inc.). In experiment 4 a Student's t-test was used used to analyze the data.
Abbreviations
AUC | = | area under the curve |
BCAA | = | branched chain amino acids |
BW | = | body weight |
DHA | = | docosahexaenoic acid |
EE | = | energy expenditure |
EPA | = | eicosapentaenoic acid |
GTT | = | glucose tolerance test |
HF/HP | = | high fat high protein |
HF/HS | = | high fat high sucrose |
iBAT | = | interscapular brown adipose tissue |
ITT | = | insulin tolerance test |
iWAT | = | inguinal white adipose tissue |
PRCF | = | percent relative cumulative frequency |
PUFA | = | polyunsaturated fatty acids |
RD | = | reference diet |
RER | = | respiratory exchange ratio |
UCP1 | = | uncouling protein 1 |
Disclosure of potential conflicts of interest
No potential conflicts of interest were disclosed.
Authors`contributions
B.L., K.K. and L.M. designed research. U.L., L.S.M., A.K.R., E.F., S.B., K.R.F., A.L.B. and J.B.H. performed experiments and all authors interpreted the results. U.L., L.S.M, KK and L.M. wrote the manuscript. U.L., L.S.M. and E.F. prepared the figures. All authors edited and revised the manuscript and approved the final version. K.K. and L.M. have primary responsibility for the final content.
KADI_S_1122855.docx
Download MS Word (1.3 MB)Acknowledgments
We thank Dr. Pavel Flachs and Prof. Jan Kopecky for kindly providing the UCP1 antibody used for immunohistochemistry and the staff at NIFES for technical assistance and animal care. We would like to specifically acknowledge the early contribution of Vigdis Misje Hagen to this project.
Funding
This project was supported by the European Union FP7 project DIABAT (HEALTH-F2–2011–278373) to J.B.H., K.K. and L.M. This work was also in part supported by The Carlsberg Foundation, The Norwegian Seafood Research Fund (FINS 900842), the Norwegian Research Council (200515/ I30), the SHARE Cross Faculty Ph.D. Initiative of the University of Copenhagen and NIFES.
References
- Halton TL, Hu FB. The effects of high protein diets on thermogenesis, satiety and weight loss: a critical review. J Am Coll Nutr 2004; 23:373-85; PMID:15466943; http://dx.doi.org/10.1080/07315724.2004.10719381
- Lepe M, Bacardi Gascon M, Jimenez Cruz A. Long-term efficacy of high-protein diets: a systematic review. Nutr Hosp 2011; 26:1256-9; PMID:22411369
- Westerterp-Plantenga MS, Lemmens SG, Westerterp KR. Dietary protein - its role in satiety, energetics, weight loss and health. Br J Nutr 2012; 108 Suppl 2:105-12; http://dx.doi.org/10.1017/S0007114512002589
- Pesta DH, Samuel VT. A high-protein diet for reducing body fat: mechanisms and possible caveats. Nutr Metab (Lond) 2014; 11:53; PMID:25489333; http://dx.doi.org/10.1186/1743-7075-11-53
- Gilbert JA, Bendsen NT, Tremblay A, Astrup A. Effect of proteins from different sources on body composition. Nutr Metab Cardiovasc Dis 2011; 21 Suppl 2:16-31; http://dx.doi.org/10.1016/j.numecd.2010.12.008
- Tastesen HS, Keenan AH, Madsen L, Kristiansen K, Liaset B. Scallop protein with endogenous high taurine and glycine content prevents high-fat, high-sucrose-induced obesity and improves plasma lipid profile in male C57BL/6J mice. Amino Acids 2014; 46(7):1659-71; PMID:24658997
- Torre-Villalvazo I, Tovar AR, Ramos-Barragan VE, Cerbon-Cervantes MA, Torres N. Soy protein ameliorates metabolic abnormalities in liver and adipose tissue of rats fed a high fat diet. J Nutr 2008; 138:462-8; PMID:18287350
- Liaset B, Madsen L, Hao Q, Criales G, Mellgren G, Marschall HU, Espe M, Frøyland L, Kristiansen K. Fish protein hydrolysate elevates plasma bile acids and reduces visceral adipose tissue mass in rats. Biochim Biophys Acta 2009; 1791:254-62; PMID:19416649; http://dx.doi.org/10.1016/j.bbalip.2009.01.016
- Jacques H, Leblanc N, Papineau R, Richard D, Cote CH. Peanut protein reduces body protein mass and alters skeletal muscle contractile properties and lipid metabolism in rats. Br J Nutr 2010; 103:1331-9; PMID:20028600; http://dx.doi.org/10.1017/S0007114509993278
- Liaset B, Hao Q, Jorgensen H, Hallenborg P, Du ZY, Ma T, Marschall HU, Kruhøffer M, Li R, Li Q, et al. Nutritional regulation of bile acid metabolism is associated with improved pathological characteristics of the metabolic syndrome. J Biol Chem 2011; 286:28382-95; PMID:21680746; http://dx.doi.org/10.1074/jbc.M111.234732
- Tastesen HS, Ronnevik AK, Borkowski K, Madsen L, Kristiansen K, Liaset B. A Mixture of Cod and Scallop Protein Reduces Adiposity and Improves Glucose Tolerance in High-Fat Fed Male C57BL/6J Mice. PLoS One 2014; 9:112859; http://dx.doi.org/10.1371/journal.pone.0112859
- Lillefosse HH, Clausen MR, Yde CC, Ditlev DB, Zhang XM, Du ZY, Bertram HC, Madsen L, Kristiansen K, Liaset B. Urinary Loss of Tricarboxylic Acid Cycle Intermediates As Revealed by Metabolomics Studies: An Underlying Mechanism to Reduce Lipid Accretion by Whey Protein Ingestion? J Proteome Res 2014; 13:2560-70; PMID:24702026; http://dx.doi.org/10.1021/pr500039t
- Lacroix M, Gaudichon C, Martin A, Morens C, Mathe V, Tome D, Huneau JF. A long-term high-protein diet markedly reduces adipose tissue without major side effects in Wistar male rats. Am J Physiol Regul Integr Comp Physiol 2004; 287:934-42; http://dx.doi.org/10.1152/ajpregu.00100.2004
- Marsset-Baglieri A, Fromentin G, Tome D, Bensaid A, Makkarios L, Even PC. Increasing the protein content in a carbohydrate-free diet enhances fat loss during 35% but not 75% energy restriction in rats. J Nutr 2004; 134:2646-52; PMID:15465761
- Morens C, Keijzer M, de Vries K, Scheurink A, van Dijk G. Effects of high-fat diets with different carbohydrate-to-protein ratios on energy homeostasis in rats with impaired brain melanocortin receptor activity. Am J Physiol Regul Integr Comp Physiol 2005; 289:156-63; http://dx.doi.org/10.1152/ajpregu.00774.2004
- Pichon L, Huneau JF, Fromentin G, Tome D. A high-protein, high-fat, carbohydrate-free diet reduces energy intake, hepatic lipogenesis, and adiposity in rats. J Nutr 2006; 136:1256-60; PMID:16614413
- Petzke KJ, Riese C, Klaus S. Short-term, increasing dietary protein and fat moderately affect energy expenditure, substrate oxidation and uncoupling protein gene expression in rats. J Nutr Biochem 2007; 18:400-7; PMID:16979329; http://dx.doi.org/10.1016/j.jnutbio.2006.07.005
- Madsen L, Pedersen LM, Liaset B, Ma T, Petersen RK, van den Berg S, Pan J, Müller-Decker K, Dülsner ED, Kleemann R, et al. cAMP-dependent signaling regulates the adipogenic effect of n-6 polyunsaturated fatty acids. J Biol Chem 2008; 283:7196-205; PMID:18070879; http://dx.doi.org/10.1074/jbc.M707775200
- Ma T, Liaset B, Hao Q, Petersen RK, Fjaere E, Ngo HT, Lillefosse HH, Ringholm S, Sonne SB, Treebak JT, et al. Sucrose counteracts the anti-inflammatory effect of fish oil in adipose tissue and increases obesity development in mice. PLoS One 2011; 6:21647; http://dx.doi.org/10.1371/journal.pone.0021647
- Freudenberg A, Petzke KJ, Klaus S. Comparison of high-protein diets and leucine supplementation in the prevention of metabolic syndrome and related disorders in mice. J Nutr Biochem 2012; 23:1524-30; PMID:22405695; http://dx.doi.org/10.1016/j.jnutbio.2011.10.005
- Hao Q, Lillefosse HH, Fjaere E, Myrmel LS, Midtbo LK, Jarlsby RH, Ma T, Jia B, Petersen RK, Sonne SB, et al. High-glycemic index carbohydrates abrogate the antiobesity effect of fish oil in mice. Am J Physiol Endocrinol Metab 2012; 302:1097-112; http://dx.doi.org/10.1152/ajpendo.00524.2011
- Lillefosse HH, Tastesen HS, Du ZY, Ditlev DB, Thorsen FA, Madsen L, Kristiansen K, Liaset B. Hydrolyzed Casein Reduces Diet-Induced Obesity in Male C57BL/6J Mice. J Nutr 2013; 143:1367-75; PMID:23843475; http://dx.doi.org/10.3945/jn.112.170415
- Layman DK, Walker DA. Potential importance of leucine in treatment of obesity and the metabolic syndrome. J Nutr 2006; 136:319S-23S; PMID:16365106
- Newgard CB, An J, Bain JR, Muehlbauer MJ, Stevens RD, Lien LF, Haqq AM, Shah SH, Arlotto M, Slentz CA, et al. A Branched-Chain Amino Acid-Related Metabolic Signature that Differentiates Obese and Lean Humans and Contributes to Insulin Resistance. Cell Metab 2009; 9:565-6; http://dx.doi.org/10.1016/j.cmet.2009.05.001
- Freudenberg A, Petzke KJ, Klaus S. Dietary L-leucine and L-alanine supplementation have similar acute effects in the prevention of high-fat diet-induced obesity. Amino Acids 2013; 44:519-28; PMID:22847780; http://dx.doi.org/10.1007/s00726-012-1363-2
- Petzke KJ, Freudenberg A, Klaus S. Beyond the Role of Dietary Protein and Amino Acids in the Prevention of Diet-Induced Obesity. Int J Mol Sci 2014; 15:1374-91; PMID:24447927; http://dx.doi.org/10.3390/ijms15011374
- Tappy L. Thermic effect of food and sympathetic nervous system activity in humans. Reprod Nutr Dev 1996; 36:391-7; PMID:8878356; http://dx.doi.org/10.1051/rnd:19960405
- Fine EJ, Feinman RD. Thermodynamics of weight loss diets. Nutr Metab (Lond) 2004; 1:15; PMID:15588283; http://dx.doi.org/10.1186/1743-7075-1-15
- Manninen AH. Is a Calorie Really a Calorie? Metabolic Advantage of Low-Carbohydrate Diets. J Int Soc Sport Nutr 2004; 1:21-26.
- Frontini A, Cinti S. Distribution and Development of Brown Adipocytes in the Murine and Human Adipose Organ. Cell Metab 2010; 11:253-6; PMID:20374956; http://dx.doi.org/10.1016/j.cmet.2010.03.004
- Cannon B, Nedergaard J. Brown adipose tissue: Function and physiological significance. Physiol Rev 2004; 84:277-359; PMID:14715917; http://dx.doi.org/10.1152/physrev.00015.2003
- Murano I, Barbatelli G, Giordano A, Cinti S. Noradrenergic parenchymal nerve fiber branching after cold acclimatisation correlates with brown adipocyte density in mouse adipose organ. J Anat 2009; 214:171-8; PMID:19018882; http://dx.doi.org/10.1111/j.1469-7580.2008.01001.x
- Vitali A, Murano I, Zingaretti MC, Frontini A, Ricquier D, Cinti S. The adipose organ of obesity-prone C57BL/6J mice is composed of mixed white and brown adipocytes. J Lipid Res 2012; 53:619-29; PMID:22271685; http://dx.doi.org/10.1194/jlr.M018846
- Bonet ML, Oliver P, Palou A. Pharmacological and nutritional agents promoting browning of white adipose tissue. Biochim Biophys Acta 2013; 1831:969-85; PMID:23246573; http://dx.doi.org/10.1016/j.bbalip.2012.12.002
- Petzke KJ, Friedrich M, Metges CC, Klaus S. Long-term dietary high protein intake up-regulates tissue specific gene expression of uncoupling proteins 1 and 2 in rats. Eur J Nutr 2005; 44:414-21; PMID:15602629; http://dx.doi.org/10.1007/s00394-004-0545-4
- Kopecky J, Rossmeisl M, Hodny Z, Syrovy I, Horakova M, Kolarova P. Reduction of dietary obesity in aP2-Ucp transgenic mice: Mechanism and adipose tissue morphology. Am J Physiol Endocrinol Metab 1996; 270:776-86
- Wu J, Cohen P, Spiegelman BM. Adaptive thermogenesis in adipocytes: is beige the new brown? Genes Dev 2013; 27:234-50; PMID:23388824; http://dx.doi.org/10.1101/gad.211649.112
- Kiskinis E, Chatzeli L, Curry E, Kaforou M, Frontini A, Cinti S, Montana G, Parker MG, Christian M. RIP140 represses the "brown-in-white" adipocyte program including a futile cycle of triacylglycerol breakdown and synthesis. Mol Endocrinol 2014; 28:344-56; PMID:24479876; http://dx.doi.org/10.1210/me.2013-1254
- Guan HP, Li Y, Jensen MV, Newgard CB, Steppan CM, Lazar MA. A futile metabolic cycle activated in adipocytes by antidiabetic agents. Nat Med 2002; 8:1122-8; PMID:12357248; http://dx.doi.org/10.1038/nm780
- Leonardsson G, Steel JH, Christian M, Pocock V, Milligan S, Bell J, So PW, Medina-Gomez G, Vidal-Puig A, White R, et al. Nuclear receptor corepressor RIP140 regulates fat accumulation. Proc Natl Acad Sci U S A 2004; 101:8437-42; PMID:15155905; http://dx.doi.org/10.1073/pnas.0401013101
- Flachs P, Rossmeisl M, Kuda O, Kopecky J. Stimulation of mitochondrial oxidative capacity in white fat independent of UCP1: a key to lean phenotype. Biochim Biophys Acta 2013; 1831:986-1003; PMID:23454373; http://dx.doi.org/10.1016/j.bbalip.2013.02.003
- National Research Council. Nutrient Requirements of Laboratory Animals, Fourth Revised Edition, 1995. Washington, DC: The National Academies Press, 1995
- She P, Reid TM, Bronson SK, Vary TC, Hajnal A, Lynch CJ, Hutson SM. Disruption of BCATm in mice leads to increased energy expenditure associated with the activation of a futile protein turnover cycle. Cell Metab 2007; 6:181-94; PMID:17767905; http://dx.doi.org/10.1016/j.cmet.2007.08.003
- Rossmeisl M, Jilkova ZM, Kuda O, Jelenik T, Medrikova D, Stankova B, Kristinsson B, Haraldsson GG, Svensen H, Stoknes I, et al. Metabolic effects of n-3 PUFA as phospholipids are superior to triglycerides in mice fed a high-fat diet: possible role of endocannabinoids. PLoS One 2012; 7:38834; http://dx.doi.org/10.1371/journal.pone.0038834
- Alvheim AR, Malde MK, Osei-Hyiaman D, Lin YH, Pawlosky RJ, Madsen L, Kristiansen K, Frøyland L, Hibbeln JR. Dietary linoleic acid elevates endogenous 2-AG and anandamide and induces obesity. Obesity (Silver Spring) 2012; 20:1984-94; PMID:22334255; http://dx.doi.org/10.1038/oby.2012.38
- El Hafidi M, Perez I, Zamora J, Soto V, Carvajal-Sandoval G, Banos G. Glycine intake decreases plasma free fatty acids, adipose cell size, and blood pressure in sucrose-fed rats. Am J Physiol Regul Integr Comp Physiol 2004; 287:1387-93; http://dx.doi.org/10.1152/ajpregu.00159.2004
- Lettieri Barbato D, Aquilano K, Baldelli S, Cannata SM, Bernardini S, Rotilio G, Ciriolo MR. Proline oxidase-adipose triglyceride lipase pathway restrains adipose cell death and tissue inflammation. Cell Death Differ 2014; 21:113-23; PMID:24096872; http://dx.doi.org/10.1038/cdd.2013.137
- Even PC, Nadkarni NA. Indirect calorimetry in laboratory mice and rats: principles, practical considerations, interpretation and perspectives. Am J Physiol Regul Integr Comp Physiol 2012; 303:R459-R76; PMID:22718809; http://dx.doi.org/10.1152/ajpregu.00137.2012
- Burnett CM, Grobe JL. Dietary effects on resting metabolic rate in C57BL/6 mice are differentially detected by indirect (O2/CO2 respirometry) and direct calorimetry. Mol Metab 2014; 3:460-4; PMID:24944905; http://dx.doi.org/10.1016/j.molmet.2014.03.003
- Rozman J, Klingenspor M, Hrabe de Angelis M. A review of standardized metabolic phenotyping of animal models. Mamm Genome 2014; 25:497-507; PMID:25199945; http://dx.doi.org/10.1007/s00335-014-9532-0
- Speakman JR. Should we abandon indirect calorimetry as a tool to diagnose energy expenditure? Not yet. Perhaps not ever. Commentary on Burnett and Grobe (2014). Mol Metab 2014; 3:342-4; PMID:24944891; http://dx.doi.org/10.1016/j.molmet.2014.04.003
- Giordano A, Smorlesi A, Frontini A, Barbatelli G, Cinti S. White, brown and pink adipocytes: the extraordinary plasticity of the adipose organ. Eur J Nutr 2014; 170:159-71
- Fjaere E, Aune UL, Roen K, Keenan AH, Ma T, Borkowski K, Kristensen DM, Novotny GW, Mandrup-Poulsen T, Hudson BD, et al. Indomethacin Treatment Prevents High Fat Diet-induced Obesity and Insulin Resistance but Not Glucose Intolerance in C57BL/6J Mice. J Biol Chem 2014; 289:16032-45; PMID:24742673; http://dx.doi.org/10.1074/jbc.M113.525220
- Hansen JB, Petersen RK, Larsen BM, Bartkova J, Alsner J, Kristiansen K. Activation of peroxisome proliferator-activated receptor gamma bypasses the function of the retinoblastoma protein in adipocyte differentiation. J Biol Chem 1999; 274:2386-93; PMID:9891007; http://dx.doi.org/10.1074/jbc.274.4.2386
- Weir JB. New methods for calculating metabolic rate with special reference to protein metabolism. J Physiol 1949; 109:1-9; PMID:15394301; http://dx.doi.org/10.1113/jphysiol.1949.sp004363
- Riachi M, Himms-Hagen J, Harper ME. Percent relative cumulative frequency analysis in indirect calorimetry: application to studies of transgenic mice. Can J Physiol Pharmacol 2004; 82:1075-83; PMID:15644949; http://dx.doi.org/10.1139/y04-117
- Barbatelli G, Murano I, Madsen L, Hao Q, Jimenez M, Kristiansen K, Giacobino JP, De Matteis R, Cinti S. The emergence of cold-induced brown adipocytes in mouse white fat depots is determined predominantly by white to brown adipocyte transdifferentiation. Am J Physiol Endocrinol Metab 2010; 298:1244-53; http://dx.doi.org/10.1152/ajpendo.00600.2009