ABSTRACT
Adipose tissue is classified as either white (WAT) or brown (BAT) and differs not only by anatomical location but also in function. WAT is the main source of stored energy and releases fatty acids in times of energy demand, whereas BAT plays a role in regulating non-shivering thermogenesis and oxidizes fatty acids released from the lipid droplet. The PLIN family of proteins has recently emerged as being integral in the regulation of fatty acid storage and release in adipose tissue. Previous work has demonstrated that PLIN protein content varies among adipose tissue depots, however an examination of endurance training-induced depot specific changes in PLIN protein expression has yet to be done. Male Sprague-dawley rats (n = 10) underwent 8-weeks of progressive treadmill training (18–25 m/min for 30–60 min at 10% incline) or remained sedentary as control. Following training, under isoflurane induced anesthesia epidydmal (eWAT), inguinal subcutaneous (iWAT) and intrascapular brown adipose tissue (BAT) was excised, and plasma was collected. Endurance training resulted in an increase in BAT PLIN5 and iWAT PLIN3 content, while there was no difference in PLIN protein content in endurance trained eWAT. Interestingly, endurance training resulted in a robust increase in ATGL and CGI-58 in eWAT alone. Together these results suggest the potential of a depot specific function of PLIN3 and PLIN5 in adipose tissue in response to endurance training.
Introduction
Adipose tissue plays a large role in regulating whole body energy homeostasisCitation1-3 and can be classified into 2 functionally different classes: brown adipose tissue (BAT) and white adipose tissue (WAT, visceral and subcutaneous depots). WAT is the main source of stored energy in the form of triglycerides in lipid droplets,Citation4 whereas BAT plays a role in regulating non-shivering thermogenesis and dissipating energy as heat.Citation2,5,6 The family of lipid droplet coat proteins known as perilipins or PLIN proteins (formerly known as PAT proteins) have emerged as being essential in regulating fatty acid storage and release from lipid droplets.Citation7–12 Previous work has indicated that PLIN protein content varies among adipose tissue depots.Citation13 It has been hypothesized that this depot specific expression of the PLIN proteins is a reflection of the functional differences of WAT and BAT.Citation13 Endurance training can have profound effects on the biochemical properties and morphology of both BAT and WAT,Citation3,14–18 however the effects of endurance training on adipose tissue PLIN proteins has yet to be investigated.
The PLIN family is composed of 5 members (PLIN1 through PLIN5),Citation19,20 each with a unique tissue distribution profile and potentially a unique role in cellular lipid metabolism.Citation13,21,22 PLIN1 is the only member of this family for which a specific role in regulating lipolysis has been determined, and it is ubiquitously expressed across adipose tissue depots.Citation13 Specifically, in a basal state PLIN1 limits the activity of the rate limiting lipase, ATGL, by directly binding to its co-activator, CGI-58.Citation23-26 While PLIN2 is expressed uniformly across adipose tissue depots, the expression of PLIN3 and PLIN5 varies among the depots.Citation13 PLIN4 is also expressed in white adipose tissue depots, but does not seem to be expressed in brown adipose tissue.Citation13 Together, this might indicate a unique role for both PLIN3 and PLIN5 in these adipose tissue depots.
PLIN5 is abundantly expressed in BAT, the most oxidative of the depots.Citation13 Similarly, PLIN5 is highly expressed in the liver, heart, and soleus, all of which have a high capacity for fatty acid oxidation.Citation13,22 Given that PLIN5 is expressed in highly oxidative tissues, PLIN5 has been hypothesized to play a role in channeling fatty acids toward mitochondria for β-oxidation.Citation13,27–29 In support of this hypothesis, PLIN5 expression is induced by physiological, pharmacological, and genetic perturbations that increase utilization of fatty acids for oxidative phosphorylation.Citation13,22,30 Further, PLIN5 has been shown to localize to mitochondria in smooth and skeletal muscle cellsCitation28,31,32 as well as link lipid droplets to mitochondria.Citation27 Interestingly, PLIN5 is also moderately expressed in epididymal WAT (eWAT) but not subcutaneous WAT (iWAT), although to a lesser degree when compared to BAT.Citation13 This may be indicative of the oxidative potential of eWAT. Several studies have reported increases in the thermogenic capacity of classical white adipose tissue depots with exercise training.Citation15,18 Endurance training can result in increased PLIN5 in other metabolically active tissues, such as skeletal muscle,Citation33,34 however it remains unknown whether endurance training results in an increased PLIN5 content in adipose tissue.
Adipose tissue PLIN3 expression is high in both subcutaneous and epididymal WAT and relatively lower in BAT.Citation13 Originally discovered as a trafficking protein, PLIN3 has been implicated in various intracellular roles and interactions with various intracellular organelles.Citation31,35–38 Specifically, in OP9 mouse stromal cells and 3T3-L1 adipocytes PLIN3 has been shown to be recruited to the endoplasmic reticulum and lipid droplet surface where it is believed to assist in fatty acid synthesis.Citation36,38 Following endurance training, skeletal muscle and mitochondrial PLIN3 protein content increases have been demonstratedCitation32,39 in addition to maintaining protein-protein interactions with lipolytic proteins following acute contractionCitation40 suggesting PLIN3 involvement in skeletal muscle lipolysis. Further, in human skeletal muscle one bout of endurance exercise results in increased PLIN3 protein content.Citation41 Little is known about PLIN3 in adipose tissue, but in subcutaneous adipose tissue from women with polycystic ovary syndrome, PLIN3 is the only PLIN protein to be responsive to endurance training.Citation42 What remains unknown is whether PLIN3 protein content is altered in a healthy rodent model in various adipose tissue depots following endurance training.
An understanding of the molecular mechanisms that govern lipolysis and lipid storage in different adipose tissue depots is an important area of investigation when considering the effects of altered adipose tissue metabolism on whole body health. To date no studies have directly compared the effects of exercise training on PLIN protein content in different adipose tissue depots. The purpose of this study is to determine the effect of endurance training on adipose PLIN protein expression in brown (BAT), subcutaneous, (iWAT) and epididymal adipose tissue (eWAT).
Results
Plasma NEFAs
Endurance training resulted in a ˜50% reduction in circulating plasma non-esterified fatty acids (p < 0.05)().
Table 1. Rodent characteristics. Change in body weights and plasma non—esterified fatty acid content.
Endurance training resulted in adipose depot specific changes in PLIN family proteins
In BAT, PLIN5 protein content was 2-fold higher in the endurance-trained compared to sedentary group (p < 0.05; ). PLIN5 was detected in eWAT with a trend toward increased content in the endurance trained group (p = 0.09; ). In iWAT, the endurance-trained group had a 1.5-fold higher PLIN3 content compared to sedentary (p < 0.05; ). While in eWAT, there were no differences between groups for any of the PLIN proteins ().
Figure 1. Endurance training results in depot specific changes in PLIN protein content. a) BAT PLIN proteins; b) iWAT PLIN proteins; c) eWAT PLIN proteins. Statistics compare sedentary vs. endurance-trained within the same protein. Data are reported as mean ± SE.* denotes significant differences between trained and sedentary (p < 0.05).
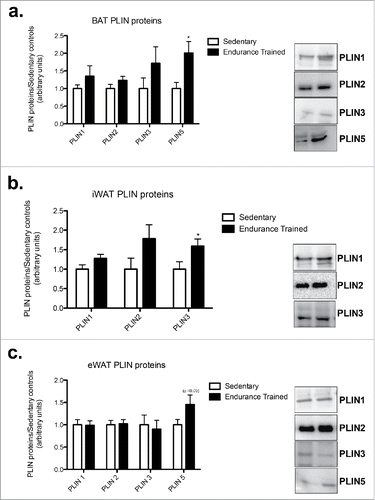
Markers of mitochondrial content are unchanged in all adipose depots following endurance training
Across all 3 depots, there were no changes in mitochondrial proteins COXIV, PDH, or UCP1 (). UCP1 was only detected in BAT ().
Figure 2. Endurance training did not alter mitochondrial protein content in adipose tissue depots. a) BAT mitochondrial proteins; b) iWAT mitochondrial proteins; c) eWAT mitochondrial proteins (COXIV: cytochrome c oxidase subunit IV; PDH: pyruvate dehydrogenase; UCP1: uncoupling protein 1. Statistics compare sedentary vs. endurance-trained within the same protein. Data are reported as mean±SE. * denotes significant differences between trained and sedentary (p < 0.05).
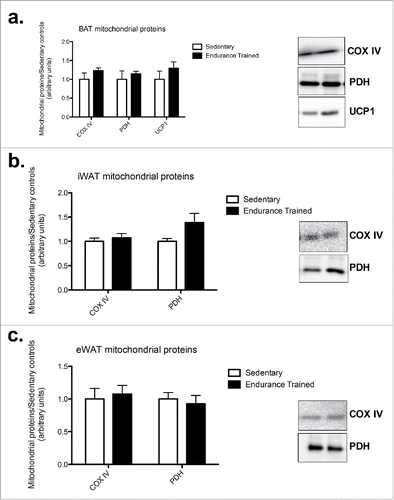
Endurance training reduced acetyl-CoA carboxylase content in BAT and eWAT
There was a 2-fold decrease in acetyl-CoA carboxylase (ACC) in BAT in the endurance-trained compared to sedentary group (p < 0.05; ). Similarly training resulted in a 3-fold decrease in ACC in eWAT (p < 0.05; ). There were no differences between groups for ACC content in iWAT ().
Figure 3. Endurance training reduced ACC content in BAT and eWAT. a) BAT lipogenic proteins; b) iWAT lipogenic proteins; c) eWAT lipogenic proteins. Statistics compare sedentary vs. endurance-trained within the same protein (FAS: fatty acid synthase; ACC: acetyl CoA-carboxylase). Data are reported as mean±SE. * denotes significant differences between trained and sedentary (p < 0.05).
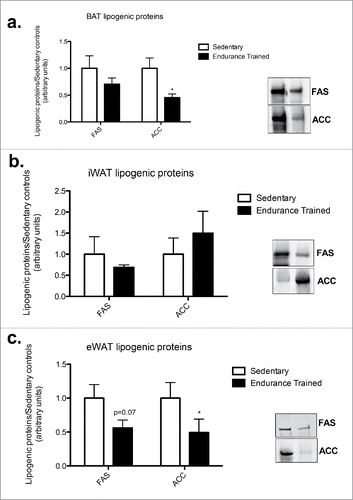
Endurance training results in depot specific differences in lipolytic proteins
In BAT, there was a 2-fold decrease in pHSLs563 in the endurance-trained group compared to sedentary (p < 0.05), with no difference in total HSL protein content or pHSLs660 (). In eWAT, there was a 1.5-fold increase in ATGL protein with almost a 4-fold increase in pATGLs406 in the endurance-trained compared to sedentary group, with a 1.3-fold increase in CGI-58 protein content (p < 0.05) (). There were no differences in any measured lipolytic proteins in iWAT between groups ().
Figure 4. Endurance training increases ATGL and CGI-58 content in eWAT. a) BAT lipolytic proteins; b) iWAT lipogenic proteins; c) eWAT lipogenic proteins (HSL: hormone sensitive lipase; ATGL: adipose triglyceride lipase; CGI-58: comparative gene identification-58). Data are reported as mean±SE. * denotes significant differences between trained and sedentary (p < 0.05).
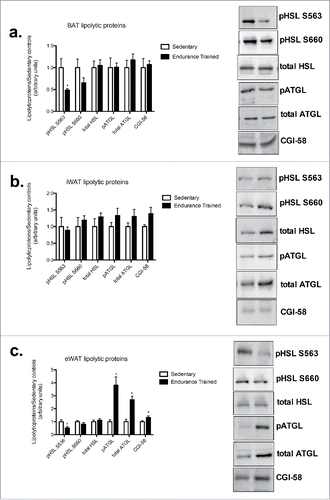
Methods
Materials
Reagents, molecular weight marker and nitrocellulose membranes for SDS-PAGE were purchased from Bio-Rad (Mississauga, ON). Polyvinlidene difluride membranes were purchased from Millipore (IPVH00010; Darmstad, Germany). Antibodies against HSL (#4107; 1:1000 5% milk), HSL S563 (#4139; 1:1000 5% milk), HSL S660 (#4126; 1:1000 5% milk), PPARgamma (#2435; 1:1000 5% milk), FAS (#3189; 1:1000 5% milk) and ACC (#3676S; 1:1000 5% milk) were from Cell Signaling (Danvers, MA, USA). Antibodies for PLIN1 (ab3526; 1:1000 5% milk) were from Abcam (Cambridge, MA, USA), PLIN2 (#610102; 1:1000 in 3% milk), and PLIN 5 (#GP31; 1:1000 in 3% milk) were purchased from Progen (Heidleberg, Germany. PLIN3 (#3883, CA; 1:1000 in 1% BSA) was purchased from ProSci Inc. (Poway, CA), and cytochrome c oxidase complex 4 (COX IV) (#MS407, TO, 1.64: 5000 in 5% milk) was purchased from MitoScience (Eugene, OR). ECL Plus was a product of Amersham Pharmacia Biotech (Arlington Heights, IL). Enhanced chemiluminescent reagents were from Perkin Elmer (Woodbridge, ON). Non-esterified free fatty acid (NEFA) kits were purchased from Wako Chemicals ((#995-34791, 995-34791, 993-35191, 991-34891, Richmond, VA).
Animals
Male Sprague-Dawley rats (N = 20, age 51–53 days) were purchased from Charles River Laboratories (Quebec, Canada). Rats were randomly assigned to either the endurance trained (ET: 256 ± 1.2g) or sedentary conditions (SED: 264.5 ± 2.8 g) (n = 10/group) and pair housed with another rat in the same condition. All animals were housed in a reverse 12:12 light-dark cycle and all had ad libitum access to food and standard rodent chow (Tekland Global 14% protein, Harlan Tekland Global, Mississauga, ON, Canada). The rats were housed in the Brock University Comparitive Biosciences Facility and all procedures and protocols were approved by the Animal Care Committee at Brock University and conformed to the Canadian Council on Animal Care guidelines.Citation43
Endurance training
Following 48 hours of acclimatization to the reverse light cycle, rats commenced endurance training as adapted from previous protocols.Citation44,45 Briefly, in week 1 rats ran on a treadmill for 30 min at a speed of 18m/min with a 10% incline and progressed to 60 min of running at a 10% incline at a speed of 25 m/min at the end of the 8 week intervention. This training protocol induces the expected increases in markers of mitochondrial content in skeletal muscle.Citation32 Two weeks into the training protocol one endurance-trained rat was omitted from training due to non-compliance.
Tissue collection
To avoid effects of the last exercise bout rats were anaesthetized 48 hours after the last training session with an inducible flow rate of 5% isoflurane and maintained at 3–5% for the duration of the surgeries.Citation46 Epididymal (eWAT), inguinal (iWAT), and intrascapular brown (BAT) adipose tissue depots were harvested, snap frozen in liquid nitrogen, and stored at −80°C. Intra-thoracic blood was collected, centrifuged 4°C (15 min at 3000g), and plasma was collected and stored at −80°C for further analysis.
Plasma fatty acids
The analysis of non-esterified fatty acid concentration was determined using a commercially available kit, following the manufacturer's instructions. Samples were run in duplicate and the average CV was <10%.
Western blotting
Adipose tissue from each depot was homogenized in buffer (10 mM Tris Cl, pH 7.5, 1 mM EGTA, 1 mM EDTA, 100 mM KCl, 250mM Sucrose) or NP-40 cell lysis buffer (3 volumes for iWAT and eWAT, 6 volumes for BAT), keeping samples on ice at all times. Homogenates were centrifuged (10 min at 5000–6000 g at 4°C), the infranatant was collected, and protein content determined. Samples were prepared to contain equal concentrations of protein that were then separated on SDS-PAGE gels (5–15%) at 120V for 60 min and wet transferred onto polyvinylidene fluoride (PVDF) or nitrocellulose membranes at 100V/transfer unit. Membranes were blocked in tris buffered saline/0.1% tween 20 (TBST) prepared with 5% non-fat dry milk or 5% BSA for 1 hour and then incubated overnight with the appropriate primary antibody. Following primary incubation, membranes were rinsed with TBST and incubated with the appropriate horseradish peroxidase-conjugated secondary antibodies for 1h at room temperature. Signals were detected using enhanced chemiluminesence and were then quantified by band densitometry using a FluorChem HD imaging system (Alpha Innotech, 166 Santa Clara, CA) and Image J software (http://rsbweb.nih.gov/ij/). Equal protein loading was evaluated via the total protein stain, Ponceau S, and variablility between lanes was less than 10%.Citation48,49 The data from the ET group was then expressed as a fold change relative to the SED.
Statistical analysis
Data are reported as means ± standard error (SE). Data was analyzed using a students unpaired t-test to determine differences between groups. Statistical significance was set at p < 0.05. Statistical analyses were performed using Prism statistical software (GraphPad Prism; La Jolla, CA).
Discussion
This study is the first to investigate the effects of endurance training on PLIN protein content across 3 metabolically distinct adipose tissue depots, epidydimal eWAT, iWAT and BAT. Novel findings from this study demonstrate that PLIN5 protein content increases in BAT with endurance training and PLIN3 increases in iWAT, while PLIN protein content does not change in eWAT. To determine if changes in PLIN protein content occur at the same time as changes in lipolytic or lipogenic proteins across the 3 different depots this study further investigated proteins involved in lipolysis and lipogenesis. Interestingly, eWAT was the only depot to show robust changes in lipolytic proteins.
PLIN5 is increased with endurance training in BAT
The high PLIN5 protein content in oxidative tissuesCitation22 has lead to the hypothesis for an involvement of PLIN5 in fatty acid oxidation. Here, we report greater PLIN5 content in BAT from endurance-trained rats compared to sedentary with unaltered mitochondrial markers. Interestingly, previous studies examining the effects of endurance exercise on BAT have found contradictory findings with some studies showing that BAT is not responsive to exercise,Citation49-52 while more recent studies have demonstrated that endurance training results in increased expression of genes related to mitochondrial biogenesis/function-related as well as fatty acid transport and metabolism in this tissue.Citation6,15,17 In skeletal muscle, PLIN5 appears to be involved in regulating fatty acid flux to the mitochondria as mitochondrial PLIN5 protein content is increased in the mitochondrial fraction of skeletal muscle following acute in-vivo stimulated contraction in sedentary and endurance trained rats.Citation32 Further, skeletal muscle PLIN5 content is increased along with mitochondrial markers with chronic endurance training.Citation32 In the present study the increase in BAT PLIN5 content was not accompanied by increases in mitochondrial proteins. It is possible that increases in PLIN5 content occur prior to increases in mitochondrial protein expression. For example, PLIN5 overexpression in rat tibialis anterior via gene electroporation promoted the expression of genes involved in fatty acid catabolism and mitochondrial oxidation.Citation29
In addition to the mitochondrial association, PLIN5 has been shown to interact with several lipolytic proteins (ATGL, CGI-58, and HSL) at rest and following mechanical and hormonally stimulated lipolysis in skeletal muscle.Citation48,53–55 It is of interest to note that although there was an increase in PLIN5 content in BAT, ATGL content remained unchanged. This might indicate that in BAT, PLIN5 may not be entirely associated with ATGL, as PLIN5 content increased independently of ATGL content. The higher PLIN5 to ATGL ratio may further indicate a tighter regulation of ATGL activity. Further work is needed to determine whether a direct PLIN5-ATGL protein interaction is present in BAT, as previously reported in CHO-K1 cells and skeletal muscle, in addition to whether PLIN5 is associated with other intracellular proteins.Citation48,56
It has also been speculated that PLIN5 plays a role in lipid accumulation, as overexpression in rat tibialis anterior results in an increase in triglyceride accumulation.Citation28 We observed a lower acetyl CoA carboxylase (ACC) content in parallel with a higher PLIN5 content in BAT from trained rats. Interestingly, acute exercise induces AMPK activation and decreases the activities of a number of enzymes involved in lipid synthesis, including ACC.Citation57 The cumulative affects each exercise bout may result in an overall increase in fat oxidation and a decrease in synthesis. Here we reported an increased PLIN5 protein content and decreased ACC protein content in BAT, however there was no significant correlation between these 2 proteins.
PLIN5 content is detected in eWAT and increased with endurance training
Interestingly, PLIN5 protein content was undetectable in iWAT and detectable in eWAT. Moreover, our results suggest a trend for increased PLIN5 content in the endurance trained group when compared to the sedentary group. Our results are contrary to Wolins and colleagues (Wolins et al., 2006) who reported PLIN5 content in iWAT and none in eWAT. This discrepancy could be due to the different animal models used as Wolins and colleagues compZleted their PLIN5 measure in C57BL/6 mice and the current study was completed in Sprague Dawley rats. Future work should aim to determine whether there are metabolic differences between rodent species.
PLIN3 is increased in iWAT with endurance training
This study demonstrates an increase in PLIN3 content in iWAT following endurance training. This is in agreement with a previous study that showed higher PLIN3 content in iWAT following a 16-week endurance training protocol in women with polycystic ovarian syndrome.Citation42 The exact role of PLIN3 in lipid metabolism is unknown, however evidence indicates that it may be involved in the regulation of lipolysis and potentially fatty acid oxidation. In skeletal muscle, PLIN3 interacts with ATGL, HSL and CGI-58,Citation48 demonstrating a potential role in lipolysis. In the current study the increase in PLIN3 content occurred without changes in any of the lipolytic or lipogenic proteins in this depot. Like PLIN5, PLIN3 also interacts with mitochondria in skeletal muscle.Citation31,32 However, there were no changes in mitochondrial proteins in this depot following endurance training, making it difficult to determine if PLIN3 plays a similar role in adipose tissue as it might in skeletal muscle. It is possible that due to the low/undetectable expression of PLIN5 in this adipose tissue depot that PLIN3 plays a similar role as PLIN5. Future work is needed to understand what role the adipose depot specific differences in PLIN proteins have in overall lipid metabolism. Future work should also determine if PLIN3 and PLIN5 are associated with mitochondria in adipose tissue, as they are in skeletal muscle.Citation31,32
Endurance training did not alter PLIN protein content in eWAT
Endurance training did not result in any change in PLIN protein content in eWAT. However, 8 weeks of training did result in robust increases in lipolytic proteins (ATGL and CGI-58). Lipolytic activity of adipose cells has been shown to vary according to the anatomic location of the fat depot from which the cells have been isolated.Citation58 When comparing rat subcutaneous adipose depots (inguinal or dorsal-subcutaneous) to visceral depots (epididymal, parametrial, or retroperitoneal), there is a lower rate of basal and stimulated lipolysis in subcutaneous adipose depots.Citation58 It is possible that these variations in lipolytic activity may be due to depot specific variations in the expression of lipolytic proteins, such ATGL.
There was no change in PLIN1 protein content in any of the adipose tissue depots post-training. To our knowledge, few studies have examined the effects of exercise training on adipose tissue PLIN1 protein content. Stephenson et al. demonstrated that 6 weeks of endurance running resulted in an increased eWAT PLIN1 protein content in high intrinsic running capacity rats when compared to low intrinsic running capacity rats.Citation59
Conclusion
In summary, this study demonstrates that there is a PLIN protein adipose tissue depot specific response to endurance training. Novel findings from this study show that in response to endurance training PLIN5 increases in BAT while PLIN3 increases in iWAT. Although eWAT appeared to be unresponsive to endurance training in terms of the PLIN proteins, it yielded large increases in lipolytic proteins ATGL, pATGL and CGI-58. PLIN5 is typically most associated with oxidative tissues, yet we witness no increases in proteins associated with oxidation, therefore it appears that PLIN5 may indicate an early response to endurance training. As PLIN5 is not expressed in iWAT, it is possible that the increase in PLIN3 in iWAT may also be an early response to endurance training. Future work is needed to fully elucidate these depot specific responses to endurance training in order to produce a clear understanding of these PLIN proteins in adipose tissue.
Disclosure of potential conflicts of interest
No potential conflicts of interest were disclosed.
Author contributions
PCT, SVR, and REKM conceived and designed the experiments. PCT and SVR performed the animal training and experiments and harvested tissues. REKM and SVR collected and assembled the biochemical data. PCT, SVR, and REKM analyzed and interpreted the data. PCT, SVR, and REKM drafted the manuscript. All authors revised the article for intellectual content and approved the final version of the manuscript. All persons designated as authors qualify for authorship, and all those who qualify for authorship are listed.
Acknowledgments
We would like to acknowledge Dr. S.J. Peters and Dr. DC. Wright for their support of this research.
Funding
S.J. Peters is funded by a Natural Sciences and Engineering Research Council of Canada (NSERC) grant. DCW is a Tier II Canada Research Chair and is supported by an Operating Grant from the Canadian Institutes of Health. REK is funded by a Postdoctoral Fellowship from the Alzheimer Society. SV Ramos is funded by an Ontario Graduate Student Scholarship.
References
- Stanford KI, Middelbeek RJ, Townsend KL, An D, Nygaard EB, Hitchcox KM, Markan KR, Nakano K, Hirshman MF, Tseng YH, Goodyear LJ. Brown adipose tissue regulates glucose homeostasis and insulin sensitivity. J Clin Investig 2013; 123:215-23; PMID:23221344; http://dx.doi.org/10.1172/JCI62308
- Stanford KI, Middelbeek RJ, Townsend KL, Lee MY, Takahashi H, So K, Hitchcox KM, Markan KR, Hellbach K, Hirshman MF, et al. A Novel Role for Subcutaneous Adipose Tissue in Exercise-Induced Improvements in Glucose Homeostasis. Diabetes 2015; 64(6):2002-14; PMID: 25605808
- Wu MV, Bikopoulos G, Hung S, Ceddia RB. Thermogenic capacity is antagonistically regulated in classical brown and white subcutaneous fat depots by high fat diet and endurance training in rats: impact on whole-body energy expenditure. J Biol Chem 2014; 289:34129-40; PMID:25344623; http://dx.doi.org/10.1074/jbc.M114.591008
- Tran TT, Kahn CR. Transplantation of adipose tissue and stem cells: role in metabolism and disease. Nat Rev Endocrinol 2010; 6:195-213; PMID:20195269; http://dx.doi.org/10.1038/nrendo.2010.20
- Himms-Hagen J. Brown adipose tissue thermogenesis: interdisciplinary studies. The FASEB journal : official publication of the Federation of American Societies for Experimental Biology 1990; 4:2890-8; PMID:NOT_FOUND
- Pistor KE, Sepa-Kishi DM, Hung S, Ceddia RB. Lipolysis, lipogenesis, and adiposity are reduced while fatty acid oxidation is increased in visceral and subcutaneous adipocytes of endurance-trained rats. Adipocyte 2015; 4:22-31; PMID:26167399; http://dx.doi.org/10.4161/21623945.2014.955423
- Brown DA. Lipid droplets: proteins floating on a pool of fat. Curr Biol 2001; 11:R446-449; PMID:11516669; http://dx.doi.org/10.1016/S0960-9822(01)00257-3
- Brasaemle DL. Thematic review series: adipocyte biology. The perilipin family of structural lipid droplet proteins: stabilization of lipid droplets and control of lipolysis. J Lipid Res 2007; 48:2547-59; PMID:17878492; http://dx.doi.org/10.1194/jlr.R700014-JLR200
- Tansey JT, Sztalryd C, Hlavin EM, Kimmel AR, Londos C. The central role of perilipin a in lipid metabolism and adipocyte lipolysis. IUBMB life 2004; 56:379-85; PMID:15545214; http://dx.doi.org/10.1080/15216540400009968
- Londos C, Sztalryd C, Tansey JT, Kimmel AR. Role of PAT proteins in lipid metabolism. Biochimie 2005; 87:45-9; PMID:15733736; http://dx.doi.org/10.1016/j.biochi.2004.12.010
- Ducharme NA, Bickel PE. Lipid droplets in lipogenesis and lipolysis. Endocrinology 2008; 149:942-9; PMID:18202123; http://dx.doi.org/10.1210/en.2007-1713
- Liu P, Ying Y, Zhao Y, Mundy DI, Zhu M, Anderson RG. Chinese hamster ovary K2 cell lipid droplets appear to be metabolic organelles involved in membrane traffic. J Biol Chem 2004; 279:3787-92; PMID:14597625; http://dx.doi.org/10.1074/jbc.M311945200
- Wolins NE, Quaynor BK, Skinner JR, Tzekov A, Croce MA, Gropler MC, Varma V, Yao-Borengasser A, Rasouli N, Kern PA, et al. OXPAT/PAT-1 is a PPAR-induced lipid droplet protein that promotes fatty acid utilization. Diabetes 2006; 55:3418-28; PMID:17130488; http://dx.doi.org/10.2337/db06-0399
- Gollisch KS, Brandauer J, Jessen N, Toyoda T, Nayer A, Hirshman MF, Goodyear LJ. Effects of exercise training on subcutaneous and visceral adipose tissue in normal- and high-fat diet-fed rats. Am J Physiol Endocrinol Metab 2009; 297:E495-504; PMID:19491293; http://dx.doi.org/10.1152/ajpendo.90424.2008
- Xu X, Ying Z, Cai M, Xu Z, Li Y, Jiang SY, Tzan K, Wang A, Parthasarathy S, He G, Rajagopalan S, Sun Q. Exercise ameliorates high-fat diet-induced metabolic and vascular dysfunction, and increases adipocyte progenitor cell population in brown adipose tissue. Am J Physiol Regul Integr Comp Physiol 2011; 300:R1115-25; PMID:21368268; http://dx.doi.org/10.1152/ajpregu.00806.2010
- Dinas PC, Nikaki A, Jamurtas AZ, Prassopoulos V, Efthymiadou R, Koutedakis Y, Georgoulias P, Flouris AD. Association between habitual physical activity and brown adipose tissue activity in individuals undergoing PET-CT scan. Clin Endocrinol (Oxf) 2015; 82:147-54; PMID:25262810; http://dx.doi.org/10.1111/cen.12620
- Slocum N, Durrant JR, Bailey D, Yoon L, Jordan H, Barton J, Brown RH, Clifton L, Milliken T, Harrington W, et al. Responses of brown adipose tissue to diet-induced obesity, exercise, dietary restriction and ephedrine treatment. Exp Toxicologic Pathol 2013; 65:549-57; PMID:22542811; http://dx.doi.org/10.1016/j.etp.2012.04.001
- Bostrom P, Wu J, Jedrychowski MP, Korde A, Ye L, Lo JC, Rasbach KA, Bostrom EA, Choi JH, Long JZ, et al. A PGC1-alpha-dependent myokine that drives brown-fat-like development of white fat and thermogenesis. Nature 2012; 481:463-8; PMID:22237023; http://dx.doi.org/10.1038/nature10777
- Miura S, Gan JW, Brzostowski J, Parisi MJ, Schultz CJ, Londos C, Oliver B, Kimmel AR. Functional conservation for lipid storage droplet association among Perilipin, ADRP, and TIP47 (PAT)-related proteins in mammals, Drosophila, and Dictyostelium. J Biol Chem 2002; 277:32253-7; PMID:12077142; http://dx.doi.org/10.1074/jbc.M204410200
- Kimmel AR, Brasaemle DL, McAndrews-Hill M, Sztalryd C, Londos C. Adoption of PERILIPIN as a unifying nomenclature for the mammalian PAT-family of intracellular lipid storage droplet proteins. J Lipid Res 2010; 51:468-71; PMID:19638644; http://dx.doi.org/10.1194/jlr.R000034
- Hsieh K, Lee YK, Londos C, Raaka BM, Dalen KT, Kimmel AR. Perilipin family members preferentially sequester to either triacylglycerol-specific or cholesteryl-ester-specific intracellular lipid storage droplets. Journal of cell science 2012; 125:4067-76; PMID:22685330; http://dx.doi.org/10.1242/jcs.104943
- Dalen KT, Dahl T, Holter E, Arntsen B, Londos C, Sztalryd C, Nebb HI. LSDP5 is a PAT protein specifically expressed in fatty acid oxidizing tissues. Biochimica et biophysica acta 2007; 1771:210-27; PMID:17234449; http://dx.doi.org/10.1016/j.bbalip.2006.11.011
- Brasaemle DL, Rubin B, Harten IA, Gruia-Gray J, Kimmel AR, Londos C. Perilipin A increases triacylglycerol storage by decreasing the rate of triacylglycerol hydrolysis. J Biol Chem 2000; 275:38486-93; PMID:10948207; http://dx.doi.org/10.1074/jbc.M007322200
- Miyoshi H, Souza SC, Zhang HH, Strissel KJ, Christoffolete MA, Kovsan J, Rudich A, Kraemer FB, Bianco AC, Obin MS, et al. Perilipin promotes hormone-sensitive lipase-mediated adipocyte lipolysis via phosphorylation-dependent and -independent mechanisms. J Biol Chem 2006; 281:15837-44; PMID:16595669; http://dx.doi.org/10.1074/jbc.M601097200
- Souza SC, Muliro KV, Liscum L, Lien P, Yamamoto MT, Schaffer JE, Dallal GE, Wang X, Kraemer FB, Obin M, Greenberg AS. Modulation of hormone-sensitive lipase and protein kinase A-mediated lipolysis by perilipin A in an adenoviral reconstituted system. J Biol Chem 2002; 277:8267-8272; PMID:11751901; http://dx.doi.org/10.1074/jbc.M108329200
- Tansey JT, Huml AM, Vogt R, Davis KE, Jones JM, Fraser KA, Brasaemle DL, Kimmel AR, Londos C. Functional studies on native and mutated forms of perilipins. A role in protein kinase A-mediated lipolysis of triacylglycerols. J Biol Chem 2003; 278:8401-6; PMID:12477720; http://dx.doi.org/10.1074/jbc.M211005200
- Wang H, Sreenevasan U, Hu H, Saladino A, Polster BM, Lund LM, Gong DW, Stanley WC, Sztalryd C. Perilipin 5, a lipid droplet-associated protein, provides physical and metabolic linkage to mitochondria. J Lipid Res 2011; 52:2159-68; PMID:21885430; http://dx.doi.org/10.1194/jlr.M017939
- Bosma M, Minnaard R, Sparks LM, Schaart G, Losen M, de Baets MH, Duimel H, Kersten S, Bickel PE, Schrauwen P, et al. The lipid droplet coat protein perilipin 5 also localizes to muscle mitochondria. Histochem Cell Biol 2012; 137:205-16; PMID:22127648; http://dx.doi.org/10.1007/s00418-011-0888-x
- Bosma M, Sparks LM, Hooiveld GJ, Jorgensen JA, Houten SM, Schrauwen P, Kersten S, Hesselink MK. Overexpression of PLIN5 in skeletal muscle promotes oxidative gene expression and intramyocellular lipid ?content without compromising insulin sensitivity. ?Biochimica et biophysica acta 2013; 1831:844-52; PMID:23353597; http://dx.doi.org/10.1016/j.bbalip.2013.01.007
- Yamaguchi T, Matsushita S, Motojima K, Hirose F, Osumi T. MLDP, a novel PAT family protein localized to lipid droplets and enriched in the heart, is regulated by peroxisome proliferator-activated receptor alpha. J Biol Chem 2006; 281:14232-40; PMID:16571721; http://dx.doi.org/10.1074/jbc.M601682200
- Ramos SV, MacPherson RE, Turnbull PC, Bott KN, LeBlanc P, Ward WE, Peters SJ. Higher PLIN5 but not PLIN3 content in isolated skeletal muscle mitochondria following acute in vivo contraction in rat hindlimb. Physiological reports 2:2014; PMID:25318747; http://dx.doi.org/10.14814/phy2.12154
- Ramos SV, Turnbull PC, MacPherson RE, LeBlanc PJ, Ward WE, Peters SJ. Changes in mitochondrial perilipin 3 and perilipin 5 protein content in rat skeletal muscle following endurance training and acute stimulated contraction. Exp Physiol 2015; 100:450-62; PMID:25663294; http://dx.doi.org/10.1113/expphysiol.2014.084434
- Shepherd SO, Cocks M, Tipton KD, Ranasinghe AM, Barker TA, Burniston JG, Wagenmakers AJ, Shaw CS. Sprint interval and traditional endurance training increase net intramuscular triglyceride breakdown and expression of perilipin 2 and 5. J Physiol 2013; 591:657-75; PMID:23129790; http://dx.doi.org/10.1113/jphysiol.2012.240952
- Peters SJ, Samjoo IA, Devries MC, Stevic I, Robertshaw HA, Tarnopolsky MA. Perilipin family (PLIN) proteins in human skeletal muscle: the effect of sex, obesity, and endurance training. Applied physiology, nutrition, and metabolism = Physiologie appliquee, nutrition et metabolisme 2012; 37:724-35; PMID:22667335; http://dx.doi.org/10.1139/h2012-059
- Hocsak E, Racz B, Szabo A, Mester L, Rapolti E, Pozsgai E, Javor S, Bellyei S, Gallyas F, Jr., Sumegi B, Szigeti A. TIP47 protects mitochondrial membrane integrity and inhibits oxidative-stress-induced cell death. FEBS letters 2010; 584(13):2953-60; PMID:20556887
- Wolins NE, Rubin B, Brasaemle DL. TIP47 associates with lipid droplets. J Biol Chem 2001; 276:5101-8; PMID: 11084026; http://dx.doi.org/10.1074/jbc.M006775200
- Badin PM, Vila IK, Louche K, Mairal A, Marques MA, Bourlier V, Tavernier G, Langin D, Moro C. High-fat diet-mediated lipotoxicity and insulin resistance is related to impaired lipase expression in mouse skeletal muscle. Endocrinology 2013; 154:1444-53; PMID:23471217; http://dx.doi.org/10.1210/en.2012-2029
- Skinner JR, Shew TM, Schwartz DM, Tzekov A, Lepus CM, Abumrad NA, Wolins NE. Diacylglycerol enrichment of endoplasmic reticulum or lipid droplets recruits perilipin 3/TIP47 during lipid storage and mobilization. J Biol Chem 2009; 284:30941-8; PMID:19748893; http://dx.doi.org/10.1074/jbc.M109.013995
- Louche K, Badin PM, Montastier E, Laurens C, Bourlier V, de Glisezinski I, Thalamas C, Viguerie N, Langin D, Moro C. Endurance exercise training up-regulates lipolytic proteins and reduces triglyceride content in skeletal muscle of obese subjects. The Journal of clinical endocrinology and metabolism 2013; 98:4863-71; PMID:24178794; http://dx.doi.org/10.1210/jc.2013-2058
- Macpherson RE, Vandenboom R, Roy BD, Peters SJ. Skeletal muscle PLIN3 and PLIN5 are serine phosphorylated at rest and following lipolysis during adrenergic or contractile stimulation. Physiological reports 2013; 1:e00084; PMID:24303154; http://dx.doi.org/10.1002/phy2.84
- Covington JD, Galgani JE, Moro C, LaGrange JM, Zhang Z, Rustan AC, Ravussin E, Bajpeyi S. Skeletal muscle perilipin 3 and coatomer proteins are increased following exercise and are associated with fat oxidation. PloS one 2014; 9:e91675; PMID:24632837; http://dx.doi.org/10.1371/journal.pone.0091675
- Covington JD, Bajpeyi S, Moro C, Tchoukalova YD, Ebenezer PJ, Burk DH, Ravussin E, Redman LM. Potential effects of aerobic exercise on the expression of perilipin 3 in the adipose tissue of women with polycystic ovary syndrome: a pilot study. European journal of endocrinology / European Federation of Endocrine Societies 2015; 172:47-58; PMID:25342854; http://dx.doi.org/10.1530/EJE-14-0492
- Olfert EDC, BM, McWilliam AA. Guide to the Care and Use of Experimental Animals, 2th ed. Vol. 1 1993, Ottawa, ON, Canada.
- Dyck DJ, Miskovic D, Code L, Luiken JJ, Bonen A. Endurance training increases FFA oxidation and reduces triacylglycerol utilization in contracting rat soleus. Am J Physiol Endocrinol Metab 2000; 278:E778-785; PMID:10780932
- Leblanc PJ, Mulligan M, Antolic A, Macpherson L, Inglis JG, Martin D, Roy BD, Peters SJ. Skeletal muscle type comparison of pyruvate dehydrogenase phosphatase activity and isoform expression: effects of obesity and endurance training. Am J Physiol Regul Integr Comp Physiol 2008; 295:R1224-30; PMID:18716035; http://dx.doi.org/10.1152/ajpregu.90320.2008
- Spriet LL, Heigenhauser GJ, Jones NL. Endogenous triacylglycerol utilization by rat skeletal muscle during tetanic stimulation. J Appl Physiol 1986; 60(1985):410-5; PMID:3949645
- Romero-Calvo I, Ocon B, Martinez-Moya P, Suarez MD, Zarzuelo A, Martinez-Augustin O, de Medina FS. Reversible Ponceau staining as a loading control alternative to actin in Western blots. Analytical Biochem 2010; 401:318-20; PMID:20206115; http://dx.doi.org/10.1016/j.ab.2010.02.036
- MacPherson RE, Ramos SV, Vandenboom R, Roy BD, Peters SJ. Skeletal muscle PLIN proteins, ATGL and CGI-58, interactions at rest and following stimulated contraction. Am J Physiol Regul Integr Comp Physiol 2013; 304:R644-50; PMID:23408028; http://dx.doi.org/10.1152/ajpregu.00418.2012
- Scarpace PJ, Yenice S, Tumer N. Influence of exercise training and age on uncoupling protein mRNA expression in brown adipose tissue. Pharmacol Biochem Behav 1994; 49:1057-9; PMID:7886075; http://dx.doi.org/10.1016/0091-3057(94)90264-X
- Segawa M, Oh-Ishi S, Kizaki T, Ookawara T, Sakurai T, Izawa T, Nagasawa J, Kawada T, Fushiki T, Ohno H. Effect of running training on brown adipose tissue activity in rats: a reevaluation. Res Commun Mol Pathol Pharmacol 1998; 100:77-82; PMID:9644721
- Shibata H, Nagasaka T. The effect of forced running on heat production in brown adipose tissue in rats. Physiology & behavior 1987; 39:377-80; PMID:3575479; http://dx.doi.org/10.1016/0031-9384(87)90238-1
- Wickler SJ, Stern JS, Glick Z, Horwitz BA. Thermogenic capacity and brown fat in rats exercise-trained by running. Metabolism 1987; 36:76-81; PMID:3796299; http://dx.doi.org/10.1016/0026-0495(87)90067-9
- Raben DM, Baldassare JJ. A new lipase in regulating lipid mobilization: hormone-sensitive lipase is not alone. Trends Endocrinol Metab 2005; 16:35-6; PMID:15734142; http://dx.doi.org/10.1016/j.tem.2005.01.009
- Schweiger M, Schreiber R, Haemmerle G, Lass A, Fledelius C, Jacobsen P, Tornqvist H, Zechner R, Zimmermann R. Adipose triglyceride lipase and hormone-sensitive lipase are the major enzymes in adipose tissue triacylglycerol catabolism. J Biol Chem 2006; 281:40236-41; PMID:17074755; http://dx.doi.org/10.1074/jbc.M608048200
- Zimmermann R, Strauss JG, Haemmerle G, Schoiswohl G, Birner-Gruenberger R, Riederer M, Lass A, Neuberger G, Eisenhaber F, Hermetter A, et al. Fat mobilization in adipose tissue is promoted by adipose triglyceride lipase. Science 2004; 306:1383-6; PMID:15550674; http://dx.doi.org/10.1126/science.1100747
- Wang H, Bell M, Sreenivasan U, Hu H, Liu J, Dalen K, Londos C, Yamaguchi T, Rizzo MA, Coleman R, Gong D, Brasaemle D, Sztalryd C. Unique regulation of adipose triglyceride lipase (ATGL) by perilipin 5, a lipid droplet-associated protein. J Biol Chem 2011; 286:15707-15; PMID:21393244; http://dx.doi.org/10.1074/jbc.M110.207779
- Park H, Kaushik VK, Constant S, Prentki M, Przybytkowski E, Ruderman NB, Saha AK. Coordinate regulation of malonyl-CoA decarboxylase, sn-glycerol-3-phosphate acyltransferase, and acetyl-CoA carboxylase by AMP-activated protein kinase in rat tissues in response to exercise. J Biol Chem 2002; 277:32571-7; PMID:12065578; http://dx.doi.org/10.1074/jbc.M201692200
- Sztalryd C, Kraemer FB. Differences in hormone-sensitive lipase expression in white adipose tissue from various anatomic locations of the rat. Metabolism 1994; 43:241-7; PMID:8121309; http://dx.doi.org/10.1016/0026-0495(94)90252-6
- Stephenson EJ, Lessard SJ, Rivas DA, Watt MJ, Yaspelkis BB, 3rd, Koch LG, Britton SL, Hawley JA. Exercise training enhances white adipose tissue metabolism in rats selectively bred for low- or high-endurance running capacity. Am J Physiol Endocrinol Metab 2013; 305:E429-38; PMID:23757406; http://dx.doi.org/10.1152/ajpendo.00544.2012