ABSTRACT
Communication between the brain and the adipose tissue has been the focus of many studies in recent years, with the “brain-fat axis” identified as a system that orchestrates the assimilation and usage of energy to maintain body mass and adequate fat stores. It is now well-known that appetite-regulating peptides that were studied as neurotransmitters in the central nervous system can act both on the hypothalamus to regulate feeding behavior and also on the adipose tissue to modulate the storage of energy. Energy balance is thus partly controlled by factors that can alter both energy intake and storage/expenditure. Two such factors involved in these processes are neuropeptide Y (NPY) and α-melanocyte stimulating hormone (α-MSH). NPY, an orexigenic factor, is associated with promoting adipogenesis in both mammals and chickens, while α-MSH, an anorexigenic factor, stimulates lipolysis in rodents. There is also evidence of interaction between the 2 peptides. This review aims to summarize recent advances in the study of NPY and α-MSH regarding their role in adipose tissue physiology, with an emphasis on the cellular and molecular mechanisms. A greater understanding of the brain-fat axis and regulation of adiposity by bioactive peptides may provide insights on strategies to prevent or treat obesity and also enhance nutrient utilization efficiency in agriculturally-important species.
Introduction
Many different factors are involved in regulating adipose tissue physiology. Because white adipose tissue is comprised of many different cell types, including preadipocytes, adipocytes, blood cells, immune cells, and mesenchymal stem cells among others, and is innervated by the sympathetic nervous system and is highly vascularized, there are multiple routes through which factors can act on cells in the adipose tissue (neural, endocrine, paracrine, autocrine, etc.) and numerous cells that play a role in the acquisition and storage of energy, including the adipocyte that stores triacylglycerols and cholesterol esters in lipid droplets that occupy most of the volume of the cell. The majority of studies in adipose physiology focus on mammalian models, with fewer studies in birds, however, with the ease of availability of chickens and the bulk of avian research being conducted in the domestic chicken, it has emerged as an attractive model for understanding the relationship between appetite regulation and adipocyte lipid metabolism.
Two peptides that play a major role in regulating food intake are neuropeptide Y (NPY) and α-melanocyte stimulating hormone (α-MSH). These factors are regarded as neurotransmitters, however because most studies do not distinguish between actions in vivo that originate centrally or peripherally (both factors and their receptors are produced in adipose tissue and both are found in the circulation), they will be referred to as “peptides” for the remainder of this review. NPY is highly orexigenic in many species, and its receptor sub-types are well characterized in mammals,Citation1 while α-MSH is anorexigenic and is part of the melanocortin system. The two peptides are also associated with each other, with the NPY system acting to regulate the activity of α-MSH in the hypothalamus by decreasing its phosphorylation capacity and amount present in the paraventricular nucleus (PVN).Citation2 Both peptides also affect adipose physiology; NPY mainly affects adipogenesis, while α-MSH primarily affects lipolysis.
Neuropeptide Y, a 36 amino acid peptide, was first isolated from the pig brain,Citation3 and its orexigenic properties have been studied in a multitude of species, including rodents,Citation4 rabbits,Citation5 teleost fish,Citation6 white-crowned sparrows,Citation7 and chickens.Citation8,9 It is involved in promoting adipogenesis in mouse 3T3-L1 cells,Citation10 and chicken preadipocytes.Citation11
Adrenocorticotropic hormone (ACTH) is a peptide derived from pro-opiomelanocortin (POMC), and α-MSH is then further derived from ACTH, as the first 13 amino acids of ACTH are what make up α-MSH.Citation12 It is involved in energy balance and is an agonist to 4 out of 5 melanocortin receptors (MCR).Citation13 It is also anorexigenic in multiple species, including rats,Citation14 goldfish,Citation15 and chicks.Citation16 A lipolytic effect mediated through MC5R was reported in mouse 3T3-L1 cells,Citation17 but is yet to be determined in chicken or any other avian species.
Although our group recently published a review on hypothalamus-adipose tissue crosstalk with a focus on NPY,Citation18 many developments have since been reported on the study of appetite-regulating factors and their role in adipose tissue metabolism. The purpose of this review is to incorporate recent information about the actions of NPY and α-MSH in adipose tissue, with an emphasis on the molecular mechanisms. Additionally, because the information reviewed is in the subject of adipose physiology; adipogenesis, adipose tissue expansion, and models used for studying adipogenesis are also discussed.
Adipogenesis and adipose tissue expansion
Adipose cells originate from mesenchymal stem cells, which are found in the adipose tissue.Citation19 Although accepted as being of mesenchymal origin, adipose cells are also derived from areas outside of the mesoderm such as the neural crest (NC).Citation20,21 Mesenchymal stem cells can differentiate into a multitude of different cell types, including adipocytes, chondrocytes, osteoblasts, and myoblastsCitation22-28 as well as neuron-like cells,Citation29-31 with considerable transcriptional regulation by a diverse array of factors to determine the route of differentiation. Adipogenesis encompasses the many steps of differentiation; from a stem cell being determined to become a preadipocyte to the preadipocyte during the early (clonal expansion) and late (terminal) stages of differentiating into the fully mature adipocyte.Citation32 For the remainder of this review, differentiation will refer to terminal differentiation of the adipocyte, unless stated otherwise.
Transcription factors and adipocyte differentiation
There are several major transcription factors that have been widely studied for their role in adipogenesis, with mechanisms underlying their actions well-understood in mammals. The transcription factors discussed herein and the times during differentiation that they are expressed are described in .
Table 1. Transcription factors involved in adipocyte differentiation.
Peroxisome proliferator activated receptor gamma (PPARγ) is part of the nuclear receptor superfamily, and is known as the “master regulator” of preadipocyte differentiation,Citation33 and is necessary for development of adipose tissue.Citation34 Expression is induced early in differentiation,Citation35 and induced expression in fibroblasts can cause differentiation into adipocytes,Citation36 as well as trans-differentiation of myoblasts into adipocytes.Citation37 Expression after adipocyte maturity is also important, as 3T3-L1 cells containing a dominant-negative PPARγ, a mutant form that is able to bind DNA but not ligands, are induced to de-differentiate.Citation38 Knockout (KO) of PPARγ in adipocytes in vivo leads to adipocyte death due to necrosis, which then stimulates the generation of new adipocytes,Citation39 cells that the authors hypothesized were derived from fibroblast-like cells; adipose progenitor cells are widely distributed in connective tissue and possess the ability to proliferate and differentiate into adipocytes.Citation39,40
Another transcription factor, sterol regulatory element binding protein (SREBP), plays a role in adipogenesis. Its main function is to control expression of enzymes involved in cholesterol, fatty acid, triacylglycerol, and lipid synthesis, as reviewed.Citation41 SREBP 1 and 2 activate transcription of more than 30 genes that are related to lipid synthesis and transport, particularly in the liver.Citation42,43 Diet-derived polyunsaturated fatty acids suppress hepatic expression of genes contributing to fatty acid synthesis, with SREBP 1 and 2 purported to be involved.Citation43 SREBP increases the activity of PPARγ by producing endogenous ligands that bind to the ligand-binding domain of PPARγ, as conditioned media from NIH 3T3 cells expressing SREBP activated PPARγ;Citation44 although it was not specified which specific endogenous ligands were produced, authors noted that secreted lipid(s) in the fatty acid family serve as endogenous ligands of PPARγ.Citation44-47 SREBP is also involved in insulin-mediated signaling to induce lipid accumulation by increasing expression of the lipogenic genes fatty acid synthase (FAS) and lipoprotein lipase (LPL).Citation48
The CCAAT enhancer binding protein (C/EBP) family of transcription factors is also important during adipocyte differentiation, with early expression of C/EBPβ and C/EBPδ during the first 2 days of differentiation leading to induction of C/EBPα.Citation32,49,50 C/EBPα KO mice die shortly after birth, but when expression is limited to the liver they survive with greatly reduced amounts of white adipose tissue.Citation51 When C/EBPα null mice have the C/EBPα gene replaced with C/EBPβ, leading to expression of C/EBPβ from the C/EBPα gene locus in addition to its own endogenous expression, mice are able to survive, but amounts of white adipose tissue are still reduced,Citation52 demonstrating that C/EBPβ is not sufficient to fully replace the function of C/EBPα in regulating adipose mass, although it is sufficient for survival.
While the C/EBP's are important for differentiation, they cannot induce differentiation without PPARγ. While C/EBPα deficient mice produce less adipose, they also do not induce endogenous PPARγ gene transcription, as fibroblast cell lines isolated from C/EBPα KO mice lack expression of PPARγ that is rescued by partially restoring expression of C/EBPα with a retroviral vector expressing C/EBPα;Citation53 thus showing the importance of communication between these 2 transcription factors. Additionally, treating mouse embryo fibroblasts (MEF) lacking a functional PPARγ gene with C/EBPβ does not induce expression of C/EBPα,Citation54 further supporting the role of communication between the C/EBP's and PPARγ in orchestrating adipocyte differentiation.
Adipose depots
Adipose tissue is divided into 2 major types, white adipose tissue (WAT) and brown adipose tissue (BAT), with WAT being an energy storage and endocrine tissue,Citation55 and BAT being involved in the production of heat, present in greater amounts in neonates and hibernators.Citation56,57 Existence of BAT is still questionable in avian species,Citation58-61 and is not reviewed here. The two main classifications of WAT depots in humans are subcutaneous adipose tissue and visceral adipose tissue, the visceral adipose tissue being the adipose surrounding the inner organs.Citation62
The different depots have many distinctions including differences in cellularity, adipokine production, and gene expression, as recently reviewed.Citation63 In the developing chicken embryo, fat is first deposited subcutaneously,Citation64 and develops through a combination of hyperplasia and hypertrophy.Citation65 Subcutaneous and clavicular fat depots are heavier than abdominal fat at 4 days post-hatch,Citation66 but as a percentage of body weight, the amount of abdominal fat increases with age.Citation66 It is unclear if birds are similar to humans in regards to metabolic differences between adipose tissue depots, although we have reported that there are differences in gene expression between adipose depots in chickens.Citation66-68
In addition to the differences we have reported in meat-type broiler chickens, we have also found adipose depot differences in a line of chickens selected for high and low body weight, the Virginia lines of chickens, which have undergone divergent selection for either low (LWS) or high (HWS) juvenile body weight at 56 days of age for 58 generations. This selection has resulted in a 10-fold difference in body weight at age of selection, with associated physiological and behavioral differences in feeding, adiposity, development, and body composition, with the LWS being lean and hypophagic, while the HWS are obese and hyperphagic as juveniles.Citation69-72 The LWS has greater PPARγ mRNA than HWS in the subcutaneous and clavicular adipose tissue, and pyruvate dehydrogenase kinase 4 (PDK4), and forkhead box 01A (FOX01), 2 enzymes important in metabolic flexibility,Citation73-77 also differ in expression between the lines, with greater expression of PDK4 in the clavicular adipose of the LWS and subcutaneous adipose of the HWS, and greater expression of FOX01 in the subcutaneous adipose of the HWS.Citation68 These differences in adipose depot expression of metabolic factors may contribute to the differences in adiposity of these chicken lines.
Models for studying adipogenesis
With the adipose being such a diverse tissue with numerous cell types present, and cross-talk with other systems in the body, many different models have emerged for studying the adipose tissue. A popular practice for in vitro studies is the use of cell lines. A common preadipocyte model, the mouse preadipocyte 3T3-L1 cell line, is very widely used to study adipogenesis. Their ability to differentiate into adipocytes and the ease through which different experiments can be conducted make them an attractive model. However, information on how these cells communicate with other cell types normally found in close proximity in vivo is impossible to determine through studies with single cell types. Cell lines are not available for every species, thus many studies in alternative animal models make use of a primary cell culture model to study adipogenesis.
Using 3T3-L1 cells allows for the study of a single cell type free of contamination by other cells that are present in the tissue. On the other hand, when generating a primary cell culture model, isolating one cell type proves more difficult especially in the absence of cell sorting technology, and thus there are usually multiple cell types present. There are numerous cell lines available, or types of cells and cell groups that can be isolated from a primary cell model, as noted in an excellent review on cell models used to study differentiation.Citation78 Additionally, while some protocols enrich for a specific cell type through isolation, other primary cell models enrich for a certain cell type through the media containing known factors that either push cells toward a certain goal, like differentiation, or enrich for a particular cell type. For example, a study by Billon et al., 2007, used a primary and secondary culture method of developing quail neural crest (NC) cells. Although these cells are not preadipocytes, they found that by including specific factors in the media cocktail, these cells were able to differentiate into adipocytes.Citation20 Another example would be isolating cells from the stromal vascular fraction (SVF), the cells isolated after completion of enzymatic digestion, centrifugation, cellular filtration, and red blood cell lysis of the adipose tissue,Citation79 a mixture of cells that include mesenchymal stem cells, preadipocytes, endothelial cells, and immune cells.Citation80-82 This technique has been applied in mice,Citation83 humans,Citation84 and chickens.Citation11
In addition to generating single cell suspensions for culture, whole tissue can also be cultured as explants. By isolating the tissue, but preserving the cross-talk between cells in the tissue, explants offer the benefit of more closely mimicking the in vivo environment of the cells.Citation85,86 As in vitro studies provide further understanding in fields of physiology, culturing whole tissue explants will become increasingly important to study conditions in vivo.
While there are numerous cell lines available for mammalian species, for avian species in vitro studies on adipogenesis often use a primary cell culture model. Some differences in methodology are adapted when studying differentiation, including using chicken serum (CS) in the differentiation cocktail, and using a combination of insulin, dexamethasone, and heparin.Citation87 The concentration of insulin is also greater than what is included in mammalian studies, because birds are naturally insulin resistant.Citation88,89 These studies are also conducted with tissue collected from the abdominal fat of young birds, usually 2–4 weeks old, because there is sufficient fat accumulation to yield ample cells for culture from a single chicken, and cell viability and proliferative capacity of adipose-derived mesenchymal stem cells decreases with age.Citation90 We also recently showed that cells isolated from birds at this age had robust proliferative capacity and ability to differentiate.Citation11
Factors that regulate both energy intake and storage in the animal
Identifying factors that regulate how adipose tissue is remodeled, as well as how energy is stored in different animals becomes increasingly important as more advances are made in understanding diseases related to the adipose tissue. The adipose is indeed an endocrine organ associated with the release of multiple hormones.Citation91 While these hormones are important in energy balance and function, crosstalk between the brain and hypothalamus, as well as the sympathetic nervous system is also important in providing reciprocal signals that serve as feedback loops in times of energy restriction or excess. Multiple studies from the Bartness group used the pseudorabies virus (PRV) to elucidate the brain network controlling the sympathetic innervation of WATCitation92 and BAT.Citation93 The PRV is a neurotropic virus that is able to infect neurons trans-synaptically, thus providing the ability to trace a neural network in a retrograde fashion when injected into a specific tissue.Citation94,95 In Siberian hamsters (a species that displays seasonal changes in appetite and adipose mass in response to alterations in photoperiod) and rats, they found that areas of the brain stem, midbrain, and hypothalamus were connected to the WAT and BAT, with the paraventricular nucleus (PVN) and medial preoptic area (MPA) in the hypothalamus showing greatest concentration of the virus in connection to the WAT and BAT.Citation92,93 They also showed that the WAT is not innervated by any parasympathetic pathways in Siberian hamsters, rats, or mice, an idea that has been contested.Citation96
Many neurotransmitters are expressed in areas other than neurons, and have effects on the fat. Serotonin is involved in regulating energy homeostasis, as KO and inhibition of tryptophan hydroxylase 1, one of the enzymes responsible for conversion of tryptophan into serotonin, causes a reduction in body weight gain, improved glucose tolerance, increased thermogenic activity in BAT and decreased lipogenesis in WAT.Citation97 Other amino acid neurotransmitters are also found in the adipose tissue; with immunohistochemical labeling used to detect gamma amino butyric acid (GABA), glutamate, the GABA producing enzyme glutamate decarboxylase, and transporters and receptors for glutamate and GABA.Citation98 There is also regulation by POMC peptides in adipose tissue to promote browning of WAT and lipolysis,Citation99,100 and sympathetic innervation of the adipose tissue.Citation101 The neurotransmitter NPY has received much attention in recent years, highlighted in our recent review,Citation18 but is still an active area of research, which is reviewed herein. The POMC-derived peptide α-MSH has also been identified as a regulator of fat mass, and while developments in relation to adipose are relatively new, they are also reviewed herein.
Adipose physiology: NPY
While well regarded as an orexigenic peptide in the brain, NPY functions in many other aspects of physiology, such as learning and memory,Citation102 tumor growth and progression,Citation103 and other processes related to adipose tissue. The effects of NPY that are related to adipose tissue and energy balance are summarized in and .
Figure 1. Adipogenic effects of NPY during adipocyte differentiation. When neuropeptide Y (NPY) is included in the differentiation cocktail of preadipocytes and cells from the stromal vascular fraction (mesenchymal stem cells (MSC) and preadipocytes (PreAd)) there is increased cellular proliferation of the precursor cells, a process thought to be mediated primarily via NPY receptor 2 (NPY2R). Throughout differentiation, there is increased expression of peroxisome proliferator activated receptor (PPARγ) and CCAAT/enhancer-binding protein α (C/EBPα), and decreased expression of uncoupling protein 1 (UCP1), thereby leading to increased lipid accumulation in the terminally differentiated adipocyte. Yellow represents the cell nucleus and red represents the lipid droplet.
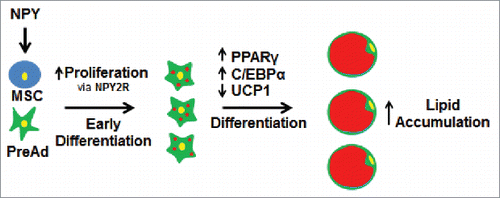
Table 2. Physiological actions of Neuropeptide Y (NPY) related to energy balance and the receptors (NPYR) involved.
NPY co-exists with norepinephrine (NE) in sympathetic nerve terminals.Citation104 The specific action of co-release of NPY and NE is often studied in the cardiovascular system,Citation105,106 but is not well annotated in the adipose tissue. Obese (fa/fa) Zucker rats reduce food intake and display enhanced metabolic rates when infused with both NPY and NE, suggestive of a synergistic effect.Citation107 In 3T3-L1 cells, NPY is able to potentiate lipolysis that is stimulated by isoproterenol, a β-adrenergic agonist,Citation108 further showing synergy between NPY and NE. In another study, mice overexpressing NPY in noradrenergic neurons show increased body, WAT, and BAT weight.Citation109 The effect is possibly determined by how much of each is present, distribution and abundance of receptor sub-types, with increases in adipose tissue development observed when NPY is more abundant, but the synergetic lipolytic effect being observed when amounts are similar.
Stem cells to preadipocytes to adipocytes
Prenatal stress can increase the chances of progeny developing obesity,Citation110 and most adipocytes originate from adipose progenitor cells that were committed prenatally or early postnatally.Citation111 To understand the effect of prenatal stress on development of adipose tissue, epinephrine (EPI) was included in the adipocyte differentiation cocktail of murine embryonic stem cells to stimulate stress, after which NPY and NPY1R and NPY2R mRNAs were up-regulated.Citation112 Treatment with EPI was also associated with increased adipogenesis, through increased proliferation, mRNA expression of FABP4 and PPARγ, and enhanced neutral lipid accumulation. This effect was blocked by combined antagonism of NPYR's 1, 2, and 5,Citation112 indicating that the NPY system is important in the generation of more adipose cells from mesenchymal stem cells in response to stress.
Preadipocytes of the 3T3-L1 cell line were more viable and proliferated more when treated with a low dose of NPY (10−15M and 10−13M), but less viable and proliferative when treated with a much higher dose (10−7M).Citation113 It was speculated that high concentrations of NPY may be contributing to differentiation of the preadipocyte rather than proliferation, explaining why the effect on proliferation is not observed at a higher concentration of NPY.Citation113 When included in the differentiation cocktail, NPY up-regulates PPARγ and C/EBPα protein expression, and increases lipid accumulation in cells.Citation113 These data indicate that NPY stimulates preadipocytes to multiply and then undergo hypertrophy during differentiation.
NPY also has effects on brown fat during cellular differentiation and after isolation of cells from the SVF of brown fat. Results from a study that used a mesenchymal stem cell line, C3H10T1/2, that is able to be induced to behave in a brown-like manner, demonstrated that including NPY in the differentiation cocktail at either 0, 1, 10, or 100 nM concentration had no effect on brown fat cell adipogenesis (it did not cause browning of the cells induced to differentiate), but was associated with inhibition of brown fat cell-associated marker uncoupling protein-1 (UCP-1),Citation114 providing more evidence for NPY's role in suppressing brown fat activation, as reviewed earlier.Citation18
Bone-adipose crosstalk
The bone and adipose tissue have considerable cross-talk, and both contribute to energy balance, with NPY and its receptors also being involved in the regulation of these 2 systems to control energy balance. A 40% reduction of plasma NPY was observed in mice that have an osteoblast-specific deletion of p38α mitogen activated protein kinase (p38 MAPK). A 20% increase in energy expenditure, measured by locomotor activity, O2 consumption, CO2 expenditure and energy expenditure monitored through an indirect calorimetric analysis, and up-regulation of UCP-1 without a change in energy intake was also observed in WAT and BAT, as well as a decrease in body weight and total adiposity. The mRNA distribution of NPY was evaluated in bone, primary osteoblasts, whole brain, hypothalamus, sympathetic superior cervical ganglia, adrenal gland, muscle, adipose, and jejunum, and was reduced in bone and primary osteoblasts, and increased in the hypothalamus and adipose of p38 MAPK-osteoblast-null mice. Although NPY was increased in the hypothalamus, the distribution of brain NPY immunoreactivity did not differ between the wild and KO mice. An increase in feeding for the first few hours was observed, but the effect normalized over time. Intraperitoneal injection of NPY partially restored adipose tissue mass in the mice lacking p38 MAPK in their osteoblasts.Citation115
A review by Shi and Baldock, 2012, highlighted the relationship between NPY, bone, and adipose tissue.Citation116 In bone, NPY represses bone formation, mainly through NPYR 1 and 2, and a lack of NPY expression increases osteoblast activity (bone formation), and bone mass. The two systems may be coordinately regulated although effects of NPY on bone and adipose homeostasis are complicated by how the brain perceives the negative energy balance state. Bone formation is similarly affected by elevated NPY in various energy states, whereas the adipose reacts differently. When there is elevated NPY, for instance during a negative energy balance state, bone metabolism is optimized for energy conservation and inhibition of bone formation, while in the adipose tissue, elevated NPY leads to increases in body weight and WAT mass.Citation117,118
Although the interaction between the adipose and bone remains to be further elucidated, they seem to be an attractive target for pharmacological intervention. NPY KO mice show increased bone formation,Citation119 thus while targeting NPY to reduce obesity, an intervention currently underway,Citation120 bone formation could possibly be positively affected.
NPY knockout, knockdown, and NPYR antagonism
There are reports of NPY KO mice, knockdown in specific tissues, as well as pharmacological inhibition of receptor sub-types (); all models to better understand the role of NPY in adipose physiology and to identify the specific receptor(s) through which it mediates various functions in adipose tissue.
Table 3. Effects of NPY receptor (NPYR) antagonism in preadipocytes and adipocytes.
As reviewed earlier,Citation18 NPY and NPYR 1, 2, and 5 are expressed in the adipose tissue and downstream effects related to energy balance are summarized in . The NPY1R has the widest body distribution, with expression being greatest in the paraventricular nucleus (PVN) of the hypothalamus.Citation121 It is regarded as the NPYR most involved in regulating food intake, although NPY2R is also expressed in the arcuate nucleus (ARC) of the hypothalamus and contributes to appetite regulation.Citation122 The NPY5R is most similar to NPY1R in amino acid sequence identity,Citation123 and is co-localized with NPY1R in many areas of the nervous system where NPY5R is expressed.Citation124 Adipose-specific production of NPY5R remains debated, as it has been detected in the adipose of rodentsCitation125,126 and chickens,Citation67 but at other times is undetectable.Citation127,128 Additionally, NPY2R was expressed in human,Citation10 mouseCitation10 and chicken preadipocytes,Citation11 while in another study NPY2R was not detected in mouse preadipocytes.Citation128 While in vivo studies may show the importance of each receptor in whole body adipose biology, in vitro studies allow more specific quantification and study of regulation in a particular cell type.
Sirtuin 1 (SIRT1) has been reported to play a role in fat metabolism and may mediate some NPY-induced changes in adipose physiology. It is a deacetylase involved in lipid mobilization from fatCitation129 that also promotes brown fat cellular remodeling,Citation130 thereby contributing to an increase in energy release.Citation131 A transcription factor, cAMP response element binding protein (CREB), is also important in this role as it induces expression of UCP-1,Citation132 which as described earlier is a factor important for the thermogenic function of BAT.Citation133
In NPY-KO mice, adipogenesis-associated factor mRNAs are down-regulated, while expression of genes involved in lipolysis increase in gonadal white adipose tissue (gWAT).Citation134 Age related decreases in gWAT lipogenesis, and thermogenesis in inguinal WAT are also reduced in NPY KO mice.Citation134 SIRT1 has been reported to promote fat mobilization due to repressed PPARγ activity,Citation129 and the changes due to NPY KO are thought to be associated with activation of SIRT1, and subsequent inactivation of PPARγ- mediated pathways. This is supported by the finding that NPY treatment inhibits lipolysis in 3T3-L1 cells via NPY1R-mediated signaling and reduced CREB and SIRT1 protein expression.Citation134 Deletion of NPY in mice clearly affects pathways involving SIRT1, as well as regulation of transcription factors PPARγ and CREB. Because the NPY system is widely distributed, targeting factors that are affected by NPY, such as SIRT1, may thereby target the fat, especially since this factor is associated with the loss of fat, a major goal of modulating the NPY system to treat obesity.Citation120
Dorsomedial hypothalamic NPY has effects independent of ARC NPY, and is a major source of NPY that has been well studied in relation to energy balance.Citation135 Additionally, this nucleus was shown to be connected to the innervation of the adipose through the afore-mentioned PRV studies.Citation92,93 Knockdown of NPY expression in the dorsomedial hypothalamus (DMH) via adeno-associated virus-mediated RNA interference reduced fat depot weights in rats and ameliorated high-fat diet-induced hyperphagia and obesity. Knockdown also promoted brown adipose tissue (BAT) development and increased UCP-1 expression.Citation136
Daily IP injection of the NPY antagonist S.A.0204 for 30 days caused a decrease in body weight, and an increase in WAT lipolysis and apoptosis in diet-induced obese rats.Citation137 Intraperitoneal injection of the NPY2R antagonist BIIE 0246 into male mice for 14 days decreased adipocyte cell number but did not change cell size, indicating that NPY2R is important in producing new cells, but may not necessarily be involved in hypertrophy of adipocytes.Citation138 Although this study did not measure gene expression, future studies could evaluate whether the genes involved in adipogenesis/lipolysis are affected by NPYR antagonism. Serum deprivation of 3T3-L1 cells can induce lipolysis, an effect that is inhibited by NPY treatment, with NPY's effects in turn blocked by the NPY1R antagonist BIBO3304, demonstrating that the ability of NPY to rescue cells from the lipolytic effect of serum starvation is specific to NPY-mediated signaling.Citation134
Insulin resistance and sensitivity
Obesity is associated with insulin resistance, a pathology where cells are unable to normally respond to insulin,Citation139 thus it is worth noting the relationship of NPY function to insulin resistance in the adipose tissue. In biopsies from human subjects, mRNA abundance of adipose-derived hormones involved in insulin sensitivity, including adiponectin, visfatin, and omentin, was correlated with mRNA expression of NPY and its receptors. In subcutaneous fat all 3 were positively correlated with expression of NPY, and adiponectin, visfatin, and omentin were positively correlated with expression of NPYR 1 and 5, NPYR 1, 2, and 5, and NPY5R, respectively. All three were also positively correlated with NPY expression in the visceral fat, and visfatin was positively correlated with NPYR 1, 2, and 5 in visceral fat. There was no correlation of expression between depots, implying that the depots function differently.Citation140
Long-term over-expression of NPY in the PVN leads to increased adipose weight and insulin resistance in mice, as determined by a euglycemic-hyperinsulinemic clamp test and intravenous glucose tolerance test. Glucose uptake was decreased in adipose tissue but was not affected in skeletal muscle, suggesting that effects of NPY overexpression on insulin resistance were tissue specific.Citation141 In the same study, 3T3-L1 cells were differentiated and treated with NPY, which decreased basal glucose consumption and decreased glucose uptake. This effect was not blocked by antagonism of NPY1R, but was blunted by antagonism of NPY5R.Citation141 It is difficult to directly attribute the increased PVN NPY to the insulin resistance in the adipose tissue, but that the in vitro effect of NPY can be blocked by antagonism of NPY5R suggests that this may be the case; nonetheless, more studies are needed to confirm this hypothesis. These studies provide evidence that NPY5R may be the key NPYR through which NPY signaling leads to insulin resistance, as overexpression of NPY is associated with insulin resistance that can be blunted when NPYR5 signaling is blocked. That adiponectin,Citation142,143 visfatin,Citation144 and omentinCitation145 are involved in insulin sensitivity and are all correlated with NPY and NPY5R expression in subcutaneous adipose tissueCitation140 also implies that NPY and NPY5R play a role in insulin sensitivity.
Although NPY is already a target for drug development to combat obesity,Citation120 these correlations must be considered when targeting the NPY system pharmacologically. Antagonizing the NPY system could decrease fat accretion while increasing bone formation, a potentially positive side effect of treating obesity; but because of their correlated expression, decreased expression of adiponectin, visfatin, or omentin could exacerbate insulin resistance. Additionally, in addition to obesity and bone physiology, NPY also effects many other systems and diseases, including anxiety, depression, epilepsy, alcoholism, pain, cancer, cardiovascular regulation, gastrointestinal tract, and circadian regulation, as reviewed.Citation1 Drug development has proved difficult when targeting NPY, as targeting central NPY is not very specific and concerns the blood brain barrier, thus targeting the periphery warrants further exploration.Citation146
NPY and adipose development in chickens
Adipose tissue develops as a consequence of both hyperplasia and hypertrophy, with hyperplasia leading to hypertrophy. We reported changes in the adipose tissue of broiler chicks during the first 2 weeks post-hatch.Citation66 Specifically, for the subcutaneous, abdominal, and clavicular fat depots at days 4 and 14 of age, there were depot-specific differences with larger adipocytes and the greatest glycerol–3–phosphate dehydrogenase (G3PDH) activity in the subcutaneous fat at day 4. The abdominal fat grew more rapidly than the other 2 depots from day 4 to 14, and clavicular fat was intermediate for most traits that were measured. These changes were most likely due to a combination of hypertrophy and hyperplasia.Citation66 Neuropeptide Y and its receptors were also measured in the study; from day 4 to 14 there was an increase in mRNA abundance of NPY and NPY receptor 5 (NPY5R) and a decrease in NPY2R in the clavicular adipose tissue. The orexigenic properties of NPY are mostly associated with NPY1R,Citation122 mRNA of which was not affected by age in clavicular adipose, but did increase in the hypothalamus of broiler chicks from day 4 to 14.Citation66 NPY receptors 1, 2, and 5 were all increased in the liver at 14 days post-hatch, implying that the NPY system may function in the liver during development.Citation66 Thus, NPY and several of its receptors are transcribed in chick adipose tissue and liver and appear to be differentially regulated during early post-hatch development, although functional implications are still unclear.
We also conducted an in vitro study showing that 4 hours of NPY treatment increased mRNA abundance of NPY and NPY2R in abdominal fat preadipocytes isolated from 14 day-old broilers.Citation11 With NPY being included in the differentiation cocktail, abundance of NPY was also increased on days 2 and 6 post induction of differentiation, whereas NPYR2 expression was not affected during this time.Citation11 That NPY2R mRNA expression was upregulated in preadipocytes in response to NPY may imply that NPY2R is more important to NPY function in the preadipocyte than in the differentiating adipocyte. On day 5 and 6 post-induction of differentiation, there was increased cell proliferation and G3PDH activity, respectively. This indicates that NPY treatment was associated with increased hyperplasia, as well as fat synthesis, as G3PDH activity is an indirect marker of triacylglycerol synthesis.Citation147,148 Thus in chicken adipose cells, NPY may contribute to both hyperplasia and hypertrophy. While NPYR 1 and 5 seem to play a role based on data from our in vivo studies, expression of NPYR 1 and 5 could not be detected in adipose cells in our in vitro studies.Citation11 This may be due to the difference in cell types included in each study. The in vivo studies included the whole adipose tissue, whereas in the in vitro studies isolated the stromal vascular fraction of cells from adipose and then enriched for mesenchymal stem cells and preadipocytes.
Interestingly, we reported heterosis for NPYR 1 and 5 mRNA in the adipose tissue of chickens from the Virginia body weight lines, suggesting that the NPY system is important for energy balance in chickens.Citation67 HWS chickens expressed more NPY and NPYR 1 and 5 mRNA in the abdominal fat,Citation67 while LWS chicks expressed more NPY and NPYR 2 and 5 in the hypothalamus.Citation149 Additionally, expression of NPY5R in the hypothalamus is regulated differently between the fed vs fasted state, where in the fed state, NPY5R was more highly expressed in the LWS, and the magnitude of difference between the LWS and HWS was lessened by fasting.Citation150
Adipose phyiology: α-MSH
The peptide α-MSH is a part of the melanocortin system, which is made up of α, β, and γ – MSH, ACTH, melanocortin receptors (MCR) 1–5, and 2 endogenous MCR antagonists, agouti and agouti-related peptide (AgRP), as reviewed.Citation151,152 All of the melanocortin peptides are derived from POMC,Citation12 and have some function in regulating food intake. Our group recently showed that ACTH, and the C-terminal fragment of ACTH, β-cell-tropin (βCT) have effects on food intake in chicks, with ACTH being anorexigenic,Citation153 and βCT being orexigenic;Citation154 the same effects on food intake were reported in rats.Citation14 All of the MSH peptides decrease food intake in mice, with α-MSH being the most potent,Citation155 but in chicks β and γ-MSH had no effect on food intake.Citation16
MC1R is mainly associated with pigmentation,Citation156 but is also involved in the control of inflammation,Citation157,158 and has the highest affinity for α-MSH.Citation159 MC2R is the ACTH receptor, and is mainly associated with the action of ACTH on the periphery,Citation160 namely the release of glucocorticoids.Citation161 It is also expressed in the adipocyte, and may function in regulating lipolysis.Citation162 MCR 3 and 4 are highly expressed in the brain,Citation163,164 and are involved in food intake and energy balance,Citation165 and MC3R-deficient mice have increased fat mass.Citation166 MC4R is the receptor for both ACTH and α-MSH in the brain of rats,Citation14 and α-MSH in the brain of chicks.Citation16 MC5R is expressed in many sebaceous and exocrine glands,Citation167 regulates fatty acid oxidation in skeletal muscle,Citation168 and is associated with lipolytic effects in the adipose.Citation17,162
In addition to its anorexigenic function, α-MSH is associated with decreasing fat mass and body weight ().Citation13,14 α-MSH promoted lipolysis in vitroCitation17 and in mice reduced their fat mass.Citation169,170 Signaling via MC5R increased lipolysis and impaired fatty acid re-esterification in 3T3-L1 adipocytes,Citation17 providing a plausible route of action of α-MSH in adipose tissue. Because POMC is a precursor to multiple peptides, KO of POMC does not correlate well with determining the function of a specific POMC cleavage product, which has led to other techniques to overexpress the specific peptides in order to ascertain their function. In two month-old male C57BL/6N mice, overexpression of α-MSH by a lentiviral vector (LVi-α-MSH-EGFP) in the nucleus tractus solitarius decreased fat mass, food intake, and body weight.Citation169 The phosphatidylinositol 3-kinase (PI3K) pathway is important in the regulation of food intake and energy homeostasis in the hypothalamus,Citation171,172 and inhibition of 2 PI3K's, PI3Kβ and PI3Kγ, led to increased NE concentrations in the adipose tissue, which then led to decreased fat mass and was associated with increased lipolysis and browning of white adipose tissue in adult mice.Citation170 It was suggested that the increase in NE was due to increased α-MSH-dependent sympathetic drive as cAMP production was also increased in the intermediolateral nucleus, an area where energy expenditure is regulated by α-MSH.Citation173 Moreover, intracerebroventricular injection of α-MSH into wild-type mice caused increased phosphorylation of hormone sensitive lipase (HSL), a lipase known to be activated by NE,Citation174,175 to the same extent as in PI3Kβ- and PI3Kγ-inhibited mice.Citation170 In humans, α-MSH stimulated lipolysis in intact white adipose tissue but not in isolated preadipocytes that were differentiated into adipocytes, suggesting that the effect may be dependent on neuronal innervation.Citation176 Clearly, α-MSH has effects on the adipose tissue, some of which may relate to its role in the sympathetic nervous system.
Figure 2. Actions of α-MSH on the adipocyte. When adipocytes are treated with α-melanocyte stimulating hormone (α-MSH), there is increased expression of nuclear hormone receptor subfamily 4 group A member 1 (NR4A1), and increased lipolysis and inhibition of re-esterification. In animals, this leads to a decrease in fat mass and body weight. These effects are thought to be mediated by melanocortin receptor 5 (MC5R). Yellow represents the cell nucleus and red represents the lipid droplet.
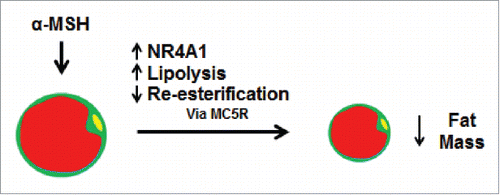
The nuclear hormone receptor (NR) 4A subgroup is involved in adipose metabolism, with effects on differentiation and proliferation.Citation177,178 Treatment of differentiated 3T3-L1 cells with an α-MSH agonist (NDP-MSH) for 2 hours was associated with increased mRNA expression of members of the NR4A subgroup, but not other subgroups in the NR superfamily.Citation179 Furthermore, siRNA-mediated knockdown of nuclear hormone receptor subfamily 4 group A member 1 (NR4A1) attenuated the effects of NDP-MSH treatment on gene expression in 3T3-L1 adipocytes. Intraperitoneal (IP) injection of NDP-MSH into 7-week-old C57 BL/6J male mice was associated with increased expression of NR4A1, suggesting that expression of NR4A1 is necessary for the action of α-MSH.
These studies collectively indicate that α-MSH has effects on the adipose tissue that are lipolytic, and signaling initiated from the brain and sympathetic nervous system can act to decrease fat mass. Involvement of the NR superfamily has also been implicated in the action of α-MSH, and MC5R may be an important receptor for mediating effects of the melanocortin system in the adipose tissue. More in vivo studies are needed to confirm that MC5R is important for adipose function, and whether or not there are different effects through the various receptors. To date, effects of the melanocortin system in adipose tissue has not been reported in non-mammalian species.
Communication between α-MSH and NPY
Both NPY and α-MSH have strong, opposing effects on food intake, with effects conserved across a range of vertebrate species. In chicks, combined intracerebroventricular (ICV) injection of both α-MSH and NPY results in a decrease in food intake,Citation180 the orexigenic effect of NPY likely overpowered by the anorexigenic effect of α-MSH. Because α-MSH is a peptide derived from POMC, post-translational processing becomes very important in regulating the quantity of the active peptide, and serves as a point of entry for intervention, either through pharmaceutical means, feedback loops, or action of other neurotransmitters. NPY has been implicated in inhibiting post-translational processing of POMC to yield α-MSH by decreasing prohormone convertase 2 (PC2) protein; this effect is associated with downregulation of early growth response protein 1 (EGR-1) and is likely occurring via NPY1R, as blocking NPY1R is able to restore PC2 activity and α-MSH release.Citation2 After ICV injection, NPY decreased the phosphorylation of CREB, an effect normally caused by α-MSH, and NPY also decreased the amount of α-MSH in the PVN.Citation2 When co-injected, α-MSH overpowers the orexigenic effect of NPY,Citation180 but when promoting positive energy balance NPY signals to decrease the amount of α-MSH in the hypothalamus.Citation2 This may imply that the effect of combined injection is more of a pharmacological response than physiological.
Potential physiological routes through which NPY and α-MSH affect adipose tissue
Although most studies concerning the interaction of NPY and α-MSH are in relation to the brain and control of food intake, there may also be interactions between the 2 in adipose tissue. Melanotan-II is a non-selective MCR agonist, and a 7 day-long central infusion into rats reduced feeding and adiposity, and when infused simultaneously with NPY reduced feeding and attenuated NPY-mediated increases in fat pad weight. Although combined injection is thus able to inhibit some of the effects of NPY, it does not block NPY-mediated inhibition of the gonadotropic and somatotropic axes,Citation181 suggesting that crosstalk between MSH and NPY is tissue and/or axis specific rather than global.
Circulating plasma levels of NPY are increased in obese and overweight humans, but circulating α-MSH levels are similar between normal, overweight and obese individuals.Citation182 This indicates that increased weight from adipose tissue has impacts on circulating NPY, but may not impact plasma α-MSH. Moreover, in obese subjects after weight loss, cerebrospinal fluid (CSF) concentrations of NPY decrease whereas α-MSH levels remained unchanged.Citation183
The interaction of NPY and α-MSH is complex, and much is still unknown as there is little reported on the role of α-MSH and its interaction with NPY and other factors in the adipose tissue. The fat-loss effect of α-MSH may not be a direct effect on the adipose, but rather an indirect effect of decreasing the amount of energy available from reduced energy intake, thus forcing the animal to tap into its energy reserves in the adipose tissue to maintain energy balance. Likewise, the fat-synthesizing effect of NPY in the adipose tissue is likely a response to increased energy intake (and thus availability) mediated via the hypothalamus.
Conclusions
Factors involved in regulating energy balance and adipose physiology are crucial to understand in light of the current obesity epidemic and increasing importance of enhancing nutrient utilization efficiency and meat production in agricultural species to meet the demand for dietary protein as the global population continues to increase. The adipose tissue is a diverse endocrine organ, with many cell types and models used to study its function. Because of its complicated physiology, there are multiple cellular pathways to explore for pharmacological targeting, but also many functions that may not directly relate to whole-body energy balance. NPY exerts multiple functions in the adipose tissue mediated by distinct receptor sub-types, but also regulates metabolism in other tissues such as bone. Knockout and antagonism of NPY are associated with reduced adiposity and body weight, but because of its diverse physiological functions, affecting solely the lipid metabolism in adipose tissue is a challenge in targeting this system to treat obesity. Regulation of α-MSH could also be a potential route of intervention, as it is associated with not only a decrease in feeding, but also decreased body weight and increased lipolysis. The interaction of these 2 peptides is also important and multi-faceted; the two have opposing effects on appetite and adipocyte metabolism and at times one may inhibit the expression and activity of the other. Further study of NPY and α-MSH functions in the brain-fat axis, as well as identification of other appetite-regulatory factors that affect adipose tissue physiology will provide useful information in the development of pharmacological strategies to battle obesity and also approaches to modulate energy utilization in animals raised for food consumption.
Disclosure of potential conflicts of interest
No potential conflicts of interest were disclosed.
References
- Brothers SP, Wahlestedt C. Therapeutic potential of neuropeptide Y (NPY) receptor ligands. EMBO Mol Med 2010; 2:429-39; PMID:20972986; http://dx.doi.org/10.1002/emmm.201000100
- Cyr NE, Toorie AM, Steger JS, Sochat MM, Hyner S, Perello M, Stuart R, Nillni EA. Mechanisms by which the orexigen NPY regulates anorexigenic α-MSH and TRH. Am J Physiol Endocrinol Metab 2013; 304:E640-50; PMID:23321476; http://dx.doi.org/10.1152/ajpendo.00448.2012
- Tatemoto K, Carlquist M, Mutt V. Neuropeptide Y-a novel brain peptide with structural similarities to peptide YY and pancreatic polypeptide. Nature 1982; 296:659-60; PMID:6896083; http://dx.doi.org/10.1038/296659a0
- Clark JT, Kalra PS, Crowley WR, Kalra SP. Neuropeptide Y and human pancreatic polypeptide stimulate feeding behavior in rats. Endocrinol 1984; 115:427-9; http://dx.doi.org/10.1210/endo-115-1-427
- Pau MY, Pau KY, Spies HG. Characterization of central actions of neuropeptide Y on food and water intake in rabbits. Physiol Behavior 1988; 44:797-802; http://dx.doi.org/10.1016/0031-9384(88)90065-0
- Matsuda K, Sakashita A, Yokobori E, Azuma M. Neuroendocrine control of feeding behavior and psychomotor activity by neuropeptideY in fish. Neuropeptides 2012; 46:275-83; PMID:23122775; http://dx.doi.org/10.1016/j.npep.2012.09.006
- Richardson RD, Boswell T, Raffety BD, Seeley RJ, Wingfield JC, Woods SC. NPY increases food intake in white-crowned sparrows: effect in short and long photoperiods. Am J Physiol 1995; 268:R1418-22; PMID:7611518
- Kuenzel WJ, Douglass LW, Davison BA. Robust feeding following central administration of neuropeptide Y or peptide YY in chicks, Gallus domesticus. Peptides 1987; 8:823-8; PMID:3432131; http://dx.doi.org/10.1016/0196-9781(87)90066-0
- Newmyer BA, Nandar W, Webster RI, Gilbert E, Siegel PB, Cline MA. Neuropeptide Y is associated with changes in appetite-associated hypothalamic nuclei but not food intake in a hypophagic avian model. Behavioural Brain Res 2013; 236:327-31; http://dx.doi.org/10.1016/j.bbr.2012.08.015
- Kuo LE, Kitlinska JB, Tilan JU, Li L, Baker SB, Johnson MD, Lee EW, Burnett MS, Fricke ST, Kvetnansky R. Neuropeptide Y acts directly in the periphery on fat tissue and mediates stress-induced obesity and metabolic syndrome. Nat Med 2007; 13:803-11; PMID:17603492; http://dx.doi.org/10.1038/nm1611
- Zhang W, Bai S, Liu D, Cline MA, Gilbert ER. Neuropeptide Y promotes adipogenesis in chicken adipose cells in vitro. Comparative Biochem Physiol Part A, Mol Integrative Physiol 2015; 181:62-70; http://dx.doi.org/10.1016/j.cbpa.2014.11.012
- Wardlaw SL. Hypothalamic proopiomelanocortin processing and the regulation of energy balance. Eur J Pharmacol 2011; 660:213-9; PMID:21208604; http://dx.doi.org/10.1016/j.ejphar.2010.10.107
- Zimanyi IA, Pelleymounter MA. The role of melanocortin peptides and receptors in regulation of energy balance. Curr Pharmaceutical Design 2003; 9:627-41; http://dx.doi.org/10.2174/1381612033391234
- Al-Barazanji KA, Miller JE, Rice SQ, Arch JR, Chambers JK. C-terminal fragments of ACTH stimulate feeding in fasted rats. Hormone Metab Res = Hormon- Und Stoffwechselforschung = Hormones Et Metabolisme 2001; 33:480-5; PMID:11544562; http://dx.doi.org/10.1055/s-2001-16941
- Cerda-Reverter JM, Schioth HB, Peter RE. The central melanocortin system regulates food intake in goldfish. Regulatory Peptides 2003; 115:101-13; PMID:12972325; http://dx.doi.org/10.1016/S0167-0115(03)00144-7
- Saneyasu T, Honda K, Kamisoyama H, Nakayama Y, Ikegami K, Hasegawa S. Alpha-melanocyte stimulating hormone plays an important role in the regulation of food intake by the central melanocortin system in chicks. Peptides 2011; 32:996-1000; PMID:21402117; http://dx.doi.org/10.1016/j.peptides.2011.03.006
- Rodrigues AR, Almeida H, Gouveia AM. Alpha-MSH signalling via melanocortin 5 receptor promotes lipolysis and impairs re-esterification in adipocytes. Biochimica Et Biophysica Acta 2013; 1831:1267-75; PMID:24046867; http://dx.doi.org/10.1016/j.bbalip.2013.04.008
- Zhang W, Cline MA, Gilbert ER. Hypothalamus-adipose tissue crosstalk: neuropeptide Y and the regulation of energy metabolism. Nutrition Metab 2014; 11:27; http://dx.doi.org/10.1186/1743-7075-11-27
- Zuk PA, Zhu M, Ashjian P, De Ugarte DA, Huang JI, Mizuno H, Alfonso ZC, Fraser JK, Benhaim P, Hedrick MH. Human adipose tissue is a source of multipotent stem cells. Mol Biol Cell 2002; 13:4279-95; PMID:12475952; http://dx.doi.org/10.1091/mbc.E02-02-0105
- Billon N, Iannarelli P, Monteiro MC, Glavieux-Pardanaud C, Richardson WD, Kessaris N, Dani C, Dupin E. The generation of adipocytes by the neural crest. Development 2007; 134:2283-92; PMID:17507398; http://dx.doi.org/10.1242/dev.002642
- Le Lievre CS, Le Douarin NM. Mesenchymal derivatives of the neural crest: analysis of chimaeric quail and chick embryos. J Embryol Exp Morphol 1975; 34:125-54; PMID:1185098
- Hauner H, Schmid P, Pfeiffer EF. Glucocorticoids and insulin promote the differentiation of human adipocyte precursor cells into fat cells. J Clin Endocrinol Metab 1987; 64:832-5; PMID:3546356; http://dx.doi.org/10.1210/jcem-64-4-832
- Grigoriadis AE, Heersche JN, Aubin JE. Differentiation of muscle, fat, cartilage, and bone from progenitor cells present in a bone-derived clonal cell population: effect of dexamethasone. J Cell Biol 1988; 106:2139-51; PMID:3384856; http://dx.doi.org/10.1083/jcb.106.6.2139
- Wakitani S, Saito T, Caplan AI. Myogenic cells derived from rat bone marrow mesenchymal stem cells exposed to 5-azacytidine. Muscle Nerve 1995; 18:1417-26; PMID:7477065; http://dx.doi.org/10.1002/mus.880181212
- Ferrari G, Cusella-De Angelis G, Coletta M, Paolucci E, Stornaiuolo A, Cossu G, Mavilio F. Muscle regeneration by bone marrow-derived myogenic progenitors. Science 1998; 279:1528-30; PMID:9488650; http://dx.doi.org/10.1126/science.279.5356.1528
- Johnstone B, Hering TM, Caplan AI, Goldberg VM, Yoo JU. In vitro chondrogenesis of bone marrow-derived mesenchymal progenitor cells. Exp Cell Res 1998; 238:265-72; PMID:9457080; http://dx.doi.org/10.1006/excr.1997.3858
- Pittenger MF, Mackay AM, Beck SC, Jaiswal RK, Douglas R, Mosca JD, Moorman MA, Simonetti DW, Craig S, Marshak DR. Multilineage potential of adult human mesenchymal stem cells. Science 1999; 284:143-7; PMID:10102814; http://dx.doi.org/10.1126/science.284.5411.143
- Wang WG, Lou SQ, Ju XD, Xia K, Xia JH. In vitro chondrogenesis of human bone marrow-derived mesenchymal progenitor cells in monolayer culture: activation by transfection with TGF-beta2. Tissue Cell 2003; 35:69-77; PMID:12589731; http://dx.doi.org/10.1016/S0040-8166(02)00106-4
- Gao S, Zhao P, Lin C, Sun Y, Wang Y, Zhou Z, Yang D, Wang X, Xu H, Zhou F, et al. Differentiation of human adipose-derived stem cells into neuron-like cells which are compatible with photocurable three-dimensional scaffolds. Tissue Eng Part A 2014; 20:1271-84; PMID:24251600; http://dx.doi.org/10.1089/ten.tea.2012.0773
- Zhu T, Yu D, Feng J, Wu X, Xiang L, Gao H, Zhang X, Wei M. GDNF and NT-3 induce progenitor bone mesenchymal stem cell differentiation into neurons in fetal gut culture medium. Cell Mol Neurobiol 2015; 35:255-64; PMID:25301495; http://dx.doi.org/10.1007/s10571-014-0120-3
- Huang T, He D, Kleiner G, Kuluz J. Neuron-like differentiation of adipose-derived stem cells from infant piglets in vitro. J Spinal Cord Med 2007; 30 Suppl 1:S35-40; PMID:17874685
- Rosen ED, MacDougald OA. Adipocyte differentiation from the inside out. Nat Rev Mol Cell Biol 2006; 7:885-96; PMID:17139329; http://dx.doi.org/10.1038/nrm2066
- Rosen ED, Walkey CJ, Puigserver P, Spiegelman BM. Transcriptional regulation of adipogenesis. Genes Dev 2000; 14:1293-307; PMID:10837022
- Barak Y, Nelson MC, Ong ES, Jones YZ, Ruiz-Lozano P, Chien KR, Koder A, Evans RM. PPAR gamma is required for placental, cardiac, and adipose tissue development. Mol Cell 1999; 4:585-95; PMID:10549290; http://dx.doi.org/10.1016/S1097-2765(00)80209-9
- Tontonoz P, Hu E, Graves RA, Budavari AI, Spiegelman BM. mPPAR gamma 2: tissue-specific regulator of an adipocyte enhancer. Genes Dev 1994; 8:1224-34; PMID:7926726; http://dx.doi.org/10.1101/gad.8.10.1224
- Tontonoz P, Hu E, Spiegelman BM. Stimulation of adipogenesis in fibroblasts by PPAR gamma 2, a lipid-activated transcription factor. Cell 1994; 79:1147-56; PMID:8001151; http://dx.doi.org/10.1016/0092-8674(94)90006-X
- Hu E, Tontonoz P, Spiegelman BM. Transdifferentiation of myoblasts by the adipogenic transcription factors PPAR gamma and C/EBP α. Proc Natl Acad Sci U S A 1995; 92:9856-60; PMID:7568232; http://dx.doi.org/10.1073/pnas.92.21.9856
- Tamori Y, Masugi J, Nishino N, Kasuga M. Role of peroxisome proliferator-activated receptor-gamma in maintenance of the characteristics of mature 3T3-L1 adipocytes. Diabetes 2002; 51:2045-55; PMID:12086932; http://dx.doi.org/10.2337/diabetes.51.7.2045
- Imai T, Takakuwa R, Marchand S, Dentz E, Bornert JM, Messaddeq N, Wendling O, Mark M, Desvergne B, Wahli W, et al. Peroxisome proliferator-activated receptor gamma is required in mature white and brown adipocytes for their survival in the mouse. Proc Natl Acad Sci U S A 2004; 101:4543-7; PMID:15070754; http://dx.doi.org/10.1073/pnas.0400356101
- Kawaguchi N, Toriyama K, Nicodemou-Lena E, Inou K, Torii S, Kitagawa Y. De novo adipogenesis in mice at the site of injection of basement membrane and basic fibroblast growth factor. Proc Natl Acad Sci U S A 1998; 95:1062-6; PMID:9448285; http://dx.doi.org/10.1073/pnas.95.3.1062
- Eberle D, Hegarty B, Bossard P, Ferre P, Foufelle F. SREBP transcription factors: master regulators of lipid homeostasis. Biochimie 2004; 86:839-48; PMID:15589694; http://dx.doi.org/10.1016/j.biochi.2004.09.018
- Horton JD. Sterol regulatory element-binding proteins: transcriptional activators of lipid synthesis. Biochem Society Transactions 2002; 30:1091-5; http://dx.doi.org/10.1042/bst0301091
- Georgiadi A, Kersten S. Mechanisms of gene regulation by fatty acids. Adv Nutr 2012; 3:127-34; PMID:22516720; http://dx.doi.org/10.3945/an.111.001602
- Kim JB, Wright HM, Wright M, Spiegelman BM. ADD1/SREBP1 activates PPARgamma through the production of endogenous ligand. Proc Natl Acad Sci U S A 1998; 95:4333-7; PMID:9539737; http://dx.doi.org/10.1073/pnas.95.8.4333
- Forman BM, Tontonoz P, Chen J, Brun RP, Spiegelman BM, Evans RM. 15-Deoxy-delta 12, 14-prostaglandin J2 is a ligand for the adipocyte determination factor PPAR gamma. Cell 1995; 83:803-12; PMID:8521497; http://dx.doi.org/10.1016/0092-8674(95)90193-0
- Kliewer SA, Lenhard JM, Willson TM, Patel I, Morris DC, Lehmann JM. A prostaglandin J2 metabolite binds peroxisome proliferator-activated receptor gamma and promotes adipocyte differentiation. Cell 1995; 83:813-9; PMID:8521498; http://dx.doi.org/10.1016/0092-8674(95)90194-9
- Krey G, Braissant O, L'Horset F, Kalkhoven E, Perroud M, Parker MG, Wahli W. Fatty acids, eicosanoids, and hypolipidemic agents identified as ligands of peroxisome proliferator-activated receptors by coactivator-dependent receptor ligand assay. Mol Endocrinol 1997; 11:779-91; PMID:9171241; http://dx.doi.org/10.1210/mend.11.6.0007
- Kim JB, Sarraf P, Wright M, Yao KM, Mueller E, Solanes G, Lowell BB, Spiegelman BM. Nutritional and insulin regulation of fatty acid synthetase and leptin gene expression through ADD1/SREBP1. J Clin Invest 1998; 101:1-9; PMID:9421459; http://dx.doi.org/10.1172/JCI1411
- Cao Z, Umek RM, McKnight SL. Regulated expression of three C/EBP isoforms during adipose conversion of 3T3-L1 cells. Genes Dev 1991; 5:1538-52; PMID:1840554; http://dx.doi.org/10.1101/gad.5.9.1538
- Yeh WC, Cao Z, Classon M, McKnight SL. Cascade regulation of terminal adipocyte differentiation by three members of the C/EBP family of leucine zipper proteins. Genes Dev 1995; 9:168-81; PMID:7531665; http://dx.doi.org/10.1101/gad.9.2.168
- Linhart HG, Ishimura-Oka K, DeMayo F, Kibe T, Repka D, Poindexter B, Bick RJ, Darlington GJ. C/EBPalpha is required for differentiation of white, but not brown, adipose tissue. Proc Natl Acad Sci U S A 2001; 98:12532-7; PMID:11606718; http://dx.doi.org/10.1073/pnas.211416898
- Chen SS, Chen JF, Johnson PF, Muppala V, Lee YH. C/EBPbeta, when expressed from the C/ebpalpha gene locus, can functionally replace C/EBPalpha in liver but not in adipose tissue. Mol Cell Biol 2000; 20:7292-9; PMID:10982846; http://dx.doi.org/10.1128/MCB.20.19.7292-7299.2000
- Wu Z, Rosen ED, Brun R, Hauser S, Adelmant G, Troy AE, McKeon C, Darlington GJ, Spiegelman BM. Cross-regulation of C/EBP α and PPAR gamma controls the transcriptional pathway of adipogenesis and insulin sensitivity. Mol Cell 1999; 3:151-8; PMID:10078198; http://dx.doi.org/10.1016/S1097-2765(00)80306-8
- Zuo Y, Qiang L, Farmer SR. Activation of CCAAT/enhancer-binding protein (C/EBP) α expression by C/EBP β during adipogenesis requires a peroxisome proliferator-activated receptor-gamma-associated repression of HDAC1 at the C/ebp α gene promoter. J Biol Chem 2006; 281:7960-7; PMID:16431920; http://dx.doi.org/10.1074/jbc.M510682200
- Henry SL, Bensley JG, Wood-Bradley RJ, Cullen-McEwen LA, Bertram JF, Armitage JA. White adipocytes: more than just fat depots. Int J Biochem Cell Biol 2012; 44:435-40; PMID:22222895; http://dx.doi.org/10.1016/j.biocel.2011.12.011
- Saely CH, Geiger K, Drexel H. Brown versus white adipose tissue: a mini-review. Gerontology 2012; 58:15-23; PMID:21135534; http://dx.doi.org/10.1159/000321319
- Cannon B, Nedergaard J. Brown adipose tissue: function and physiological significance. Physiol Rev 2004; 84:277-359; PMID:14715917; http://dx.doi.org/10.1152/physrev.00015.2003
- Saarela S, Keith JS, Hohtola E, Trayhurn P. Is the “mammalian” brown fat-specific mitochondrial uncoupling protein present in adipose tissues of birds? Comp Biochem Physiol B 1991; 100:45-9; PMID:1756620
- Mezentseva NV, Kumaratilake JS, Newman SA. The brown adipocyte differentiation pathway in birds: an evolutionary road not taken. BMC Biol 2008; 6:17; PMID:18426587; http://dx.doi.org/10.1186/1741-7007-6-17
- Johnston DW. The absence of brown adipose tissue in birds. Comp Biochem Physiol A Comp Physiol 1971; 40:1107-8; PMID:4400095; http://dx.doi.org/10.1016/0300-9629(71)90298-2
- Mozo J, Emre Y, Bouillaud F, Ricquier D, Criscuolo F. Thermoregulation: what role for UCPs in mammals and birds? Biosci Rep 2005; 25:227-49; PMID:16283555; http://dx.doi.org/10.1007/s10540-005-2887-4
- Bjorndal B, Burri L, Staalesen V, Skorve J, Berge RK. Different adipose depots: their role in the development of metabolic syndrome and mitochondrial response to hypolipidemic agents. J Obes 2011; 2011:490650; PMID:21403826; http://dx.doi.org/10.1155/2011/490650
- Dodson MV, Du M, Wang S, Bergen WG, Fernyhough-Culver M, Basu U, Poulos SP, Hausman GJ. Adipose depots differ in cellularity, adipokines produced, gene expression, and cell systems. Adipocyte 2014; 3:236-41; PMID:26317047; http://dx.doi.org/10.4161/adip.28321
- Speake BK, Farkas K, Ratchford IA, Noble RC. Adipose tissue development in the chick embryo. Biochem Society Transactions 1996; 24:161; http://dx.doi.org/10.1042/bst024161s
- Chen P, Suh Y, Choi YM, Shin S, Lee K. Developmental regulation of adipose tissue growth through hyperplasia and hypertrophy in the embryonic Leghorn and broiler. Poultry Sci 2014; 93:1809-17; http://dx.doi.org/10.3382/ps.2013-03816
- Bai S, Wang G, Zhang W, Zhang S, Rice BB, Cline MA, Gilbert ER. Broiler chicken adipose tissue dynamics during the first two weeks post-hatch. Comparative Biochem Physiol Part A, Mol Integrative Physiol 2015; 189:115-23; http://dx.doi.org/10.1016/j.cbpa.2015.08.002
- Zhang W, Sumners LH, Siegel PB, Cline MA, Gilbert ER. Quantity of glucose transporter and appetite-associated factor mRNA in various tissues after insulin injection in chickens selected for low or high body weight. Physiol Genomics 2013; 45:1084-94; PMID:24046279; http://dx.doi.org/10.1152/physiolgenomics.00102.2013
- Zhang S, McMillan RP, Hulver MW, Siegel PB, Sumners LH, Zhang W, Cline MA, Gilbert ER. Chickens from lines selected for high and low body weight show differences in fatty acid oxidation efficiency and metabolic flexibility in skeletal muscle and white adipose tissue. Int J Obesity 2014; 38:1374-82; http://dx.doi.org/10.1038/ijo.2014.8
- Dunnington EA, Siegel PB. Long-term divergent selection for eight-week body weight in white Plymouth rock chickens. Poultry Sci 1996; 75:1168-79; http://dx.doi.org/10.3382/ps.0751168
- Zelenka DJ, Dunnington EA, Cherry JA, Siegel PB. Anorexia and sexual maturity in female white rock chickens. Increasing the feed intake. Behav Genet 1988; 18:383-7; PMID:3219115; http://dx.doi.org/10.1007/BF01260938
- Dunnington EA, Siegel PB, Cherry JA, Jones DE, Zelenka DJ. Physiological traits in adult female chickens after selection and relaxation of selection for 8-week body-weight. J Anim Breed Genet 1986; 103:51-8; http://dx.doi.org/10.1111/j.1439-0388.1986.tb00066.x
- Dunnington EA, Honaker CF, McGilliard ML, Siegel PB. Phenotypic responses of chickens to long-term, bidirectional selection for juvenile body weight-Historical perspective. Poultry Sci 2013; 92:1724-34; http://dx.doi.org/10.3382/ps.2013-03069
- Kwon HS, Harris RA. Mechanisms responsible for regulation of pyruvate dehydrogenase kinase 4 gene expression. Adv Enzyme Regul 2004; 44:109-21; PMID:15581486; http://dx.doi.org/10.1016/j.advenzreg.2003.11.020
- Constantin-Teodosiu D, Constantin D, Stephens F, Laithwaite D, Greenhaff PL. The role of FOXO and PPAR transcription factors in diet-mediated inhibition of PDC activation and carbohydrate oxidation during exercise in humans and the role of pharmacological activation of PDC in overriding these changes. Diabetes 2012; 61:1017-24; PMID:22315317; http://dx.doi.org/10.2337/db11-0799
- Sugden MC. PDK4: A factor in fatness? Obes Res 2003; 11:167-9; PMID:12582209; http://dx.doi.org/10.1038/oby.2003.26
- Guarente L, Kenyon C. Genetic pathways that regulate ageing in model organisms. Nature 2000; 408:255-62; PMID:11089983; http://dx.doi.org/10.1038/35041700
- Gross DN, van den Heuvel APJ, Birnbaum MJ. The role of FoxO in the regulation of metabolism. Oncogene 2008; 27:2320-36; PMID:18391974; http://dx.doi.org/10.1038/onc.2008.25
- Poulos SP, Dodson MV, Hausman GJ. Cell line models for differentiation: preadipocytes and adipocytes. Exp Biol Med (Maywood) 2010; 235:1185-93; PMID:20864461; http://dx.doi.org/10.1258/ebm.2010.010063
- Hollenberg CH, Vost A. Regulation of DNA synthesis in fat cells and stromal elements from rat adipose tissue. J Clin Invest 1969; 47:2485-98; PMID:4304653; http://dx.doi.org/10.1172/JCI105930
- Riordan NH, Ichim TE, Min WP, Wang H, Solano F, Lara F, Alfaro M, Rodriguez JP, Harman RJ, Patel AN, et al. Non-expanded adipose stromal vascular fraction cell therapy for multiple sclerosis. J Transl Med 2009; 7:29; PMID:19393041; http://dx.doi.org/10.1186/1479-5876-7-29
- Schipper HS, Prakken B, Kalkhoven E, Boes M. Adipose tissue-resident immune cells: key players in immunometabolism. Trends Endocrinol Metab 2012; 23:407-15; PMID:22795937; http://dx.doi.org/10.1016/j.tem.2012.05.011
- Frisbie DD, Kisiday JD, Kawcak CE, Werpy NM, McIlwraith CW. Evaluation of adipose-derived stromal vascular fraction or bone marrow-derived mesenchymal stem cells for treatment of osteoarthritis. J Orthop Res 2009; 27:1675-80; PMID:19544397; http://dx.doi.org/10.1002/jor.20933
- Zamperone A, Pietronave S, Merlin S, Colangelo D, Ranaldo G, Medico E, Di Scipio F, Berta GN, Follenzi A, Prat M. Isolation and characterization of a spontaneously immortalized multipotent mesenchymal cell line derived from mouse subcutaneous adipose tissue. Stem Cells Dev 2013; 22:2873-84; PMID:23777308; http://dx.doi.org/10.1089/scd.2012.0718
- Chen SY, Mahabole M, Horesh E, Wester S, Goldberg JL, Tseng SC. Isolation and characterization of mesenchymal progenitor cells from human orbital adipose tissue. Invest Ophthalmol Vis Sci 2014; 55:4842-52; PMID:24994870; http://dx.doi.org/10.1167/iovs.14-14441
- Thalmann S, Juge-Aubry CE, Meier CA. Explant cultures of white adipose tissue. Methods Mol Biol 2008; 456:195-9; PMID:18516562; http://dx.doi.org/10.1007/978-1-59745-245-8_14
- Priya N, Sarcar S, Majumdar AS, SundarRaj S. Explant culture: a simple, reproducible, efficient and economic technique for isolation of mesenchymal stromal cells from human adipose tissue and lipoaspirate. J Tissue Eng Regen Med 2014; 8:706-16; PMID:22837175; http://dx.doi.org/10.1002/term.1569
- Ramsay TG, Rosebrough RW. Hormonal regulation of postnatal chicken preadipocyte differentiation in vitro. Comparative Biochem Physiol Part B, Biochem Mol Biol 2003; 136:245-53; http://dx.doi.org/10.1016/S1096-4959(02)00261-0
- Braun EJ, Sweazea KL. Glucose regulation in birds. Comparative Biochem Physiol Part B, Biochem Mol Biol 2008; 151:1-9; http://dx.doi.org/10.1016/j.cbpb.2008.05.007
- Simon J, Milenkovic D, Godet E, Cabau C, Collin A, Metayer-Coustard S, Rideau N, Tesseraud S, Derouet M, Crochet S, et al. Insulin immuno-neutralization in fed chickens: effects on liver and muscle transcriptome. Physiol Genomics 2012; 44:283-92; PMID:22214599; http://dx.doi.org/10.1152/physiolgenomics.00057.2011
- Choudhery MS, Badowski M, Muise A, Pierce J, Harris DT. Donor age negatively impacts adipose tissue-derived mesenchymal stem cell expansion and differentiation. J Transl Med 2014; 12:8; PMID:24397850; http://dx.doi.org/10.1186/1479-5876-12-8
- Kershaw EE, Flier JS. Adipose tissue as an endocrine organ. J Clin Endocrinol Metab 2004; 89:2548-56; PMID:15181022; http://dx.doi.org/10.1210/jc.2004-0395
- Bamshad M, Aoki VT, Adkison MG, Warren WS, Bartness TJ. Central nervous system origins of the sympathetic nervous system outflow to white adipose tissue. Am J Physiol 1998; 275:R291-9; PMID:9688991
- Bamshad M, Song CK, Bartness TJ. CNS origins of the sympathetic nervous system outflow to brown adipose tissue. Am J Physiol 1999; 276:R1569-78; PMID:10362733
- Jansen AS, Farwell DG, Loewy AD. Specificity of pseudorabies virus as a retrograde marker of sympathetic preganglionic neurons: implications for transneuronal labeling studies. Brain Res 1993; 617:103-12; PMID:8397044; http://dx.doi.org/10.1016/0006-8993(93)90619-X
- Strack AM, Loewy AD. Pseudorabies virus: a highly specific transneuronal cell body marker in the sympathetic nervous system. J Neurosci 1990; 10:2139-47; PMID:1695943
- Giordano A, Song CK, Bowers RR, Ehlen JC, Frontini A, Cinti S, Bartness TJ. White adipose tissue lacks significant vagal innervation and immunohistochemical evidence of parasympathetic innervation. Am J Physiol Regulatory Integrative Comparative Physiol 2006; 291:R1243-55; http://dx.doi.org/10.1152/ajpregu.00679.2005
- Oh CM, Namkung J, Go Y, Shong KE, Kim K, Kim H, Park BY, Lee HW, Jeon YH, Song J, et al. Regulation of systemic energy homeostasis by serotonin in adipose tissues. Nat Commun 2015; 6:6794; PMID:25864946; http://dx.doi.org/10.1038/ncomms7794
- Nicolaysen A, Gammelsaeter R, Storm-Mathisen J, Gundersen V, Iversen PO. The components required for amino acid neurotransmitter signaling are present in adipose tissues. J Lipid Res 2007; 48:2123-32; PMID:17600303; http://dx.doi.org/10.1194/jlr.M700021-JLR200
- Dodd GT, Decherf S, Loh K, Simonds SE, Wiede F, Balland E, Merry TL, Münzberg H, Zhang ZY, Kahn BB, et al. Leptin and insulin act on POMC neurons to promote the browning of white fat. Cell 2015; 160:88-104; PMID:25594176; http://dx.doi.org/10.1016/j.cell.2014.12.022
- Zhang Y, Rodrigues E, Li G, Gao Y, King M, Carter CS, Tumer N, Cheng KY, Scarpace PJ. Simultaneous POMC gene transfer to hypothalamus and brainstem increases physical activity, lipolysis and reduces adult-onset obesity. Eur J Neurosci 2011; 33:1541-50; PMID:21366729; http://dx.doi.org/10.1111/j.1460-9568.2011.07633.x
- Bowers RR, Festuccia WT, Song CK, Shi H, Migliorini RH, Bartness TJ. Sympathetic innervation of white adipose tissue and its regulation of fat cell number. Am J Physiol Regulatory, Integrative Comparative Physiol 2004; 286:R1167-75; http://dx.doi.org/10.1152/ajpregu.00558.2003
- Gotzsche CR, Woldbye DP. The role of NPY in learning and memory. Neuropeptides 2015; 55:79–89; PMID:26454711
- Tilan J, Kitlinska J. Neuropeptide Y (NPY) in tumor growth and progression: Lessons learned from pediatric oncology. Neuropeptides 2015; 55:55-66
- Lundberg JM, Franco-Cereceda A, Hemsen A, Lacroix JS, Pernow J. Pharmacology of noradrenaline and neuropeptide tyrosine (NPY)-mediated sympathetic cotransmission. Fundamental Clin Pharmacol 1990; 4:373-91; http://dx.doi.org/10.1111/j.1472-8206.1990.tb00692.x
- Cortes V, Donoso MV, Brown N, Fanjul R, Lopez C, Fournier A, Huidobro-Toro JP. Synergism between neuropeptide Y and norepinephrine highlights sympathetic cotransmission: studies in rat arterial mesenteric bed with neuropeptide Y, analogs, and BIBP 3226. J Pharmacol Exp Ther 1999; 289:1313-22; PMID:10336522
- Ruohonen ST, Savontaus E, Rinne P, Rosmaninho-Salgado J, Cavadas C, Ruskoaho H, Koulu M, Pesonen U. Stress-induced hypertension and increased sympathetic activity in mice overexpressing neuropeptide Y in noradrenergic neurons. Neuroendocrinol 2009; 89:351-60; http://dx.doi.org/10.1159/000188602
- al-Arabi A, Andrews JF. Synergistic action by neuropeptide Y (NPY) and norepinephrine (NE) on food intake, metabolic rate, and brown adipose tissue (BAT) causes remarkable weight loss in the obese (fa/fa) Zucker rat. Biomed Sci Instrum 1997; 33:216-25; PMID:9731362
- Li R, Guan H, Yang K. Neuropeptide Y potentiates β-adrenergic stimulation of lipolysis in 3T3-L1 adipocytes. Regulatory Peptides 2012; 178:16-20; PMID:22750277; http://dx.doi.org/10.1016/j.regpep.2012.06.002
- Vahatalo LH, Ruohonen ST, Makela S, Kovalainen M, Huotari A, Makela KA, Määttä JA, Miinalainen I, Gilsbach R, Hein L, et al. Neuropeptide Y in the noradrenergic neurones induces obesity and inhibits sympathetic tone in mice. Acta Physiol (Oxf) 2015; 213:902-19; PMID:25482272; http://dx.doi.org/10.1111/apha.12436
- Ravelli GP, Stein ZA, Susser MW. Obesity in young men after famine exposure in utero and early infancy. N Engl J Med 1976; 295:349-53; PMID:934222; http://dx.doi.org/10.1056/NEJM197608122950701
- Tang W, Zeve D, Suh JM, Bosnakovski D, Kyba M, Hammer RE, Tallquist MD, Graff JM. White fat progenitor cells reside in the adipose vasculature. Science 2008; 322:583-6; PMID:18801968; http://dx.doi.org/10.1126/science.1156232
- Han R, Kitlinska JB, Munday WR, Gallicano GI, Zukowska Z. Stress hormone epinephrine enhances adipogenesis in murine embryonic stem cells by up-regulating the neuropeptide Y system. PloS One 2012; 7:e36609; PMID:22570731; http://dx.doi.org/10.1371/journal.pone.0036609
- Tang HN, Man XF, Liu YQ, Guo Y, Tang AG, Liao EY, Zhou HD. Dose-dependent effects of neuropeptide Y on the regulation of preadipocyte proliferation and adipocyte lipid synthesis via the PPARgamma pathways. Endocrine J 2015; 62:835-46; http://dx.doi.org/10.1507/endocrj.EJ15-0133
- Wan Y, Xue R, Wang Y, Zhang Q, Huang S, Wu W, Ye H, Zhang Z, Li Y. The effect of neuropeptide Y on brown-like adipocyte's differentiation and activation. Peptides 2015; 63:126-33; PMID:25451330; http://dx.doi.org/10.1016/j.peptides.2014.10.018
- Rodriguez-Carballo E, Gamez B, Mendez-Lucas A, Sanchez-Freutrie M, Zorzano A, Bartrons R, Alcántara S, Perales JC, Ventura F. p38alpha function in osteoblasts influences adipose tissue homeostasis. FASEB J 2015; 29:1414-25; PMID:25550462; http://dx.doi.org/10.1096/fj.14-261891
- Shi YC, Baldock PA. Central and peripheral mechanisms of the NPY system in the regulation of bone and adipose tissue. Bone 2012; 50:430-6; PMID:22008645; http://dx.doi.org/10.1016/j.bone.2011.10.001
- Baldock PA, Sainsbury A, Allison S, Lin EJ, Couzens M, Boey D, Enriquez R, During M, Herzog H, Gardiner EM. Hypothalamic control of bone formation: distinct actions of leptin and y2 receptor pathways. J Bone Miner Res 2005; 20:1851-7; PMID:16160743; http://dx.doi.org/10.1359/JBMR.050523
- Ducy P, Amling M, Takeda S, Priemel M, Schilling AF, Beil FT, Shen J, Vinson C, Rueger JM, Karsenty G. Leptin inhibits bone formation through a hypothalamic relay: a central control of bone mass. Cell 2000; 100:197-207; PMID:10660043; http://dx.doi.org/10.1016/S0092-8674(00)81558-5
- Baldock PA, Lee NJ, Driessler F, Lin S, Allison S, Stehrer B, Lin EJ, Zhang L, Enriquez RF, Wong IP, et al. Neuropeptide Y knockout mice reveal a central role of NPY in the coordination of bone mass to body weight. PloS One 2009; 4:e8415; PMID:20027231; http://dx.doi.org/10.1371/journal.pone.0008415
- Sato N, Ogino Y, Mashiko S, Ando M. Modulation of neuropeptide Y receptors for the treatment of obesity. Expert Opin Ther Pat 2009; 19:1401-15; PMID:19743896; http://dx.doi.org/10.1517/13543770903251722
- Parker RM, Herzog H. Regional distribution of Y-receptor subtype mRNAs in rat brain. Eur J Neurosci 1999; 11:1431-48; PMID:10103138; http://dx.doi.org/10.1046/j.1460-9568.1999.00553.x
- Gehlert DR. Role of hypothalamic neuropeptide Y in feeding and obesity. Neuropeptides 1999; 33:329-38; PMID:10657510; http://dx.doi.org/10.1054/npep.1999.0057
- Blomqvist AG, Herzog H. Y-receptor subtypes–how many more? Trends Neurosci 1997; 20:294-8; PMID:9223221; http://dx.doi.org/10.1016/S0166-2236(96)01057-0
- Naveilhan P, Neveu I, Arenas E, Ernfors P. Complementary and overlapping expression of Y1, Y2 and Y5 receptors in the developing and adult mouse nervous system. Neuroscience 1998; 87:289-302; PMID:9722158; http://dx.doi.org/10.1016/S0306-4522(98)00141-9
- Gong HX, Guo XR, Fei L, Guo M, Liu QQ, Chen RH. Lipolysis and apoptosis of adipocytes induced by neuropeptide Y-Y5 receptor antisense oligodeoxynucleotides in obese rats. Acta Pharmacol Sin 2003; 24:569-75; PMID:12791184
- Rosmaninho-Salgado J, Cortez V, Estrada M, Santana MM, Goncalves A, Marques AP, Cavadas C. Intracellular mechanisms coupled to NPY Y2 and Y5 receptor activation and lipid accumulation in murine adipocytes. Neuropeptides 2012; 46:359-66; PMID:22981159; http://dx.doi.org/10.1016/j.npep.2012.08.006
- Singer K, Morris DL, Oatmen KE, Wang T, `DelProposto J, Mergian T, Cho KW, Lumeng CN. Neuropeptide Y is produced by adipose tissue macrophages and regulates obesity-induced inflammation. PloS one 2013; 8:e57929; PMID:23472120; http://dx.doi.org/10.1371/journal.pone.0057929
- Yang K, Guan H, Arany E, Hill DJ, Cao X. Neuropeptide Y is produced in visceral adipose tissue and promotes proliferation of adipocyte precursor cells via the Y1 receptor. FASEB J 2008; 22:2452-64; PMID:18323405; http://dx.doi.org/10.1096/fj.07-100735
- Picard F, Kurtev M, Chung N, Topark-Ngarm A, Senawong T, Machado De Oliveira R, Leid M, McBurney MW, Guarente L. Sirt1 promotes fat mobilization in white adipocytes by repressing PPAR-gamma. Nature 2004; 429:771-6; PMID:15175761; http://dx.doi.org/10.1038/nature02583
- Xu F, Lin B, Zheng X, Chen Z, Cao H, Xu H, Liang H, Weng J. GLP-1 receptor agonist promotes brown remodelling in mouse white adipose tissue through SIRT1. Diabetologia 2016; 59:1059-69; PMID:26924394; http://dx.doi.org/10.1007/s00125-016-3896-5
- Wu J, Cohen P, Spiegelman BM. Adaptive thermogenesis in adipocytes: is beige the new brown? Genes Dev 2013; 27:234-50; PMID:23388824; http://dx.doi.org/10.1101/gad.211649.112
- Xue B, Coulter A, Rim JS, Koza RA, Kozak LP. Transcriptional synergy and the regulation of Ucp1 during brown adipocyte induction in white fat depots. Mol Cell Biol 2005; 25:8311-22; PMID:16135818; http://dx.doi.org/10.1128/MCB.25.18.8311-8322.2005
- Fedorenko A, Lishko PV, Kirichok Y. Mechanism of fatty-acid-dependent UCP1 uncoupling in brown fat mitochondria. Cell 2012; 151:400-13; PMID:23063128; http://dx.doi.org/10.1016/j.cell.2012.09.010
- Park S, Fujishita C, Komatsu T, Kim SE, Chiba T, Mori R, Shimokawa I. NPY antagonism reduces adiposity and attenuates age-related imbalance of adipose tissue metabolism. FASEB J 2014; 28:5337-48; PMID:25205743; http://dx.doi.org/10.1096/fj.14-258384
- Bi S, Kim YJ, Zheng F. Dorsomedial hypothalamic NPY and energy balance control. Neuropeptides 2012; 46:309-14; PMID:23083763; http://dx.doi.org/10.1016/j.npep.2012.09.002
- Chao PT, Yang L, Aja S, Moran TH, Bi S. Knockdown of NPY expression in the dorsomedial hypothalamus promotes development of brown adipocytes and prevents diet-induced obesity. Cell Metab 2011; 13:573-83; PMID:21531339; http://dx.doi.org/10.1016/j.cmet.2011.02.019
- Margareto J, Aguado M, Oses-Prieto JA, Rivero I, Monge A, Aldana I, Marti A, Martínez JA. A new NPY-antagonist strongly stimulates apoptosis and lipolysis on white adipocytes in an obesity model. Life Sci 2000; 68:99-107; PMID:11132250; http://dx.doi.org/10.1016/S0024-3205(00)00916-4
- Alasvand M, Rashidi B, Haghjooy Javanmard S, Khazaei M. Effect of blockade of neuropeptide Y receptor on aortic intima-media thickness and adipose tissue characteristics in normal and obese mice. Iranian J Basic Medical Sci 2015; 18:443-8
- Samuel VT, Shulman GI. Mechanisms for insulin resistance: common threads and missing links. Cell 2012; 148:852-71; PMID:22385956; http://dx.doi.org/10.1016/j.cell.2012.02.017
- Nway NC, Sitticharoon C, Chatree S, Maikaew P. Correlations between the expression of the insulin sensitizing hormones, adiponectin, visfatin, and omentin, and the appetite regulatory hormone, neuropeptide Y and its receptors in subcutaneous and visceral adipose tissues. Obesity Res Clin Practice 2016; 10:256–63.
- Long M, Zhou J, Li D, Zheng L, Xu Z, Zhou S. Long-term over-expression of neuropeptide Y in hypothalamic paraventricular nucleus contributes to adipose tissue insulin resistance partly via the Y5 receptor. PloS One 2015; 10:e0126714; PMID:25993471; http://dx.doi.org/10.1371/journal.pone.0126714
- Rao JR, Keating DJ, Chen C, Parkington HC. Adiponectin increases insulin content and cell proliferation in MIN6 cells via PPARgamma-dependent and PPARgamma-independent mechanisms. Diabetes Obes Metab 2012; 14:983-9; PMID:22594400; http://dx.doi.org/10.1111/j.1463-1326.2012.01626.x
- Golubovic MV, Dimic D, Antic S, Radenkovic S, Djindjic B, Jovanovic M. Relationship of adipokine to insulin sensitivity and glycemic regulation in obese women–the effect of body weight reduction by caloric restriction. Vojnosanit Pregl 2013; 70:284-91; PMID:23607240; http://dx.doi.org/10.2298/VSP1303284V
- Fukuhara A, Matsuda M, Nishizawa M, Segawa K, Tanaka M, Kishimoto K, Matsuki Y, Murakami M, Ichisaka T, Murakami H, et al. Visfatin: a protein secreted by visceral fat that mimics the effects of insulin. Science 2005; 307:426-30; PMID:15604363; http://dx.doi.org/10.1126/science.1097243
- Yang RZ, Lee MJ, Hu H, Pray J, Wu HB, Hansen BC, Shuldiner AR, Fried SK, McLenithan JC, Gong DW. Identification of omentin as a novel depot-specific adipokine in human adipose tissue: possible role in modulating insulin action. Am J Physiol Endocrinol Metab 2006; 290:E1253-61; PMID:16531507; http://dx.doi.org/10.1152/ajpendo.00572.2004
- Yulyaningsih E, Zhang L, Herzog H, Sainsbury A. NPY receptors as potential targets for anti-obesity drug development. Br J Pharmacol 2011; 163:1170-202; PMID:21545413; http://dx.doi.org/10.1111/j.1476-5381.2011.01363.x
- Swierczynski J, Zabrocka L, Goyke E, Raczynska S, Adamonis W, Sledzinski Z. Enhanced glycerol 3-phosphate dehydrogenase activity in adipose tissue of obese humans. Mol Cell Biochem 2003; 254:55-9; PMID:14674682; http://dx.doi.org/10.1023/A:1027332523114
- Sledzinski T, Korczynska J, Goyke E, Stefaniak T, Proczko-Markuszewska M, Kaska L, Swierczynski J. Association between cytosolic glycerol 3-phosphate dehydrogenase gene expression in human subcutaneous adipose tissue and BMI. Cell Physiol Biochem 2013; 32:300-9; PMID:23942261; http://dx.doi.org/10.1159/000354438
- Rice BB, Zhang W, Bai S, Siegel PB, Cline MA, Gilbert ER. Insulin-induced hypoglycemia associations with gene expression changes in liver and hypothalamus of chickens from lines selected for low or high body weight. General Comparative Endocrinol 2014; 208C:1-4; http://dx.doi.org/10.1016/j.ygcen.2014.08.010
- Yi J, Gilbert ER, Siegel PB, Cline MA. Fed and fasted chicks from lines divergently selected for low or high body weight have differential hypothalamic appetite-associated factor mRNA expression profiles. Behavioural Brain Res 2015; 286:58-63; http://dx.doi.org/10.1016/j.bbr.2015.02.008
- Yang Y. Structure, function and regulation of the melanocortin receptors. Eur J Pharmacol 2011; 660:125-30; PMID:21208602; http://dx.doi.org/10.1016/j.ejphar.2010.12.020
- Rodrigues AR, Almeida H, Gouveia AM. Intracellular signaling mechanisms of the melanocortin receptors: current state of the art. Cell Mol Life Sci 2015; 72:1331-45; PMID:25504085; http://dx.doi.org/10.1007/s00018-014-1800-3
- Shipp SL, Yi J, Dridi S, Gilbert ER, Cline MA. The central anorexigenic mechanism of adrenocorticotropic hormone involves the caudal hypothalamus in chicks. Neuropeptides 2015; 53:29-35; PMID:26297349; http://dx.doi.org/10.1016/j.npep.2015.07.005
- Shipp SL, Smith ML, Gilbert ER, Cline MA. Beta-cell-tropin is associated with short-term stimulation of food intake in chicks. General Comparative Endocrinol 2015; 224:278-82; http://dx.doi.org/10.1016/j.ygcen.2015.08.004
- Tung YC, Piper SJ, Yeung D, O'Rahilly S, Coll AP. A comparative study of the central effects of specific proopiomelancortin (POMC)-derived melanocortin peptides on food intake and body weight in pomc null mice. Endocrinol 2006; 147:5940-7; http://dx.doi.org/10.1210/en.2006-0866
- Rees JL. Genetics of hair and skin color. Annu Rev Genet 2003; 37:67-90; PMID:14616056; http://dx.doi.org/10.1146/annurev.genet.37.110801.143233
- Catania A, Gatti S, Colombo G, Lipton JM. Targeting melanocortin receptors as a novel strategy to control inflammation. Pharmacol Rev 2004; 56:1-29; PMID:15001661; http://dx.doi.org/10.1124/pr.56.1.1
- Catania A, Lonati C, Sordi A, Carlin A, Leonardi P, Gatti S. The melanocortin system in control of inflammation. Scientific World J 2010; 10:1840-53; PMID:20852827; http://dx.doi.org/10.1100/tsw.2010.173
- Mountjoy KG. The human melanocyte stimulating hormone receptor has evolved to become “super-sensitive” to melanocortin peptides. Mol Cell Endocrinol 1994; 102:R7-11; PMID:7926261; http://dx.doi.org/10.1016/0303-7207(94)90113-9
- Hadley ME, Haskell-Luevano C. The proopiomelanocortin system. Ann N Y Acad Sci 1999; 885:1-21; PMID:10816638; http://dx.doi.org/10.1111/j.1749-6632.1999.tb08662.x
- Vale W, Spiess J, Rivier C, Rivier J. Characterization of a 41-residue ovine hypothalamic peptide that stimulates secretion of corticotropin and β-endorphin. Science 1981; 213:1394-7; PMID:6267699; http://dx.doi.org/10.1126/science.6267699
- Boston BA. The role of melanocortins in adipocyte function. Ann N Y Acad Sci 1999; 885:75-84; PMID:10816642; http://dx.doi.org/10.1111/j.1749-6632.1999.tb08666.x
- Mountjoy KG. Distribution and function of melanocortin receptors within the brain. Adv Exp Med Biol 2010; 681:29-48; PMID:21222258; http://dx.doi.org/10.1007/978-1-4419-6354-3_3
- Mountjoy KG, Mortrud MT, Low MJ, Simerly RB, Cone RD. Localization of the melanocortin-4 receptor (MC4-R) in neuroendocrine and autonomic control circuits in the brain. Mol Endocrinol 1994; 8:1298-308; PMID:7854347
- Schwartz MW, Woods SC, Porte D, Jr, Seeley RJ, Baskin DG. Central nervous system control of food intake. Nature 2000; 404:661-71; PMID:10766253
- Chen AS, Marsh DJ, Trumbauer ME, Frazier EG, Guan XM, Yu H, Rosenblum CI, Vongs A, Feng Y, Cao L, et al. Inactivation of the mouse melanocortin-3 receptor results in increased fat mass and reduced lean body mass. Nat Genet 2000; 26:97-102; PMID:10973258; http://dx.doi.org/10.1038/79254
- Fathi Z, Iben LG, Parker EM. Cloning, expression, and tissue distribution of a fifth melanocortin receptor subtype. Neurochem Res 1995; 20:107-13; PMID:7739752; http://dx.doi.org/10.1007/BF00995160
- An JJ, Rhee Y, Kim SH, Kim DM, Han DH, Hwang JH, Jin YJ, Cha BS, Baik JH, Lee WT, et al. Peripheral effect of α-melanocyte-stimulating hormone on fatty acid oxidation in skeletal muscle. J Biol Chem 2007; 282:2862-70; PMID:17127674; http://dx.doi.org/10.1074/jbc.M603454200
- Eerola K, Rinne P, Penttinen AM, Vahatalo L, Savontaus M, Savontaus E. α-MSH overexpression in the nucleus tractus solitarius decreases fat mass and elevates heart rate. J Endocrinol 2014; 222:123-36; PMID:24829220; http://dx.doi.org/10.1530/JOE-14-0064
- Perino A, Beretta M, Kilic A, Ghigo A, Carnevale D, Repetto IE, Braccini L, Longo D, Liebig-Gonglach M, Zaglia T, et al. Combined inhibition of PI3Kbeta and PI3Kgamma reduces fat mass by enhancing α-MSH-dependent sympathetic drive. Sci Signal 2014; 7:ra110; PMID:25406378; http://dx.doi.org/10.1126/scisignal.2005485
- Gerozissis K. Brain insulin, energy and glucose homeostasis; genes, environment and metabolic pathologies. Eur J Pharmacol 2008; 585:38-49; PMID:18407262; http://dx.doi.org/10.1016/j.ejphar.2008.01.050
- Becattini B, Marone R, Zani F, Arsenijevic D, Seydoux J, Montani JP, Dulloo AG, Thorens B, Preitner F, Wymann MP, et al. PI3Kgamma within a nonhematopoietic cell type negatively regulates diet-induced thermogenesis and promotes obesity and insulin resistance. Proc Natl Acad Sci U S A 2011; 108:E854-63; PMID:21949398; http://dx.doi.org/10.1073/pnas.1106698108
- Rossi J, Balthasar N, Olson D, Scott M, Berglund E, Lee CE, Choi MJ, Lauzon D, Lowell BB, Elmquist JK. Melanocortin-4 receptors expressed by cholinergic neurons regulate energy balance and glucose homeostasis. Cell Metab 2011; 13:195-204; PMID:21284986; http://dx.doi.org/; http://dx.doi.org/10.1016/j.cmet.2011.01.010
- Mottillo EP, Granneman JG. Intracellular fatty acids suppress β-adrenergic induction of PKA-targeted gene expression in white adipocytes. Am J Physiol Endocrinol Metab 2011; 301:E122-31; PMID:21505145; http://dx.doi.org/10.1152/ajpendo.00039.2011
- Stralfors P, Belfrage P. Phosphorylation of hormone-sensitive lipase by cyclic AMP-dependent protein kinase. J Biol Chem 1983; 258:15146-52; PMID:6317686
- Moller CL, Pedersen SB, Richelsen B, Conde-Frieboes KW, Raun K, Grove KL, Wulff BS. Melanocortin agonists stimulate lipolysis in human adipose tissue explants but not in adipocytes. BMC Res Notes 2015; 8:559; PMID:26459134; http://dx.doi.org/10.1186/s13104-015-1539-4
- Martinez-Gonzalez J, Badimon L. The NR4A subfamily of nuclear receptors: new early genes regulated by growth factors in vascular cells. Cardiovasc Res 2005; 65:609-18; PMID:15664387; http://dx.doi.org/10.1016/j.cardiores.2004.10.002
- Maxwell MA, Muscat GE. The NR4A subgroup: immediate early response genes with pleiotropic physiological roles. Nucl Recept Signal 2006; 4:e002; PMID:16604165; http://dx.doi.org/10.1621/nrs.04002
- Wang SC, Myers SA, Eriksson NA, Fitzsimmons RL, Muscat GE. Nr4a1 siRNA expression attenuates α-MSH regulated gene expression in 3T3-L1 adipocytes. Mol endocrinol 2011; 25:291-306; PMID:21239615; http://dx.doi.org/10.1210/me.2010-0231
- Cline MA, Smith ML. Central α-melanocyte stimulating hormone attenuates behavioral effects of neuropeptide Y in chicks. Physiol Behavior 2007; 91:588-92; http://dx.doi.org/10.1016/j.physbeh.2007.03.021
- Raposinho PD, White RB, Aubert ML. The melanocortin agonist Melanotan-II reduces the orexigenic and adipogenic effects of neuropeptide Y (NPY) but does not affect the NPY-driven suppressive effects on the gonadotropic and somatotropic axes in the male rat. J Neuroendocrinol 2003; 15:173-81; PMID:12535159; http://dx.doi.org/10.1046/j.1365-2826.2003.00962.x
- Baltazi M, Katsiki N, Savopoulos C, Iliadis F, Koliakos G, Hatzitolios AI. Plasma neuropeptide Y (NPY) and α-melanocyte stimulating hormone (a-MSH) levels in patients with or without hypertension and/or obesity: a pilot study. Am J Cardiovasc Dis 2011; 1:48-59; PMID:22254185
- Nam SY, Kratzsch J, Kim KW, Kim KR, Lim SK, Marcus C. Cerebrospinal fluid and plasma concentrations of leptin, NPY, and α-MSH in obese women and their relationship to negative energy balance. J Clin Endocrinol Metab 2001; 86:4849-53; PMID:11600552
- Rosmaninho-Salgado J, Marques AP, Estrada M, Santana M, Cortez V, Grouzmann E, Cavadas C. Dipeptidyl-peptidase-IV by cleaving neuropeptide Y induces lipid accumulation and PPAR-gamma expression. Peptides 2012; 37:49-54; PMID:22819773; http://dx.doi.org/10.1016/j.peptides.2012.06.014
- Labelle M, Boulanger Y, Fournier A, St Pierre S, Savard R. Tissue-specific regulation of fat cell lipolysis by NPY in 6-OHDA-treated rats. Peptides 1997; 18:801-8; PMID:9285928; http://dx.doi.org/10.1016/S0196-9781(97)00028-4
- Della-Zuana O, Revereault L, Beck-Sickinger A, Monge A, Caignard DH, Fauchere JL, Henlin JM, Audinot V, Boutin JA, Chamorro S, et al. A potent and selective NPY Y5 antagonist reduces food intake but not through blockade of the NPY Y5 receptor. Int J Obes Relat Metab Disord 2004; 28:628-39; PMID:14758341; http://dx.doi.org/10.1038/sj.ijo.0802435
- Hahn TM, Breininger JF, Baskin DG, Schwartz MW. Coexpression of Agrp and NPY in fasting-activated hypothalamic neurons. Nat Neurosci 1998; 1:271-2; PMID:10195157; http://dx.doi.org/10.1038/1082
- Ishihara A, Kanatani A, Mashiko S, Tanaka T, Hidaka M, Gomori A, Iwaasa H, Murai N, Egashira S, Murai T, et al. A neuropeptide Y Y5 antagonist selectively ameliorates body weight gain and associated parameters in diet-induced obese mice. Proc Natl Acad Sci U S A 2006; 103:7154-8; PMID:16636293; http://dx.doi.org/10.1073/pnas.0510320103
- Billington CJ, Briggs JE, Grace M, Levine AS. Effects of intracerebroventricular injection of neuropeptide Y on energy metabolism. Am J Physiol 1991; 260:R321-7; PMID:1996719
- Shimada K, Ohno Y, Okamatsu-Ogura Y, Suzuki M, Kamikawa A, Terao A, Kimura K. Neuropeptide Y activates phosphorylation of ERK and STAT3 in stromal vascular cells from brown adipose tissue, but fails to affect thermogenic function of brown adipocytes. Peptides 2012; 34:336-42; PMID:22374387; http://dx.doi.org/10.1016/j.peptides.2012.02.012