ABSTRACT
Retinoic acid (RA) is essential for early developmental processes and stem cell differentiation, but less is known about its contributions to adult tissues and stem cells including adipose tissue. We previously demonstrated that many genes involved in RA synthesis and downstream pathway are differentially expressed in adipose-derived stem cells (ASCs) from visceral fat compared to those from subcutaneous fat, leading to changes in their early adipogenic functions. In order to study potential contributions of RA in adipose tissue, we measured tissue RA levels using a technique based on surface-enhanced Raman spectroscopy (SERS). The data indicate heretofore underappreciated abundance of endogenous RA in mouse adipose tissue compared to other tissues and dynamic changes of RA concentrations after high fat diet feeding. Our results lay the foundation for further investigation on the functional role of RA in adipose tissue development and metabolism.
Introduction
Adipose tissue is now recognized as an important endocrine organ that mediates lipid storage, metabolism, insulin signaling, adipokine secretion and inflammation. However, pathophysiological contributions of white fat/adipose tissue (WF) are not uniform when it is expanded during onset of obesity. Subcutaneous (SC) fat is considered protective against developing metabolic diseases because this depot is anti-inflammatory, insulin sensitive, stores excess calories safely, and secretes ‘good’ adipokines.Citation1-3 In contrast, expansion of visceral (VS) fat is detrimental and results in local inflammation, insulin resistance, lipid spillover into circulation and secretion of ‘bad’ adipokines. The molecular basis underlying this difference, however, remains obscure. It was previously shown that different depots of adipose tissue and cells in human and rodents exhibit distinct gene expression patterns, especially in the group of developmental genes (e.g. Hox family).Citation4-6 However, how these developmental gene differences lead to pathophysiological differences is not clear. In vitro, this difference can be recapitulated by adipocyte differentiation of progenitor or stem cell populations from these fat depots. Cells from SC fat undergo adipogenesis well under standard adipogenic stimuli whereas VS fat-derived cells poorly differentiate under the same condition.Citation7-9 We hypothesized that at least part of these adipogenic differences originate from intrinsic differences in stem cell populations, adipose-derived stem cells (ASCs), from these 2 depots.
Retinoic acid (RA) is a vitamin A metabolite that is an essential signaling molecule for many developmental processes, mainly through specific binding to its nuclear receptors (i.e. Retinoic Acid Receptors [RARs] and Retinoid X Receptors [RXRs]) and regulating downstream target genes.Citation10,11 Although liver is presumably the main storage location of vitamin A species, adipose tissue was proposed to be a dynamic and important site of their storage and activities.Citation12 However, less is understood about the developmental and metabolic contributions of RA in adipose tissue, partly due to challenge of measuring endogenous RA levels. To our knowledge, only one paper comprehensively quantified endogenous RA levels of different tissues by liquid chromatography coupled with tandem mass spectrometry.Citation13 Their results indicate that while liver contains the highest amount of all-trans RA, epididymal adipose tissue is among the second abundant group of mouse tissues together with kidney and brain, when the amount was calculated per weight of tissue. The amount of other fat depots such as SC-WF and brown fat was not examined. Here we summarize our recent study on the role of RA and its signaling pathway in adipogenic functions of ASCs from SC and VS fat depots. We then assessed endogenous concentrations of adipose and other tissues by developing a technique of RA detection based on surface-enhanced Raman spectroscopy (SERS) and revealed heretofore unrecognized abundance of RA in different fat depots compared to other tissues.
Results and discussion
In order to identify a molecular mechanism that underlies cellular differences between SC and VS adipose tissues, we previously performed genome-wide gene expression analyses involving RNA-seq of tissues and microarray of ASCs.Citation14 Gene ontology of these analyses indicated that genes categorized in “retinoid metabolic process” are among the top annotation that represents differential expression in SC versus VS at both tissue and stem cell levels. These include genes involved in RA synthesis / metabolism and its downstream targets, which were confirmed by quantitative real-time PCR and summarized in . We found that genes mediating RA production (RDH10, DHRS3, ALDH1A2, and CRBP1) are higher in VS-ASCs than SC-ASCs and that CYP26B gene, which participates in metabolizing RA, is downregulated in VS-ASCs. As expected from the gene expression changes, endogenous RA levels were found higher in VS-ASCs than SC-ASCs.Citation14 Consequently, many genes known to be downstream targets of RA were confirmed to be differentially expressed. These include VS-enriched genes (NR2F1, MGP, RARRES1, RARRES2, PLAT, PTGS1, CDKN2B, HOXA4, HOXA5, BAPX1, F3, MEIS1, and CDH6) and SC-enriched genes (MME, PITX2, TNC, ENPP2, CTSK, and CRYAB). Among the VS-enriched genes, NR2F1, MGP, RARRES1, RARRES2, PLAT, CDKN2B, HOXA4, HOXA5, F3, and CDH6 showed increased expression when SC-ASCs were treated with exogenous RA in the concentration-dependent manner. On the other hand, many of the SC-enriched genes including MME, TNC, ENPP2, and CRYAB exhibited concentration-dependent decrease in expression when SC-ASCs were subjected to exogenous RA.Citation14 Among the differentially expressed genes includes RARB, one of the RA receptors (RARβ) that is induced by RA itself. Functionally, exogenous RA inhibits adipocyte differentiation of ASCs when added at the early stage (either before differentiation or the first 6 d of adipogenesis), but not at the late stage.Citation14 During adipogenesis of SC-ASCs, RA treatment significantly inhibited expression of late adipogenic genes (CEBPA and PPARG), but not early adipogenic genes (CEBPB and CEBPD). This gene expression changes are very similar to those during adipogenesis of VS-ASCs. These results suggested that excess amount of RA mediates functional differences of ASCs from 2 depots. In fact, blocking RA-specific receptor activities by treatment of VS-ASCs with pan-RARs antagonist (BMS493) or RARβ-specific antagonist (LE135) significantly reversed adipogenic defects caused by excessive RA. In order to identify a potential upstream regulator that leads to overproduction of RA, we investigated the contribution of WT1, which is a mesothelial developmental marker and transcription factor that is specifically expressed in VS fat in mice.Citation15 We found that WT1, which was also specifically expressed in VS-ASCs, binds to promoter of human ALDH1A2 gene and controls its expression. Knockdown of WT1 dramatically suppressed ALDH1A2 expression and significantly improved the adipocyte differentiation defect of VS-ASCs.Citation14 Collectively, our results pointed to the role of RA in mediating depot-specific adipogenic gene program and development in human WF.
Figure 1. RA pathway is differentially regulated in stem cells from VS and SC fat depots. A schematic illustrating RA synthesis / metabolism and downstream pathway regulated by RA. RA is produced in 2 sequential steps from retinol to retinal (a.k.a. retinaldehyde) by RDH10 and DHRS3, then to all-trans RA by 3 isoforms of ALDH1. WT1, a developmental marker of mesothelial layer including VS fat, controls expression of ALDH1A2 isoform. CRBP1 transports cellular retinol and retinal. Finally CYP26 family metabolizes RA into hydroxy-RA. RA acts as a major signaling molecule that binds to its receptors, RARs and RXRs. RARs then bind to its response elements (RARE), leading to modulation of various downstream target genes. Genes highlighted in red are those upregulated in VS-ASCs whereas those highlighted in blue upregulated in SC-ASCs.
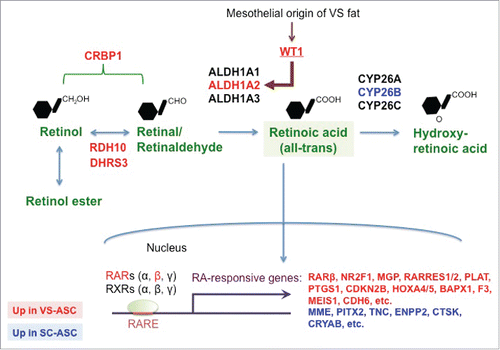
These findings prompted us to further explore RA in the context of adipose tissue. While a number of studies have investigated the potential role of retinol binding proteins such as CRBP1 and RBP4 in adipose tissue,Citation16,17 much less is known about the potential significance of endogenous RA in this tissue, partially due to challenges of analytical techniques to detect RA. We previously employed 3 methods to measure endogenous RA levels, namely indirect Luciferase-based reporter assay under the control of RA response element, LC/MS-based measurement, and SERS-based quantification.Citation14 We further took advantage of the SERS method we developed in assessing endogenous RA levels for adipose tissue in comparison to other tissues from mice. The principle of our protocol is similar to ELISA using HRP. In order to perform the peroxidase reaction we tested various commercially available chromogenic substrates that undergo instant oxidation reaction with peroxidase enzymes in presence of H2O2. We then selected 3,3',5,5'-tetramethylbenzidine (TMB) as the ideal substrate for our study due to its unique property, less toxicity and more sensitivity compared to commonly used peroxidase reactants.Citation18,19 Hence, TMB was used as peroxidase substrate for detection and quantification of RA. shows the peroxidase reaction of TMB to TMB2+ using HRP conjugate in presence of H2O2. Upon the completion of the peroxidase reaction in presence of HRP conjugate TMB which was colorless is converted to a blue colored intermittent product charge transfer complex (CTC). Strong acids such as sulfuric acid or hydrochloric acid can be used to terminate the reaction, which results in a yellow colored product TMB2+. The SERS method is sensitive to CTC and TMB2+, making the detection and quantification possible. This peroxidase product was then mixed with the 60 nm gold colloid. shows the SERS spectra of the reactant and product. The pure reactant TMB does not exhibit any Raman activity whereas TMB2+ showed very distinct and clear spectra as a result of azide bond formation. The product SERS spectra show specific Raman bands at 1191, 1337, and 1605 cm−1 that is characteristic of TMB2+.Citation14,19 The intensity of the specific Raman band corresponding to 1605 cm−1 wavenumber was selected as the peak of interest as its intensity is inversely proportional to the concentration of RA present in the sample ().
Figure 2. SERS measurement of RA levels. (A) Reaction scheme for the peroxidase reaction of TMB to TMB2+ using HRP conjugate as enzyme. Peroxidase product TMB2+ was then mixed with Au colloid for SERS measurement. (B) SERS spectra of the reactant TMB and product TMB2+ are shown for comparison. (C) RA concentration dependent SERS spectra of TMB2+. RA levels were calculated based on the intensity of 1605 cm−1 peak from TMB2+.
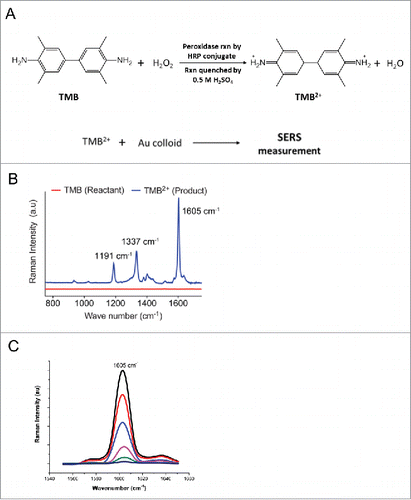
This SERS method was used to quantify endogenous RA levels present in different mouse tissues. Tissues from different organs of mice under study were inguinal Subcutaneous white fat (SC-WF), epididymal Visceral white fat (VS-WF), Brown fat (BF) from the interscapular region, Eyes, Liver, Skeletal muscle (Quadriceps) (SkM), Heart, Spleen (Sple.), Kidney (Kidn.) and Pancreas (Panc.). As shown in , we found from the current SERS study that VS fat showed relatively high, but not statistically significant, RA concentration compared to SC fat in normal chow (NC)-fed mice. When normalized against protein concentration, all adipose tissue depots including VS, SC and BF show significantly higher RA levels than other organs such as liver and eye, which were believed to be main sites of RA signaling. We also performed RA measurement in the high fat (HF) diet-fed mice. The trend seems to be somewhat different in the case of mice fed with HF diet. It was found that SC fat exhibits slightly higher concentration of RA compared to VS fat in HF mice although it does not reach statistical significance (). In contrast, the RA level in BF remains slightly below that of VS and SC fat in mice under both HF diet and NC diet. Also, there were changes in RA levels observed between fat from NC and HF diet, but none of them were statistically significant. This may be due to saturated levels of RA detected by our experimental setting; the RA levels in fats are high and may be beyond the threshold level detected by our system. Alternatively, the fat samples collected were from different age groups of NC (10-week old) and HF (20-week old) mice; this may account for the lack of significant difference in RA levels.
Figure 3. Endogenous RA levels in different tissues of mice under NC diet. Different tissues were harvested from 10-week old, male C57Bl/6J mice fed on normal chow (NC) diet (n = 4). Tissues investigated for RA concentrations by SERS were SC-WF (inguinal Subcutaneous White Fat), VS-WF (epididymal Visceral White Fat), BF (Brown Fat from the interscapular region), Eyes, Liver, SkM (quadriceps Skeletal Muscle), Heart, Sple. (Spleen), Kidn. (Kidney), Panc. (Pancreas). This result is a representative of 2 independent experiments. *p < 0.05, **p < 0.01.
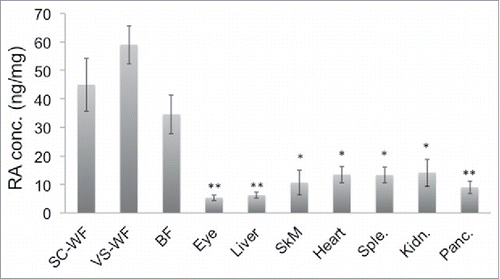
Figure 4. Endogenous RA levels in different tissues of mice under HF diet. The same set of tissues as in were harvested from 20-week old, male C57Bl/6J mice fed on high fat (HF) diet for 12 weeks (n = 3). The tissues were then measured for their RA levels by SERS. The result shown is a representative of 2 independent experiments. *p < 0.05, **p < 0.01.
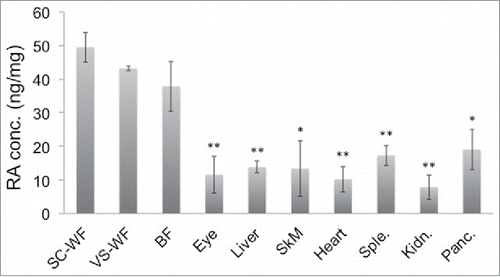
Kane and NapoliCitation20 have shown a reliable method using LC/MS to quantify endogenous retinoids including RA in adult mouse tissues; their method detected RA in epididymal adipose tissues in adult mouse in the pmol/g range. Together with this reportCitation20 and our results, it is concluded that our detection method can quantify previously unrecognized abundance and differences in endogenous RA and its potential importance in adipose tissue, which necessitates further studies on its pathway in this tissue.
Materials and methods
Experimental procedures of ASCs
Human ASCs were isolated and cultured as previously described.Citation8,21,22 All the experiments performed on these ASCs were recently reported.Citation14
Mouse experiments
Animal procedure described was approved by and compliant with the guidelines of Institutional Animal Care and Use Committee (IACUC) of A*STAR's Biological Resource Center. Tissues from different organs were harvested from each mouse and their tissue lysate was prepared. 10-week old, male C57Bl/6J mice fed on normal chow (NC) diet and 20-week old, male C57Bl/6J mice fed on high fat (HF) diet were used to harvest various organs. The mice on HF diet (OpenSource diets-D12492) were 8-weeks old when they were started on HF diet and they were fed for 12 weeks. The individual weights of every organ was recorded. Once the organs were harvested, they were immediately snap frozen in liquid nitrogen and lysates were made by mechanical disruption in 1X PBS. We then performed centrifugation step (12,000 xg, 10 minutes at 4°C) in order to collect the supernatant containing RA in it. The samples were tested along with the known standard RA to elucidate the final concentration of RA present in each organ. Each experiment was performed in triplicate and the average value is represented for each sample. For each sample the SERS results obtained were normalized against the concentrations of protein present, which were determined by Bradford assay (Bio-Rad Laboratories Inc.).
RA measurement by SERS
Human retinoic acid ELISA kit was obtained from MyBioSource. All reagents and samples were brought to room temperature before use. The samples and concentrated HRP (horseradish peroxidase)-conjugate were centrifuged before the assay. 2 µL of the sample supernatant was diluted with 48 µL of the sample diluent provided in the kit and 15 µl of the concentrated HRP-conjugate was diluted with 1485 µl of HRP-conjugate diluent. Additionally one empty well was set as blank. 50 µl of diluted sample was added to each micro-well followed by addition of 50 µl of HRP-conjugate. Each micro-well was coated with anti-retinoic acid solution to capture RA (MyBioSource). The plate was shaken gently for 1 min to ensure thorough mixing and then covered with the adhesive strip provided. The samples were incubated for 60 min at 25°C. A plate layout was used to record standard and samples assayed. Each micro-well was aspirated and washed, repeating the process 4 times for a total of 5 washes. Each micro-well was washed with 200 µl of Wash buffer from the kit and left to stand for 2 minutes. All the micro-wells were emptied devoid of any liquid for good performance. After the last wash, the plates were dried by inverting and blotting against clean kim wipes/paper towels to remove residual Wash buffer. Finally, 90 µl of TMB which is a peroxidase substrate (MyBioSource) was added to each micro-well except the blank. The plate was protected from light by covering it using adhesive tape and incubated for 20 min at 25°C. After 20 min 50 µl of Stop Solution of the kit was added to each micro-well to quench the reaction and the plate was tapped gently to ensure thorough mixing. In order to do the SERS measurement, Au colloid gold nanoparticle with 60 nm average particle size (from BBI solutions, USA) was used as plasmonic substrate for signal enhancement for this study. 10 µl aliquot of the sample solution was added onto 45 µl of gold nanoparticles with thorough mixing. 20 µl of the resultant solution was used for SERS measurements.
SERS measurements were done using Raman inVia microscope from Renishaw, UK.
The conditions for the SERS measurements are as per our previous work.Citation14,19 Briefly we used 633 nm excitation laser with 10% laser power, 1800 line/mm grating along with cooled CCD. For sample focusing we used 20X objective lens with N.A 0.4. Notch filter was used to block Rayleigh scattering. The laser power used for this study corresponds to 0.28 mW. Each measurement needs 10S integration time and SERS measurements were taken at multiple locations on the sample and in the end the data were averaged. Wire 3.4, Renishaw software was used to do the necessary background correction needed. Silicon was used for the calibration of the laser output.
Data analysis
All results are presented as means +/− SEM. Comparison between groups was analyzed using t-tests (2-tailed; paired). Differences with p value of less than 0.05 were considered significant.
Abbreviations
ASC | = | adipose-derived stem cell |
BF | = | brown fat tissue |
HRP | = | horseradish peroxidase |
RA | = | retinoic acid |
RAR | = | retinoic acid receptor |
RXR | = | retinoid X receptor |
SC | = | subcutaneous |
SERS | = | surface-enhanced Raman spectroscopy |
TMB | = | 3,3′,5,5′-Tetramethylbenzidine |
VS | = | visceral |
WF | = | white fat tissue |
Disclosure of potential conflicts of interest
S. Sugii is a co-founder and shareholder of Adigenics Pte Ltd, which has not had any financial or scientific influence on this study.
Author contributions
J.P., S.Sr. and H.Q.L. performed the experiments, analyzed data and wrote the manuscript. M.O. supervised the experiments and edited the manuscript. S.Su. supervised the experiments, analyzed data and wrote the manuscript.
Acknowledgments
We thank members of Laboratory of Metabolic Medicine (LMM), Bio-Optical Imaging Group (BOIG) for helping our research activities.
Funding
This work is supported by an intramural funding from Biomedical Research Council of Agency for Science, Technology and Research (A*STAR) to M.O. and S.Su.
References
- Sugii S, Olson P, Sears DD, Saberi M, Atkins AR, Barish GD, Hong SH, Castro GL, Yin YQ, Nelson MC, et al. PPARgamma activation in adipocytes is sufficient for systemic insulin sensitization. Proc Natl Acad Sci U S A 2009; 106:22504-9; PMID:20018750; http://dx.doi.org/10.1073/pnas.0912487106
- Tran TT, Kahn CR. Transplantation of adipose tissue and stem cells: role in metabolism and disease. Nat Rev Endocrinol 2010; 6:195-213; PMID:20195269; http://dx.doi.org/10.1038/nrendo.2010.20
- Tran TT, Yamamoto Y, Gesta S, Kahn CR. Beneficial effects of subcutaneous fat transplantation on metabolism. Cell Metab 2008; 7:410-20; PMID:18460332; http://dx.doi.org/10.1016/j.cmet.2008.04.004
- Vohl MC, Sladek R, Robitaille J, Gurd S, Marceau P, Richard D, Hudson TJ, Tchernof A. A survey of genes differentially expressed in subcutaneous and visceral adipose tissue in men. Obesity Res 2004; 12:1217-22; PMID:15340102; http://dx.doi.org/10.1038/oby.2004.153
- Gesta S, Bluher M, Yamamoto Y, Norris AW, Berndt J, Kralisch S, Boucher J, Lewis C, Kahn CR. Evidence for a role of developmental genes in the origin of obesity and body fat distribution. Proc Natl Acad Sci U S A 2006; 103:6676-81; PMID:16617105; http://dx.doi.org/10.1073/pnas.0601752103
- Tchkonia T, Lenburg M, Thomou T, Giorgadze N, Frampton G, Pirtskhalava T, Cartwright A, Cartwright M, Flanagan J, Karagiannides I, et al. Identification of depot-specific human fat cell progenitors through distinct expression profiles and developmental gene patterns. Am J Physiol Endocrinol Metab 2007; 292:E298-307; PMID:16985259; http://dx.doi.org/10.1152/ajpendo.00202.2006
- Macotela Y, Emanuelli B, Mori MA, Gesta S, Schulz TJ, Tseng YH, Kahn CR. Intrinsic differences in adipocyte precursor cells from different white fat depots. Diabetes 2012; 61:1691-9; PMID:22596050; http://dx.doi.org/10.2337/db11-1753
- Ong WK, Tan CS, Chan KL, Goesantoso GG, Chan XH, Chan E, Yin J, Yeo CR, Khoo CM, So JB, et al. Identification of specific cell-surface markers of adipose-derived stem cells from subcutaneous and visceral fat depots. Stem Cell Rep 2014; 2:171-9; PMID:24527391; http://dx.doi.org/10.1016/j.stemcr.2014.01.002
- Tchkonia T, Tchoukalova YD, Giorgadze N, Pirtskhalava T, Karagiannides I, Forse RA, Koo A, Stevenson M, Chinnappan D, Cartwright A, et al. Abundance of two human preadipocyte subtypes with distinct capacities for replication, adipogenesis, and apoptosis varies among fat depots. Am J Physiol Endocrinol Metab 2005; 288:E267-77; PMID:15383371; http://dx.doi.org/10.1152/ajpendo.00265.2004
- Balmer JE, Blomhoff R. Gene expression regulation by retinoic acid. J Lipid Res 2002; 43:1773-808; PMID:12401878; http://dx.doi.org/10.1194/jlr.R100015-JLR200
- D'Ambrosio DN, Clugston RD, Blaner WS. Vitamin A metabolism: an update. Nutrients 2011; 3:63-103; PMID:21350678; http://dx.doi.org/10.3390/nu3010063
- Bonet ML, Canas JA, Ribot J, Palou A. Carotenoids and their conversion products in the control of adipocyte function, adiposity and obesity. Archives Biochem Biophys 2015; 572:112-25; PMID:25721497; http://dx.doi.org/10.1016/j.abb.2015.02.022
- Kane MA, Folias AE, Wang C, Napoli JL. Quantitative profiling of endogenous retinoic acid in vivo and in vitro by tandem mass spectrometry. Anal Chem 2008; 80:1702-8; PMID:18251521; http://dx.doi.org/10.1021/ac702030f
- Takeda K, Sriram S, Chan XH, Ong WK, Yeo CR, Tan B, Lee SA, Kong KV, Hoon S, Jiang H, et al. Retinoic acid mediates visceral-specific adipogenic defects of human adipose-derived stem cells. Diabetes 2016; 65:1164-78; PMID:26936961; http://dx.doi.org/10.2337/db15-1315
- Chau YY, Bandiera R, Serrels A, Martinez-Estrada OM, Qing W, Lee M, Slight J, Thornburn A, Berry R, McHaffie S, et al. Visceral and subcutaneous fat have different origins and evidence supports a mesothelial source. Nat Cell Biol 2014; 16:367-75; PMID:24609269; http://dx.doi.org/10.1038/ncb2922
- Frey SK, Vogel S. Vitamin A metabolism and adipose tissue biology. Nutrients 2011; 3:27-39; PMID:22254074; http://dx.doi.org/10.3390/nu3010027
- Kotnik P, Fischer-Posovszky P, Wabitsch M. RBP4: a controversial adipokine. Eur J Endocrinol 2011; 165:703-11; PMID:21835764; http://dx.doi.org/10.1530/EJE-11-0431
- Laing S, Hernandez-Santana A, Sassmannshausen J, Asquith DL, McInnes IB, Faulds K, Graham D. Quantitative detection of human tumor necrosis factor alpha by a resonance raman enzyme-linked immunosorbent assay. Anal Chem 2011; 83:297-302; PMID:21121688; http://dx.doi.org/10.1021/ac1024039
- Perumal J, Balasundaram G, Mahyuddin AP, Choolani M, Olivo M. SERS-based quantitative detection of ovarian cancer prognostic factor haptoglobin. Int J Nanomedicine 2015; 10:1831-40; PMID:25834423; http://dx.doi.org/10.2147/IJN.S75552
- Kane MA, Napoli JL. Quantification of endogenous retinoids. Methods Mol Biol 2010; 652:1-54; PMID:20552420; http://dx.doi.org/10.1007/978-1-60327-325-1_1
- Sugii S, Kida Y, Berggren WT, Evans RM. Feeder-dependent and feeder-independent iPS cell derivation from human and mouse adipose stem cells. Nat Protoc 2011; 6:346-58; PMID:21372815; http://dx.doi.org/10.1038/nprot.2010.199
- Sugii S, Kida Y, Kawamura T, Suzuki J, Vassena R, Yin YQ, Lutz MK, Berggren WT, Izpisua Belmonte JC, Evans RM. Human and mouse adipose-derived cells support feeder-independent induction of pluripotent stem cells. Proc Natl Acad Sci U S A 2010; 107:3558-63; PMID:20133714; http://dx.doi.org/10.1073/pnas.0910172106