ABSTRACT
The regulation of adipose tissue expansion by adipocyte hypertrophy and/or hyperplasia is the topic of extensive investigations given the potential differential contribution of the 2 processes to the development of numerous chronic diseases associated with obesity. We recently discovered that the loss-of-function of the Src homology domain-containing protein Nck2 in mice promotes adiposity accompanied with adipocyte hypertrophy and impaired function, and enhanced adipocyte differentiation in vitro. Moreover, in severely-obese human's adipose tissue, we found that Nck2 expression is markedly downregulated. In this commentary, our goal is to expand upon additional findings providing further evidence for a unique Nck2-dependent mechanism regulating adipogenesis. We propose that Nck2 should be further investigated as a regulator of the reliance of white adipose tissue on hyperplasia versus hypertrophy during adipose tissue expansion, and hence, as a potential novel molecular target in obesity.
Introduction
Obesity is characterized by an expansion of white adipose tissue that relies on a combination of increases in either adipocyte cell size (hypertrophy) and/or adipocyte number (hyperplasia).Citation1 In the latter process, new adipocytes are generated from in situ differentiation of existing precursor cells through a process defined as adipogenesis. In obesity, the clinical impact of adipogenesis dysregulation seems complex. While enhanced differentiation of adipocyte precursors could contribute to adipose tissue expansion and thus to the pathogenesis of obesity, hyperplastic adipose tissue expansion appears to be less metabolically detrimental than hypertrophic expansion. Indeed, hypertrophied adipocytes have been associated with metabolic disorders due to their ability to constitutively release higher levels of fatty acids, adipokines and proinflammatory cytokines.Citation2,3 These, jointly, contribute to ectopic lipids deposition and to insulin resistance in peripheral tissues, resulting in increased prevalence of obesity-related type 2 diabetes that is observed beyond a certain adipocyte diameter threshold.Citation4 Hence, while contributing to adipose tissue mass, overcoming an “adipogenesis blockade” may in fact have protective metabolic outcomes, as therapeutically highlighted by the thiazolidinediones (TZD's).
Adipogenesis
Adipogenesis is a complex process involving coordinated temporal and spatial control of a precise transcriptional network regulating adipocyte-specific gene expression. Members of the CCAAT/enhancer-binding proteins (C/EBPs) family of transcription factors serve as master regulators in initiating the differentiation transcriptional network. Indeed, C/EBPβ and C/EBPδ are expressed early following the induction of adipogenesis. As a result, the C/EBPα and peroxisome proliferator-activated receptor γ (PPARγ) axis is activated and these function together as late-acting master transcriptional activators of most genes defining the terminal adipocyte phenotype.Citation5,6 Although C/EBPα and PPARγ seem to cooperatively induce transcriptional activation of adipogenic genes by occupying common transcription factor hotspots,Citation7 PPARγ appears to be a dominant actor in promoting adipocyte differentiation. Indeed, PPARγ ectopic expression was reported to restore adipogenesis in mouse embryonic fibroblasts lacking C/EBPα,Citation8 while the latter failed to compensate for the lack of PPARγ.Citation9 Important progress was made during the past decades in identifying the importance and the role of PPARγ in mediating transcriptional regulation of adipocyte differentiation, including the identification of TZD's as pharmacological PPARγ ligands. Yet, specific temporal dynamics involving various protein-protein and protein-DNA interactions regulating transcriptional activity of PPARγ during adipogenesis still remain largely unknown.
PPARγ regulation
As an essential factor for adipocyte differentiation, PPARγ has been implicated in multiple steps during the entire differentiation process. Indeed, PPARγ contributes to growth arrest following mitotic clonal expansion in early steps of adipocyte differentiation by inhibiting E2F/DP DNA binding activity,Citation10 induction of p21,Citation11 or cyclin D1 expression.Citation12 In addition, PPARγ is a direct transcriptional activator of most specific genes encoding critical proteins regulating adipogenesis and adipocyte function. Among them, aP2 (FABP4), which is required for transport of free fatty acids, perilipin (PLIN1), which covers the surface of mature lipid droplets in adipocytes and regulates lipolysis, and lipoprotein lipase (LPL), adiponectin (ADIPOQ), CD36 (CD36), glycerol-3-phosphate dehydrogenase (GPD1) and insulin-responsive glucose transporter 4 (SLC2A4), all involved in regulating lipid and glucose metabolism.Citation13,14 Playing a “master regulator” role, the transcriptional activity of PPARγ is highly regulated during the adipogenic program by post-translational modifications. Indeed, certain growth factors, cytokines and stress conditions repress PPARγ's transcriptional activity and impair adipogenesis by inducing the phosphorylation of PPARγ on Ser112 via mitogen-activated protein kinases (MAPK)/ extracellular-signal regulated kinases 1 and 2 (ERK1/2),Citation15 or the stress-activated MAPKs JNK and p38.Citation16,17 In contrast, PPARγ phosphorylation on the same site by the cyclin-dependent kinases Cdk7 and Cdk9 promotes PPARγ activity, further illustrating the complexity of the regulation of PPARγ by phosphorylation. Beyond phosphorylation, PPARγ's transcriptional activity in white adipose tissue may also be repressed by sumoylation,Citation18 ubiquitinationCitation19 and nitrosylation.Citation20 Another means of PPARγ regulation involves its association, under either unliganded or liganded states, with proteins that behave as coactivators such as PGC-1αCitation21 or corepressors such as N-Cor and SMRT.Citation22 Finally, the subcellular localization of PPARγ clearly determines its transcriptional activity, but surprisingly, little is known about the molecular mechanisms regulating its nuclear-cytoplasmic shuttling. Growth factors or cytokines stimulation exported PPARγ from the nucleus toward the cytosol by direct interaction with the Mitogen-Activated Protein Kinase 1 (MEK1),Citation23 an upstream activator of ERK1/2 that was already mentioned to negatively regulate PPARγ through Ser112 phosphorylation.Citation15 In contrast, PPARγ nuclear localization was facilitated by its interaction with nocturnin, a protein encoded by one of the circadian clock genes,Citation24 further illustrating the importance of protein binding partners in regulating PPARγ nuclear-cytoplasmic transport and biologic function.
A critical role for Nck2 in regulating adipogenesis
In a recent study we identified the Src homology (SH) domain-containing protein Nck2 (non catalytic region of tyrosine kinase 2) as a novel regulator of adipogenesis in vitro, and demonstrated that its absence in mice contributes to the development of hypertrophic adiposity and metabolic syndrome.Citation25 In addition, we uncovered that among severely-obese humans, Nck2 expression is downregulated in visceral white adipose tissue,Citation25 while it is upregulated during differentiation of human Simpson-Golabi-Behmel syndrome (SGBS) preadipocytes (). These findings jointly suggest that Nck2 may regulate the relative contribution of hypertrophy and hyperplasia to white adipose tissue expansion in mice and humans. Nck2 is a member of a family of adaptor proteins, which also includes Nck1. These 47 kDa cytosolic proteins, although encoded by different genes, share high degree of amino acids identity. Both Nck1 and 2 are devoid of catalytic activity, and are composed of 3 N-terminal SH3 domains and a unique C-terminal SH2 domain. Through their highly conserved SH domains, Nck1 and 2 function as scaffolds that facilitate assembly of molecular complexes linking signaling from cell surface and endoplasmic reticulum (ER) localized transmembrane receptors to crucial cellular processes, such as proliferation, cytoskeletal reorganization and stress response.Citation26-28 Within this context, we identified a major role for Nck in regulating signaling from the ER through direct interaction with IRE1-α (Inositol-required enzyme 1-α) and PERK (double-stranded RNA-like ER kinase),Citation28-32 2 fundamental components of the unfolded protein response (UPR), whose dysregulation has been implicated in the development of obesity.Citation33 It is noteworthy that obesity creates ER stress inducing sustained activation of the UPR in liver and adipose tissue,Citation34,35 potentially through increased production of reactive oxygen speciesCitation35 and impaired Ca2+ homeostasis.Citation36 In obesity, ER stress-induced activation of the UPR is clearly associated with dysregulated function of the adipose tissue by altering insulin sensitivity, cytokines and adipokines secretion,Citation37,38 but also downregulation of C/EBPα and PPARγ.Citation39 Paradoxically, transient activation of IRE1α and PERK play an important role in normal adipocyte development: The spliced form of XBP1 (XBP1s), generated by activated IRE1α RNAse activity, has been reported to promote C/EBPα expression through direct binding and activation of the Cebpa promoter.Citation40 Furthermore, PERK deficiency impaired expression of lipogenic genes and lipid accumulation.Citation41 During adipocyte differentiation and in adipose tissue, we found that the deficiency of Nck2 results in increased PERK activity and signaling, correlating with higher expression of lipogenic genes.Citation25 PERK activation leads to phosphorylation of the eukaryotic initiation factor 2 α-subunit (peIF2α), which reduces overall rate of translation initiation, but simultaneously upregulates expression of transcription factors such as activating transcription factor 4 (ATF4) and C/EBP homologous protein (CHOP).Citation42,43 Through the peIF2α-ATF4 canonical pathway, PERK critically contributes to fat storage and lipogenesis by inducing lipogenic genes expression during adipocyte differentiation in multiple cell types via ATF4-induced expression of the ER membrane-bound transcription factor sterol element binding 1c (SREBP1c).Citation41,44 However, the exact mechanism by which disrupting Nck2-dependent control of PERK in white adipose tissue, thereby promoting adipocyte hypertrophy and white adipose tissue dysfunction and obesity has not been fully elucidated.
Figure 1. Expression of Nck2 in human SGBS (Simpson-Golabi-Behmel syndrome) cells during adipocyte differentiation. Nck2 levels were evaluated in SGBS cells at different time points during differentiation by western blot using a specific antibody that recognized human Nck2 (OriGene TA321585) and total cell lysate normalized for protein content. HSP90 was used as loading control. Upper panel represents western blot from a typical experiment, while the bottom panel represents quantitation of Nck2 expression normalized over HSP90 compared with day 0 from 3 independent experiments. Data are the mean ± SEM. *statistical significance at least p ≤ 0.05 compared with Day 0 using unpaired Student t-test.
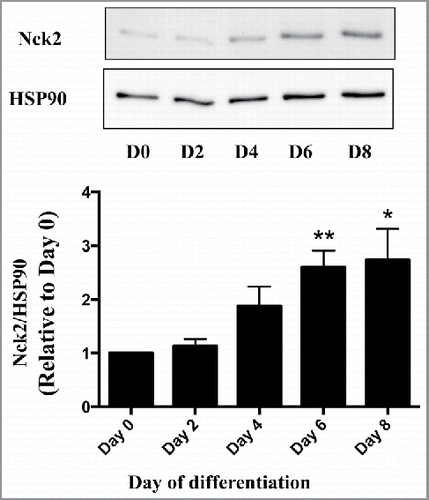
By contrast to loss-of-function of Nck2 in mouse 3T3-L1 preadipocyte, which promotes adipogenesis, gain-of-function of Nck2 strongly impairs differentiation (). This is supported by a significant decrease in the induction of the fatty acid binding protein 4 (Fabp4) mRNA and lipid droplet formation.Citation25 As described above, induction of PPARγ is the primary driver of adipocyte differentiation and Fabp4 expression, resulting from the early induction of the transcription factors C/EBPβ and C/EBPδ during adipogenesis. In Nck2 overexpressing 3T3-L1 preadipocytes subjected to the classical hormonal differentiation protocol, the levels of C/EBPβ and C/EBPα mRNAs are equivalent to the levels found in similarly treated, empty-vector-transfected 3T3-L1 cells (). In agreement, C/EBPα protein levels are also unchanged in response to Nck2 overexpression (), further implying that Nck2 is not involved in regulating early transcriptional events during adipocyte differentiation. However, mRNA and protein levels of PPARγ are unexpectedly increased in Nck2 overexpressing 3T3-L1 cells () in spite of their seemingly impaired differentiation () and decreased expression of classical PPARγ gene targets such as perilipin (). Interestingly, higher expression of PPARγ may represent an adaptive response to lower PPARγ activity that may be in fact ascribed to lower level of Fabp4 mRNA,Citation25 which could potentially results in low level of Fabp4 (aP2), a condition that has been previously reported to mediate a negative feedback loop on PPARγ expression.Citation45 However, Nck2 overexpression hampered PPARγ nuclear translocation, as revealed by immunofluorescence (), strongly suggesting a novel mechanism by which Nck2 may limit adipogenesis. In this perspective, the possibility that the SH domain-containing adaptor protein Nck2 may provide a fine control of adipogenesis via directly or indirectly controlling PPARγ cytoplasmic retention, is an interesting avenue that remains to be defined (). Potentially, Nck2 might regulate PPARγ nuclear-cytoplasmic distribution by directly interacting with, and neutralizing, the adaptor protein downstream of tyrosine kinase 1 (Dok1).Citation46 This protein was shown to mediate insulin signaling and promote adipogenesis by counteracting the inhibitory effect of ERK1/2 on PPARγ transcriptional activity.Citation47 Alternatively, given our observation that overexpression of Nck2 limits PERK activation and signaling,Citation28,31 which is required for adipogenesis to occur,Citation48 impaired adipocyte differentiation in 3T3-L1 overexpressing Nck2 might result from attenuated PERK activation and signaling. A detailed investigation of the role of Nck2 is required to understand its function during adipogenesis and to determine whether like for loss-of-function, Nck2 gain-of-function effects on adipogenesis involve Nck2-dependent regulation of PERK activation and signaling during adipocyte differentiation.
Figure 2. Overexpression of Nck2 in murine 3T3-L1 preadipocytes. A) Equivalent amount of proteins from total cell lysates of 3T3-L1 preadipocytes stably transfected with pcDNA3.1 or pcDNA3.1 encoding Flag-Nck2 were probed with anti-Flag antibody to detect Flag-Nck2 or RasGap antibody as loading control. B) Phase-contrast images (10X) of 3T3-L1 preadipocytes control or overexpressing Nck2 at day 0, 6 and 20 of differentiation. C) Cebpa and Cebpb mRNA levels determined using qPCR in control and Nck2 overexpressing 3T3-L1 cells at day 15 of differentiation. Shown is the mean ± SEM of 2 experiments performed in triplicate. D) Equivalent amount of proteins from total cell lysates of control and Nck2 overexpressing 3T3-L1 cells at day 15 of differentiation were subjected to western blot analyses using indicated antibodies. Shown is a typical experiment performed in duplicate. E) Pparg mRNA levels determined using qPCR in indicated 3T3-L1 cells at 15 d of differentiation. Shown is the mean ± SEM of 4 independent experiments. **, statistical significant at p ≤ 0.01 using unpaired Student t-test.
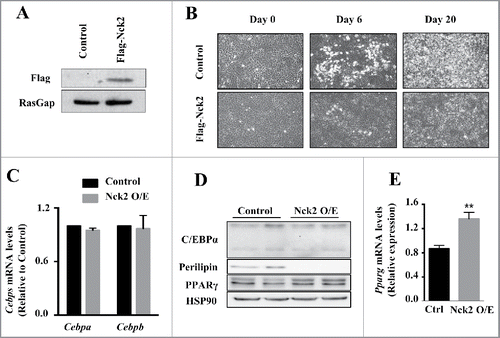
Figure 3. PPARγ cellular distribution in induced control and Nck2 overexpressing 3T3-L1 cells. A) DIC and fluorescent images of control and Nck2 overexpressing 3T3-L1 cells induced for differentiation under the same set up were used to investigate PPARγ nuclear localization. DIC: light microscopy images at 40X; Blue: nuclear staining with Dapi; Red: PPARγ immunofluorescence using a specific PPARγ antibody and a secondary antibody-coupled to Alexa Fluor® 594; Merge: superposition of Dapi and PPARγ signals. B) Model of adipogenesis regulation by Nck2. In response to adipogenic cues, loss-of-function of Nck2 promotes adipogenesis by enhancing PPARγ nuclear translocation, while Nck2 gain-of-function promotes PPARγ expression, but directly or indirectly, represses PPAR γ nuclear translocation.
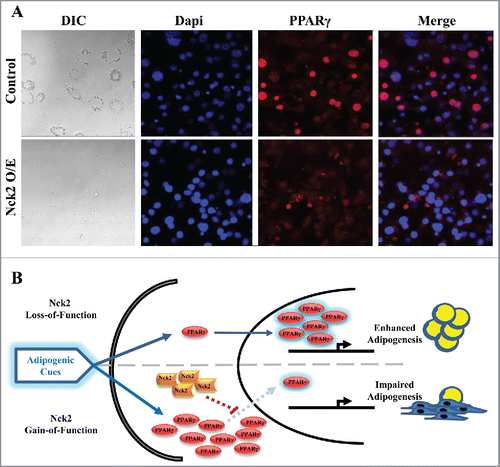
Conclusions and key outstanding questions
Existing pharmacological approaches to promote healthy white adipose tissue and weight loss in obese humans are still limited and accompanied by major secondary effects that dampened their clinical potential. A better understanding of how the SH domain-containing adaptor protein Nck2 regulates white adipose tissue homeostasis may provide a unique opportunity to highlight novel ways to overcome obesity and inspire effective pharmacological strategies to prevent obesity-related chronic disorders. To pursue this objective, additional investigation to further decode the role of Nck2 in adipocyte differentiation and function should address whether a direct protein-protein interaction relationship exists between Nck2 and PPARγ in their endogenous expression levels. Next, it would be important to decipher if manipulating the expression of Nck2 represents a potential avenue to take advantage of the beneficial aspect of adipocyte hyperplasia vs hypertrophy in obesity. Finally, in mature adipocytes, it is worthwhile to elucidate if Nck2 is critical for maintaining the mature adipocyte differentiation state, keeping it fully functional. Providing answers to these questions may be a unique opportunity to elucidate the intricate mechanisms linking WAT biology with obesity and related diseases.
Disclosure of potential conflict of interest
No potential conflicts of interest were disclosed.
Funding
This work was funded by the Canadian Institutes for Health Research (CIHR, MOP-115045) grant to L.L. and by a grant from the Zavalkoff Foundation to A.R. and L.L. N.H. was supported by a student fellowship from the McGill University Health Center Research Institute (MUHC-RI).
References
- Brook CG, Lloyd JK, Wolf OH. Relation between age of onset of obesity and size and number of adipose cells. Br Med J 1972; 2(5804):25-7; PMID:5015967; https://doi.org/10.1136/bmj.2.5804.25
- Wueest S, Rapold RA, Rytka JM, Schoenle EJ, Konrad D. Basal lipolysis, not the degree of insulin resistance, differentiates large from small isolated adipocytes in high-fat fed mice. Diabetologia 2009; 52(3):541-6; PMID:19048227; https://doi.org/10.1007/s00125-008-1223-5
- Skurk T, Alberti-Huber C, Herder C, Hauner H. Relationship between adipocyte size and adipokine expression and secretion. J Clin Endocrinol Metab 2007; 92(3):1023-33; PMID:17164304; https://doi.org/10.1210/jc.2006-1055
- Cotillard A, Poitou C, Torcivia A, Bouillot JL, Dietrich A, Kloting N, Grégoire C, Lolmede K, Blüher M, Clément K. Adipocyte size threshold matters: link with risk of type 2 diabetes and improved insulin resistance after gastric bypass. J Clin Endocrinol Metab 2014; 99(8):E1466-70; PMID:24780048; https://doi.org/10.1210/jc.2014-1074
- Rosen ED, MacDougald OA. Adipocyte differentiation from the inside out. Nat Rev Mol Cell Biol 2006; 7(12):885-96; PMID:17139329; https://doi.org/10.1038/nrm2066
- Tang QQ, Lane MD. Adipogenesis: from stem cell to adipocyte. Annu Rev Biochem 2012; 81:715-36; PMID:22463691; https://doi.org/10.1146/annurev-biochem-052110-115718
- Lefterova MI, Zhang Y, Steger DJ, Schupp M, Schug J, Cristancho A, Feng D, Zhuo D, Stoeckert CJ Jr, Liu XS, et al. PPARgamma and C/EBP factors orchestrate adipocyte biology via adjacent binding on a genome-wide scale. Genes Dev 2008; 22(21):2941-52; PMID:18981473; https://doi.org/10.1101/gad.1709008
- Wu Z, Rosen ED, Brun R, Hauser S, Adelmant G, Troy AE, McKeon C, Darlington GJ, Spiegelman BM. Cross-regulation of C/EBP alpha and PPAR gamma controls the transcriptional pathway of adipogenesis and insulin sensitivity. Mol Cell 1999; 3(2):151-8; PMID:10078198; https://doi.org/10.1016/S1097-2765(00)80306-8
- Rosen ED, Hsu CH, Wang X, Sakai S, Freeman MW, Gonzalez FJ, Spiegelman BM. C/EBPalpha induces adipogenesis through PPARgamma: a unified pathway. Genes Dev 2002; 16(1):22-6; PMID:11782441; https://doi.org/10.1101/gad.948702
- Altiok S, Xu M, Spiegelman BM. PPARgamma induces cell cycle withdrawal: inhibition of E2F/DP DNA-binding activity via down-regulation of PP2A. Genes Dev 1997; 11(15):1987-98; PMID:9271121; https://doi.org/10.1101/gad.11.15.1987
- Morrison RF, Farmer SR. Role of PPARgamma in regulating a cascade expression of cyclin-dependent kinase inhibitors, p18(INK4c) and p21(Waf1/Cip1), during adipogenesis. J Biol Chem 1999; 274(24):17088-97. Epub 1999/06/08; PMID:10358062; https://doi.org/10.1074/jbc.274.24.17088
- Wang C, Fu M, D'Amico M, Albanese C, Zhou JN, Brownlee M, Lisanti MP, Chatterjee VK, Lazar MA, Pestell RG. Inhibition of cellular proliferation through IkappaB kinase-independent and peroxisome proliferator-activated receptor gamma-dependent repression of cyclin D1. Mol Cell Biol 2001; 21(9):3057-70. Epub 2001/04/05; PMID:11287611; https://doi.org/10.1128/MCB.21.9.3057-3070.2001
- Rosen ED, Sarraf P, Troy AE, Bradwin G, Moore K, Milstone DS, Spiegelman BM, Mortensen RM. PPAR gamma is required for the differentiation of adipose tissue in vivo and in vitro. Mol Cell 1999; 4(4):611-7; PMID:10549292; https://doi.org/10.1016/S1097-2765(00)80211-7
- Siersbæk R, Nielsen R, Mandrup S. PPARγ in adipocyte differentiation and metabolism– Novel insights from genome-wide studies. FEBS Lett 2010; 584(15):3242-9; PMID:20542036; https://doi.org/10.1016/j.febslet.2010.06.010
- Hu E, Kim JB, Sarraf P, Spiegelman BM. Inhibition of adipogenesis through MAP kinase-mediated phosphorylation of PPARgamma. Science 1996; 274(5295):2100-3; PMID:8953045; https://doi.org/10.1126/science.274.5295.2100
- Adams M, Reginato MJ, Shao D, Lazar MA, Chatterjee VK. Transcriptional activation by peroxisome proliferator-activated receptor gamma is inhibited by phosphorylation at a consensus mitogen-activated protein kinase site. J Biol Chem 1997; 272(8):5128-32; PMID:9030579; https://doi.org/10.1074/jbc.272.8.5128
- Camp HS, Tafuri SR, Leff T. c-Jun N-terminal kinase phosphorylates peroxisome proliferator-activated receptor-gamma1 and negatively regulates its transcriptional activity. Endocrinology 1999; 140(1):392-7; PMID:9886850
- Pourcet B, Pineda-Torra I, Derudas B, Staels B, Glineur C. SUMOylation of human peroxisome proliferator-activated receptor alpha inhibits its trans-activity through the recruitment of the nuclear corepressor NCoR. J Biol Chem 2010; 285(9):5983-92; PMID:19955185; https://doi.org/10.1074/jbc.M109.078311
- Hauser S, Adelmant G, Sarraf P, Wright HM, Mueller E, Spiegelman BM. Degradation of the peroxisome proliferator-activated receptor gamma is linked to ligand-dependent activation. J Biol Chem 2000; 275(24):18527-33; PMID:10748014; https://doi.org/10.1074/jbc.M001297200
- Cao Y, Gomes SA, Rangel EB, Paulino EC, Fonseca TL, Li J, Teixeira MB, Gouveia CH, Bianco AC, Kapiloff MS, et al. S-nitrosoglutathione reductase-dependent PPARgamma denitrosylation participates in MSC-derived adipogenesis and osteogenesis. J Clin Invest 2015; 125(4):1679-91; PMID:25798618; https://doi.org/10.1172/JCI73780
- Puigserver P, Spiegelman BM. Peroxisome proliferator-activated receptor-gamma coactivator 1 alpha (PGC-1 alpha): transcriptional coactivator and metabolic regulator. Endocr Rev 2003; 24(1):78-90; PMID:12588810; https://doi.org/10.1210/er.2002-0012
- Yu C, Markan K, Temple KA, Deplewski D, Brady MJ, Cohen RN. The nuclear receptor corepressors NCoR and SMRT decrease peroxisome proliferator-activated receptor gamma transcriptional activity and repress 3T3-L1 adipogenesis. J Biol Chem 2005; 280(14):13600-5. Epub 2005/02/05; PMID:15691842; https://doi.org/10.1074/jbc.M409468200
- Burgermeister E, Chuderland D, Hanoch T, Meyer M, Liscovitch M, Seger R. Interaction with MEK causes nuclear export and downregulation of peroxisome proliferator-activated receptor gamma. Mol Cell Biol 2007; 27(3):803-17; PMID:17101779; https://doi.org/10.1128/MCB.00601-06
- Kawai M, Green CB, Lecka-Czernik B, Douris N, Gilbert MR, Kojima S, Ackert-Bicknell C, Garg N, Horowitz MC, Adamo ML, et al. A circadian-regulated gene, Nocturnin, promotes adipogenesis by stimulating PPAR-gamma nuclear translocation. Proc Natl Acad Sci U S A 2010; 107(23):10508-13; PMID:20498072; https://doi.org/10.1073/pnas.1000788107
- Dusseault J, Li B, Haider N, Goyette MA, Côté JF, Larose L. Nck2 deficiency in mice results in increased adiposity associated with adipocyte hypertrophy and enhanced adipogenesis. Diabetes 2016; 65(September):2652-66. Epub June 20, 2016.
- Braverman LE, Quilliam LA. Identification of Grb4/Nck beta, a src homology 2 and 3 domain-containing adapter protein having similar binding and biological properties to Nck. J Biol Chem 1999; 274:5542-9; PMID:10026169; https://doi.org/10.2337/db15-1559
- Jones N, Blasutig IM, Eremina V, Ruston JM, Bladt F, Li H, Huang H, Larose L, Li SS, Takano T, et al. Nck adaptor proteins link nephrin to the actin cytoskeleton of kidney podocytes. Nature 2006; 440(7085):818-23; PMID:16525419; https://doi.org/10.1038/nature04662
- Latreille M, Larose L. Nck in a complex containing the catalytic subunit of protein phosphatase 1 regulates eukaryotic initiation factor 2alpha signaling and cell survival to endoplasmic reticulum stress. J Biol Chem 2006; 281(36):26633-44; PMID:16835242; https://doi.org/10.1074/jbc.M513556200
- Ngyuen DT, Kebache S, Fazel A, Wong HN, Jenna S, Emaldali A, Lee EH, Bergeron JJ, Kaufman RJ, Larose L, et al. Nck-dependent Activation of Extracellular-regulated Kinase-1 and Regulation of Cell Survival during Endoplasmic Reticulum Stress. Mol Biol Cell 2004; 15:4248-60; PMID:15201339; https://doi.org/10.1091/mbc.E03-11-0851
- Latreille M, Laberge MK, Bourret G, Yamani L, Larose L. Deletion of Nck1 attenuates hepatic ER stress signaling and improves glucose tolerance and insulin signaling in liver of obese mice. Am J Physiol Endocrinol Metab 2011; 300(3):E423-34. Epub 2010/07/01; PMID:20587749; https://doi.org/10.1152/ajpendo.00088.2010
- Yamani L, Latreille M, Larose L. Interaction of Nck1 and PERK phosphorylated at Y(5)(6)(1) negatively modulates PERK activity and PERK regulation of pancreatic beta-cell proinsulin content. Mol Biol Cell 2014; 25(5):702-11; PMID:24371088; https://doi.org/10.1091/mbc.E13-09-0511
- Yamani L, Li B, Larose L. Nck1 deficiency improves pancreatic beta cell survival to diabetes-relevant stresses by modulating PERK activation and signaling. Cell Signal 2015; 27(12):2555-67; PMID:26434994; https://doi.org/10.1016/j.cellsig.2015.09.016
- Lee J, Ozcan U. Unfolded Protein Response Signaling and Metabolic Diseases. J Biol Chem 2014; 289(3):1203-11; PMID:24324257; https://doi.org/10.1074/jbc.R113.534743
- Ozcan U, Cao Q, Yilmaz E, Lee AH, Iwakoshi NN, Ozdelen E, Tuncman G, Görgün C, Glimcher LH, Hotamisligil GS. Endoplasmic reticulum stress links obesity, insulin action, and type 2 diabetes. Science 2004; 306(5695):457-61; PMID:15486293; https://doi.org/10.1126/science.1103160
- Cullinan SB, Diehl JA. Coordination of ER and oxidative stress signaling: the PERK/Nrf2 signaling pathway. Int J Biochem Cell Biol 2006; 38(3):317-32; PMID:16290097; https://doi.org/10.1016/j.biocel.2005.09.018
- Park SW, Zhou Y, Lee J, Lee J, Ozcan U. Sarco(endo)plasmic reticulum Ca2+-ATPase 2b is a major regulator of endoplasmic reticulum stress and glucose homeostasis in obesity. Proc Natl Acad Sci U S A 2010; 107(45):19320-5; PMID:20974941; https://doi.org/10.1073/pnas.1012044107
- Gregor MF, Hotamisligil GS. Thematic review series: Adipocyte Biology. Adipocyte stress: the endoplasmic reticulum and metabolic disease. J Lipid Res 2007; 48(9):1905-14.
- Gregor MF, Yang L, Fabbrini E, Mohammed BS, Eagon JC, Hotamisligil GS, Klein S. Endoplasmic reticulum stress is reduced in tissues of obese subjects after weight loss. Diabetes 2009; 58(3):693-700. Epub 2008/12/11; PMID:19066313; https://doi.org/10.2337/db08-1220
- Lefterova MI, Mullican SE, Tomaru T, Qatanani M, Schupp M, Lazar MA. Endoplasmic reticulum stress regulates adipocyte resistin expression. Diabetes 2009; 58(8):1879-86; PMID:19491212; https://doi.org/10.2337/db08-1706
- Sha H, He Y, Chen H, Wang C, Zenno A, Shi H, Yang X, Zhang X, Qi L. The IRE1±-XBP1 Pathway of the Unfolded Protein Response Is Required for Adipogenesis. Cell Metab 2009; 9(6):556-64; PMID:19490910; https://doi.org/10.1016/j.cmet.2009.04.009
- Bobrovnikova-Marjon E, Hatzivassiliou G, Grigoriadou C, Romero M, Cavener DR, Thompson CB, Diehl JA. PERK-dependent regulation of lipogenesis during mouse mammary gland development and adipocyte differentiation. Proc Natl Acad Sci U S A 2008; 105(42):16314-9; PMID:18852460; https://doi.org/10.1073/pnas.0808517105
- Kaufman RJ. Orchestrating the unfolded protein response in health and disease. J Clin Invest 2002; 110(10):1389-98; PMID:12438434; https://doi.org/10.1172/JCI0216886
- Schroder M, Kaufman RJ. The Mammalian unfolded protein response. Annu Rev Biochem 2005; 74:739-89; PMID:15952902; https://doi.org/10.1146/annurev.biochem.73.011303.074134
- Yu Y, Pierciey FJ, Jr., Maguire TG, Alwine JC. PKR-like endoplasmic reticulum kinase is necessary for lipogenic activation during HCMV infection. PLoS Pathog 2013; 9(4):e1003266. Epub 2013/04/18; PMID:23592989; https://doi.org/10.1371/journal.ppat.1003266
- Garin-Shkolnik T, Rudich A, Hotamisligil GS, Rubinstein M. FABP4 attenuates PPARgamma and adipogenesis and is inversely correlated with PPARgamma in adipose tissues. Diabetes 2014; 63(3):900-11; PMID:24319114; https://doi.org/10.2337/db13-0436
- Wick MJ, Dong LQ, Hu D, Langlais P, Liu F. Insulin receptor-mediated p62dok tyrosine phosphorylation at residues 362 and 398 plays distinct roles for binding GTPase-activating protein and Nck and is essential for inhibiting insulin-stimulated activation of Ras and Akt. J Biol Chem 2001; 276(46):42843-50; PMID:11551902; https://doi.org/10.1074/jbc.M102116200
- Hosooka T, Noguchi T, Kotani K, Nakamura T, Sakaue H, Inoue H, Ogawa W, Tobimatsu K, Takazawa K, Sakai M, et al. Dok1 mediates high-fat diet-induced adipocyte hypertrophy and obesity through modulation of PPAR-gamma phosphorylation. Nat Med 2008; 14(2):188-93; PMID:18204460; https://doi.org/10.1038/nm1706
- Han J, Murthy R, Wood B, Song B, Wang S, Sun B, Malhi H, Kaufman RJ. ER stress signalling through eIF2alpha and CHOP, but not IRE1alpha, attenuates adipogenesis in mice. Diabetologia 2013; 56(4):911-24. Epub 2013/01/15; PMID:23314846; https://doi.org/10.1007/s00125-012-2809-5