ABSTRACT
Non-communicable diseases (NCDs) such as cardiovascular disease, diabetes and cancer were responsible for 68% of all deaths worldwide in 2012. The regional distribution of lipid deposited within adipose tissue (AT) - so called body fat distribution (BFD) - is a strong risk factor for NCDs. BFD is highly heritable; however, the genetic basis of BFD is almost entirely unknown. Genome-wide association studies have identified several loci associated with BFD, including at Plexin D1 (PLXND1) - a gene known to modulate angiogenesis. We recently demonstrated that zebrafish homozygous for a null mutation in plxnd1 had a reduced capacity to store lipid in visceral AT (VAT) leading to altered BFD. Moreover, we found that type V collagens were upregulated in plxnd1 mutants, and mediated the inhibitory effect of Plxnd1 on VAT growth. These results strengthen evidence that Plxnd1 influences BFD in human populations, and validate zebrafish as a model to study BFD. However, many pertinent questions remain unanswered. Here we outline potential Plxnd1 mechanisms of action in AT, and describe the genetic architecture at human PLXND1 that is associated with BFD and NCD susceptibility.
Adipose tissues (ATs) regulate energy homeostasis by supplying and sequestering energy-dense lipid in response to fluctuations in energy status. As such, AT provides an organism with energetic stability.Citation1 Evolutionarily, the energy insurance provided by AT confers tremendous selective advantages for a population when confronted with diverse physiologic burdens. However, in modern societies - when energy-dense food is readily available, food consumption is high and physical activity is low - excessive lipid deposition within AT can lead to AT dysfunction and systemic metabolic disturbance increasing risk for non-communicable diseases (NCDs) such as cardiovascular disease, diabetes and cancer. In 2012, NCDs accounted for 68% of all deaths worldwide.Citation2,3 Of the 52.8 million deaths globally in 2010, ischemic heart disease and stroke collectively killed 12.9 million (24% of all deaths), 1.3 million deaths were caused by diabetes (2.5%) and 8 million died from cancer (15%). Therefore, understanding factors that influence or predict NCD risk is an important public health challenge.
ATs are highly heterogeneous and deposited in diverse regional locations throughout the body. Regionally distinct ATs have unique molecular and metabolic attributes that influence whole-animal physiology. Accumulation of AT in the upper body (an android BFD) is associated with increased risk for NCDs.Citation4 Whereas accumulation of AT in the lower body, primarily the legs and thighs (a gynoid BFD) protects from NCD risk.Citation4 Android BFD is characterized by increases in visceral AT (VAT, AT within the abdominal cavity) and abdominal subcutaneous AT (SAT, AT between skin and muscle), while gynoid BFD is characterized by increased gluteal and femoral SAT.Citation4 Understanding factors that regulate the diverse patterns of BFD within human populations is likely to provide important new therapeutic interventions for NCDs.
Heritability estimates from twin studies suggest that BFD is under extensive genetic control.Citation5,6 However, the genetic basis of BFD is essentially unknown. Recently, the Genetic Investigation of ANthropometric Traits (GIANT) consortium performed large-scale meta-analyses of genome-wide association studies (GWAS) to identify loci associated with waist-hip ratio (WHR) – a surrogate measure of android and gynoid patterns of BFD.Citation7,8 Intriguingly, GWAS have found WHR-associated loci are independent from more generalized adiposity traits, suggesting that a distinct genetic architecture underlies BFD.Citation9 GWAS provide an unbiased and comprehensive assessment of genetic loci associated with WHR. However, functional characterization of GWAS loci is essential to identify mechanisms influencing BFD and disease susceptibility.
The rs10804591 single nucleotide polymorphism (SNP) identified in Shungin et al. (2015) encodes a C → A base change, at 3q22.1 ∼8 kb upstream of the PLXND1 transcriptional start site ().Citation7 The rs10804591 A allele (effect allele, EA) was associated with increased WHRadjBMI (WHR adjusted for BMI) (P = 2.31 × 10−6), increased susceptibility to type 2 diabetes (P = 1.67 × 10−3), increased fasting glucose (P = 0.048), increased fasting insulin (P = 6.08 × 10−3), increased blood triglycerides (P = 9.37 × 10−4), decreased Adiponectin (P = 7.81 × 10−3), increased risk for coronary artery disease (P = 0.018) and decreased height (P = 2.53 × 10−5). Similar to many of the BFD-associated SNPs, rs10804591 demonstrated a high degree of sexual dimorphism – often exerting a stronger effect in women ().Citation7 Further, the effect in males and females appeared different, with males also exhibiting reductions in both waist and hip circumferences ().Citation7 Gender differences in adiposity are well known, with females having a higher body fat percentage, and greater gluteal-femoral AT relative to males.Citation10 BFD is regulated by sex hormones, as evidenced by redistribution of AT toward an android distribution following menopause.Citation11,12 rs10804591 EA is common, present at a frequency of 28%, 65%, 28%, 78% within African, American, East Asian, and European populations respectively (1000G Phase 1, a 51% frequency in all individuals). Intriguingly, rs10804591 is located within a predicted promoter flank region 5’ of PLXND1 (asterisk in ), suggesting that rs10804591 might regulate PLXND1 expression. However, the mechanism of rs10804591 action is completely unknown. Importantly, within individuals of European ancestry, rs10804591 is also linked to 41 other common SNPs clustered 5’ to PLXND1 (> 0.7 R2 linkage disequilibrium) (), and many of these linked SNPs also reside in predicted regulatory regions (). Searching the Genotype-Tissue Expression (GTEx) Project revealed that the majority of the 41 SNPs at PLXND1 were associated with PLXND1 mRNA changes in whole blood (N = 338). No associations were found with expression changes in VAT (N = 185); however, this is potentially due to lower sample size. The investigation of functional variants at PLXND1 is likely to provide exciting new insights into the genetic underpinnings of BFD.
Figure 1. Common variants and regulatory features at human PLXND1. Four tracks are depicted at the PLXND1 locus. In descending order: WHRadjBMI Variants; 49 variants linked to rs10804591 (European ancestry, 1000G Phase 1, >0.7 R2 in linkage disequilibrium of rs10804591). Genes; Havana annotated genes. Arrows within the first exon indicate direction of transcription. All Variants; common variants from 1000 G Phase 1 with a frequency of at least 1% within populations of European ancestry. Regulatory Features; regulation marks predicted by the Ensembl Regulatory Build.Citation66 Vertical black bars are 100 kb (A) or 10 kb (B) apart. Brackets in A denote the region shown in B.
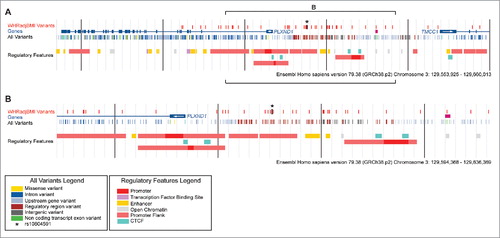
Figure 2. β-coefficients and standard deviation for rs10804591. Bar charts indicate the β-coefficients and standard deviation (SD) for rs10804591 for waist-hip ratio adjusted for BMI (A; WHRadjBMI), waist circumference adjusted for BMI (WCadjBMI), and hip circumference adjusted for BMI (C, HIPadjBMI). All data are taken from Shungin et al. (2015). Asterisks indicate genome-wide significance (P < 5 × 10–8). Data are classified into 3 groups; sex-combined (black bars), female-only (white bars), and male-only (gray bars). Data are from GWAS or metabochip (MC) cohorts as described in Shungin et al. (2015).
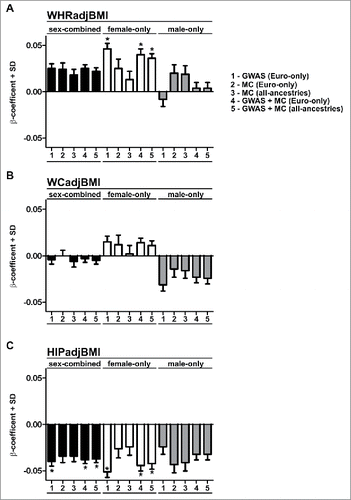
PLXND1 is a multipass transmembrane receptor for a variety of Semaphorin (SEMA) ligands, including SEMA3ECitation13,14 and SEMA4A.Citation15 Binding of SEMA3E/4A to PLXND1 suppresses angiogenesis - the process of new blood vessel formation from existing vessels,Citation13–15 and mutation of Plxnd1 in mouse and zebrafish causes hypervascularization of multiple tissues.Citation16,17 Therefore, PLXND1 is a potent anti-angiogenic molecule. The role of angiogenesis is of particular relevance to AT biology as angiogenesis is known to regulate lipid accumulation in AT,Citation18–20 and stimulation of angiogenesis specifically in AT can normalize metabolic disturbances present in obesity.Citation20,21 Furthermore, depot-specific angiogenesis has been linked to systemic insulin resistance – a precursor to diabetes,Citation22 suggesting that depot-specific differences in angiogenesis may underlie regional AT expansion and NCD progression. Further, we found that PLXND1 mRNA was positively associated with hypertrophic morphology in VAT, and was increased in obese type 2 diabetics relative to lean and healthy obese subjects.Citation23
Prior to analysis of human PLXND1, we turned to zebrafish as a tractable in vivo model system to functionally evaluate the role of PlxnD1 on BFD. Zebrafish possess AT that is morphologically, molecularly, and functionally homologous to mammalian white AT.Citation23–30 Further the molecular mechanisms governing AT dynamics seems conserved from zebrafish to mammals, as suggested by modulators of nuclear receptors exerting similar effects.Citation31 Fluorescent lipophilic dyes such as Nile Red and BODIPY can be used to visualize and quantify regional AT in live zebrafish (). Analysis of zebrafish homozygous for the functionally null plxnd1 allele, fov01b, revealed an altered BFD, characterized by reduced VATCitation23 (both pancreatic and abdominal VAT deposits) [30]. On closer inspection we found plxnd1 mutant VAT was in a hyperplastic and hyperproliferative state, with an induction of type V collagens in vascular endothelial cells and altered extracellular matrix (ECM) composition.Citation23 Maintenance of the hyperplastic/hyperproliferative state was dependent on collagen type V α 1 (col5a1) and conferred resistance to VAT expansion coupled with improved glucose tolerance after exposure to a high-fat diet.Citation23 These data suggest that the ECM microenvironment can determine the proliferative capacity and growth of VAT, and that vascular endothelial cell-derived Plxnd1 modulates the VAT ECM microenvironment in part through Col5a1 (). Regarding this mechanism, here we discuss a potential Integrin-mediated pathway by which PlxnD1 may regulate ECM composition.
Figure 3. Fluorescent lipophilic dyes to study body fat distribution in zebrafish. Nile Red stained zebrafish demonstrating neutral lipid stored within ATs (labeled in yellow) at 2 developmental stages. The arrows indicate VAT. SL = standard length (a measure of the fish length from the snout to the caudal peduncle).
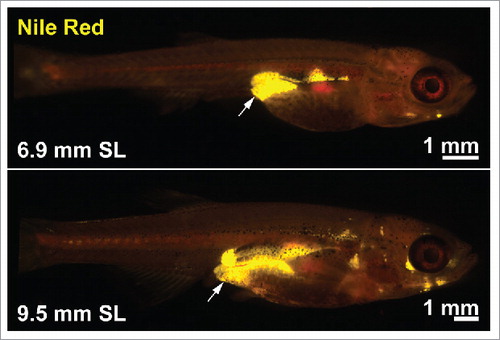
Figure 4. Schematic illustrating the hypothesized mechanism by which vascular endothelial cell-derived Plxnd1 determines ECM composition and VAT expandability. A. Overview of the hyperproliferative and hyperplastic microenvironment of plxnd1 mutant zebrafish VAT. B. Schematic on the interaction between PlxnD1, Integrins and ECM composition.
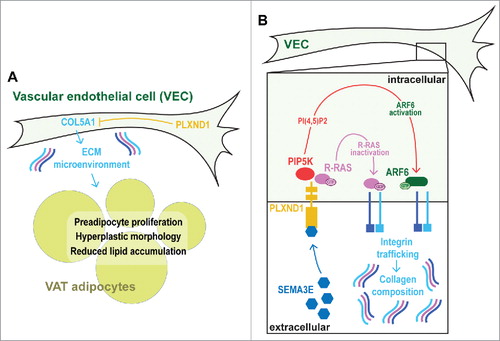
Integrins are heterodimeric collagen receptors that mediate cross-talk between the cell cytoplasm and extracellular ECM.Citation32 The Integrin family of genes comprises 18 distinct α subunits and 8 β subunits, which can dimerize to produce 24 heterodimeric combinations (the Integrin code). Integrin expression can be regulated transcriptionally,Citation33–37 post-transcriptionally by miRNAs,Citation38 and also at the post-translational level. For example, Integrins can form an inactive ‘closed confirmation’ with low affinity for extracellular ligands, or an active ‘open confirmation’ with high affinity for ligands. Regulation of these states has been well studied, with multiple regulators identified (e.g., SHARPIN and SHANK).Citation39,40 Although how this form of Integrin regulation impacts adipose tissue is currently unknown. Intriguingly, the metabolic sensor, AMP-activated protein kinase (AMPK), was recently also identified as a regulator of β1 Integrin activity.Citation41 However, a role for AMPK in regulating adipose ECM and growth is also unknown. Distinct Integrin heterodimers possess different ECM-binding potentials,Citation42 and regulate ECM abundance and composition by modulating collagen synthesis and turnover.Citation43,44 Therefore, we hypothesize that PlxnD1 modulates the collagen composition of VAT by regulating the Integrin code displayed on the vascular endothelial cell-surface. It is known that Integrin expression changes during adipocyte differentiation,Citation45 and that overexpression of Integrin α5 in preadipocytes leads to enhanced proliferation and attenuated differentiation.Citation45 GTPases hydrolyze guanosine triphosphate (GTP), and the GTPase, Rac, is normally downregulated during adipocyte differentiation.Citation45 Overexpression of Integrin α5 increases Rac activity, suggesting that GTPase levels are critical for preadipocyte proliferation and differentiation.Citation45,46 In support, Focal Adhesion Kinase (FAK) plays a central role in Integrin signaling and is essential for adipose expansion.Citation47,48 GTPases control many cell functions, including deposition and maintenance of Integrins on the vascular endothelial cell surface.Citation49–53 Plexin receptors are well known to regulate GTPase activity via their intracellular GTPase activating-protein (GAP) domain,Citation54 and recent studies demonstrated that binding of SEMA3E to PLXND1 in Human Umbilical Vein Endothelial Cells (HUVECs), inactivated the GTPase activity of R-Ras.Citation14 Work from the same laboratory further found that PLXND1 stimulated ARF6 GTPase activity by local production of phosphatidylinositol 4,5-biphosphate (PI(4,5)P2) by type I phosphatidylinositol-4-phosphate-5-kinase (PIP5K) β.Citation55 Both of these pathways acted to modulate Integrin presentation on the HUVEC surface.Citation56 The role of Integrins has not been fully elucidated in AT.Citation45,57 Further, the role of endothelial cell-localized Integrins on AT formation and growth appears essentially unstudied. However, based on the established mechanisms described above, we speculate that Integrin composition on the surface of VAT endothelial cells may play an important role in PlxnD1-mediated regulation of BFD.
To test this hypothesis it will be necessary to manipulate the Integrin code on vascular endothelial cells and assess effects on ECM and VAT growth. Such experiments may be conducted by using the Tie2-CreTg (Tek-Cre) transgenic mouse lineCitation58 to produce vascular endothelial cell-specific Integrin knockouts. Similar experiments have been performed previously to assess an endothelial cell-specific role for β1 Integrins.Citation59–62 As we hypothesize that β1 Integrins mediate crosstalk between VAT endothelial cells and the ECM to regulate VAT growth,Citation55 it will be essential to temporally control Cre-mediated recombination due to embryonic defects in β1 Integrin knockout mice by using inducible Cre lines.Citation59 Although the conditional knockout strategy described above allows the ablation of Integrins to be restricted to endothelial cells, and further controlled by using inducible Cre lines, it would also be desirable to restrict Integrin ablation to endothelial cells specifically within VAT. However, to our knowledge no such transgenic line currently exists that expresses solely in VAT endothelial cells. Therefore, such an experimental strategy will induce Integrin knockout in endothelial cells across the body, potentially leading to secondary effects on VAT growth. Molecular profiles of tissue-specific endothelial cells has been performed for a variety of tissue types,Citation63 therefore a similar strategy in adipose tissues may yield adipose-specific endothelial cell profiles that may be used for transgenic strategies. However, to circumvent secondary effects, endothelial cell and adipocyte co-cultures may also need to be performed.Citation64,65
Disclosure of potential conflicts of interest
No potential conflicts of interest were disclosed.
Funding
This work was supported by American Heart Association Postdoctoral Fellowships (11POST7360004 and 13POST16930097), a University of Edinburgh and British Heart Foundation Fellowship, and a Diabetes UK grant (16/0005494) to JENM; and grants from NIH (R56-DK091356, R21-ES023369, R01-DK093399), and a Pilot Research Project Award from the University Cancer Research Fund at UNC Chapel Hill to JFR.
References
- Wells JC. The evolution of human adiposity and obesity: where did it all go wrong? Dis Model Mech. 2012;5(5):595-607. doi:10.1242/dmm.009613. PMID:22915021
- Gostin LO. Non-communicable diseases: healthy living needs global governance. Nature. 2014;511(7508):147-9. doi:10.1038/511147a. PMID:25013878
- Lozano R, Naghavi M, Foreman K, Lim S, Shibuya K, Aboyans V, Abraham J, Adair T, Aggarwal R, Ahn SY, et al. Global and regional mortality from 235 causes of death for 20 age groups in 1990 and 2010: a systematic analysis for the Global Burden of Disease Study 2010. Lancet. 2012;380(9859):2095-128. doi:10.1016/S0140-6736(12)61728-0. PMID:23245604
- Karpe F, Pinnick KE. Biology of upper-body and lower-body adipose tissue–link to whole-body phenotypes. Nat Rev Endocrinol. 2015;11(2):90-100. doi:10.1038/nrendo.2014.185. PMID:25365922
- Malis C, Rasmussen EL, Poulsen P, Petersen I, Christensen K, Beck-Nielsen H, Astrup A, Vaag AA. Total and regional fat distribution is strongly influenced by genetic factors in young and elderly twins. Obes Res. 2005;13(12):2139-45. doi:10.1038/oby.2005.265. PMID:16421348
- Cardon LR, Carmelli D, Fabsitz RR, Reed T. Genetic and environmental correlations between obesity and body fat distribution in adult male twins. Hum Biol 1994;66(3):465-79. PMID:8026816
- Shungin D, Winkler TW, Croteau-Chonka DC, Ferreira T, Locke AE, Mägi R, Strawbridge RJ, Pers TH, Fischer K, Justice AE, et al. New genetic loci link adipose and insulin biology to body fat distribution. Nature. 2015;518(7538):187-96. doi:10.1038/nature14132. PMID:25673412
- Heid IM, Jackson AU, Randall JC, Winkler TW, Qi L, Steinthorsdottir V, Thorleifsson G, Zillikens MC, Speliotes EK, Mägi R, et al. Meta-analysis identifies 13 new loci associated with waist-hip ratio and reveals sexual dimorphism in the genetic basis of fat distribution. Nat Genet. 2010;42(11):949-60. doi:10.1038/ng.685. PMID:20935629
- Loos RJ. Genetic determinants of common obesity and their value in prediction. Best Pract Res Clin Endocrinol Metab. 2012;26(2):211-26. doi:10.1016/j.beem.2011.11.003. PMID:22498250
- Blaak E. Gender differences in fat metabolism. Curr Opin Clin Nutr Metab Care. 2001;4(6):499-502. doi:10.1097/00075197-200111000-00006. PMID:11706283
- Ley CJ, Lees B, Stevenson JC. Sex- and menopause-associated changes in body-fat distribution. Am J Clin Nutr. 1992;55(5):950-4. PMID:1570802
- Svendsen OL, Hassager C, Christiansen C. Age- and menopause-associated variations in body composition and fat distribution in healthy women as measured by dual-energy X-ray absorptiometry. Metabolism. 1995;44(3):369-73. doi:10.1016/0026-0495(95)90168-X. PMID:7885283
- Gu C, Yoshida Y, Livet J, Reimert DV, Mann F, Merte J, Henderson CE, Jessell TM, Kolodkin AL, Ginty DD. Semaphorin 3E and plexin-D1 control vascular pattern independently of neuropilins. Science. 2005;307(5707):265-8. doi:10.1126/science.1105416. PMID:15550623
- Sakurai A, Gavard J, Annas-Linhares Y, Basile JR, Amornphimoltham P, Palmby TR, Yagi H, Zhang F, Randazzo PA, Li X, et al. Semaphorin 3E initiates antiangiogenic signaling through plexin D1 by regulating Arf6 and R-Ras. Mol Cell Biol. 2010;30(12):3086-98. doi:10.1128/MCB.01652-09. PMID:20385769
- Toyofuku T, Yabuki M, Kamei J, Kamei M, Makino N, Kumanogoh A, Hori M. Semaphorin-4A, an activator for T-cell-mediated immunity, suppresses angiogenesis via Plexin-D1. EMBO J. 2007;26(5):1373-84. doi:10.1038/sj.emboj.7601589. PMID:17318185
- Gitler AD, Lu MM, Epstein JA. PlexinD1 and semaphorin signaling are required in endothelial cells for cardiovascular development. Dev Cell. 2004;7(1):107-16. doi:10.1016/j.devcel.2004.06.002. PMID:15239958
- Torres-Vazquez J, Gitler AD, Fraser SD, Berk JD, Van N Pham, Fishman MC, Childs S, Epstein JA, Weinstein BM. Semaphorin-plexin signaling guides patterning of the developing vasculature. Dev Cell. 2004;7(1):117-23. doi:10.1016/j.devcel.2004.06.008. PMID:15239959
- Rupnick MA, Panigrahy D, Zhang CY, Dallabrida SM, Lowell BB, Langer R, Folkman MJ. Adipose tissue mass can be regulated through the vasculature. Proc Natl Acad Sci U S A. 2002;99(16):10730-5. doi:10.1073/pnas.162349799. PMID:12149466
- Brakenhielm E, Cao R, Gao B, Angelin B, Cannon B, Parini P, Cao Y. Angiogenesis inhibitor, TNP-470, prevents diet-induced and genetic obesity in mice. Circ Res. 2004;94(12):1579-88. doi:10.1161/01.RES.0000132745.76882.70. PMID:15155527
- Sung HK, Doh KO, Son JE, Park JG, Bae Y, Choi S, Nelson SM, Cowling R, Nagy K, Michael IP, et al. Adipose vascular endothelial growth factor regulates metabolic homeostasis through angiogenesis. Cell Metab. 2013;17(1):61-72. doi:10.1016/j.cmet.2012.12.010. PMID:23312284
- Sun K, Wernstedt Asterholm I, Kusminski CM, Bueno AC, Wang ZV, Pollard JW, Brekken RA, Scherer PE. Dichotomous effects of VEGF-A on adipose tissue dysfunction. Proc Natl Acad Sci U S A. 2012;109(15):5874-9. doi:10.1073/pnas.1200447109. PMID:22451920
- Gealekman O, Guseva N, Hartigan C, Apotheker S, Gorgoglione M, Gurav K, Tran KV, Straubhaar J, Nicoloro S, Czech MP, et al. Depot-specific differences and insufficient subcutaneous adipose tissue angiogenesis in human obesity. Circulation. 2011;123(2):186-94. doi:10.1161/CIRCULATIONAHA.110.970145. PMID:21200001
- Minchin JE, Dahlman I, Harvey CJ, Mejhert N, Singh MK, Epstein JA, Arner P, Torres-Vázquez J, Rawls JF. Plexin D1 determines body fat distribution by regulating the type V collagen microenvironment in visceral adipose tissue. Proc Natl Acad Sci U S A. 2015;112(14):4363-8. doi:10.1073/pnas.1416412112. PMID:25831505
- McMenamin SK, Minchin JE, Gordon TN, Rawls JF, Parichy DM. Dwarfism and increased adiposity in the gh1 mutant zebrafish vizzini. Endocrinology. 2013;154(4):1476-87. doi:10.1210/en.2012-1734. PMID:23456361
- Flynn EJ, 3rd, Trent CM, Rawls JF. Ontogeny and nutritional control of adipogenesis in zebrafish (Danio rerio). J Lipid Res. 2009;50(8):1641-52. doi:10.1194/jlr.M800590-JLR200. PMID:19366995
- Imrie D, Sadler KC. White adipose tissue development in zebrafish is regulated by both developmental time and fish size. Dev Dyn. 2010;239(11):3013-23. doi:10.1002/dvdy.22443. PMID:20925116
- Minchin JE, Rawls JF. In vivo analysis of white adipose tissue in zebrafish. Methods Cell Biol. 2011;105:63-86. PMID:21951526
- Song Y, Cone RD. Creation of a genetic model of obesity in a teleost. FASEB J. 2007;21(9):2042-9. doi:10.1096/fj.06-7503com. PMID:17341684
- Tingaud-Sequeira A, Ouadah N, Babin PJ. Zebrafish obesogenic test: a tool for screening molecules that target adiposity. J Lipid Res. 2011;52(9):1765-72. doi:10.1194/jlr.D017012. PMID:21724975
- Minchin JE, Rawls JF. A classification system for zebrafish adipose tissues. Dis Model Mech. 2017;10(6):797-809. doi:10.1242/dmm.025759. PMID:28348140
- Ouadah-Boussouf N, Babin PJ. Pharmacological evaluation of the mechanisms involved in increased adiposity in zebrafish triggered by the environmental contaminant tributyltin. Toxicol Appl Pharmacol. 2016;294:32-42. doi:10.1016/j.taap.2016.01.014. PMID:26812627
- Bridgewater RE, Norman JC, Caswell PT. Integrin trafficking at a glance. J Cell Sci. 2012;125(Pt 16):3695-701. doi:10.1242/jcs.095810. PMID:23027580
- Kim LT, Yamada KM. The regulation of expression of integrin receptors. Proc Soc Exp Biol Med. 1997;214(2):123-31. doi:10.3181/00379727-214-44078. PMID:9034129
- Boudreau NJ, Jones PL. Extracellular matrix and integrin signalling: the shape of things to come. Biochem J. 1999;339(Pt 3):481-8. doi:10.1042/bj3390481. PMID:10215583
- Cheli Y, Kanaji S, Jacquelin B, Chang M, Nugent DJ, Kunicki TJ. Transcriptional and epigenetic regulation of the integrin collagen receptor locus ITGA1-PELO-ITGA2. Biochim Biophys Acta. 2007;1769(9–10):546-58. doi:10.1016/j.bbaexp.2007.06.004. PMID:17669516
- Hotchin NA, Watt FM. Transcriptional and post-translational regulation of beta 1 integrin expression during keratinocyte terminal differentiation. J Biol Chem. 1992;267(21):14852-8. PMID:1378840
- Kim C, Ye F, Ginsberg MH. Regulation of integrin activation. Annu Rev Cell Dev Biol. 2011;27:321-45. doi:10.1146/annurev-cellbio-100109-104104. PMID:21663444
- Chen W, Harbeck MC, Zhang W, Jacobson JR. MicroRNA regulation of integrins. Transl Res. 2013;162(3):133-43. doi:10.1016/j.trsl.2013.06.008. PMID:23859989
- Lilja J, Zacharchenko T, Georgiadou M, Jacquemet G, De Franceschi N, Peuhu E, Hamidi H, Pouwels J, Martens V, Nia FH, et al. SHANK proteins limit integrin activation by directly interacting with Rap1 and R-Ras. Nat Cell Biol. 2017;19(4):292-305. doi:10.1038/ncb3487. PMID:28263956
- Peuhu E, Kaukonen R, Lerche M, Saari M, Guzmán C, Rantakari P, De Franceschi N, Wärri A, Georgiadou M, Jacquemet G, et al. SHARPIN regulates collagen architecture and ductal outgrowth in the developing mouse mammary gland. EMBO J. 2017;36(2):165-182. doi:10.15252/embj.201694387. PMID:27974362
- Georgiadou M, Lilja J, Jacquemet G, Guzmán C, Rafaeva M, Alibert C, Yan Y, Sahgal P, Lerche M, Manneville JB, et al. AMPK negatively regulates tensin-dependent integrin activity. J Cell Biol. 2017;216(4):1107-1121. doi:10.1083/jcb.201609066. PMID:28289092
- Kim SH, Turnbull J, Guimond S. Extracellular matrix and cell signalling: the dynamic cooperation of integrin, proteoglycan and growth factor receptor. J Endocrinol. 2011;209(2):139-51. doi:10.1530/JOE-10-0377. PMID:21307119
- Gardner H, Broberg A, Pozzi A, Laato M, Heino J. Absence of integrin alpha1beta1 in the mouse causes loss of feedback regulation of collagen synthesis in normal and wounded dermis. J Cell Sci. 1999;112(Pt 3):263-72. PMID:9885280
- Ivaska J, Reunanen H, Westermarck J, Koivisto L, Kähäri VM, Heino J. Integrin alpha2beta1 mediates isoform-specific activation of p38 and upregulation of collagen gene transcription by a mechanism involving the alpha2 cytoplasmic tail. J Cell Biol. 1999;147(2):401-16. doi:10.1083/jcb.147.2.401. PMID:10525544
- Liu J, DeYoung SM, Zhang M, Zhang M, Cheng A, Saltiel AR. Changes in integrin expression during adipocyte differentiation. Cell Metab. 2005;2(3):165-77. doi:10.1016/j.cmet.2005.08.006. PMID:16154099
- Morandi EM, Verstappen R, Zwierzina ME, Geley S, Pierer G, Ploner C. ITGAV and ITGA5 diversely regulate proliferation and adipogenic differentiation of human adipose derived stem cells. Sci Rep. 2016;6:28889. doi:10.1038/srep28889. PMID:27363302
- Luk CT, Shi SY, Cai EP, Sivasubramaniyam T, Krishnamurthy M, Brunt JJ, Schroer SA, Winer DA, Woo M. FAK signalling controls insulin sensitivity through regulation of adipocyte survival. Nat Commun. 2017;8:14360. doi:10.1038/ncomms14360. PMID:28165007
- Li JJ, Xie D. Cleavage of focal adhesion kinase (FAK) is essential in adipocyte differentiation. Biochem Biophys Res Commun. 2007;357(3):648-54. doi:10.1016/j.bbrc.2007.03.184. PMID:17442274
- Zhang Z, Vuori K, Wang H, Reed JC, Ruoslahti E. Integrin activation by R-ras. Cell. 1996;85(1):61-9. doi:10.1016/S0092-8674(00)81082-X. PMID:8620538
- Conklin MW, Ada-Nguema A, Parsons M, Riching KM, Keely PJ. R-Ras regulates beta1-integrin trafficking via effects on membrane ruffling and endocytosis. BMC Cell Biol. 2010;11:14. doi:10.1186/1471-2121-11-14. PMID:20167113
- McHugh BJ, Buttery R, Lad Y, Banks S, Haslett C, Sethi T. Integrin activation by Fam38A uses a novel mechanism of R-Ras targeting to the endoplasmic reticulum. J Cell Sci. 2010;123(Pt 1):51-61. doi:10.1242/jcs.056424. PMID:20016066
- Powelka AM, Sun J, Li J, Gao M, Shaw LM, Sonnenberg A, Hsu VW. Stimulation-dependent recycling of integrin beta1 regulated by ARF6 and Rab11. Traffic. 2004;5(1):20-36. doi:10.1111/j.1600-0854.2004.00150.x. PMID:14675422
- Hongu T, Funakoshi Y, Fukuhara S, Suzuki T, Sakimoto S, Takakura N, Ema M, Takahashi S, Itoh S, Kato M, et al. Arf6 regulates tumour angiogenesis and growth through HGF-induced endothelial beta1 integrin recycling. Nat Commun. 2015;6:7925. doi:10.1038/ncomms8925. PMID:26239146
- Oinuma I, Ishikawa Y, Katoh H, Negishi M. The Semaphorin 4D receptor Plexin-B1 is a GTPase activating protein for R-Ras. Science. 2004;305(5685):862-5. doi:10.1126/science.1097545. PMID:15297673
- Sakurai A, Jian X, Lee CJ, Manavski Y, Chavakis E, Donaldson J, Randazzo PA, Gutkind JS. Phosphatidylinositol-4-phosphate 5-kinase and GEP100/Brag2 protein mediate antiangiogenic signaling by semaphorin 3E-plexin-D1 through Arf6 protein. J Biol Chem. 2011;286(39):34335-45. doi:10.1074/jbc.M111.259499. PMID:21795701
- Sakurai A, Doci CL, Gutkind JS. Semaphorin signaling in angiogenesis, lymphangiogenesis and cancer. Cell Res. 2012;22(1):23-32. doi:10.1038/cr.2011.198. PMID:22157652
- Farnier C, Krief S, Blache M, Diot-Dupuy F, Mory G, Ferre P, Bazin R. Adipocyte functions are modulated by cell size change: potential involvement of an integrin/ERK signalling pathway. Int J Obes Relat Metab Disord. 2003;27(10):1178-86. doi:10.1038/sj.ijo.0802399. PMID:14513065
- Kisanuki YY, Hammer RE, Miyazaki J, Williams SC, Richardson JA, Yanagisawa M. Tie2-Cre transgenic mice: a new model for endothelial cell-lineage analysis in vivo. Dev Biol. 2001;230(2):230-42. doi:10.1006/dbio.2000.0106. PMID:11161575
- Carlson TR, Hu H, Braren R, Kim YH, Wang RA. Cell-autonomous requirement for beta1 integrin in endothelial cell adhesion, migration and survival during angiogenesis in mice. Development. 2008;135(12):2193-202. doi:10.1242/dev.016378. PMID:18480158
- Lei L, Liu D, Huang Y, Jovin I, Shai SY, Kyriakides T, Ross RS, Giordano FJ. Endothelial expression of beta1 integrin is required for embryonic vascular patterning and postnatal vascular remodeling. Mol Cell Biol. 2008;28(2):794-802. doi:10.1128/MCB.00443-07. PMID:17984225
- Tanjore H, Zeisberg EM, Gerami-Naini B, Kalluri R. Beta1 integrin expression on endothelial cells is required for angiogenesis but not for vasculogenesis. Dev Dyn. 2008;237(1):75-82. doi:10.1002/dvdy.21385. PMID:18058911
- Zovein AC, Luque A, Turlo KA, Hofmann JJ, Yee KM, Becker MS, Fassler R, Mellman I, Lane TF, Iruela-Arispe ML. Beta1 integrin establishes endothelial cell polarity and arteriolar lumen formation via a Par3-dependent mechanism. Dev Cell. 2010;18(1):39-51. doi:10.1016/j.devcel.2009.12.006. PMID:20152176
- Nolan DJ, Ginsberg M, Israely E, Palikuqi B, Poulos MG, James D, Ding BS, Schachterle W, Liu Y, Rosenwaks Z, et al. Molecular signatures of tissue-specific microvascular endothelial cell heterogeneity in organ maintenance and regeneration. Dev Cell. 2013;26(2):204-19. doi:10.1016/j.devcel.2013.06.017. PMID:23871589
- Choi JH, Gimble JM, Vunjak-Novakovic G, Kaplan DL. Effects of hyperinsulinemia on lipolytic function of three-dimensional adipocyte/endothelial co-cultures. Tissue Eng Part C Methods. 2010;16(5):1157-65. doi:10.1089/ten.tec.2009.0760. PMID:20144013
- Choi JH, Bellas E, Gimble JM, Vunjak-Novakovic G, Kaplan DL. Lipolytic function of adipocyte/endothelial cocultures. Tissue Eng Part A. 2011;17(9–10):1437-44. doi:10.1089/ten.tea.2010.0527. PMID:21247356
- Zerbino DR, Wilder SP, Johnson N, Juettemann T, Flicek PR. The ensembl regulatory build. Genome Biol. 2015;16:56. doi:10.1186/s13059-015-0621-5. PMID:25887522