ABSTRACT
Numerous studies have shown that feeding rodents n-3 polyunsaturated fatty acids attenuates adiposity. Moreover, meta-analyses of human dietary intervention studies indicate that fish oil (eicosapentaenoic and docosahexaenoic acid) supplementation might reduce waist circumference. A recent line of research suggests that browning of white adipose depots and activation of uncoupled respiration in brown fat contributes to these effects. This mini-review summarizes the observations in rodents, highlights several mechanisms that might explain these observations and discusses the translational potential. Given the available in vivo evidence and the ability of human adipocytes to aquire a beige phenotype in response to eicosapentaenoic acid incubation, future studies should test the hypothesis that fish oil activates thermogenic brown and beige adipose tissue in humans.
Introduction
Two types of tissue constitutes the human adipose organ. White adipose tissue (WAT) stores excess energy as triglycerides in subcutaneous and visceral depots and, when enlarged, these depots burden other organ systems mechanically and drives metabolic derangements [Citation1]. In addition to WAT, minor depots of brown adipose tissue (BAT) are localized in cervical, supraclavicular and paravertebral regions and play a major role in non-shivering thermogenesis (NST) [Citation2]. However, NST is not only restricted to brown adipocytes as certain stimuli can cause so-called beige adipocytes to emerge within WAT depots in a process termed ‘browning’ [Citation2]. In contrast to unilocular white adipocytes, both brown and beige fat cells are multilocular and, importantly, their abundant mitochondria contains uncoupling protein 1 (UCP1). By being present within the inner mitochondrial membrane, UCP1 allows protons to bypass ATP synthase when reentrying the mitochondrial matrix. As a result, respiration becomes uncoupled and the potential energy of the electrochemical gradient is dissipated as heat [Citation2]. Cold exposure is a classical activator of NST. Upon cooling, norepinephrine (NE) is released from sympathetic nerves innervating BAT. NE is a ligand for β3-adrenergic receptors on the surface of brown and beige adipocytes, which when activated triggers an intracellular signaling cascade leading to expression of thermogenic genes and uptake of glucose and lipids from the bloodstream [Citation3]. Given these effects, activation of thermogenic fat cells represents a potential strategy for counteracting obesity and its associated cardiometabolic disorders.
Interestingly, both activation of BAT and browning of WAT have been shown to be induced by ingestion of specific bioactive food components [Citation4]. Fish oil, which contains the long-chain n-3 polyunsaturated fatty acids (n-3 LCPUFAs), eicosapentaenoic acid (EPA) and docosahexaenoic acid (DHA), is one such example. Numerous studies have shown that n-3 PUFAs, both n-3 LCPUFA and α-linolenic acid, attenuate adiposity in rodents [Citation5,Citation6] and while these findings have been attributed to induction of fatty acid oxidation and suppression of lipogenesis previously [Citation7], a recent line of research indicates that activation of thermogenic adipocytes mediates at least part of its anti-obesity effect [Citation8–Citation12]. In this mini-review, we describe current evidence regarding effects of marine n-3 LCPUFAs on the biogenesis of thermogenic fat cells and NST in adipose tissues. Furthermore, we highlight several mechanisms that might underlie these observations and lastly, we discuss the translational potential of the findings.
Fish oil and thermogenic adipose tissues
More than twenty years ago, it was reported that a diet enriched in PUFAs but otherwise normal with regards to total fat content increased both the level of UCP1 and the capacity for NST in murine interscapular BAT (iBAT) [Citation13]. More recently, it was shown that refeeding rats a diet enriched in PUFAs, but not in saturated fatty acids, following two weeks of food restriction increased iBAT mass and expression of Ucp1 [Citation14]. These findings suggest that PUFAs may improve the thermogenic capacity of BAT. Yet, PUFAs are a heterogenous class of fatty acids that differ in chain length, degree of unsaturation and localization of the double bonds. While EPA and DHA have been shown to attenuate adiposity and promote thermogenesis in brown and beige adipocytes, the n-6 LCPUFA arachidonic acid seems to impair browning of murine WAT and differentiation of human preadipocytes into beige fat cells [Citation15,Citation16]. Along these lines, it is interesting to note that a high dietary ratio of n-6 to n-3 fatty acids has been proposed as a factor contributing to the development of obesity [Citation6,Citation17] and that a low n-6/n-3 ratio has recently been suggested to promote adipose thermogenesis [Citation16].
Brown adipose tissue
Feeding rats marine n-3 LCPUFAs has been found to stimulate the thermogenic activity of iBAT [Citation18]. This finding is supported by other experiments in rats that showed increased Ucp1 expression [Citation19] and protein levels [Citation20] in iBAT following administration of n-3 LCPUFA. Furthermore, when rats were fed fish oil in combination with conjugated linoleic acid, another lipid proposed to stimulate biogenesis of thermogenic fat cells [Citation21], iBAT mass increased and expression of Ucp1 was induced in this adipose depot [Citation22]. These findings from rats are in line with what has been demonstrated in mice. While fish oil did not affect expression of Ucp1 in two studies [Citation23,Citation24], several other investigations have demonstrated that it induces Ucp1 expression in murine iBAT [Citation8–Citation10,Citation12,Citation25]. Apart from inducing expression of Ucp1, fish oil has also been shown to increase both transcript and protein levels of several other thermogenic and oxidative genes in iBAT [Citation8-Citation10,Citation12] and, importantly, histological evidence has shown denser UCP1-staining and increased multilocularity in iBAT in fish oil-fed mice [Citation8,Citation10].
White adipose depots
In addition to increased UCP1 levels in iBAT, browning of WAT depots has also been reported following fish oil administration. With regards to murine epididymal WAT (eWAT), two studies have reported an induction of Ucp1 expression following fish oil treatment [Citation24,Citation26], and when fish oil were combined with fucoxanthin, another dietary compound suggested to activate thermogenic fat cells [Citation27,Citation28], both transcript and protein levels of UCP1 increased in eWAT [Citation29]. However, other investigations have not found evidence of eWAT browning in response to fish oil [Citation12,Citation30] and inconsistent findings have also been reported with regards to expression of other oxidative genes in eWAT [Citation12,Citation23,Citation24,Citation26,Citation30].
In inguinal WAT (iWAT), fish oil has been reported to increase expression of several browning marker genes such as Ucp1, Prdm16, Tbx1, Pgc-1α, Cidea, Adrb3, Cd137, and Cpt-1β [Citation8,Citation11]. Levels of UCP1 were also observed to increase in these studies [Citation8,Citation11] and, importantly, Bargut and coworkers demonstrated that both 119 g and 238 g of fish oil per kg feed (approximately equivalent to 36 – 71 g n-3 LCPUFA per kg feed) induced a multilocular appearance of iWAT in a dose-dependent manner [Citation11]. However, another study employing a similar dose (36 g EPA per kg feed) found no evidence of iWAT browning [Citation12]. The doses used in both of these studies are very high compared to what is relevant for human consumption, as it is estimated to be equivalent to around 14 – 28 g/d of n-3 LCPUFA in humans assuming that we eat around 400 g/d of food with a water content like rodent pellets. The daily intake of marine n-3 LCPUFAs in fish-eating adults is ∼0.5 g/d [Citation31] and intake in a traditional Inuit diet is maximum 8 g/d [Citation32]. Thus, the amount of n-3 LCPUFA required to induce iWAT browning is much higher than what is not even of pharmacological relevance.
Does fish oil induce non-shivering thermogenesis?
Given that fish oil administration induces thermogenic gene expression in iBAT and browning of iWAT, fish oil-fed mice would be expected to display physiological changes reflecting an increase in NST. This has been tested by challenging mice with a cold test (8°C for 45 minutes). As anticipated, fish oil-fed mice maintained higher rectal temperatures and released more heat compared to the controls [Citation9]. Furthermore, when mice were housed at 23°C, fish oil feeding was also associated with increased rectal temperature and higher oxygen consumption [Citation8]. These findings suggest that the thermogenic gene induction observed in isolated tissues and cells translates into an improved capacity for uncoupled respiration in brown and beige adipocytes in vivo. In fact, in vitro studies have demonstrated that EPA improved respiratory capacity of brown adipocytes as evidenced by an increased mitochondrial content, improved glycolytic capacity [Citation12] and increased basal, uncoupled and maximal respiration [Citation9].
Molecular mechanisms
Given these findings from rodents, it is relevant to ask whether the effect of fish oil is adipocyte-autonomous or mediated via other cells in adipose tissue, e.g. immune cells, neurons or endothelial cells? Several in vitro studies have reported that EPA promotes brown adipogenesis [Citation9,Citation33,Citation34]. Interestingly, these effects are apparently specific for EPA as one of the studies found that DHA did not promote brown adipogenesis [Citation33] or affect the mitochondrial mass of brown adipocytes [Citation12]. Similar EPA-specific effects have been observed for beige adipogenesis, as EPA, but not DHA, has been observed to promote differentiation of white preadipocytes towards a beige phenotype [Citation33-Citation35]. Hence, these in vitro findings clearly indicate that EPA is able to promote both brown and beige adipogenesis in an adipocyte-autonomous manner and one potential intracellular mediator of this effect might be AMP-activated kinase (AMPK) [Citation36,Citation37].
Given that obesity is associated with reduced BAT activity [Citation2], it could be argued that the thermogenic effect of fish oil observed in vivo might be attributable to reduced adiposity and thus restored ‘normal’ thermogenic capacity. While this might be a contributing factor, the in vitro findings indicate that fish oil may also have a direct thermogenic effect. This notion is supported by in vivo studies showing that mice fed a high-fat diet combined with fish oil displayed elevated UCP1 levels in iBAT [Citation9,Citation12] and generated more heat [Citation9] compared to low-fat fed controls, despite that the two groups of mice had similar fat masses [Citation9] and that fish oil-fed mice have been found to weigh more than the low fat-fed controls [Citation12].
Apart from acting directly on adipocytes, fish oil is also likely to stimulate thermogenic adipocytes by acting as a secretagouge of adipokines such as apelin [Citation38,Citation39], by activating afferent sympathetic nerves in the gastrointestinal tract [Citation8,Citation40], and possibly also by modulating low-grade inflammation in adipose tissues () [Citation41]. Various immune cells reside in fat tissue and several of these have been implicated in the regulation of adipocyte thermogenesis [Citation42]. Like adipocytes, both macrophages [Citation43] and eosinophils [Citation44] have a G-protein-coupled receptor termed G protein-coupled receptor 120 (GPR120) or free fatty acid receptor 4 (FFAR4) embedded in their plasma membrane. As implied by its name, dietary fatty acids including n-3 LCPUFAs are ligands of GPR120 [Citation45] and pharmacological activation of this cell-surface receptor seems to induce secretion of interleukin-4 (IL-4) from eosinophils [Citation44] and to supress release of pro-inflammatory mediators from macrophages [Citation43]. These findings are interesting for two reasons. First, pro-inflammatory cytokines impair the thermogenic properties of brown and beige adipocytes [Citation46-Citation50] and secondly, IL-4 seems to promote WAT browning by sustaining macrophages in the alternatively-activated and anti-inflammatory M2 phenotype [Citation42]. Hence, it could be speculated that the immunomodulatory effects of GPR120 activation might contribute to fish oil-induced WAT browning and BAT thermogenesis. Additionally, in adipocytes GPR120 might also mediate fish oil-induced adipose thermogenesis by promoting angiogenesis. Several studies have highlighted the involvement of this process in WAT browning [Citation51–Citation55] and, in humans, an increased capillarization of WAT has been observed in response to EPA administration [Citation41]. Moreover, an in vitro study [Citation56] has shown that incubation of mature 3T3-L1 adipocytes with EPA induced expression and secretion of vascular endothelial growth factor A (VEGF-A), a pro-angiogenic protein [Citation55]. Because these effects occured in a GPR120-dependent manner, a mechanistic link can be proposed between EPA, GPR120 and VEGF-A. Another adipokine that has been implicated in adipose thermogenesis is fibroblast growth factor 21 (FGF21). Like VEGF-A, secretion of FGF21 from fat cells is also dependent upon GPR120, as recently reported by Quesada-López and coworkers [Citation34]. They demonstrated that protein level of GPR120 increased in iBAT and iWAT following cold exposure and that oral administration of GW9508, a GPR120 agonist, induced expression of thermogenic genes in iBAT and the emergence of multilocular beige adipocytes in iWAT [Citation34]. Likewise, in vitro studies showed that treatment with GW9508 induced brown and beige adipogenesis and increased heat production in mature thermogenic fat cells [Citation34]. Importantly, the results obtained by this pharmacological approach were mimicked in vitro by incubating adipocytes with EPA, and the importance of GPR120 as a mediator was highlighted by the fact that precursor cells derived from iBAT and iWAT of GPR120 knockout mice were less responsive to EPA treatment [Citation34]. Apart from stimulating brown/beige adipocyte differentiation and heat production, EPA was also found to increase secretion of FGF21 from these fat cells in a GPR120-dependent manner. Unfortunately, this study did not directly test whether FGF21 mediated the effects of EPA, but based upon additional mechanistic investigations using GW9508 and studies in mice and adipocytes that lack FGF21, it was proposed that this adipokine mediates at least part of the thermogenic effects occuring in response to GPR120 activation [Citation34]. While FGF21 and VEGF-A are external factors that promote adipose thermogenesis in an endocrine/autocrine manner, microRNAs act intracellularly to orchestrate brown and beige adipocyte biogenesis and function [Citation57]. Interestingly, both EPA-mediated induction of brown adipogenesis in vitro and upregulation of Ucp1 expression in murine iBAT occurs in association with increased expression of several microRNAs [Citation9]. One study reported that induction of miR-30b in response to EPA treatment was regulated by GPR120 [Citation9] and because miR-30b targets Rip140, a co-repressor that inhibits expression of Ucp1 [Citation57], miR-30b might play a role in mediating the effects of EPA on BAT [Citation9].
Figure 1. A mechanistic model highlighting potential mechanisms underlying fish oil-induced biogenesis and activation of thermogenic adipocytes. In vivo evidence has suggested that fish oil activates TRPV1 in the gastrointestinal tract and that this, by stimulating the sympathetic nerves that innervate fat cells, causes adipose thermogenesis. Fish oil also acts as a ligand of GPR120 and activation of this cell-surface receptor seems to induce several effects that might contribute to the thermogenic effects of fish oil. In adipocytes this includes expression of miR-30b and secretion of FGF21. Furthermore, secretion of VEGF-A promotes angiogenesis and anti-inflammatory effects are induced in immune cells upon activation of GPR120. In addition to these mechanisms, activation of AMPK in adipocytes and secretion of apelin might be implicated as well.
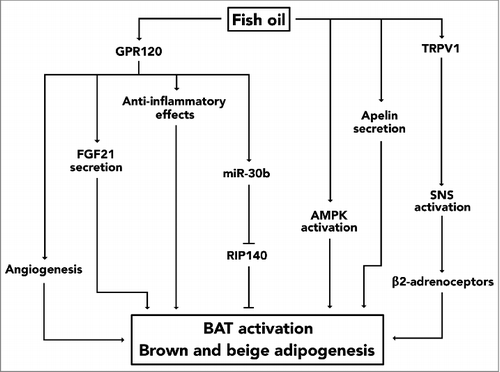
In mice, knockout of the ion channel termed 'transient receptor potential cation channel subfamily V member 1 (TRPV1) abolished the induction of Ucp1 expression that occurs in both iBAT and iWAT in response to fish oil administration [Citation8]. Given that subdiaphragmatic vagotomy also impairs expression of Ucp1 in the same adipose depots, it has been suggested that fish oil could promote adipose thermogenesis by acting as ligand of TRPV1 in those afferent nerves of the gastrointestinal tract that activate the sympathetic nervous system (SNS) [Citation8]. This proposed mechanism might be part of a larger interorgan axis that extends from TRPV1 in the digestive system, via the brain, to adipose β2-adrenergic receptors [Citation40].
Translational potential
While it has frequently been reported that n-3 LCPUFA antagonizes adiposity in rodents [Citation5], it is less clear if similar effects can be obtained in humans (For a commentary, see [Citation58]). Dietary intervention studies in adults have generally shown that fish oil supplementation does not decrease body weight [Citation59–Citation61]. In contrast to the effect on total body mass, some studies have indicated that fish oil might specifically reduce fat mass. As such, fish oil supplementation has been suggested to attenuate accretion of adipose mass in growing infants [Citation62] and in young, normalweight adults, it has been reported that ingestion of a diet containing 6 g/d of fish oil for three weeks increased fat oxidation and decreased fat mass [Citation59]. Furthermore, several meta-analyses have shown reductions in waist circumference in response to fish oil supplementation [Citation61,Citation63,Citation64], suggesting that these bioactive marine lipids might promote loss of fat mass preferentially from visceral depots. Whether such potential effects in humans could be due to adipose NST is currently unknown.
Recently, it has been reported that human adipocytes, like those of mice, can acquire a beige phenotype when treated with EPA. Using primary human preadipocytes derived from abdominal and mammary subcutanous WAT of lean female donors, Fleckenstein-Elsen and coworkers showed that differentiation in the presence of 20 μM EPA caused a ∼4-fold induction of UCP1 expression [Citation35]. In another in vitro study, subcutaneous adipocytes from overweight donors were stimulated with either 100 or 200 μM EPA. While 100 μM of EPA promoted mitochondrial biogenesis and increased expression of various thermogenic genes, UCP1 expression was only induced in response to an EPA concentration of 200 μM [Citation65]. One explanation for this difference between results in adipocytes derived from lean and overweight donors might be that adiposity impairs the potential of WAT to undergo browning [Citation50,Citation66,Citation67] and that a higher dose of EPA is therefore required in order to induce browning of adipocytes in the obese state.
Another important aspect to consider when evaluating the translational potential is whether the molecular mechanisms deduced from animal experiments are compatible with human physiology. The plasma concentration of both DHA and EPA has been reported to reach ∼150 μM after four weeks of dietary supplementation with 1.3 g/d of fish oil [Citation68] and given that ∼99% of free fatty acids in plasma are bound to albumin, it has been estimated that the plasma concentration of unbound fatty acid anions is in the range of 0.01 to 10 μM [Citation69]. Considering these points and that GPR120 has been shown to display EC50 values for DHA and EPA of ∼2 – 5 μM [Citation70,Citation71], GPR120 can be regarded as a potentially relevant mediator of fish oil-induced adipose thermogenesis in humans. Besides GPR120, a mechanistic role for the SNS is supported by an observed increase in both iBAT and iWAT NE turnover and elevated urinary excretion of catecholamines in fish oil-fed mice [Citation8]. In humans, however, it is unclear whether fish oil also displays a sympathostimulatory effect. In the previously mentioned dietary intervention study by Couet and colleagues, it was reported that urinary excretion of catecholamines increased following three weeks on a fish oil-enriched diet (6 g/d) [Citation59]. However, the increase was not significant, which may be due to low power as the study only included six participants. In contrast, a larger intervention study reported that circulating NE levels decreased in young and healthy adults following two months of fish oil supplementation (0.76 g/d) [Citation72]. Furthermore, support for a decreased overall sympathetic tone might be inferred from meta-analyses that have shown modest reductions in both heart rate [Citation73] and blood pressure [Citation74] following ingestion of fish oil. While this suggests that fish oil may not elevate systemic NE levels, it does not exclude the possibility that n-3 LCPUFAs may directly activate the sympathetic nerves that innervate BAT. Here, it is of relevance to mention that ingestion of other dietary TRPV1 agonists have been shown to increase energy expenditure in humans in a BAT-dependent manner [Citation75,Citation76]. These studies combined with the in vitro and in vivo findings highlighted above, justify further thorough clinical investigations of the proposed thermogenic impact of fish oil in humans.
Conclusion
A high dietary ratio between n-6 and n-3 fatty acids has been proposed to contribute to the development of obesity. In rodents, fish oil has been shown to alleviate adiposity and this appears to be partially attributed to activation of thermogenic brown and beige adipocytes. In vitro studies have shown that EPA induces expression of thermogenic genes in brown adipocytes and promotes differentiation of white preadipocytes into beige fat cells. Furthermore, feeding mice and rats diets enriched in fish oil appears to upregulate UCP1 levels in iBAT, induce browning of iWAT and promote thermogenesis under cold conditions. Various mechanisms might mediate these effects. While activation of TRPV1 in the digestive system has been proposed to induce NST, several different mechanisms are potentially involved in adipose tissue. These include secretion of apelin and activation of AMPK but also GPR120-mediated angiogenesis, amelioration of low-grade inflammation, release of FGF21 and induction of miR-30b expression. Dietary intervention studies suggest that humans might experience minor reductions in fat mass following fish oil supplementation, but it is currently unknown whether fish oil activates human BAT. Human white preadipocytes have been shown to respond to EPA incubation by differentiating towards a beige phenotype in the same way as in murine fat cells. These findings, combined with observations in rodents, suggest that fish oil may also increase human energy expenditure by activation of thermogenic fat cells. Future intervention studies should therefore test the hypothesis that fish oil supplementation promotes NST in human brown and beige adipose tissue.
Abbreviations
AMPK | = | AMP-activated kinase |
BAT | = | brown adipose tissue |
DHA | = | docosahexaenoic acid |
EPA | = | eicosapentaenoic acid |
eWAT | = | epididymal white adipose tissue |
FFAR4 | = | free fatty acid receptor 4 |
FGF21 | = | fibroblast growth factor 21 |
GPCR | = | G protein-coupled receptor |
GPR120 | = | G protein-coupled receptor 120 |
iBAT | = | interscapular brown adipose tissue |
IL-4 | = | interleukin-4 |
iWAT | = | inguinal white adipose tissue |
n-3 LCPUFAs | = | long-chain n-3 polyunsaturated fatty acids |
NE | = | norepinephrine |
NST | = | non-shivering thermogenesis |
SNS | = | sympathetic nervous system |
TRPV1 | = | transient receptor potential cation channel subfamily V member 1 |
UCP1 | = | uncoupling protein 1 |
VEGF-A | = | vascular endothelial growth factor A |
WAT | = | white adipose tissue. |
Disclosure statements
LHL has received research materials and research funding from Rimfrost A/S and Orkla Care Denmark. JL and LL report no conflict of interest.
Additional information
Funding
References
- Heymsfield SB, Wadden TA. Mechanisms, pathophysiology and management of obesity. N Engl J Med. 2017;376:254–266. doi:10.1056/NEJMra1514009
- Scheele C, Nielsen S. Metabolic regulation and the anti-obesity perspectives of human brown fat. Redox Biol. 2017;12:770–775. doi:10.1016/j.redox.2017.04.011
- Hoeke G, Kooijman S, Boon MR, et al. Role of brown fat in lipoprotein metabolism and atherosclerosis. Circ Res. 2016;118:173–182. doi:10.1161/CIRCRESAHA.115.306647
- Okla M, Kim J, Koehler K, et al. Dietary factors promoting brown and beige fat development and thermogenesis. Adv Nutr. 2017;8:473–483. doi:10.3945/an.116.014332
- Li JJ, Huang CJ, Xie D. Anti-obesity effects of conjugated linoleic acid, docosahexaenoic acid and eicosapentaenoic acid. Mol Nutr Food Res. 2008;52:631–645. doi:10.1002/mnfr.200700399
- Ailhaud G, Guesnet P, Cunnane SC. An emerging risk factor for obesity: does disequilibrium of polyunsaturated fatty acid metabolism contribute to excessive adipose tissue development? Br J Nutr. 2008;100:461–470. doi:10.1017/S0007114508911569
- Martínez-Fernández L, Laiglesia LM, Huerta AE, et al. Omega-3 fatty acids and adipose tissue function in obesity and metabolic syndrome. Prostaglandins Other Lipid Mediat. 2015;121:24–41. doi:10.1016/j.prostaglandins.2015.07.003
- Kim M, Goto T, Yu R, et al. Fish oil intake induces UCP1 upregulation in brown and white adipose tissue via the sympathetic nervous system. Sci Rep. 2015;5:18013. doi:10.1038/srep18013
- Kim J, Okla M, Erickson A, et al. Eicosapentaenoic acid potentiates brown thermogenesis through FFAR4-dependent upregulation of miR-30b and miR-378. J Biol Chem. 2016;291:20551–20562. doi:10.1074/jbc.M116.721480
- Bargut TC, Silva-e-Silva AC, Souza-Mello V, et al. Mice fed fish oil diet and upregulation of brown adipose tissue thermogenic markers. Eur J Nutr. 2016;55:159–169. doi:10.1007/s00394-015-0834-0
- Bargut TC, Souza-Mello V, Mandarim-de-Lacerda CA, et al. Fish oil diet modulates epididymal and inguinal adipocyte metabolism in mice. Food Funct. 2016;7:1468–1476. doi:10.1039/C5FO00909J
- Pahlavani M, Razafimanjato F, Ramalingam L, et al. Eicosapentaenoic acid regulates brown adipose tissue metabolism in high-fat-fed mice and in clonal brown adipocytes. J Nutr Biochem. 2017;39:101–109. doi:10.1016/j.jnutbio.2016.08.012
- Sadurskis A, Dicker A, Cannon B, et al. Polyunsaturated fatty acids recruit brown adipose tissue: increased UCP content and NST capacity. Am J Physiol. 1995;269:351–360.
- Crescenzo R, Mazzoli A, Cancelliere R, et al. Polyunsaturated fatty acids stimulate de novo lipogenesis and improve glucose homeostasis during refeeding with high fat diet. Front Physiol. 2017;8:178. doi:10.3389/fphys.2017.00178
- Pisani DF, Ghandour RA, Beranger GE, et al. The ω6-fatty acid, arachidonic acid, regulates the conversion of white to brite adipocyte through a prostaglandin/calcium mediated pathway. Mol Metab. 2014;3:834–847. doi:10.1016/j.molmet.2014.09.003
- Ghandour RA, Colson C, Giroud M, et al. Impact of dietary ω3 polyunsaturated fatty acid supplementation on brown and brite adipocyte function. J Lipid Res. 2018;[ Jan 17]. DOI:10:1194.
- Simopoulos AP. An increase in the omega-6/omega-3 fatty acid ratio increases the risk for obesity. Nutrients. 2016;8:128. doi:10.3390/nu8030128
- Oudart H, Groscolas R, Calgari C, et al. Brown fat thermogenesis in rats fed high-fat diets enriched with n-3 polyunsaturated fatty acids. Int J Obes Relat Metab Disord. 1997;21:955–962. doi:10.1038/sj.ijo.0800500
- Takahashi Y, Ide T. Dietary n-3 fatty acids affect mRNA level of brown adipose tissue uncoupling protein 1, and white adipose tissue leptin and glucose transporter 4 in the rat. Br J Nutr. 2000;84:175–184.
- Kawada T, Kayahashi S, Hida Y, et al. Fish (Bonito) oil supplementation enhances the expression of uncoupling protein in brown adipose tissue of rat. J Agric Food Chem. 1998;46:1225–1227. doi:10.1021/jf9711000
- Shen W, McIntosh MK. Nutrient regulation: Conjugated linoleic acid's inflammatory and browning properties in adipose tissue. Annu Rev Nutr. 2016;36:183–210. doi:10.1146/annurev-nutr-071715-050924
- Sneddon AA, Rayner DV, Mitchell SE, et al. Dietary supplementation with conjugated linoleic acid plus n-3 polyunsaturated fatty acid increases food intake and brown adipose tissue in rats. Nutrients. 2009;1:178–196. doi:10.3390/nu1020178
- Flachs P, Rühl R, Hensler M, et al. Synergistic induction of lipid catabolism and anti-inflammatory lipids in white fat of dietary obese mice in response to calorie restriction and n-3 fatty acids. Diabetologia. 2011;54:2626–2638. doi:10.1007/s00125-011-2233-2
- Villarroya J, Flachs P, Redondo-Angulo I, et al. Fibroblast growth factor-21 and the beneficial effects of long-chain n-3 polyunsaturated fatty acids. Lipids. 2014;49:1081–1089. doi:10.1007/s11745-014-3948-x
- Ludwig T, Worsch S, Heikenwalder M, et al. Metabolic and immunomodulatory effects of n-3 fatty acids are different in mesenteric and epididymal adipose tissue of diet-induced obese mice. Am J Physiol Endocrinol Metab. 2013;304:1140–1156. doi:10.1152/ajpendo.00171.2012
- Bhaskaran S, Unnikrishnan A, Ranjit R, et al. A fish oil diet induces mitochondrial uncoupling and mitochondrial unfolded protein response in epididymal white adipose tissue of mice. Free Radic Biol Med. 2017;108:704–714. doi:10.1016/j.freeradbiomed.2017.04.028
- Maeda H. Nutraceutical effects of fucoxanthin for obesity and diabetes therapy: a review. J Oleo Sci. 2015;64:125–132. doi:10.5650/jos.ess14226
- Kim KM, Kim SM, Cho DY, et al. The effect of Xanthigen on the expression of brown adipose tissue assessed by 18F-FDG PET. Yonsei Med J. 2016;57:1038–1041. doi:10.3349/ymj.2016.57.4.1038
- Okada T, Mizuno Y, Sibayama S, et al. Antiobesity effects of Undaria lipid capsules prepared with scallop phospholipids. J Food Sci. 2011;76:2–6. doi:10.1111/j.1750-3841.2010.01878.x
- Flachs P, Horakova O, Brauner P, et al. Polyunsaturated fatty acids of marine origin upregulate mitochondrial biogenesis and induce beta-oxidation in white fat. Diabetologia. 2005;48:2365–2375. doi:10.1007/s00125-005-1944-7
- Welch AA, Shakya-Shrestha S, Lentjes MA, et al. Dietary intake and status of n-3 polyunsaturated fatty acids in a population of fish-eating and non-fish-eating meat-eaters, vegetarians, and vegans and the product-precursor ratio of α-linolenic acid to long-chain n-3 polyunsaturated fatty acids: results from the EPIC-Norfolk cohort. Am J Clin Nutr. 2010;92:1040–1051. doi:10.3945/ajcn.2010.29457
- Bang HO, Dyerberg J, Sinclair HM. The composition of the Eskimo food in north western Greenland. Am J Clin Nutr. 1980;33:2657–2661. doi:10.1093/ajcn/33.12.2657
- Zhao M, Chen X. Eicosapentaenoic acid promotes thermogenic and fatty acid storage capacity in mouse subcutaneous adipocytes. Biochem Biophys Res Commun. 2014;450:1446–1451. doi:10.1016/j.bbrc.2014.07.010
- Quesada-López T, Cereijo R, Turatsinze JV, et al. The lipid sensor GPR120 promotes brown fat activation and FGF21 release from adipocytes. Nat Commun. 2016;7:13479. doi:10.1038/ncomms13479
- Fleckenstein-Elsen M, Dinnies D, Jelenik T, et al. Eicosapentaenoic acid and arachidonic acid differentially regulate adipogenesis, acquisition of a brite phenotype and mitochondrial function in primary human adipocytes. Mol Nutr Food Res. 2016;60:2065–2075. doi:10.1002/mnfr.201500892
- Lorente-Cebrián S, Bustos M, Marti A, et al. Eicosapentaenoic acid stimulates AMP-activated protein kinase and increases visfatin secretion in cultured murine adipocytes. Clin Sci (Lond). 2009;117:243–249. doi:10.1042/CS20090020
- Abdul-Rahman O, Kristóf E, Doan-Xuan QM, et al. AMP-activated kinase (AMPK) activation by AICAR in human white adipocytes derived from pericardial white adipose tissue stem cells induces a partial beige-like phenotype. PLoS One. 2016;11:e0157644. doi:10.1371/journal.pone.0157644
- Higuchi K, Masaki T, Gotoh K, et al. Apelin, an APJ receptor ligand, regulates body adiposity and favors the messenger ribonucleic acid expression of uncoupling proteins in mice. Endocrinology. 2007;148:2690–2697. doi:10.1210/en.2006-1270
- Yuzbashian E, Zarkesh M, Asghari G, et al. Is apelin gene expression and concentration affected by dietary intakes? a systematic review. Crit Rev Food Sci Nutr. 2017;[ Jan 26];1-9. DOI:10:1080.
- Lund J, Gillum MP. Towards leanness by ‘feeding’ a novel thermogenic pathway? Trends Endocrinol Metab. 2016;27:529–530.
- Spencer M, Finlin BS, Unal R, et al. Omega-3 fatty acids reduce adipose tissue macrophages in human subjects with insulin resistance. Diabetes. 2013;62:1709–1717. doi:10.2337/db12-1042
- Brestoff JR, Artis D. Immune regulation of metabolic homeostasis in health and disease. Cell. 2015;161:146–160. doi:10.1016/j.cell.2015.02.022
- Oh DY, Talukdar S, Bae EJ, et al. GPR120 is an omega-3 fatty acid receptor mediating potent anti-inflammatory and insulin-sensitizing effects. Cell. 2010;142:687–698. doi:10.1016/j.cell.2010.07.041
- Konno Y, Ueki S, Takeda M, et al. Functional analysis of free fatty acid receptor GPR120 in human eosinophils: implications in metabolic homeostasis. PLoS One. 2015;10:e0120386. doi:10.1371/journal.pone.0120386
- Im DS. Functions of omega-3 fatty acids and FFA4 (GPR120) in macrophages. Eur J Pharmacol. 2016;785:36–43. doi:10.1016/j.ejphar.2015.03.094
- Sakamoto T, Takahashi N, Sawaragi Y, et al. Inflammation induced by RAW macrophages suppresses UCP1 mRNA induction via ERK activation in 10T1/2 adipocytes. Am J Physiol Cell Physiol. 2013;304:729–738. doi:10.1152/ajpcell.00312.2012
- Estève D, Boulet N, Volat F, et al. Human white and brite adipogenesis is supported by MSCA1 and is impaired by immune cells. Stem Cells. 2015;33:1277–1291. doi:10.1002/stem.1916
- Sakamoto T, Nitta T, Maruno K, et al. Macrophage infiltration into obese adipose tissues suppresses the induction of UCP1 level in mice. Am J Physiol Endocrinol Metab. 2016;310:676–687. doi:10.1152/ajpendo.00028.2015
- Goto T, Naknukool S, Yoshitake R, et al. Proinflammatory cytokine interleukin-1β suppresses cold-induced thermogenesis in adipocytes. Cytokine. 2016;77:107–114. doi:10.1016/j.cyto.2015.11.001
- Chung KJ, Chatzigeorgiou A, Economopoulou M, et al. A self-sustained loop of inflammation-driven inhibition of beige adipogenesis in obesity. Nat Immunol. 2017;18:654–664. doi:10.1038/ni.3728
- Elias I, Franckhauser S, Ferré T, et al. Adipose tissue overexpression of vascular endothelial growth factor protects against diet-induced obesity and insulin resistance. Diabetes. 2012;61:1801–1813. doi:10.2337/db11-0832
- During MJ, Liu X, Huang W, et al. Adipose VEGF links the white-to-brown fat switch with environmental, genetic, and pharmacological stimuli in male mice. Endocrinology. 2015;156:2059–2073. doi:10.1210/en.2014-1905
- Min SY, Kady J, Nam M, et al. Human ‘brite/beige’ adipocytes develop from capillary networks, and their implantation improves metabolic homeostasis in mice. Nat Med. 2016;22:312–318. doi:10.1038/nm.4031
- Seki T, Hosaka K, Lim S, et al. Endothelial PDGF-CC regulates angiogenesis-dependent thermogenesis in beige fat. Nat Commun. 2016;7:12152. doi:10.1038/ncomms12152
- Park J, Kim M, Sun K, et al. VEGF-A-expressing adipose tissue shows rapid beiging and enhanced survival after transplantation and confers IL-4-independent metabolic improvements. Diabetes. 2017;66:1479–1490. doi:10.2337/db16-1081
- Hasan AU, Ohmori K, Konishi K, et al. Eicosapentaenoic acid upregulates VEGF-A through both GPR120 and PPARγ mediated pathways in 3T3-L1 adipocytes. Mol Cell Endocrinol. 2015;406:10–18. doi:10.1016/j.mce.2015.02.012
- Shamsi F, Zhang H, Tseng YH. MicroRNA regulation of brown adipogenesis and thermogenic energy expenditure. Front Endocrinol (Lausanne). 2017;8:205. doi:10.3389/fendo.2017.00205
- Madsen L, Kristiansen K. Of mice and men: factors abrogating the antiobesity effect of omega-3 fatty acids. Adipocyte. 2012;1:173–176. doi:10.4161/adip.20689
- Couet C, Delarue J, Ritz P, et al. Effect of dietary fish oil on body fat mass and basal fat oxidation in healthy adults. Int J Obes Relat Metab Disord. 1997;21:637–643. doi:10.1038/sj.ijo.0800451
- Martínez-Victoria E, Yago MD. Omega 3 polyunsaturated fatty acids and body weight. Br J Nutr. 2012;107:107–116. doi:10.1017/S000711451200150X
- Du S, Jin J, Fang W, et al. Does fish oil have an anti-obesity effect in overweight/obese adults? a meta-analysis of randomized controlled trials. PLoS One. 2015;10:e0142652. doi:10.1371/journal.pone.0142652
- Andersen AD, Michaelsen KF, Hellgren LI, et al. A randomized controlled intervention with fish oil versus sunflower oil from 9 to 18 months of age: exploring changes in growth and skinfold thicknesses. Pediatr Res. 2011;70:368–374. doi:10.1203/PDR.0b013e318229007b
- Bender N, Portmann M, Heg Z, et al. Fish or n3-PUFA intake and body composition: a systematic review and meta-analysis. Obes Rev. 2014;15:657–665. doi:10.1111/obr.12189
- Zhang YY, Liu W, Zhao TY, et al. Efficacy of omega-3 polyunsaturated fatty acids supplementation in managing overweight and obesity: A meta-analysis of randomized clinical trials. J Nutr Health Aging. 2017;21:187–192. doi:10.1007/s12603-016-0755-5
- Laiglesia LM, Lorente-Cebrián S, Prieto-Hontoria PL, et al. Eicosapentaenoic acid promotes mitochondrial biogenesis and beige-like features in subcutaneous adipocytes from overweight subjects. J Nutr Biochem. 2016;37:76–82. doi:10.1016/j.jnutbio.2016.07.019
- Carey AL, Vorlander C, Reddy-Luthmoodoo M, et al. Reduced UCP-1 content in in vitro differentiated beige/brite adipocytes derived from preadipocytes of human subcutaneous white adipose tissues in obesity. PLoS One. 2014;9:e91997. doi:10.1371/journal.pone.0091997
- Kern PA, Finlin BS, Zhu B, et al. The effects of temperature and seasons on subcutaneous white adipose tissue in humans: Evidence for thermogenic gene induction. J Clin Endocrinol Metab. 2014;99:2772–2779. doi:10.1210/jc.2014-2440
- Yurko-Mauro K, Kralovec J, Bailey-Hall E, et al. Similar eicosapentaenoic acid and docosahexaenoic acid plasma levels achieved with fish oil or krill oil in a randomized double-blind four-week bioavailability study. Lipids Health Dis. 2015;14:99. doi:10.1186/s12944-015-0109-z
- Spector AA, Hoak JC. Letter: Fatty acids, platelets, and microcirculatory obstruction. Science. 1975;190:490–492. doi:10.1126/science.1166323
- Ulven T, Christiansen E. Dietary fatty acids and their potential for controlling metabolic diseases through activation of FFA4/GPR120. Annu Rev Nutr. 2015;35:239–263. doi:10.1146/annurev-nutr-071714-034410
- Miyamoto J, Kasubuchi M, Nakajima A, et al. Anti-inflammatory and insulin-sensitizing effects of free fatty acid receptors. Handb Exp Pharmacol. 2017;236:221–231. doi:10.1007/164_2016_47
- Hamazaki K, Itomura M, Huan M, et al. Effect of ω-3 fatty acid-containing phospholipids on blood catecholamine concentrations in healthy volunteers: A randomized, placebo-controlled, double-blind trial. Nutrition. 2005;21:705–710. doi:10.1016/j.nut.2004.07.020
- Mozaffarian D, Geelen A, Brouwer IA, et al. Effect of fish oil on heart rate in humans: A meta-analysis of randomized controlled trials. Circulation. 2005;112:1945–1952. doi:10.1161/CIRCULATIONAHA.105.556886
- Miller PE, Van Elswyk M, Alexander DD. Long-chain omega-3 fatty acids eicosapentaenoic acid and docosahexaenoic acid and blood pressure: A meta-analysis of randomized controlled trials. Am J Hypertens. 2014;27:885–896. doi:10.1093/ajh/hpu024
- Yoneshiro T, Aita S, Kawai Y, et al. Nonpungent capsaicin analogs (capsinoids) increase energy expenditure through the activation of brown adipose tissue in humans. Am J Clin Nutr. 2012;95:845–850. doi:10.3945/ajcn.111.018606
- Sugita J, Yoneshiro T, Hatano T, et al. Grains of paradise (Aframomum melegueta) extract activates brown adipose tissue and increases whole-body energy expenditure in men. Br J Nutr. 2013;110:733–738. doi:10.1017/S0007114512005715