ABSTRACT
There is increasing evidence that the global rise in temperature is contributing to the onset of diabetes, which could be mediated by a concomitant reduction in brown fat activity. Brown (and beige) fat are characterised as possessing a unique mitochondrial protein uncoupling protein (UCP)1 that when activated can rapidly generate large amounts of heat. Primary environmental stimuli of UCP1 include cold-exposure and diet, leading to increased activity of the sympathetic nervous system and large amounts of lipid and glucose being oxidised by brown fat. The exact contribution remains controversial, although recent studies indicate that the amount of brown and beige fat in adult humans has been greatly underestimated. We therefore review the potential mechanisms by which glucose could be utilised within brown and beige fat in adult humans and the extent to which these are sensitive to temperature and diet. This includes the potential contribution from the peridroplet and cytoplasmic mitochondrial sub-fractions recently identified in brown fat, and whether a proportion of glucose oxidation could be UCP1-independent. It is thus predicted that as new methods are developed to assess glucose metabolism by brown fat, a more accurate determination of the thermogenic and non-thermogenic functions could be feasible in humans.
KEYWORDS:
There is increasing evidence that the rise in diabetes is partly mediated by the increase in global temperatures over the past 20 years.Citation1,Citation2 This has been observed across the general population in the USACitation3 and, in pregnant women in Canada relative to the onset of gestational diabetes.Citation4 Moreover, the prevalence of gestational diabetes in Canada is higher in the summer and rising ambient temperatures in the 3–4 weeks prior to third trimester glucose tolerance testing can predict gestational diabetes onset.Citation5 Consequently as brown fat is highly sensitive to changes in ambient temperature and is normally activated by cold exposure it would be expected to become less active as temperature rises.Citation6,Citation7 The unique capacity of brown fat to rapidly respond to cold exposure resides within uncoupling protein (UCP)1 that is located on the inner mitochondrial membrane.Citation8 When activated this results in the free flow of protons across the inner mitochondrial membrane,Citation8 thereby bypassing the need to convert ADP to ATP, as occurs in the mitochondria of all other tissues.
Figure 1. Summary of the potential change in glucose utilisation by brown and beige fat between (A) warm and (B) cool ambient temperature increases. Overall the fraction of whole body-glucose utilisation increases in parallel with an increase in the amount of brown and beige fat, but this is lower in the warm. It is based on calculated estimates of glucose oxidation in adult humans as determined in the cold (e.g.Citation13) or after feeding (e.g.Citation40).
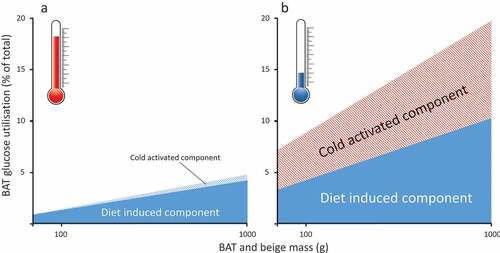
Figure 2. Summary of the potentially different responses between the peridroplet and cytoplasmic mitochondrial fractions within brown (and beige) fat to oxidative metabolism in response to diet or cold-exposure.
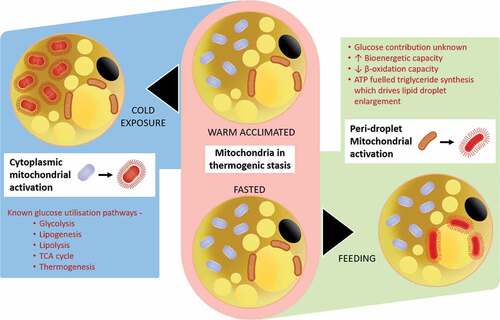
The presence of brown fat is adult humans was originally identified from positron emission tomography-computed tomography (PET-CT) studies in cancer patients,Citation9 and has been confirmed across a range of ethnicities including Caucasian,Citation6 AsianCitation10 and AfricanCitation11 populations. This technique is dependent on subjects showing an increase in radio-labelled glucose uptake within their brown fat, a response that can be modulated by season and sensitivity to cold.Citation12 Consequently the extent to which environmentally induced changes in brown fat function can impact on glucose homeostasis remains a matter of debate.Citation13 It should be noted that with repeated PET-CT scans on the same subject then brown fat is identifiable in most, if not all, adults,Citation14 and comparable quantification of brown fat has been shown between PET-CT and thermal imaging.Citation15 Consequently, it is likely that brown fat is present in all adults,Citation16 and as shown in rodents its temperature fluctuates appreciably over a 24h period.Citation17 The acute sensitivity of brown fat to changes in temperature would thus mean that an overall rise in current global temperature (see https://climate.nasa.gov/vital-signs/global-temperature/) would be sufficient to reduce its activity on a population wide basis. Moreover, if the United Nations report on climate breakdown (see http://www.ipcc.ch/report/sr15/) is not swiftly acted upon then an even greater challenge would present itself.
What is the contribution of brown fat to whole body glucose homeostasis?
The primary factors that determine glucose consumption by brown fat are the total amount of fat, its rate of glucose oxidation and capacity to transport glucose.Citation18 A number of important recent publications have demonstrated that summary estimates appear to substantially underestimate each of these measures. It is therefore highly likely that current calculations suggesting only 1% of total daily glucose utilisation is partitioned across brown fat are inaccurate.Citation13 The total contribution of brown fat should therefore be revised due to the following:
The amount of brown fat in adult humans is routinely underestimated, mainly due to the current imaging techniques and the difficulty in measurement because of the mixing of brown and beige fat with other white fat depots in multiple sites in the body.Citation19 Beige fat is defined as being a discrete region within white fat that possesses UCP1 although at approximately ten-fold lower concentrations than “classic” brown fat,Citation20
Brown fat can be activated by dietCitation21,Citation22 to the same degree as by cold exposure.Citation21 The extent to which these dual activation pathways may be additive is unknown as current studies on cold exposure have been conducted in fasted subjects.
Brown fat shows appreciable metabolic activity in warm ambient temperatures, effects that remain for at least two hours after removal of cold exposure.Citation23
It is now apparent that the total amount of brown and/or beige fat in adult humans could be up to ten-fold higher, even in obese adults.Citation19 This is based on studies that have been able to conduct repeated PET-CT scans of the same individual,Citation14 together with further refinements in image analysis.Citation19 Furthermore, a significant proportion of adipocytes present in brown or beige depots do not appear to be activated by acute cold exposure. Consequently, we suggest that as much as 20% of daily glucose oxidation could be potentially accounted for within brown fat, as a consequence of either diet and/or cold exposure (see ). This is in accord with the recognition that brown fat has a regulatory role in glucose homeostasisCitation18 explaining why cold-induced stimulation of brown fat has the potential to improve glucose metabolism in both leanCitation24 and diabeticCitation25 subjects. Indeed, it has recently been shown in obese adults, that long term caloric restriction sufficient to reduce body weight by 16.5% (primarily due to fat loss) promoted the brown adipocyte content in subcutaneous fat by 10%.Citation26 At the same time, fasting blood insulin and glucose were improved. Furthermore, in humans, brown fat appears to exhibit a glucose responsive biorhythm that is disrupted when the abundance of brown fat is low.Citation27
Is glucose metabolism by brown fat independent of UCP1 mediated thermogenesis?
Glucose utilisation within UCP1-containing adipocytes in brown and beige fat can occur independently of UCP1-mediated thermogenesis.Citation18 This would explain the observation of substantial glucose present in brown fat depots,Citation28 and its appreciable utilisation even at thermoneutrality.Citation23 Glucose present within brown fat could act, in part, as a reserve to be utilised during cold exposure, as the amount of glucose taken up within supraclavicular brown fat, for example, is closely associated with cold-induced thermogenesis.Citation28 Cold exposure is also likely to be accompanied by increased uptake of triglycerides which, in murine obesity models, results in improved glucose homeostasis and up to a five-fold rise in glucose uptake within interscapular brown fat.Citation29 If triglyceride uptake is inhibited pharmacologically, then the uptake of glucose by brown fat is greatly reduced whereas, in other tissues such as skeletal muscle, it is unaffected.Citation30 Gene deletion studies in mice indicate an increasing number of pathways which can restrict glucose uptake by brown fat.Citation18 These appear to be linked to glucose transporter 4, e.g. the GAP complex RalGAP which, when inactivated, results in a seven-fold rise in glucose uptake by brown fat.Citation31 It is likely that other pathways are involved and that these may differ between brown and beige adipocytes. For example, deletion of endonuclease G is associated with increased expression of thermogenic genes in beige, but not brown, adipocytes.Citation32 This, is turn, is accompanied with improved glucose homeostasis and reduced white fat mass. Indeed, multiple pathways are involved and extend to a wide range of signalling molecules as identified in mice e.g. DJ-1,Citation33 although these need confirming in humans.
Two types of brown fat mitochondria and their differential roles in energy balance
The concept that the regulation of UCP1 differs between brown and beige adipocytes and that the utilisation of glucose by these different cell populations requires further investigation. Glucose oxidation by beige fat has been shown to be independent of UCP1 and is, therefore, non-classical.Citation34 The potential divergence in mitochondrial function between dietary and cold-induced thermogenesis could be partly explained by the recent discovery that brown fat contains two different types of mitochondria i.e. the peridroplet and cytoplasmic mitochondrial sub-fractions.Citation35 It has been suggested that these fractions are functionally different in their bioenergetic capacity and fatty acid oxidation despite both possessing UCP1. One potential consequence is that there is a greater recruitment of lipid-droplets within the peridroplet mitochondrial domain after feeding,Citation35 and perhaps cytoplasmic mitochondria are dominant with cold exposure (see ). Such an adaptation to feeding would be in accord with the diurnal rhythm in brown fat activity seen in mice, which is consistent with a lower postprandial lipid response, in the morning compared to evening in humans.Citation36 The fundamentally different processes between the peridroplet and cytoplasmic mitochondrial sub-fractionsCitation35 have yet to be examined in different human disease states. These types of investigations could determine whether glucose metabolism differs between each domain. They could also start to explain the recent demonstration of considerable heterogeneity in nutrient, including glucose uptake by brown adipocytes.Citation37
Future research on the role of brown and/or beige adipocytes on glucose homeostasis
Given the increasing evidence that brown and/or beige fat has a role in both dietary and cold-induced thermogenesis, more focus is now required on the impact of diet especially under thermoneutral conditions.Citation38 A combined effect of diet and cold exposure could therefore herald ground-breaking approaches to diabetes prevention and/or treatment. The urgent need to make such an intervention is high-lighted by the continued rise in global temperatures, and the increased duration of “summer” (see https://www.metoffice.gov.uk/binaries/content/assets/mohippo/pdf/uk-climate/state-of-the-uk-climate/soc_supplement-002.pdf) which currently appear to be largely unpreventable. Moreover, the impact of ageing needs to be considered as this is accompanied with a “natural” decline in brown fat mass, which could underpin the onset of type 2 diabetes.Citation39 Critically, more sophisticated assessments (including the potential use of glucose tracers) to accurately assess glucose uptake by brown adipose tissue and of UCP1, both in vivo and in vitro, are required to enable a more accurate partitioning of its thermogenic and non-thermogenic functions.
Disclosure statement
No potential conflict of interest was reported by the authors.
References
- Symonds ME, Aldiss P, Dellschaft N, Law J, Fainberg HP, Pope M, Sacks H, Budge H. Brown adipose tissue development and function and its impact on reproduction. J Endocrinol. 2018;238(1): R53–R62.
- Ruiz JR, Martinez-Tellez B, Sanchez-Delgado G, Osuna-Prieto FJ, Rensen PCN, Boon MR. Role of human brown fat in obesity, metabolism and cardiovascular disease: strategies to turn up the heat. Prog Cardiovasc Dis. 2018;61(2):232–245.
- Blauw LL, Aziz NA, Tannemaat MR, Blauw CA, de Craen AJ, Pijl H, Rensen PC. Diabetes Incidence and glucose intolerance prevalence increase with higher outdoor temperature. BMJ Open Diabetes Res Care. 2017;5(1): e000317.
- Booth GL, Luo J, Park AL, Feig DS, Moineddin R, Ray JG. Influence of environmental temperature on risk of gestational diabetes. Cmaj. 2017;189(19): E682–E689.
- Retnakaran R, Ye C, Kramer CK, Hanley AJ, Connelly PW, Sermer M, Zinman B. Impact of daily incremental change in environmental temperature on beta cell function and the risk of gestational diabetes in pregnant women. Diabetologia. 2018;61: 2633–2642.
- Au-Yong ITH, Thorn N, Ganatra R, Perkins AC, Symonds ME. Brown adipose tissue and seasonal variation in humans. Diabetes. 2009;58(11):2583–2587.
- Cypess AM, Lehman S, Williams G, Tal I, Rodman D, Goldfine AB, Kuo FC, Palmer EL, Tseng Y-H, Doria A, et al. Identification and importance of brown adipose tissue in adult humans. N Engl J Med. 2009;360(15):1509–1517.
- Cannon B, Nedergaard J. Brown adipose tissue: function and physiological significance. Physiol Rev. 2004;84(1):277–359.
- Matsushita M, Yoneshiro T, Aita S, Kameya T, Sugie H, Saito M. Impact of brown adipose tissue on body fatness and glucose metabolism in healthy humans. Int J Obes (Lond). 2014;38(6):812–817.
- Bakker LE et al. Brown adipose tissue volume in healthy lean south asian adults compared with white caucasians: a prospective, case-controlled observational study. Lancet Diabetes Endocrinol. 2014;2(3): 210–217.
- Perkins AC, Mshelia DS, Symonds ME, Sathekge M. Prevalence and pattern of brown adipose tissue distribution of 18f-Fdg in patients undergoing Pet-Ct in a subtropical climatic zone. Nucl Med Commun. 2013;34(2):168–174.
- Cypess AM, Haft CR, Laughlin MR, Hu HH. Brown fat in humans: consensus points and experimental guidelines. Cell Metab. 2014;20(3):408–415.
- Carpentier AC, Blondin DP, Virtanen KA, Richard D, Haman F, Turcotte EE. Brown adipose tissue energy metabolism in humans. Front Endocrinol (Lausanne). 2018;9: 447.
- Gerngross C, Schretter J, Klingenspor M, Schwaiger M, Fromme T. Active brown fat during 18F-FDG PET/CT imaging defines a patient group with characteristic traits and an increased probability of brown fat redetection. J Nucl Med. 2017;58(7): 1104–1110.
- Law, J., et al. Thermal imaging is a noninvasive alternative to PET/CT for measurement of brown adipose tissue activity in humans. J Nucl Med. 2018;59: 516–522.
- Law J, Chalmers J, Morris DE, Robinson L, Budge H, Symonds ME. The use of infrared thermography in the measurement and characterization of brown adipose tissue activation. Temp. 2017; 1–15.
- Ootsuka Y, de Menezes RC, Zaretsky DV, Alimoradian A, Hunt J, Stefanidis A, Oldfield BJ, Blessing WW. Brown adipose tissue thermogenesis heats brain and body as part of the brain-coordinated ultradian basic rest-activity cycle. Neuroscience. 2009;164(2):849–861.
- Hankir MK, Klingenspor M. Brown adipocyte glucose metabolism: a heated subject. EMBO Rep. 2018;19:9.
- Leitner BP, Huang S, Brychta RJ, Duckworth CJ, Baskin AS, McGehee S, Tal I, Dieckmann W, Gupta G, Kolodny GM, et al. Mapping of human brown adipose tissue in lean and obese young men. Proc Natl Acad Sci U S A. 2017;114(32):8649–8654.
- Nedergaard J, Cannon B. Ucp1 Mrna does not produce heat. Biochemi Biophys Acta. 2013;1831(5):943–949.
- Din, M. U., et al. Postprandial oxidative metabolism of human brown fat indicates thermogenesis. Cell Metab. 2018.
- Scotney H, Symonds ME, Law J, Budge H, Sharkey D, Manolopoulos KN. Glucocorticoids modulate human brown adipose tissue thermogenesis in vivo. Metabolism. 2017;70: 125–132.
- Leitner BP, Weiner LS, Desir M, Kahn PA, Selen DJ, Tsang C, Kolodny GM, Cypess AM. Kinetics of human brown adipose tissue activation and deactivation. Int J Obesity. 2018. doi:10.1038/s41366-018-0104-3.
- Iwen KA, Backhaus J, Cassens M, Waltl M, Hedesan OC, Merkel M, Heeren J, Sina C, Rademacher L, Windjäger A, et al. Cold-induced brown adipose tissue activity alters plasma fatty acids and improves glucose metabolism in men. J Clin Endocrinol Metab. 2017;102(11):4226–4234.
- Hanssen MJW, Hoeks J, Brans B, van der Lans AAJJ, Schaart G, van Den Driessche JJ, Jörgensen JA, Boekschoten MV, Hesselink MKC, Havekes B, et al. Short-term cold acclimation improves insulin sensitivity in patients with type 2 diabetes mellitus. Nat Med. 2015;21(8):863–865.
- Perdikari A, Leparc GG, Balaz M, Pires ND, Lidell ME, Sun W, Fernandez-Albert F, Müller S, Akchiche N, Dong H, et al. Batlas: deconvoluting brown adipose tissue. Cell Rep. 2018;25(3):784–97 e4.
- Lee P, Bova R, Schofield L, Bryant W, Dieckmann W, Slattery A, Govendir MA, Emmett L, Greenfield JR. Brown adipose tissue exhibits a glucose-responsive thermogenic biorhythm in humans. Cell Metab. 2016;23(4):602–609.
- Weir, G., et al. Substantial metabolic activity of human brown adipose tissue during warm conditions and cold-induced lipolysis of local triglycerides. Cell Metab. 2018;27:1348–1355.e4.
- Bartelt A, Bruns OT, Reimer R, Hohenberg H, Ittrich H, Peldschus K, Kaul MG, Tromsdorf UI, Weller H, Waurisch C, et al. Brown adipose tissue activity controls triglyceride clearance. Nat Med. 2011;17(2):200–205.
- Blondin DP, Frisch F, Phoenix S, Guérin B, Turcotte ÉE, Haman F, Richard D, Carpentier AC. Inhibition of intracellular triglyceride lipolysis suppresses cold-induced brown adipose tissue metabolism and increases shivering in humans. Cell Metab. 2017;25(2):438–447.
- Skorobogatko Y, Dragan M, Cordon C, Reilly SM, Hung C-W, Xia W, Zhao P, Wallace M, Lackey DE, Chen X-W, et al. Rala controls glucose homeostasis by regulating glucose uptake in brown fat. Proc Natl Acad Sci U S A. 2018;115(30):7819–7824.
- Pardo R, Blasco N, Vilà M, Beiroa D, Nogueiras R, Cañas X, Simó R, Sanchis D, Villena JA. Endog knockout mice show increased brown adipocyte recruitment in white adipose tissue and improved glucose homeostasis. Endocrinology. 2016;157(10):3873–3887.
- Wu R, Liu X-M, Sun J-G, Chen H, Ma J, Dong M, Peng S, Wang J-Q, Ding J-Q, Li D-H, et al. Dj-1 maintains energy and glucose homeostasis by regulating the function of brown adipose tissue. Cell Discov. 2017;3:16054.
- Ikeda K, Kang Q, Yoneshiro T, Camporez JP, Maki H, Homma M, Shinoda K, Chen Y, Lu X, Maretich P, et al. Ucp1-independent signaling involving serca2b-mediated calcium cycling regulates beige fat thermogenesis and systemic glucose homeostasis. Nat Med. 2017;23(12):1454–1465.
- Benador, I. Y., et al. mitochondria bound to lipid droplets have unique bioenergetics, composition, and dynamics that support lipid droplet expansion. Cell Metab. 2018;27(4): 869–885.e6.
- van Den Berg R, Kooijman S, Noordam R, Ramkisoensing A, Abreu-Vieira G, Tambyrajah LL, Dijk W, Ruppert P, Mol IM, Kramar B, et al. A diurnal rhythm in brown adipose tissue causes rapid clearance and combustion of plasma lipids at wakening. Cell Rep. 2018;22(13):3521–3533.
- He, C., et al. Nanosims imaging reveals unexpected heterogeneity in nutrient uptake by brown adipocytes. Biochem Biophys Res Commun. 2018;504:899–902.
- Symonds ME, Aldiss P, Pope M, Budge H. Recent advances in our understanding of brown and beige adipose tissue: the good fat that keeps you healthy. F1000Res. 2018;7.
- Blondin DP, Labbé SM, Noll C, Kunach M, Phoenix S, Guérin B, Turcotte ÉE, Haman F, Richard D, Carpentier AC. Selective impairment of glucose but not fatty acid or oxidative metabolism in brown adipose tissue of subjects with type 2 diabetes. Diabetes. 2015;64(7):2388–2397.
- Gerich JE. Role of the kidney in normal glucose homeostasis and in the hyperglycaemia of diabetes mellitus: therapeutic implications. Diabet Med. 2010;27(2):136–142.