Abstract
There has been a growing interest in the use of B cells for cancer vaccines, since they have yielded promising results in preclinical animal models. Contrary to dendritic cells (DCs), we know little about the migration behavior of B cells in vivo. Therefore, we investigated the interactions between CD40-activated B (CD40B) cells and cytotoxic T cells in vitro and the migration behavior of CD40B cells in vivo. Dynamic interactions of human antigen-presenting cells (APCs) and T cells were observed by time-lapse video microscopy. The migratory and chemoattractant potential of CD40B cells was analyzed in vitro and in vivo using flow cytometry, standard transwell migration assays, and imaging of fluorescently labeled murine CD40B cells. Murine CD40B cells show migratory features similar to human CD40B cells. They express important lymph node homing receptors which were functional and induced chemotaxis of T cells in vitro. Striking differences were observed with regard to interactions of human APCs with T cells. CD40B cells differ from DCs by displaying a rapid migratory pattern undergoing highly dynamic, short-lived and sequential interactions with T cells. In vivo, CD40B cells are home to the secondary lymphoid organs where they accumulate in the B cell zone before traveling to the B/T cell boundary. Moreover, intravenous (i.v.) administration of murine CD40B cells induced an antigen-specific cytotoxic T cell response. Taken together, this data show that CD40B cells home secondary lymphoid organs where they physically interact with T cells to induce antigen-specific T cell responses, thus underscoring their potential as cellular adjuvant for cancer immunotherapy.
Introduction
Professional APCs play a crucial role in the induction of T cell immunity. Active cancer immunotherapy aims to achieve tumor control through the induction of a tumor-directed immune response. Due to the potent antigen-presenting function of DCs, DC-based cellular vaccines represent one of the most promising and best-studied immunotherapeutic approaches. The complex cellular interactions between DCs and T cells, which are necessary to induce antitumor T-cell immunity, have been extensively studied.Citation1,2 The insights gained from these experiments permitted a gradual improvement of the design of DC-based cancer vaccines and have finally led to the approval of the first DC-based cancer vaccine.Citation3,4 However, DCs have two major disadvantages concerning the clinical application: first, they are rare in peripheral blood and cannot be expanded ex vivo without laborious efforts and in a sufficiently high purity (>50%). Second, they fail to express CD62L, one of the key molecules for homing to the secondary lymphoid organs. Therefore, less than 5% of injected DCs reach the draining lymph nodes.Citation5
In recent years, there has been a growing interest in the use of B cells for cellular immunotherapy. B cell-based cancer vaccines have yielded promising results in preclinical animal models.Citation6,7 Signaling via CD40 is probably the most potent activation stimulus for B cells. It dramatically enhances their ability to present antigen, to activate T cells, and to induce T-cell proliferation.Citation6,8 CD40B cells are potent APCs and are capable of priming both CD4+ and CD8+ T-cell responses.Citation9,10 They have therefore been studied by several groups as a cellular cancer vaccine.Citation11-13 One advantageous feature of CD40B cells is that they can be generated from small amounts of peripheral blood in large quantities at a relatively low cost, even from cancer patients.Citation13 Furthermore, CD40B cells are resistant to inhibition by many of the tumor factors known to induce immunosuppression, such as PGE2, TGF-β, and IL-10.Citation14,15
Contrary to DCs, we know little about the induction of T cell immunity by B cell-based cancer vaccines. Numerous studies have investigated the nature of T-B cell interactions, but those studies mainly focused in the T cell-dependent humoral immune response.Citation16 How B cells induce CD8+ T cell responses and which differences exist in comparison to DCs is currently not well understood. Furthermore, little is known about the migratory behavior of CD40B cells in vivo. Unlike DCs, it is not the B cells' physiological role to survey peripheral tissue and home to secondary lymphoid organs upon antigen uptake. However, human CD40B cells express the respective factors and receptors crucial for homing to secondary lymphoid organs including CD62L, CCR7/CXCR4, and leukocyte function antigen-1.Citation17 The receptors CCR7 and CXCR4 are functional and induce chemotaxis toward increasing chemokine concentrations of their ligands CCL21 and CXCL12, respectively. Moreover, human CD40B cells express chemokines that induce chemotaxis of T cells, namely CCL17 (TARC), CCL5 (RANTES), CCL22 (MDC), and CXCL10 (IP-10).Citation17,18 Indeed, supernatants of CD40 B cells increase the migration of both CD4+ and CD8+ T cells in standard transwell migrations assays, clearly demonstrating the chemotactic potential of CD40B cells.
A deeper knowledge of the cellular dynamics and cellular encounter between T cell and CD40B cells could provide guidance for the further optimization of B-cell based immunotherapy. Therefore, we investigated the interactions between human CD40B cells and cytotoxic T cells in vitro and the migratory behavior of murine CD40B cells in vivo. Here, we show that CD40B cells efficiently home to the secondary lymphoid organs in vivo where they undergo dynamic and short-lived interactions with T cells that result in the induction of antigen-specific cytotoxic T cell responses.
Results
CD40B cells establish short-lived and dynamic interactions with cognate T cells
To characterize the T-B cell interactions on a single cell level, we analyzed three-dimensional migration in collagen matrix using time-lapse video microscopy. We compared T cell-APC interactions of both resting and human CD40B cells with those of immature and mature human DCs. 1 × 106 CD8+ human T cells of a cyclin D1-specific T-cell clone were embedded within the collagen matrix together with the different APC subsets. Prior to the coculture with cyclin D1-specific T cells, the different APC subsets were pulsed with peptide (). Striking differences between the interactions patterns of DCs or B cells with T cells were observed.
Figure 1. Interactions between CD40B cells and CTLs are short-lived. Cyclin D1-specific T cells were embedded in 3D collagen matrices together with different APCs: resting B cell (B cell), CD40B cells (CD40B), immature (DCimm) and mature (DCmat) DCs. APCs were pulsed with 10 µg/mL of the peptide cyclinD1_228 were indicated. Cell movements were recorded by time-lapse video microscopy and the duration of individual T cell-APC contacts was analyzed. (A) Each dot represents one contact. Bars represent the median. *p < 0.001. Data are pooled from 34 films from 9 independent experiments. (B) The percentage of cell contacts that last longer than 45 min of all contacts are shown. *p < 0.002.
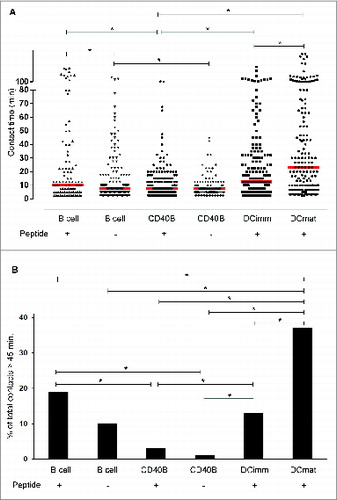
DCs engaged in much longer contacts with T cells than did B cells (; Movie S1). Interestingly, both resting and CD40B cells differ from immature and mature DCs by displaying a rapid migratory pattern undergoing highly dynamic, short-lived, and sequential interactions with cognate T cells (Movies S2–4). On average mature DCs stayed in contact with T cells more than twice as long as resting or CD40B cells. For DCs, we observed a reciprocal relationship between activation status and duration of APC–T-cell contact. Whereas the median contact duration for immature DC−T-cell pairs was 12.5 min, mature DC−T-cell contacts lasted significantly longer with a median contact duration of 23.3 min. T cells predominantly engaged with immature DCs or with mature DCs in a short-lived manner but they additionally formed stable long contacts (individual mature DC−T-cell contacts lasting up to >8 h). The percentage of stable contacts (>45 min) was significantly higher in mature DCs than in immature DCs (). T cells crawled along the surface of the DCs, and eventually ‘stuck’ to one site and stayed there during the whole contact (Movie S1).
CD40B-T-cell interactions were transient and short-lived, lasting only few minutes with median contact duration of 7.5 min (; Movies S3–4). The majority of interactions between unstimulated B cells and T cells were also short-lived with a median duration of 10 min, but significantly longer than the contact time between CD40B cells and T cells (7.5 min). Whereas in DCs the duration of contact seemed to be reciprocal with APC maturation, the correlation of APC activation and contact duration in B cells appeared to be inverse. When comparing the percentage of stable contacts (>45 min), the proportion of long-lived contacts was significantly higher in unstimulated B cells than CD40B cells (). The analysis of cellular movements revealed that unstimulated B-T cell pairs were often motile. An unstimulated B cell typically positioned itself at the leading edge of an elongated T cell (Movie S2). Occasionally, unstimulated B cells engaged more than one T cell. CD40B cells displayed a rapid migratory pattern. CD40B -T cell pairs were more motile than unstimulated B-T cell pairs frequently changing the orientation of their movement. These observations indicate that the binding force between T cells and B cells is high enough to overcome considerable shear forces imposed on migration by the tight collagen network. A CD40B cell typically established contact with two T cells and up to four simultaneously (Movie S4). CD40B cells appeared to have a DC-like morphology and motility in 3D time-lapse video microscopy. This is supported by flow cytometric analysis revealing that CD40B cells were much larger than unstimulated B cells (data not shown). T cells crawled on the larger surface of the CD40B cells similarly to the way they did along the DC surface (Movies S1 and 3).
Contrary to previous reports, which demonstrated that peptide-loaded resting murine B cells maintain long-lived interactions with CD4+ T cellsCitation19, we found that on average peptide-pulsed resting human B cells form primarily short-lived contacts with antigen-specific T cells (median contact duration of 10 min). The median contact duration remained almost unchanged for unstimulated B cells engaging T cells in the presence (10 min) or absence of antigen (7.5 min). Moreover, there was no difference for CD40B cells pairing with T cells in the presence (7.5 min) or absence of antigen (7.5 min) ().
Murine CD40B cells express functional receptors crucial for homing to secondary lymphoid organs
For in vivo experiment, we used the established mouse model, in which analogous to the human culture system murine B cells can be activated and expanded by culture on CD40L-expressing feeder cells.Citation20,21 To characterize the migration behavior of CD40B cells, we determined whether murine CD40B cells show comparable migratory and chemotactic characteristics compared to human CD40B cells in vitro.Citation17 First, we analyzed the expression of surface molecules involved in B-cell migration and T-cell attraction. The unstimulated B cell population consisted of more than 95% B220+ B cells (), which highly expressed CD62L and CXCR5 but only low or no CCR7 or CXCR4 (). Following CD40L stimulation (7 d) (), B cells upregulated the activation markers I-Ab, CD80, and CD86 which is consistent with APC functionality. CD40B cells moderately downregulated CD62L and CXCL5 expression, while up-regulating the expression of both CXCR4 and CCR7 ().
Figure 2. CD40B cells express crucial molecules for homing in to secondary lymphoid organs. Purified B cells were stimulated with tmuCD40L HeLa in the presence of rmIL-4. After 3 d, cells were stimulated with fresh CD40L and rmIL-4. (A and B) The B cells were phenotyped for the indicated molecules before stimulation with CD40L and (C) on day 7 of culture. Expression on cells gated for B220+ CD3− is presented in the histograms. Shaded histograms represent staining with isotype matched control antibodies; black lines staining with specific antibody. One representative experiment out of 9 is shown.
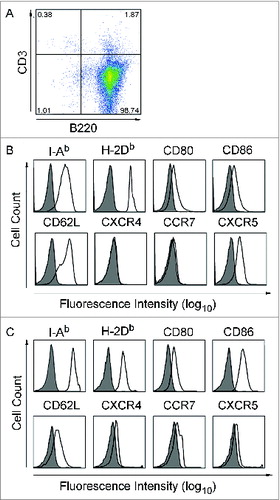
This cytokine expression pattern suggests a migration potential of CD40B cells toward CCL21, CXCL12, and CXCL13 (summarized in ).Citation22,23 To assess the functionality of CCR7, CXCR4, and CXCR5 expressed on murine CD40B cells, standard migration assays were performed. 5 × 105 B cells were transferred to the upper chamber of a transwell culture insert. Cells that migrated through the transwell membrane toward the respective ligands were counted (). Migration of CD40B cells was induced by all three chemokines tested: CCL21, a chemokine expressed in the T cell zones of secondary lymphoid organs and the ligand for CCR7; CXCL12, the ligand for CXCR4; and CXCL13, a chemokine made in B cell zones of lymphoid organs and the ligand for CXCR5.Citation22 CD40-activation significantly increased migration of B cells toward these chemokines when compared to unstimulated B cells. The migration of CD40B cells was dose-dependent. Unexpectedly, unstimulated B cells migrated in significant higher numbers toward CCL21 or CXCL12 than toward CXCL13. Directed migration of CD40B cells toward CXCL12 along a gradient was also demonstrated in 3D collagen gel, although the velocity of the cells was unusually slow (Fig. S1).
Figure 3. Chemokines involved in lymphocyte positioning in the secondary lymphoid organs. CCL21/ CCL19, chemokines expressed in the T-cell zones of secondary lymphoid organs and the ligand for CCR7 (A); CXCL12, the ligand for CXCR4 mostly involved in T-cell zone entry (B); and CXCL13, a chemokine made in B-cell zones of lymphoid organs and the ligand for CXCR5 (C).Citation22,23 The chemokine expression pattern of CD40B cells (D) allows them to migrate toward the B/T cell boundary (E).
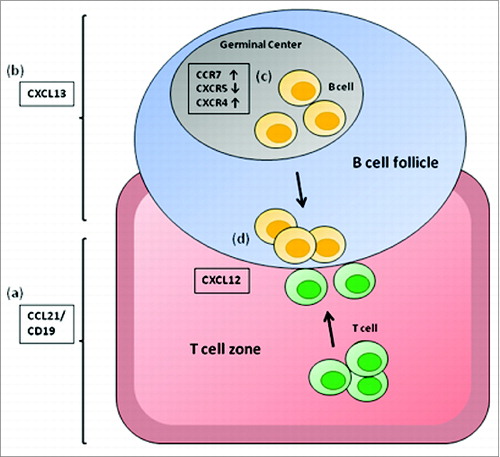
Figure 4. B cell migrate toward homing chemokines and attract T cells in vitro. (A) Unstimulated CD19+ B cells (gray columns) and CD40B cells (black columns) were tested for migration toward CCL21, CXCL12, and CXCL13. CD40B medium alone was used to record spontaneous migration (NC). Cells were allowed to migrate for 3 h through a 5 μm filter transwell assay. (B) Purified CD4+ T cells were allowed to migrate for 2 h through a 5 μm filter to the lower chamber of transwell plates containing supernatant from murine CD40B cells, the chemokine CXCL12 (SDF1a) or CD40B medium alone (NC). Mean values ± SD from 3 experiments are shown. * p < 0.05, ** p < 0.01.
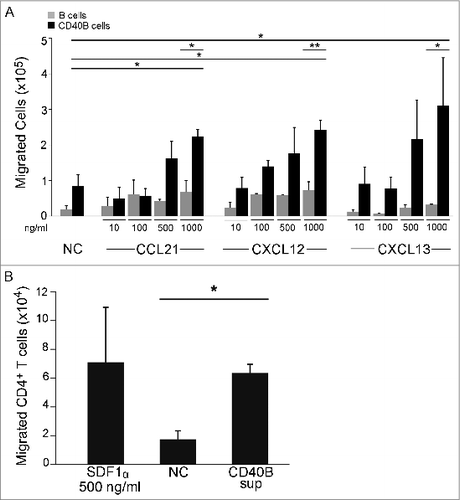
To determine whether murine CD40B cells attract T cells, filter migration assays were conducted (). Purified CD4+ T cells were transferred to the upper chamber and allowed to migrate for 2 h toward supernatant from murine CD40B cells, which was added to the lower chamber. We used CXCL12 (500 ng/mL) and muCD40 B medium as positive and negative controls, respectively. When supernatant from CD40B cell cultures was added to the lower chamber, T cell migration increased 2.6 fold above background, indicating that CD40B cells secret T cell-attracting chemokines.
CD40B cells home to secondary lymphoid organs in vivo
Little is known about the in vivo migration of activated B cells. In order to assess their migration kinetics, we injected CD40B cells generated from luciferase+ or GFP-transgenic mice into C57BL/6 mice via different routes and tracked their location after different time periods. B cell from luciferase+ or GFP-transgenic mice could be stimulated with CD40L without affecting their autofluorescence. Moreover, these cells displayed the typical homing and APC phenotype (data not shown). Administration routes were chosen to assess the following biological and clinical aspects: first, possible paths of homing to peripheral lymph nodes, lymph or blood vessels; and second, their relevance for clinical application. First, the general migration of CD40B cells after i.v. or subcutaneous (s.c.) injection was assessed by injecting 5 × 106 luciferase+ CD40B cells into C57BL/6 mice. s.c. injection did not result in migration to the secondary lymphoid organs at any of the observed time points (, right column). However, after i.v. injection the luciferase+ CD40B cells appeared in the spleen within 12 h and over the period of 5 d migrated to the abdominal lymph nodes (, left column). Luciferase+ CD40B cells could be detected in the abdominal lymph nodes for up to 15 d (data not shown). Cell positioning in vivo was shown to be determined by the balance of responsiveness to chemoattractants produced and released in separate but adjacent zones.Citation24 To determine cell positioning of CD40B cells in the spleen, we analyzed cryosections of spleens after injection of up to 10 × 106 GFP+ CD40B cells into C57BL/6 mice by immunofluorescence. In accordance with their increased expression of CCR7 and decreased expression of CXCR5, we found that 12 h after i.v. injection GFP+ CD40B cells accumulate in the B-cell zone of the splenic white pulp and to a lesser extend at the B/T cell boundary close to the T-cell zone (, upper panel). About 2% of GFP+ CD40B cells were found in the T-cell zone. Over a time period of 5 d the percentage of CD40B cells found in the T cell zone increased to 4%. Moreover, we observed an increase of CD40B cells at the B/T cell border. In contrast, very few GFP+ CD40B cells could be detected in secondary lymphoid organs during the 5 d after s.c. injection. By counting the number of GFP+ CD40B cells in cryosections of spleen and lymph nodes, the results of the luciferase experiments were confirmed (, lower panel). The number of CD40B cells in the spleen after i.v. injection increased with time from 75.6 ± 10 GFP+ cell/mm2 12 h after cell transfer to 128 ± 28.8 GFP+ CD40B cells 5 d after cell transfer. In contrast to the luciferase+ CD40B cells, 5 d after s.c. injection GFP+ CD40B cells were also detectable in the spleen. However, the number of cells when injected s.c. was significantly lower than after i.v. delivery.
Figure 5. CD40B cells migrate to spleens and lymph nodes in healthy and tumor-bearing mice. 5–10 × 106 CD40B cells from luciferase+ mice (A and C) or GFP+ mice (B) were injected into C57BL/6 mice subcutaneously (s.c.) or intravenously (i.v.). 12 hours (12 h), 36 hours (36 h) or 5 days (5 d) after injection, mice were analyzed for the presence of the CD40B cells either by imaging in the IVIS 200 system in healthy mice (A) or EL4 tumor-bearing mice (C). Cy3 stained to detect all B220+ B cells (red); GFP+ CD40B cells (green) by confocal microscopy (B, upper panel). Representative pictures out of 3 experiments are shown. The number of GFP+ CD40B cells was determined at the indicated time points (B, lower panel). Mean values from one representative experiment are shown; n = 2 mice per time point and condition from each independent experiment.
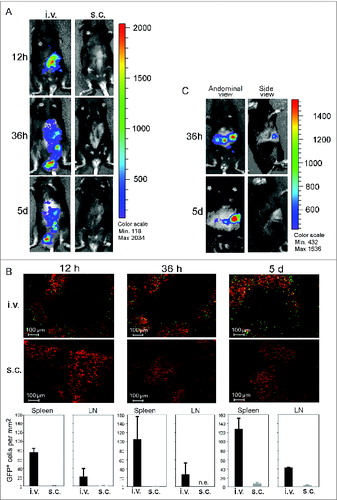
To assess whether the homing of CD40B cells to secondary lymphoid organs is affected by the presence of a tumor we tracked the migration of luciferase+ CD40B cells following i.v. injection into C57BL/6 mice which have been inoculated with EL4 cells. During the 5 d after injection we did not observe any effects of the tumor on the distribution of CD40B cells after injection. In EL4 tumor-bearing mice, luciferase+ CD40B cells showed the same migration behavior as in healthy mice by traveling to the spleen and the lymph nodes. Importantly, CD40B cells also migrated to the tumor draining lymph nodes, the site where they are most likely to encounter tumor antigen (, left column).Citation25 No luciferase+ CD40B cells showed up in the tumor (, right column).
CD40B cells induce a cytotoxic T cell response in vivo
Finally, we confirmed that CD40B cells are indeed superior APCs compared to non-activated B cells by performing in vivo cytotoxicity assays. C57BL/6 mice were immunized i.v. three times in an interval of 7 d with 5 × 106 peptide-pulsed CD40B cells, peptide-pulsed B cells or peptide plus CpG in incomplete Freund's adjuvant as positive control. On day 21, CFSE+ peptide-pulsed target cells were injected into immunized mice. The in vivo cytolytic activity of antigen-specific CD8+ T cells was determined by calculating the specific lysis from the ratio of target cells in spleens. The specific lysis of immunized mice was normalized to the specific lysis of negative controls. The antigen-specific response was significantly higher in mice that were immunized with CD40B cells (23.55% ± 3.7) than in mice that were immunized with non-activated B cells (15.32 ± 0.9) ().
Figure 6. CD40B induce specific lysis of target cells. C57BL/6N mice were immunized with 5 × 106 APCs, i.e., CD40B cells (CD40Bs) or B cells, PBS+IFA as negative controls (NC), or incomplete Freund's adjuvant + PBS + OVA-Protein + CpG as positive controls (PC) three times i.v. in an interval of 7 d The ratio of CFSE+ target cells in spleens was determined by FACS and the specific lysis was calculated by normalizing values to negative controls. Bar charts show mean values ± SD of specific lysis of one independent experiments with three mice per group. Significant differences calculated with ordinary one-way ANOVA are marked by an asterisk. * p ≤ 0.05.
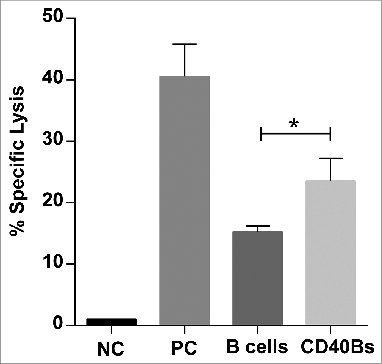
Discussion
The scientific community has focused on understanding the APC function of DCs, since they are considered to be the most potent professional APCs. As a consequence, we know less about antigen presentation by other professional APCs, i.e., B cells and macrophages, and how these differences affect interactions with T cells. We therefore studied the cellular dynamics of the encounter between CD40B cells and T cells, which is essential for further refinement of a CD40B-cell based immunotherapeutic approach.
In order to induce an efficient T cell response APCs must physically encounter T cells that recognize their cognate antigen. The secondary lymphoid organs provide the optimal microenvironment to facilitate the complex processes required for productive interaction between APCs and T lymphocytes. Our in vivo migration experiments show for the first time that CD40B cells specifically home to the secondary lymphoid organs. After adoptive transfer CD40B cells were detected in high numbers in peripheral lymph nodes and in spleen of recipient mice. This finding implies a clear advantage of CD40B cells over DCs, which remain predominantly at the injection site. One of the main disadvantages of using DCs as a cellular adjuvant, is their lack of CD62L expression and the resulting impaired homing to the secondary lymphoid organsCitation26 Following intradermal injection only few DCs migrate to the draining lymphoid tissue, i.e., ˜1% in mice and humans.Citation5,27 Our work demonstrates that with regard to CD40B-based vaccination the i.v. route is superior to the s.c. route. CD40B cells that were injected s.c. could not be detected at any of the observed time points, which indicates that the cells did not migrate to the secondary lymphoid organs. In contrast to CD40 B cells, it has been shown that murine DC migration to lymph nodes is very low after i.v. injection.Citation28 Moreover, most reports that examined homing of s.c. injected DCs both in mice and humans found it to be largely restricted to the draining lymph nodes; and homing of i.v. injected DCs to be restricted to spleen and non-lymphoid tissues.Citation28-32
Once they reach the secondary lymphoid organs naive T cells primarily locate to the T-cell zones or interfollicular areas whereas naive B cells can be found in the B-cell zones or follicular areas. Colocalization of APCs and antigen-specific T cells within the specialized architecture of the secondary lymphoid organs is a critical prerequisite for mounting an effective T cell response. Following entry into the lymph nodes B cells need to position themselves at the B-T cell boundary of the lymphoid organs. Our data demonstrate that CD40B cells display a pattern of chemokine receptors that enables them to migrate to the B-T-cell boundary where they can engage with T cells. The balance between CCR7- and CXCR5-mediated signals determines the localization of B cells within secondary lymphoid organs.Citation24,33 CCR7 is the receptor for CCL19 and CCL21, which are both produced in the T-cell zone (), and CXCR5 is the receptor for CXCL13, which is made in the B-cell zone (). The responsiveness to CCL19/CCL21 and CXCL13 determines the exact positioning of B cells within the lymphoid organs. CD40 activation of B cells induced an upregulation of CCR7, while downregulating CXCR5 (). This chemokine receptor expression pattern allows CD40B cells to move to the T/B cell boundary of lymphoid follicles, where they are optimally located to encounter their cognate T cells ().Citation34 Interestingly, chronically autoantigen engaged B cells localize to the B/T cell boundary owing to reduced CXCR5 expression.Citation35 This fact supports the idea that the balance of responsiveness to the B-cell vs. T-cell zone is crucial. Contrary to CD40B cells, unstimulated B cells displayed high amounts of CXCR5 and very low levels of CCR7 on their surface, thus indicating that they might preferentially localize in the B cell follicles. In addition, migration assays confirmed that the homing molecules on CD40B cells are functional and that CD40B cells are able to induce chemotaxis by T cells. Our experiments show that murine CD40B-cell induce migration of murine CD4+ T cells at similar levels as human CD40B cells.Citation17 This finding further indicates that CD40B cells could recruit T cells for interaction.
The chemokine receptor expression pattern of the resting B cells and CD40B cells correlated with their migration in vivo. In the case of unstimulated B cells, 100% of the cells localized in the B-cell follicles, whereas in the case of CD40B cells about one-tenth of the cells localized at the edge of the T-cell zone, thus suggesting that CD40B cells are able to move to the B/T cell boundary to interact with T cells arising from the T cell zone. Moreover, about 2% of the CD40B cells were found within the T-cell zone. Five days after cell transfer, the percentage of CD40B cells found in the T-cell zone increased to 4% and the number of CD40B cells that gathered in the B/T cell boundary also increased. Thus, we were able to support our initial hypothesis that CD40B cells move toward the T-cell zone. In accordance with our findings, Reif and colleagues described that B cells that encountered antigen in the T-cell distal region of the follicle, rapidly upregulated CCR7, while sustaining their expression of CXCR5.Citation24 This change caused the antigen-engaged B cells to move to the B/T cell boundary in response to CCL21 to interact with activated helper T cells when initiating adaptive immune responses.Citation34 The fact that CD40B cells move toward the B-zone/T-zone boundary is of great interest as this is the site at which possible interaction with naive T cells and activation of antigen-specific T cells occurs.
The consequences of the encounter between an antigen-specific T cell and an APC that presents the cognate antigen is subsequently determined by spatiotemporal coordination their physical cell–cell interactions. Thus far, the kinetics of these interactions has mostly been studied using murine cells. In this report we compared the interactions between different human APCs, i.e., immature/mature DC or unstimulated/CD40B cells, and human antigen-specific T cells. We studied cognate interactions between different APCs and antigen-experienced T cells and found that human mature DC established both short-lived and long-lived interactions with human antigen-specific T cells. The presence of very long contacts between DC and T cells (individual mature DC−T-cell contacts lasting up to >8 h) was unexpected and in contrast with previous work in a collagen matrix model.Citation36-38
In our study, the majority of the duration of contacts with cognate T cells were short both for resting and activated B cells. In the study of Gunzer et al. the presence of antigen dramatically augmented the duration of contact between B cells and T cells.Citation19 In contrast, in our study, antigen on B cells did not substantially affect the contact duration. Although at the level of statistical significance, the differences were rather small. The reason for this difference could, however, result from the different antigen concentration used to pulse the APCs. In the aforementioned study the authors used 100 μg/mL ovalbumin to load the APCs, whereas we used 10 μg/mL. Differences in the studied T-cell populations, naive T cells vs. antigen-experienced T cells, could be another reason for this difference.
With regard to the intercellular interaction dynamics of the different APCs with T cells, our data demonstrate that human DCs display a promiscuous behavior. DCs paired with up to five T cells simultaneously. Migrating T cells crawled along the DC surface and the dynamic behavior of both the APCs and T cells did not impede the contact formation. T cells showed similar dynamic behavior when in contact with CD40B cells. Similar to DCs, more than one T cell had contact to a CD40B cell at the same time. These findings are consistent with previous reports using murine cells.Citation36 In contrast, unstimulated B cells typically paired with only one and occasionally with two T cells, which is consistent with in vivo observations by others.Citation34
Earlier studies have postulated that long-term contact is a universal feature of all cognate APC–T-cell interactions.Citation39 More recent studies have challenged the notion of an absolute requirement of stable long-lasting interactions between APC and T cell for T-cell activation.Citation19,40 Our finding that B cells primarily form short-term serial interactions with cognate T cells is in agreement with the latter observations. However, the mechanisms by which such dynamic and sequential interactions foster B-cell activation remains unclear. Cahalan et al. proposed that the high motility and frequent B/T cell partner exchange may enhance T/B cell cooperation and thereby improve the likelihood of effective T-cell help. Moreover, it has recently been reported that dynamic interactions with frequent partner exchange between antigen-presenting B cells and cognate T cells serve to regulate the decision-making process between T cell activation and tolerance induction.Citation41
In summary, our findings provide evidence that following i.v. injection CD40B cells efficiently home to lymph nodes and spleen in vivo, where they move toward the edge of the T-cell zone. Furthermore, our results reveal fundamental differences in how DCs and B cells interact with antigen-specific T cells. Contrary to DCs, peptide-loaded CD40B cells primarily undergo highly dynamic, short-lived, and sequential interactions with cognate T cells. Our results demonstrate that murine CD40B cells have migratory and chemotactic properties in vitro that are similar to those of human CD40B cells. Thus, the murine CD40B system represents a valid experimental mouse model to study the mechanisms of CD40B-based cancer immunotherapy in vivo. In the murine model, we discovered that CD40-activated home most efficiently to the secondary lymphoid organs following i.v. injection. This finding is of clinical relevance, because it suggests that future clinical trials of B-cell based tumor vaccination should favor the i.v. route of administration. Taken together, this data underscore the potential of CD40B cells as a cellular platform for cancer immunotherapy.
Materials and Methods
Blood samples and mice
Buffy coat preparations were obtained from healthy donors at the blood bank of our institution. The donors gave their consent and all the experiments were approved by our institutional ethical board. C57BL/6 mice were obtained from Harlan Laboratories (Borchen, Germany). DeleterCre/GFP transgenic mice (on C57BL/6 background) were kindly provided by Prof. Dr. Jens Brüning (Laboratory of Mouse Genetics and Metabolism, Institute for Genetics, University of Cologne, Germany). Luciferase+ BL6 mice were kindly provided by Prof. Dr. Robert Zeiser (Laboratory for Allo-Immunregulation, Department for Internal Medicine I, University Hospital Freiburg). Mice were housed under specific pathogen-free conditions and all animal experiments were approved by the Cologne regional animal care committee.
Human peripheral blood mononuclear cells (PBMCs) or murine splenocytes were isolated using Ficoll/Paque PLUS (GE Healthcare Life Science, 17-1440-02) density-gradient centrifugation. B cells were enriched using positive immunomagnetic selection with human or murine CD19 microbeads (Miltenyi Biotec, 130-050-301 and 130-052-201) according to manufacturer's protocol. Murine T cells were purified by positive selection using CD4+ MACS beads (Miltenyi, Bergisch Gladbach, Germany). Human Cyclin D1-specific T cell clones were generated as described previously.Citation42
Generation of murine and human CD40B cells
Human and murine B cells were activated and expanded using the established system of adherent murine and human epithelial cell lines expressing human or murine CD40L, respectively. NIH3T3/tCD40L and tmuCD40L HeLa feeder cells were kindly provided by Gordon Freeman (DFCI, Boston, USA) and Professor Clemens Wendtner (Klinikum Schwabing, Munich, Germany), respectively. Cell culture was performed as described previouslyCitation20,21. Briefly, CD19+ B cells or PBMCs were seeded at a density of 1 × 106 cell/mL on lethally irradiated feeder cells. Cell passaging of feeder cells was done twice per week. Human B cells were cultured in Iscove's modified Dulbecco medium (Life Technologies, 21980-065), supplemented with 10% heat-inactivated human AB serum, 5 µg/mL insulin, 15 µg/mL gentamicin. 50 U/mL of recombinant human IL-4 (Immunotools, 11340045) were freshly added. Murine B cells were cultured in RPMI 1640 medium (Life Technologies, 21875-091) supplemented with 580 µg/mL Glutamine, 10% Fetal-bovine serum, 1% HEPES, 1% MEM, and 15 µg/mL gentamicin. 50 U/mL of recombinant murine IL-4 (Immunotools, 12340042) were freshly added. After 2–3 weeks in culture the CD40B cells had a purity of >95%.
Generation of immature and mature DCs
DCs were generated from blood-derived monocytes as described previously.Citation43 Briefly, PBMCs were isolated from buffy coats by Pancoll density-gradient centrifugation (Pan Biotech, P04-60500). Monocytes were subsequently enriched by CD14 MicroBeads (Miltenyi Biotec, 130-050-201) and purified CD14+ monocytes (purity 95%–98%) were cultured in serum-free CellGro DC medium (CellGenix, 20801–0500) supplemented with 800 U/mL GM-CSF and 500 U/mL IL-4 (both Immunotools, 11343122 and 11340045). DCs were matured with anti-CD40 antibody and TNFα following previously established protocols.Citation44 The typical phenotype of DCs (immature or mature) after 9 d of culture was assured by staining with CD80, CD83, HLA-DR, CD1a (all markers positive), CD3 and CD19 (both negative), and CD14 (low or negative).
In vitro migration
Migration of B cells toward rmSDF1α/CXCL12, CXCL13, and CCL21 (all PrepoTech, 300-28A, 300-47 and 300-35) was assessed using a transwell migration assay. Chemokines were diluted to the concentrations of 10 ng/mL, 100 ng/mL, 500 ng/mL, and 1000 ng/mL in 600 μL muCD40B medium and preincubated for 20 min at 37°C before assay. 600 μL of muCD40B medium alone was used as negative control. Target cells were washed twice with PBS and resuspended in muCD40B medium at a density of 5 × 106/mL. 100 μL of this cell suspension was added to the upper chamber of a Costar transwell culture insert (Sigma, CLS3464-48EA) and incubated at 37°C for 3 h; the chemokines were added to the lower chamber. After 3 h, migrated cells were collected from the lower wells.
For analysis of T-cell migration, purified CD4+ T cells were resuspended in muCD40B medium to a concentration of 5 × 106/mL and 100 μL of this cell suspension were added to the upper chamber. 600 μL of murine CD40B supernatants were added to the lower chamber. CXCL12 (PrepoTech, 300-28A) at a concentration of 500 ng/mL was used as a positive control. After 2 h, migrated T cells were collected from the lower wells.
Migration assays in Collagen gels were performed in µ-Slides Chemotaxis3D (Ibidi, 80326) according to manufacturer's protocol.
In vivo migration
Murine freshly isolated CD19+ B cells or CD40B cells from DeleterCre/GFP mice were injected i.v. or s.c. into C57BL/6 mice. Mice were killed and spleen, lung, liver, axillary, and inguinal lymph nodes were removed. The primary antibodies Biotin anti-mouse CD45R/B220 (BD Biosciences, 553086) and anti-mouse CD3 (Acris Antibodies, SM1754P) and streptavidin-conjugated Cy3 and Cy5 (Jackson Immuno Research, 016-160-084 and 016-170-084) were used to stain the sections of organs. For negative controls, samples were stained only with streptavidin-conjugated antibodies. Analysis of the organs was performed using a Laser Scanning Microscope 510 Meta microscope (Zeiss, Oberkochen, Germany) with an LD Plan Neofluar 40 × 0.6 (dry). For documentation, images at a resolution of 1388 × 1040 pixels were acquired using the microscope Axiovert 200 M, with an objective A-Plan 10 × 0.25 Ph 1, and an AxioCamMR camera. GFP was excited at 488 nm and Cy3 at 543 nm. The following emission filters were used: BP 505-530 for GFP and HQ 620/60 for Cy3.
Murine freshly isolated CD19+ B cells or CD40B cells from Luc+ mice were injected i.v. or s.c. into C57BL/6 mice. Detection of Luc+ B cells was performed by injecting 7.5 mg D-Luciferin in 250 mL 1× PBS i.p. into wild type mice. The luciferin was allowed to distribute in the mouse for 5 min before mice were narcotized with 1.5–4% Insofluran. Mice were shaved prior to imaging in order to minimize interference by the fur. Imaging was performed in the Xenogen IVIS 200 (Perkin Elmer). Mice were constantly kept under narcosis with 1.5–4% Insofluran at 37°C. Bioluminescence pictures were analyzed with the Living Image Software (Perkin Elmer).
In vivo cytotoxicity assay
APCs were loaded with Ovalbumin (OVA)-protein for 1 h at 37°C before injecting 5 × 106 cells/mouse i.v. in 100 µL PBS. Mice were immunized three times in an interval of 7 d On day 21, OVA-peptide (SIINFEKL)-pulsed CFSE-labeled target cells were injected i.p. into immunized mice. Twenty-four hours later, spleens were removed and analyzed for killing of peptide-pulsed target cells. The ratio of unpulsed vs. pulsed (RatioUP) target cells was determined by dividing the percentage of CFSE low cells by the percentage of CFSE high cells. Specific lysis was calculated by the following formula:% Specific Lysis = (1-(RatioUP Negative Control/RatioUP Immunized))*100.
Flow cytometry
Cell phenotypes were evaluated using the following fluorophore-conjugated antibodies: anti-human CD3, CD8, CD19, CD80, and CD86 (BD Biosciences; 557596, 555658, 555415, 557226, 553692), CD14, CXCR4 and CD4 (Biolegend; 325617, 590202, 344620), CD20 (Life Technologies, MHCD2030), CD45RA (Beckman Coulter, IM27114); anti-mouse CXCR5, CD86, CD19, CD3e, CD4, CD80, and CD8a (BD Biosciences; 551959, 553692, 552854, 557596, 552775, 553768, 551162), B220 (Life Technologies, RM2627), H2Db and CD62L (Caltag Medsystems; MM3804, RM4304), I-Ab (Biolegend, 116417). 0.5 × 106 cells were washed twice with PBS and incubated with antibodies for 15 min at 4°C. The data were collected on a BD FACSCanto and on a Gallios flow cytometer (Beckman Coulter, Brea, CA, USA) and analyzed using FlowJo (Tree Star, Ashland, USA) or Kaluza (Beckman Coulter, Brea, CA, USA) software.
Analysis of cell-cell interactions in a 3D collagen matrix
Time-lapse video microscopy analysis of B-cell/DC–T-cell interactions within 3D collagen gels were performed as described previouslyCitation38 Briefly, human B cells or DCs were loaded with cyclin D1-derived peptide p228 (RLTRFLSRV; from Thermo Electron, Ulm, Germany) derived from cyclin D1 at 10 μg/mL and cocultivated with human CFSE-labeled CD8+ T cells from a T-cell clone (CTL clone 5B7) specific for cyclin D1Citation42 in 3D collagen gels at 37°C. Contact duration was monitored by time-lapse microscopy using an Olympus BX 61 microscope with a UAPO lens (20 ×/NA 0.75) and a FView camera with AnalySIS software from SIS (Münster, Germany). Data were acquired from 34 movies from 9 independent experiments. At least 100 contacts were scored for each condition.
Statistical analysis
Differences between mean values were calculated by the two-tailed Student's t test for unpaired data or by One-way Analysis of Variance (ANOVA) test, which was corrected by Dunnett multiple comparison correction if more than two groups were compared, using GraphPad Prism 4 (GraphPad Software, San Diego, CA, USA). A p value of less than 0.05 was considered statistically significant.
Disclosure of Potential Conflicts of Interest
No potential conflicts of interest were disclosed.
Supplemental Material
Supplemental data for this article can be accessed on the publisher's website
1038684_supplemental_movies__4__and_data.zip
Download Zip (6.4 MB)Funding
NKG was supported by Fundació La Caixa and the German Academic Exchange Service (DAAD). MvB-B was supported by a Max Eder Research Grant of the Deutsche Krebshilfe.
References
- Bousso P, Robey E. Dynamics of CD8+ T cell priming by dendritic cells in intact lymph nodes. Nat Immunol 2003; 4:579-85; PMID:12730692; http://dx.doi.org/10.1038/ni928
- Miller MJ, Safrina O, Parker I, Cahalan MD. Imaging the single cell dynamics of CD4+ T cell activation by dendritic cells in lymph nodes. J Exp Med 2004; 200:847-56; PMID:15466619; http://dx.doi.org/10.1084/jem.20041236
- Galluzzi L, Senovilla L, Vacchelli E, Eggermont A, Fridman WH, Galon J, Sautès-Fridman C, Tartour E, Zitvogel L, Kroemer G. Trial watch: dendritic cell-based interventions for cancer therapy. Oncoimmunology 2012; 1:1111-34; PMID:23170259; http://dx.doi.org/10.4161/onci.21494
- Kantoff PW, Higano CS, Shore ND, Berger ER, Small EJ, Penson DF, Redfern CH, Ferrari AC, Dreicer R, Sims RB et al. Sipuleucel-T immunotherapy for castration-resistant prostate cancer. N Engl J Med 2010; 363:411-22; PMID:20818862; http://dx.doi.org/10.1056/NEJMoa1001294
- Steinman RM, Banchereau J. Taking dendritic cells into medicine. Nature 2007; 449:419-26; PMID:17898760; http://dx.doi.org/10.1038/nature06175
- Ahmadi T, Flies A, Efebera Y, Sherr DH. CD40 ligand-activated, antigen-specific B cells are comparable to mature dendritic cells in presenting protein antigens and major histocompatibility complex class I- and class II-binding peptides. Immunology 2008; 124:129-40; PMID:18067555; http://dx.doi.org/10.1111/j.1365-2567.2007.02749.x
- Sorenmo KU, Krick E, Coughlin CM, Overley B, Gregor TP, Vonderheide RH, Mason NJ. CD40-activated B cell cancer vaccine improves second clinical remission and survival in privately owned dogs with non-Hodgkin's lymphoma. PLoS One 2011; 6:e24167; PMID:21904611; http://dx.doi.org/10.1371/journal.pone.0024167
- Carpenter EL, Mick R, Rüter J, Vonderheide RH. Activation of human B cells by the agonist CD40 antibody CP-870,893 and augmentation with simultaneous toll-like receptor 9 stimulation. J Transl Med 2009; 7:93; PMID:19906293; http://dx.doi.org/10.1186/1479-5876-7-93
- Von Bergwelt-Baildon MS, Vonderheide RH, Maecker B, Hirano N, Anderson KS, Butler MO, Xia Z, Zeng WY, Wucherpfennig KW, Nadler LM et al. Human primary and memory cytotoxic T lymphocyte responses are efficiently induced by means of CD40-activated B cells as antigen-presenting cells: potential for clinical application. Blood 2002; 99:3319-25; PMID:11964299; http://dx.doi.org/10.1182/blood.V99.9.3319
- Fujiwara H, Melenhorst JJ, El Ouriaghli F, Kajigaya S, Grube M, Sconocchia G, Rezvani K, Price DA, Hensel NF, Douek DC et al. In vitro induction of myeloid leukemia-specific CD4 and CD8 T cells by CD40 ligand-activated B cells gene modified to express primary granule proteins. Clin Cancer Res 2005; 11:4495-503; PMID:15958635; http://dx.doi.org/10.1158/1078-0432.CCR-04-2363
- Mason NJ, Coughlin CM, Overley B, Cohen JN, Mitchell EL, Colligon TA, Clifford CA, Zurbriggen A, Sorenmo KU, Vonderheide RH. RNA-loaded CD40-activated B cells stimulate antigen-specific T-cell responses in dogs with spontaneous lymphoma. Gene Ther 2008; 15:955-65; PMID:18337841; http://dx.doi.org/10.1038/gt.2008.22
- Park MY, Kim HS, Woo SJ, Kim CH, Park JS, Sohn HJ, Kim HJ, Oh ST, Kim TG. Efficient antitumor immunity in a murine colorectal cancer model induced by CEA RNA-electroporated B cells. Eur J Immunol 2008; 38:2106-17; PMID:18624349; http://dx.doi.org/10.1002/eji.200737960
- Kondo E, Gryschok L, Klein-Gonzalez N, Rademacher S, Weihrauch MR, Liebig T, Shimabukuro-Vornhagen A, Kochanek M, Draube A, von Bergwelt-Baildon MS. CD40-activated B cells can be generated in high number and purity in cancer patients: analysis of immunogenicity and homing potential. Clin Exp Immunol 2009; 155:249-56; PMID:19040609; http://dx.doi.org/10.1111/j.1365-2249.2008.03820.x
- Shimabukuro-Vornhagen A, Draube A, Liebig TM, Rothe A, Koc M, von Bergwelt-Baildon MS. The immunosuppressive factors IL-10, TGF-β, and VEGF do not affect the antigen-presenting function of CD40-activated B cells. J Exp Clin Cancer Res 2012; 31:47; PMID:22592077; http://dx.doi.org/10.1186/1756-9966-31-47
- Shimabukuro-Vornhagen A, Draube A, Liebig T, Popov A, Rothe A, von Bergwelt-Baildon M. The properties of human CD40-activated B cells as antigen-presenting cells are not affected by PGE2. Oncol Rep 2013; 29:1061-5; PMID:23292511; http://dx.doi.org/10.1038/gt.2008.22
- Bishop GA, Hostager BS. The CD40-CD154 interaction in B cell-T cell liaisons. Cytokine Growth Factor Rev 2003; 14:297-309; PMID:12787567; http://dx.doi.org/10.1016/S1359-6101(03)00024-8
- Von Bergwelt-Baildon M, Shimabukuro-Vornhagen A, Popov A, Klein-Gonzalez N, Fiore F, Debey S, Draube A, Maecker B, Menezes I, Nadler LM et al. CD40-activated B cells express full lymph node homing triad and induce T-cell chemotaxis: potential as cellular adjuvants. Blood 2006; 107:2786-9; PMID:16357329; http://dx.doi.org/10.1182/blood-2004-01-0113
- Shimabukuro-Vornhagen A, Zoghi S, Liebig TM, Wennhold K, Chemitz J, Draube A, Kochanek M, Blaschke F, Pallasch C, Holtick U et al. Inhibition of protein geranylgeranylation specifically interferes with CD40-dependent B cell activation, resulting in a reduced capacity to induce T cell immunity. J Immunol 2014; 193:5294-305; PMID:25311809; http://dx.doi.org/10.4049/jimmunol.1203436
- Gunzer M, Weishaupt C, Hillmer A, Basoglu Y, Friedl P, Dittmar KE, Kolanus W, Varga G, Grabbe S. A spectrum of biophysical interaction modes between T cells and different antigen-presenting cells during priming in 3-D collagen and in vivo. Blood 2004; 104:2801-9; PMID:15256430; http://dx.doi.org/10.1182/blood-2004-03-1193
- Liebig TM, Fiedler A, Zoghi S, Shimabukuro-Vornhagen A, von Bergwelt-Baildon MS. Generation of human CD40-activated B cells. J Vis Exp [Internet] 2009; PMID:19838159; http://dx.doi.org/10.3791/1373. Available from: http://www.ncbi.nlm.nih.gov/entrez/query.fcgi?cmd=Retrieve&db=PubMed&dopt=Citation&list_uids=19838159
- Liebig TM, Fiedler A, Klein-Gonzalez N, Shimabukuro-Vornhagen A, von Bergwelt-Baildon M. Murine model of CD40-activation of B cells. J Vis Exp [Internet] 2010 [cited 2010 Mar 30]; PMID:20208476; http://dx.doi.org/10.3791/1734. Available from: http://www.ncbi.nlm.nih.gov/pubmed/20208476
- Cyster JG. Chemokines and cell migration in secondary lymphoid organs. Science 1999; 286:2098-102; PMID:10617422; http://dx.doi.org/10.1126/science.286.5447.2098
- Stein JV, Nombela-Arrieta C. Chemokine control of lymphocyte trafficking: a general overview. Immunology 2005; 116:1-12; PMID:16108812; http://dx.doi.org/10.1111/j.1365-2567.2005.02183.x
- Reif K, Ekland EH, Ohl L, Nakano H, Lipp M, Förster R, Cyster JG. Balanced responsiveness to chemoattractants from adjacent zones determines B-cell position. Nature 2002; 416:94-9; PMID:11882900; http://dx.doi.org/10.1038/416094a
- Jeanbart L, Ballester M, de Titta A, Corthésy P, Romero P, Hubbell JA, Swartz MA. Enhancing efficacy of anticancer vaccines by targeted delivery to tumor-draining lymph nodes. Cancer Immunol Res 2014; 2:436-47; PMID:24795356; http://dx.doi.org/10.1158/2326-6066.CIR-14-0019-T
- Von Andrian UH, Mempel TR. Homing and cellular traffic in lymph nodes. Nature reviews 2003; 3:867-78; PMID:14668803; http://dx.doi.org/10.1038/nri1222
- De Vries IJ, Krooshoop DJ, Scharenborg NM, Lesterhuis WJ, Diepstra JH, Van Muijen GN, Strijk SP, Ruers TJ, Boerman OC, Oyen WJ, et al. Effective migration of antigen-pulsed dendritic cells to lymph nodes in melanoma patients is determined by their maturation state. Cancer research 2003; 63:12-7; PMID:12517769
- Eggert AA, Schreurs MW, Boerman OC, Oyen WJ, de Boer AJ, Punt CJ, Figdor CG, Adema GJ. Biodistribution and vaccine efficiency of murine dendritic cells are dependent on the route of administration. Cancer Res 1999; 59:3340-5; PMID:10416590
- Huck SP, Tang S-C, Andrew KA, Yang J, Harper JL, Ronchese F. Activation and route of administration both determine the ability of bone marrow-derived dendritic cells to accumulate in secondary lymphoid organs and prime CD8+ T cells against tumors. Cancer Immunol Immunother 2008; 57:63-71; PMID:17609951; http://dx.doi.org/10.1007/s00262-007-0350-z
- Kupiec-Weglinski JW, Austyn JM, Morris PJ. Migration patterns of dendritic cells in the mouse. Traffic from the blood, and T cell-dependent and -independent entry to lymphoid tissues. J Exp Med 1988; 167:632-45; PMID:3258009; http://dx.doi.org/10.1084/jem.167.2.632
- Morse MA, Coleman RE, Akabani G, Niehaus N, Coleman D, Lyerly HK. Migration of human dendritic cells after injection in patients with metastatic malignancies. Cancer Res 1999; 59:56-8; PMID:9892184
- Robert C, Klein C, Cheng G, Kogan A, Mulligan RC, von Andrian UH, Kupper TS. Gene therapy to target dendritic cells from blood to lymph nodes. Gene Ther 2003; 10:1479-86; PMID:12900763; http://dx.doi.org/10.1038/sj.gt.3302008
- Scandella E, Fink K, Junt T, Senn BM, Lattmann E, Förster R, Hengartner H, Ludewig B. Dendritic cell-independent B cell activation during acute virus infection: a role for early CCR7-driven B-T helper cell collaboration. J Immunol 2007; 178:1468-76; PMID:17237395; http://dx.doi.org/10.4049/jimmunol.178.3.1468
- Okada T, Miller MJ, Parker I, Krummel MF, Neighbors M, Hartley SB, O’Garra A, Cahalan MD, Cyster JG. Antigen-engaged B cells undergo chemotaxis toward the T zone and form motile conjugates with helper T cells. PLoS Biol 2005; 3:e150; PMID:15857154; http://dx.doi.org/10.1371/journal.pbio.0030150
- Ekland EH, Forster R, Lipp M, Cyster JG. Requirements for follicular exclusion and competitive elimination of autoantigen-binding B cells. J Immunol 2004; 172:4700-8; PMID:15067045; http://dx.doi.org/10.4049/jimmunol.172.8.4700
- Gunzer M, Schäfer A, Borgmann S, Grabbe S, Zänker KS, Bröcker EB, Kämpgen E, Friedl P. Antigen presentation in extracellular matrix: interactions of T cells with dendritic cells are dynamic, short lived, and sequential. Immunity 2000; 13:323-32; PMID:11021530; http://dx.doi.org/10.1016/S1074-7613(00)00032-7
- Varga G, Balkow S, Wild MK, Stadtbaeumer A, Krummen M, Rothoeft T, Higuchi T, Beissert S, Wethmar K, Scharffetter-Kochanek K et al. Active MAC-1 (CD11b/CD18) on DCs inhibits full T-cell activation. Blood 2007; 109:661-9; PMID:17003381; http://dx.doi.org/10.1182/blood-2005-12-023044
- Balkow S, Heinz S, Schmidbauer P, Kolanus W, Holzmann B, Grabbe S, Laschinger M. LFA-1 activity state on dendritic cells regulates contact duration with T cells and promotes T-cell priming. Blood 2010; 116:1885-94; PMID:20530790; http://dx.doi.org/10.1182/blood-2009-05-224428
- Lanzavecchia A, Sallusto F. Regulation of T cell immunity by dendritic cells. Cell 2001; 106:263-6; PMID:11509174; http://dx.doi.org/10.1016/S0092-8674(01)00455-X
- Faroudi M, Zaru R, Paulet P, Müller S, Valitutti S. Cutting edge: T lymphocyte activation by repeated immunological synapse formation and intermittent signaling. J Immunol 2003; 171:1128-32; PMID:12874197; http://dx.doi.org/10.4049/jimmunol.171.3.1128
- Matheu MP, Su Y, Greenberg ML, Blanc CA, Parker I, Scott DW, Cahalan MD. Toll-like receptor 4-activated B cells out-compete Toll-like receptor 9-activated B cells to establish peripheral immunological tolerance. Proc Natl Acad Sci USA 2012; 109:E1258-66; PMID:22511718; http://dx.doi.org/10.1073/pnas.1205150109
- Kondo E, Maecker B, Weihrauch MR, Wickenhauser C, Zeng W, Nadler LM, Schultze JL, von Bergwelt-Baildon MS. Cyclin D1-specific cytotoxic T lymphocytes are present in the repertoire of cancer patients: implications for cancer immunotherapy. Clin Cancer Res 2008; 14:6574-9; PMID:18927298; http://dx.doi.org/10.1158/1078-0432.CCR-08-0825
- Shimabukuro-Vornhagen A, Liebig TM, Koslowsky T, Theurich S, von Bergwelt-Baildon MS. The ratio between dendritic cells and T cells determines whether prostaglandin E2 has a stimulatory or inhibitory effect. Cell Immunol 2013; 281:62-7; PMID:23454682; http://dx.doi.org/10.1016/j.cellimm.2013.01.001
- Von Bergwelt-Baildon MS, Popov A, Saric T, Chemnitz J, Classen S, Stoffel MS, Fiore F, Roth U, Beyer M, Debey S, et al. CD25 and indoleamine 2,3-dioxygenase are up-regulated by prostaglandin E2 and expressed by tumor-associated dendritic cells in vivo: additional mechanisms of T-cell inhibition. Blood 2006; 108:228-37; PMID:16522817; http://dx.doi.org/10.1182/blood-2005-08-3507